- Institute of Molecular Pathobiochemistry, Experimental Gene Therapy and Clinical Chemistry, RWTH University Hospital Aachen, Aachen, Germany
Lipocalin 2 (LCN2) is a secreted protein that belongs to the Lipocalins, a group of transporters of small lipophilic molecules such as steroids, lipopolysaccharides, iron, and fatty acids in circulation. Two decades after its discovery and after a high variety of published findings, LCN2's altered expression has been assigned to critical roles in several pathological organ conditions, including liver injury and steatosis, renal damage, brain injury, cardiomyopathies, muscle-skeletal disorders, lung infection, and cancer in several organs. The significance of this 25-kDa lipocalin molecule has been impressively increased during the last years. Data from several studies indicate the role of LCN2 in physiological conditions as well as in response to cellular stress and injury. LCN2 in the liver shows a protective role in acute and chronic injury models where its expression is highly elevated. Moreover, LCN2 expression is being considered as a potential strong biomarker for pathological conditions, including rheumatic diseases, cancer in human organs, hepatic steatosis, hepatic damage, and inflammation. In this review, we summarize experimental and clinical findings linking LCN2 to the pathogenesis of liver disease.
Introduction
LCN2 has many synonyms including neutrophil gelatinase associated lipocalin (NGAL), 24p3, oncogenic lipocalin, siderocalin, 25 kDa α2-microglobulin-related protein, and uterocalin. The different names are justified by predicting functions of LCN2 or tissue in which the expression was first detected. LCN2 belongs to the broad family of lipocalins that were first proposed to have unifying functions in the transport of hydrophobic substances. This ever expanding family of proteins share limited regions of sequence homology and a common tertiary structure architecture. Details on the structure, characteristics, and most known functions of members of the lipocalin family were highlighted in a previous report (Asimakopoulou and Weiskirchen, 2015).
Human LCN2 was initially isolated and purified by Kjeldsen and coworkers as a 25-kDa neutrophil protein (Table 1), that is in part associated with gelatinase from human neutrophils (Kjeldsen et al., 1993). One year later, the same group cloned the full length human LCN2 cDNA and showed that this lipocalin is mainly expressed in myeloid cells (Bundgaard et al., 1994) and later assigned to a cluster of at least three lipocalins on the long arm of human chromosome 9 by in situ hybridization that is syntenic to a region of mouse chromosome 4 (Chan et al., 1994).
The first critical roles given to LCN2 were proposed from its “lipocalin structure” that is most closely related to the structures of the epididymal retinoic acid-binding proteins and the major urinary protein (Goetz et al., 2000). The typical unifying three dimensional fold of lipocalins encompasses an eight-stranded, anti-parallel, symmetrical β-barrel fold with a cylindrical shape (Figure 1).
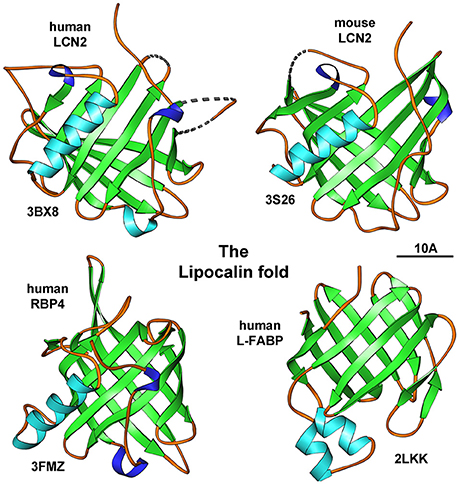
Figure 1. The lipocalin fold. Members of the lipocalin family have a typical eight-stranded, anti-parallel, symmetrical β-barrel fold structure. Depicted are human and mouse LCN2, human retniol-binding protein 4 (RBP4), and human liver fatty acid-binding protein (L-FABP). The depicted structures were generated using the Ribbons XP software (version 3.0) and coordinates 3BX8, 3S26, 3FMZ, and 2LKK deposited in the RCSB Protein Data Bank (http://www.rcsb.org). A size marker (10 Å) is given.
Later it was shown that murine and human LCN2 are both iron-trafficking proteins that interfere with the activity of iron-responsive genes (Yang et al., 2002). The initial finding that LCN2 is involved in iron delivery pointed to a potential key role of LCN2 in immunity. This assumption was further confirmed in experiments showing that LCN2 has the capacity to limit bacterial growth by sequestering the iron-laden siderophore after infection in mice (Flo et al., 2004; Holmes et al., 2005; Berger et al., 2006). After this very first allocation of LCN2 as a significant factor in guaranteeing immune balance, the repertoire of LCN2 functions was further expanded to many biological processes, including inflammation, intoxication, immune defense, organogenesis, and cancer.
In this review, we will summarize some of the major findings of LCN2 in the pathogenesis of organ disease with a special emphasis on inflammatory liver diseases.
Regulation of LCN2
LCN2 starts being expressed in the embryonic stage (Mallbris et al., 2002) and it has been shown to be activated strongly in inflamed organs such as liver, heart, lungs, bone marrow, kidney, and spleen (Aigner et al., 2007; Borkham-Kamphorst et al., 2011). LCN2 expression is also induced during neutrophil maturation (Kjeldsen et al., 1993). It has been demonstrated to decrease at an older age, especially in the kidney and liver. Further, LCN2 is dramatically increased in cells by addition of dexamethasone and retinoic acid (Garay-Rojas et al., 1996). During bacterial infection, the expression of LCN2 has been proved to be upregulated in several tissues such as liver (Xu et al., 2015), diverse mucosal tissues (Kjeldsen et al., 2000), lung (Chan et al., 2009), and epithelial tissue (Friedl et al., 1999). In all these tissues LCN2 behaves as an acute phase protein. At sites of infection LCN2 is secreted by neutrophils, macrophages, activated leukocytes, and adipocytes (Kjeldsen et al., 1993; Meheus et al., 1993; Flo et al., 2004). The different reports unanimously show that LCN2 is either increased in the tissue by several resident cell types or alternatively by circulating immune cells that are recruited into the inflamed tissue.
In in vitro and in vivo experimentation, LCN2 was found to be induced by several factors such as lipopolysaccharide (LPS), cytokines, retinoic acid, growth factors, and insulin (Meheus et al., 1993; Sorensen et al., 2003; Bu et al., 2006; Sunil et al., 2007; Tan et al., 2009; Hamzic et al., 2013). LPS has been shown to be a strong inducer of LCN2 in liver and lungs (Sunil et al., 2007; Borkham-Kamphorst et al., 2013). The stimulatory capacity of LPS in mice is extremely high when compared to other hepatotoxins such as carbon tetrachloride and Concanavalin A (Borkham-Kamphorst et al., 2013). There are several other biomolecules and pathways known to contribute to the expression of LCN2 in inflammatory cells, macrophage cell lines, epithelial cells, breast cancer cell lines, and hepatocytes (Meheus et al., 1993; Seth et al., 2002; Bu et al., 2006; Borkham-Kamphorst et al., 2011; Kienzl-Wagner et al., 2015; Xu et al., 2015). Cytokines that induce the expression of LCN2 include the interleukins IL-6, IL-1β, IL-10, IL-17, tumor necrosis factor (TNF-α), and tumor growth factor (TGF-α) (Sorensen et al., 2003; Bu et al., 2006; Borkham-Kamphorst et al., 2011; Hamzic et al., 2013; Vazquez et al., 2015; Xu et al., 2015). The nuclear factor-κB (NF-κB) transcription activity seems to be the main pathway involved in LCN2 stimulation (Bu et al., 2006). The close linkage to the NF-κB pathway and diverse pro-inflammatory cytokines that act through the activation of NF-κB was also found in different models of hepatic injury (Borkham-Kamphorst et al., 2011).
Up to date, LCN2 has been found to bind only on two receptors. Hvidberg and colleagues first described that LCN2 binds to Megalin, representing a member of the low-density lipoprotein receptor family (Hvidberg et al., 2005) while another study described a 24p3R receptor of LCN2 that confers the ability to undergo either iron uptake or apoptosis (Devireddy et al., 2005). Although the concept that LCN2 does induce iron efflux or stimulate apoptosis was somewhat challenged (Correnti et al., 2012), a more recent fundamental study performed with human homolog LCN2R (also known as SLC22A17) demonstrated that the N-terminal part of this receptor has a soluble extracellular domain that is intrinsically disordered with the capacity to preferentially interact with LCN2 in its non-iron bound form suggesting that this cellular receptor might discriminate between the iron-free (apo-) and iron-bound (holo-) form of LCN2 (Cabedo Martinez et al., 2016).
LCN2 Pathophysiological Expression in Tissue
LCN2 is reported to be expressed in numerous pathological and experimental-induced conditions in many organs and tissues such as liver, kidney, lungs, bone, brain, and heart (Figure 2). Several of the published findings on main organs are listed in Table 2.
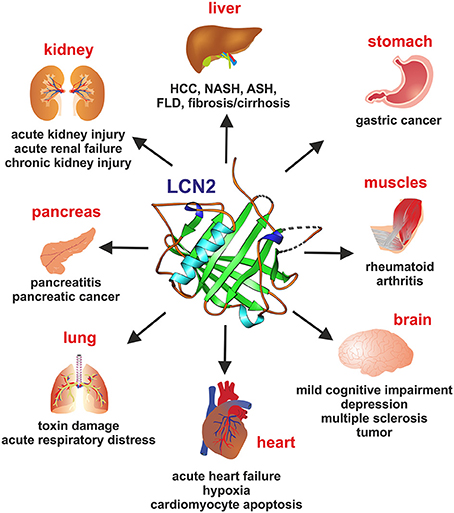
Figure 2. Lipocalin 2 (LCN2) in organ damage. Representative pathophysiological or malignancies conditions for which elevated LCN2 concentrations in representative organs are indicative are depicted.
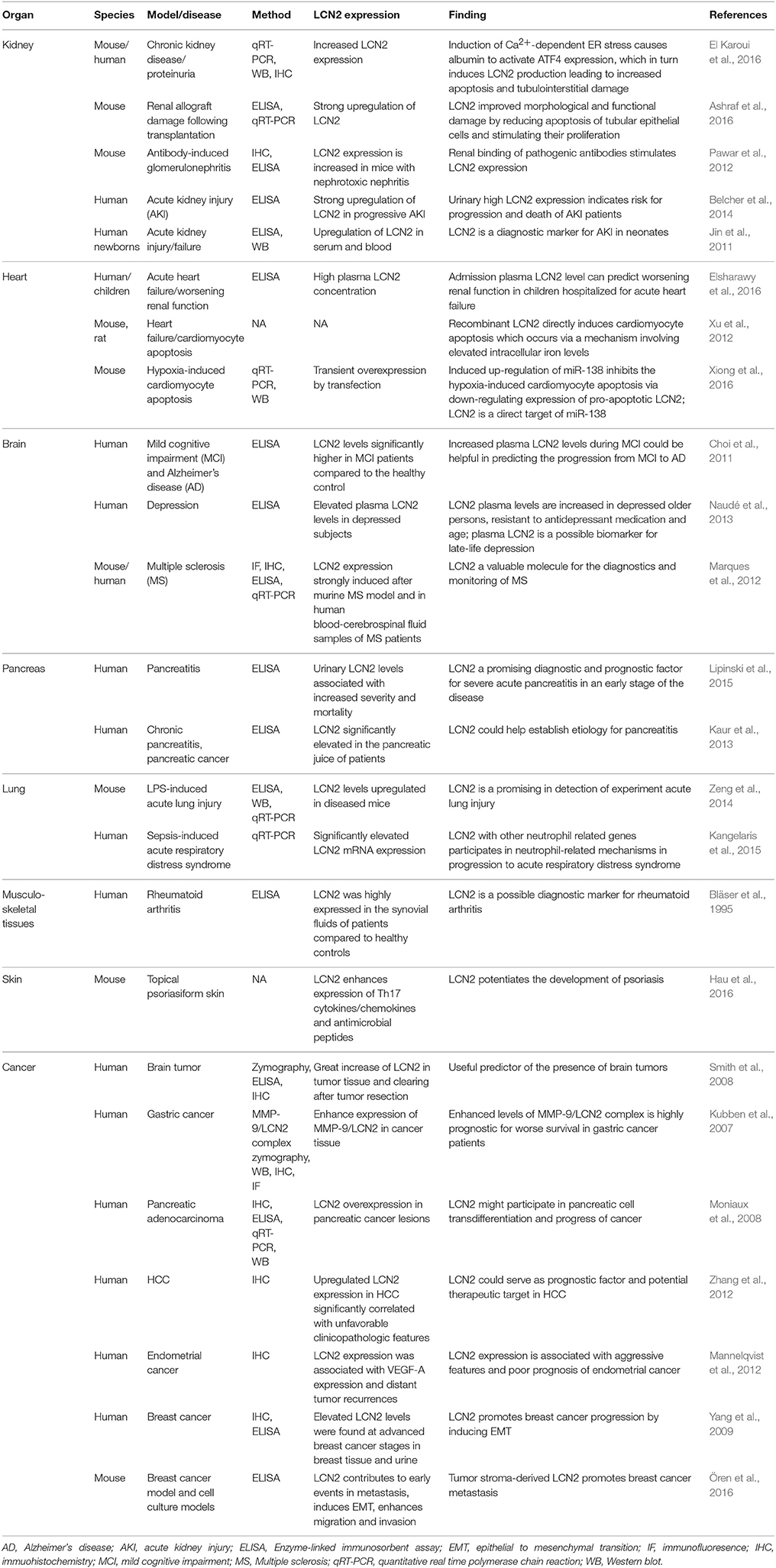
Table 2. Selected experimental and clinical findings associated with altered LCN2 expression in organ disorders.
Here we will first focus on some of the most recent reports on LCN2 expression and organ disorders to understand the possible major functions of LCN2 and subsequently highlight the importance of LCN2 in the pathogenesis of hepatic disease.
During the last years, LCN2 has been well-studied as a potential biomarker for kidney injury or to estimate the outcome of respective diseases (Coca et al., 2008; Heyne et al., 2012; Paragas et al., 2012; Barreto et al., 2014; Matsa et al., 2014). In one multicenter, prospective cohort study, a significant correlation between urinary injury biomarkers (LCN2, IL-18, KIM-1, L-FABP, and albuminuria) and disease outcome was found in which LCN2 only distinguished progression alone from no progression, suggesting that LCN2 as other proteins with similar behavior could potentially serve to identify patients at highest risk of progression and death (Belcher et al., 2014). More recently a systematic review and meta-analysis on LCN2 in acute kidney injury (AKI) or failure investigating more than 50 studies concluded that blood LCN2 could be used as a diagnostic marker for AKI in newborns (Jiang and Cui, 2015). Moreover, several investigators have suggested urinary LCN2 as an early diagnostic marker in renal impairment and AKI in chronic cirrhosis (Gerbes et al., 2011; Fagundes et al., 2012; Verna et al., 2012; Wong and Murray, 2014).
In addition to acute injury in the kidney, another study reported an active critical role of LCN2 activity in the formation of chronic kidney disease (Viau et al., 2010). In this report, it was found in experimental models of chronic kidney disease in mice from different genetic backgrounds, that the severity of renal lesions was strongly dependent on LCN2 that significantly correlated with hyperproliferation and disease progression in both mice and humans. Moreover, the authors found that LCN2 mediates the mitogenic effects of EGFR that critically predicts the severity of renal lesions after nephron reduction. These findings gave LCN2 a critical role in the pathogenetic pathways that lead to progressive renal failure and cystogenesis (Viau et al., 2010). In extension to their observation, the same group recently showed that ATF4 is mandatory for the efficient induction of LCN2 in the course of albumin-induced tubular damage (El Karoui et al., 2016). In this condition, the high concentration of LCN2 induces endoplasmic reticulum stress-induced apoptosis leading to chronic kidney disease, proteinuria and severe tubulointerstitial damage, which ultimately leads to end-stage renal disease (El Karoui et al., 2016). The authors proposed a model of tubulointerstitial injury caused by proteinuria where during endoplasmic reticulum (ER) stress, albumin activates the activating transcription factor 4 (ATF4) which in turn induces LCN2 production that leads further to apoptosis and tubulointerstitial damage. Most interestingly, providing mouse models and human clinical data, it was conclusively proved that 4-phenylbutyric acid that is used as a drug in humans to allow the kidneys to excrete excess nitrogen, has the ability to reduce ER stress thereby preventing proteinuria-induced renal lesions and LCN2 overexpression. The inhibition of LCN2 in this pathway reduced mortality and protected kidneys from degradation, morphological and functional (El Karoui et al., 2016). Similarly, a crucial role of LCN2 was proven in a mouse model of nephrotoxic nephritis in which the injection of LCN2 promoted inflammation, apoptosis, and exacerbated nephritis (Pawar et al., 2012).
In contrast to these findings, another study investigating acute allograft rejection in mice, showed that the treatment with recombinant LCN2 after transplantation reduced the extent of kidney allograft rejection, renal allograft damage, apoptosis of tubular epithelial cells, and induced their proliferation. The proliferation led to an amelioration of damage morphology and improved allograft function (Ashraf et al., 2016). These two studies paradigmatically demonstrate that LCN2 is a versatile biomolecule with outstanding pathophysiological, diagnostically, and potential therapeutic relevance.
The heart and kidney have numerous similarities and their relationship makes it understandable that renal dysfunction/failure often accompanies cardiac dysfunction/failure and the opposite (Shah and Greaves, 2010). Except the significant role that LCN2 seems to play in renal disorders, studies on cardiomyopathies indicate a wider role of LCN2. So far it was known that acute heart failure is frequently associated with worsening renal function in adult patients. Although investigations on the role of LCN2 in the heart have only recently begun, a very recent report showed that plasma LCN2 levels can also predict worsening renal function in hospitalized children with acute heart failure (Elsharawy et al., 2016). Mechanistically, a recent review on cardiomyopathies discusses how LCN2 can regulate heart failure based on iron overload and iron deficiency (Chan et al., 2015). The authors highlight that LCN2 is a very critical factor involved in regulating various cellular mechanisms including oxidative stress, mitochondrial dysfunction, ER stress, and autophagy that all can contribute to cardiac dysfunction when perturbed and optimal iron levels are absent (Chan et al., 2015).
Based on the role of LCN2 in iron transport, it was also speculated that circulating LCN2 levels may reflect the body's iron status in haemodialysis patients (Bolignano et al., 2009). LCN2 leads to cardiomyocyte apoptosis by causing intracellular iron accumulation (Xu et al., 2012). On the other side, the up-regulation of the miR-138 gene inhibits the hypoxia-induced cardiomyocyte apoptosis via down-regulating of LCN2 acting pro-apoptotic (Xiong et al., 2016). All these data indicate that LCN2 is a very sensitive regulator of cell survival and apoptosis in cardiomyocytes. In addition, these strong indications of LCN2's correlation to heart disorders makes further elucidation of LCN2 role in heart failure a very interesting and crucial topic to study.
Several conflicting results on the role of LCN2 in neurological processes are published. Some studies point to its neurodeleterious effects, while others indicate neuroprotection. In a clinical study that enrolled 41 patients with mild cognitive impairment (MCI), 62 patients with Alzheimer's disease and 38 healthy elderly control subjects, it was found that MCI, which is a prodromal-inflammation stage of Alzheimer's disease, is correlated with elevated plasma LCN2 levels (Choi et al., 2011). Depression was also associated with LCN2 plasma levels in a cohort of 350 depressed persons that were compared to 129 non-depressed older subjects (Naudé et al., 2013). The study further revealed that the concentration of LCN2 was higher in patients with a recurrent depression compared to those with a first episode and independent of antidepressant treatment. A study performed in an experimental model of multiple sclerosis (MS) in mice and also analyzing cerebrospinal fluid samples taken from human patients suffering from MS showed increased LCN2 levels in the active phases of experimental autoimmune encephalomyelitis and in MS patients when compared to healthy individuals. Remarkably, the increase of LCN2 levels in the experimental animal model was reverted by the humanized monoclonal antibody Natalizumab that in humans is used for MS treatment supporting the notion that LCN2 measurement should be included in the diagnostic panel of markers for MS (Marques et al., 2012). However, the precise impact of LCN2 in the brain is still in the very beginning and further studies are required to unravel its roles in the nervous system.
Additionally, LCN2 has been studied in the endocrine gland disorders. In 2013 a study had strongly suggested LCN2 in the alkaline pancreatic juice as a marker for differentiating diseased from non-diseased pancreas and that LCN2 could contribute to understanding the etiology of pancreatitis (Kaur et al., 2013). Similarly, a study investigating the urinary levels of LCN2 in a cohort of more than 100 patients with inflammation of the pancreas found that the levels of LCN2 correlated with the severity of acute pancreatitis and mortality rate indicating that LCN2 could also be useful as biomarker for acute pancreatitis (Lipinski et al., 2015).
Preliminary studies performed in mice also point to a diagnostic role of LCN2 in LPS-induced septic acute lung injury (Zeng et al., 2014). In the same line, an investigation analyzing early sequence of events leading to the development of the acute respiratory distress syndrome, representing a common complication of sepsis found that the mRNA levels of LCN2 and other genes involved in the initial neutrophil response are upregulated in acute respiratory distress syndrome in respective patients (Kangelaris et al., 2015). These studies on LCN2 show that analyzing functional aspects of this lipocalin could also help to understand the pathogenesis or progression of lung diseases.
Moreover, strong indications of functions of LCN2 have been identified in musculoskeletal diseases. Bläser and colleagues reported in 1995 that very high concentrations of LCN2 were found in the synovial fluids (viscous fluid present in the cavities of movable joints) of patients suffering from inflammatory rheumatoid arthritis (Bläser et al., 1995). LCN2 was also proposed in a study analyzing a murine model of cartilage degradation a biomarker to estimate the severity of degeneration and inflammation (Wilson et al., 2008). In addition, LCN2 transgenic mice were marked by a smaller phenotype, presented bones micro-architectural changes in both endochondrial and intramembranous bones, and further showed a reduced deposition in the osteoblast bone matrix and impairment in expansion of bone marrow cavity (Costa et al., 2013).
Dysbalanced LCN2 expression has also been associated with cancer formation and progression in several organs. According to numerous studies in which LCN2 was quantitated by immunological methods such as immunohistochemistry, ELISA, gel zymography, and Western blots in urine, LCN2 has been proposed as an early marker of bladder, brain and endometrial cancer (Monier et al., 2000, 2002; Roy et al., 2008; Smith et al., 2008; Mannelqvist et al., 2012). Moreover, it was suggested to serve as a diagnostic marker for oesophageal and breast cancer progression or metastasis, gastric cancer and pancreatic tumors when measured as an individual biomarker or in a complex with other proteins (Kubben et al., 2007; Zhang et al., 2007; Moniaux et al., 2008; Yang et al., 2009; Du et al., 2011; Ören et al., 2016). In renal and thyroid solid tumors, LCN2 showed a positive correlation with malignant phenotypes (Iannetti et al., 2008; Barresi et al., 2010, 2012a,b). In ovarian solid tumors, LCN2 was associated with cancer differentiation, whereas LCN2 was also proved to be correlated with hepatocarcinoma and lung tumor progression (Friedl et al., 1999; Zhang et al., 2012). Even though LCN2 is suggested to have a role in the early stages of tumor development or as a prognostic marker, there is an urgent need for elucidating the role of LCN2 by studying its effects on metastasis behavior. Of course, the multitude of pathological events (cancer, inflammation, metastasis, organ failure) and affected organs (kidney, liver, heart, brain, pancreas, muscle) that give rise to alterations in LCN2 serum or urine levels may further require the definition of clear cut-off values that are indicative for a specific disease.
Hepatic LCN2 Expression
During the last years, LCN2 has been studied widely as a possible non-invasive biomarker for several organ disorders, as outlined above. Hepatic expression of LCN2 in disease is the most intensively studied chapter with almost 1/4 of the published findings targeting the roles of LCN2 in liver homeostasis. The liver is the vital organ for fat and carbohydrate metabolism. It is the body's production facility for many metabolites, hormones, and proteins that also detoxifies substances and excretes metabolites. Thus, any functional impairment in liver affects undesirably the whole organism. Experimental and clinical findings indicating altered hepatic LCN2 expression in numerous disease conditions are given in Figure 3 whereas the most recent findings are gathered in Table 3.
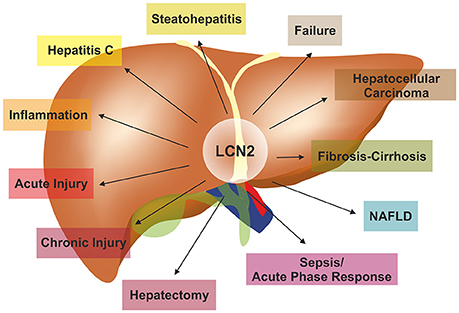
Figure 3. LCN2 in the pathogenesis of liver diseases. LCN2 is a versatile adiponectin that influences all kinds of liver diseases. For more details on experimental and clinical findings associated with altered LCN2 expression refer to Table 3.
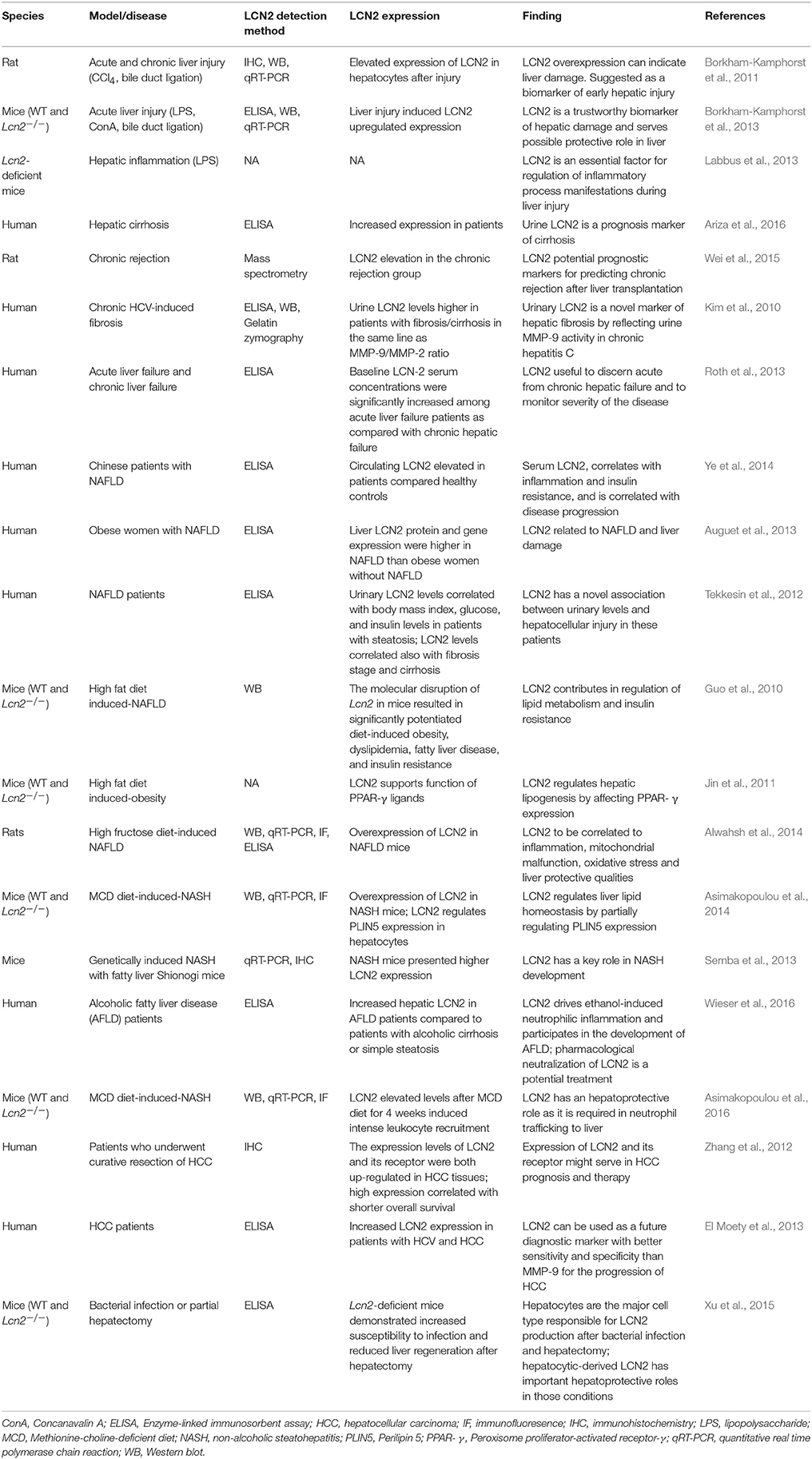
Table 3. Selected experimental and clinical findings associated with altered LCN2 expression in liver.
To sum up, alterations in LCN2 expression and functions are reported in many hepatic conditions, including fatty liver, hepatic inflammation, hepatitis C, hepatic regeneration, acute and chronic liver injury, cirrhosis, and liver failure. In the following we will discuss some of the most striking observations that were placed in context with LCN2.
Hepatic Injury—Inflammation
Based on experimental models of hepatic injury in which mice received injections of carbon tetrachloride (CCl4) or rats were subjected to bile duct ligation, a marked increase in LCN2 expression was found (Borkham-Kamphorst et al., 2011). The increase of LCN2 expression was correlated with liver damage and was inducible in cultured hepatocytes by diverse inflammatory stimuli, suggesting that LCN2 is as an early biomarker of liver inflammation (Borkham-Kamphorst et al., 2011; Labbus et al., 2013). In line, Ariza and colleagues found in a cohort of more than 700 patients with cirrhosis that urine LCN2 was markedly increased in the patients when compared to healthy control samples, establishing urine LCN2 as a prognostic marker of cirrhosis (Ariza et al., 2016). Most interestingly, in this study it was demonstrated that the measurement of urine LCN2 had a better predictive power than the measurement of serum LCN2 in discriminating acute-on-chronic liver failure (ACLF) patients from cirrhotic patients with acute decompensation. The authors suggested that this is due to the fact that the association between urine LCN2 and ACLF was not exclusively related to kidney function and that further urine but not serum LCN2 is independently associated with ACLF.
Similarly, the quantities of LCN2 in fresh spot urine samples were found to correlate with fibrosis score and reflected the increased activity of matrix metalloproteinase-9 (MMP-9) in urine in patients suffering from chronic hepatic C infection (Kim et al., 2010). Unfortunately, this study correlated LCN2 levels only to the grade of fibrosis as assessed according to the METAVIR fibrosis score in only 18 patients that had no or mild fibrosis and 24 patients with significant fibrosis, while the amount of inflammation (i.e., the activity) within the diseased liver was not considered. In a subsequent study, that included a total of n = 192 patients with chronic liver diseases of any etiology and healthy controls showed that LCN2 is a reliable indicator of liver damage that is positively correlated with inflammation, but is not correlated with the degree of liver fibrosis per se (Borkham-Kamphorst et al., 2013). In line with these findings, it was proposed that LCN2 serum levels may be diagnostically useful to discern acute from chronic hepatic failure (Roth et al., 2013). However, a recent report showed that LCN2 in plasma and urine are both suitable as independent predictive factors of acute-on-chronic liver failure that correlates with liver failure and systemic inflammation (Ariza et al., 2016).
Compared to normal controls, the livers of LCN2 deficient mice were more injured when exposed to short term application of CCl4, LPS and Concanavalin A, or subjected to bile duct ligation. This was reflected by elevated levels of aminotransferases and increased expression of inflammatory cytokines such as IL-1β, IL-6, and TNF-α which in turn resulted in prolonged NF-κB activation and sustained activation of STAT1, STAT3, and JNK pathways in hepatocytes. Based on these findings, it was supposed that as a counterpart to all these observations LCN2 has also hepatoprotective roles in phases of acute liver injury (Borkham-Kamphorst et al., 2013). In the same study, it was noted that hepatocytes of mice that lack LCN2 showed lipid droplet accumulation and increased apoptosis giving a slight hint of LCN2 as a modulating factor in lipid metabolism. In the same study it was demonstrated that although patients with chronic liver disease exhibited significantly higher concentrations compared to healthy volunteers, the levels of LCN2 were not suitable to discriminate between non-cirrhotic and cirrhotic liver-diseased patients, suggesting that LCN2 levels provide no correlation to the degree of liver fibrosis but provide a significant positive correlation to inflammation.
Fatty Liver Disease and Steatohepatitis
Fatty liver disease (FLD) is the abnormal hepatic fat accumulation (steatosis) that can also progress to fat accumulation combined with inflammation in the liver (steatohepatitis). In principle, there are three types of fatty liver. First, non-alcoholic fatty liver disease (NAFLD), a condition with prevalence up to 1–3 in western societies, where liver accumulates fat reaching more than 5% of the total liver weight because of factors independent of excessive alcohol intake (Baumeister et al., 2008; Bellentani et al., 2010; Fotbolcu and Zorlu, 2016). NAFLD is not just a liver disease as it is associated with cardiovascular disease, chronic kidney disease, type 2 diabetes, obesity, and dyslipidemia (Fotbolcu and Zorlu, 2016). NAFLD can progress to non-alcoholic steatohepatitis (NASH) where lipid accumulation is accompanied by inflammation (steatohepatitis) that cause irreversible hepatic damage (Neuman et al., 2016). Second, alcoholic fatty liver disease (AFLD), the second most common fatty liver condition, is as the name reveals the toxic accumulation of fat as a result of long-term excessive alcohol consumption. AFLD appears with smaller prevalence than NAFLD, but in patients both conditions lead to hepatic structural changes, obesity, metabolic disorders, and abnormal glucose tolerance (Reddy and Rao, 2006; Kotronen et al., 2010; Lívero and Acco, 2016). The third most widespread form of FLD is the acute fatty liver of pregnancy, a life threatening complication, for both mother and fetus, which develops in the last months of pregnancy (Zhang Y. P. et al., 2016).
Although there is a high prevalence of all kinds of FLDs in modern societies, no reliable non-invasive method for disease assessment has been identified yet. Therefore, the histological examination of liver tissue after liver biopsy remains still the gold standard for evaluating the degree of hepatic necro-inflammation and fibrosis (Fierbinteanu-Braticevici et al., 2010). During the last years, the increase of the enzyme alanine transaminase (ALT) was commonly measured clinically as a non-invasive biomarker for FLD assessment. However, the specificity of this marker for FLD is rather low, because it is also indicative of hepatocellular abnormalities without distinction between damage and steatosis (Musana et al., 2004; Myers, 2009; Barr et al., 2010). There are numerous studies available that correlated LCN2 with obesity, insulin resistance and diabetes (Yan et al., 2007; Law et al., 2010) rendering LCN2 as a possible biomarker for assessment of FLD.
A study that investigated the development of NAFLD in Chinese subjects revealed a close correlation of serum LCN2 with the progression of the disease and the occurrence of insulin resistance (Ye et al., 2014). Similarly, the circulating levels of LCN2 and hepatic expression of LCN2 were significantly higher in female NAFLD patients than in women with severe obesity with non-significant liver disease (Auguet et al., 2013), again suggesting LCN2 as a good biomarker for assessment of NAFLD. This study also showed that the administration of TNF-α, IL-6, resistin, and adiponectin stimulated expression in HepG2 cells, confirming previous reports demonstrating that the expression of LCN2 is moderated by pro-inflammatory triggers (Borkham-Kamphorst et al., 2011). Moreover, in another study analyzing adult patients with NAFLD, urinary LCN2 levels correlated well with the body mass index, insulin resistance, and lipid profiles (Tekkesin et al., 2012).
In experiments in Lcn2-deficient mice fed with a high fat diet to induce experimental steatosis, it was shown that the lack of LCN2 expression significantly potentiated diet-induced obesity, dyslipidemia, FLD, and insulin resistance (Guo et al., 2010). In a subsequent study, it was demonstrated that LCN2 is a critical modulator of PPAR-γ activation at the level of the recruitment of coactivators/corepressors thereby impacting adipogenesis and lipogenesis in adipose tissue and liver (Jin et al., 2011).
The impact of LCN2 for the formation of obesity, inflammation, and obesity-associated metabolic dysfunction was also shown in rat models after feeding with a high fructose diet for 4–8 weeks. As in other models tested, the expression of LCN2 correlated with hepatic inflammation, mitochondrial malfunction, and oxidative stress (Alwahsh et al., 2014). We have demonstrated in a nutritional mouse model of NAFLD and in primary hepatocyte cell culture that LCN2 is directly linked to the intracellular formation and accumulation of hepatic lipid droplet accumulation partly via regulation of the lipid droplet protein Perilipin 5 (Asimakopoulou et al., 2014). Comparative analysis of wild type and Lcn2 deficient mice revealed that the Lcn2 lacking mice accumulated more lipids in their livers when fed a Methionine- and Choline-deficient diet (Asimakopoulou et al., 2014). Again, this finding indicates that LCN2 has an essential function in liver homeostasis and lipid metabolism (Figure 4).
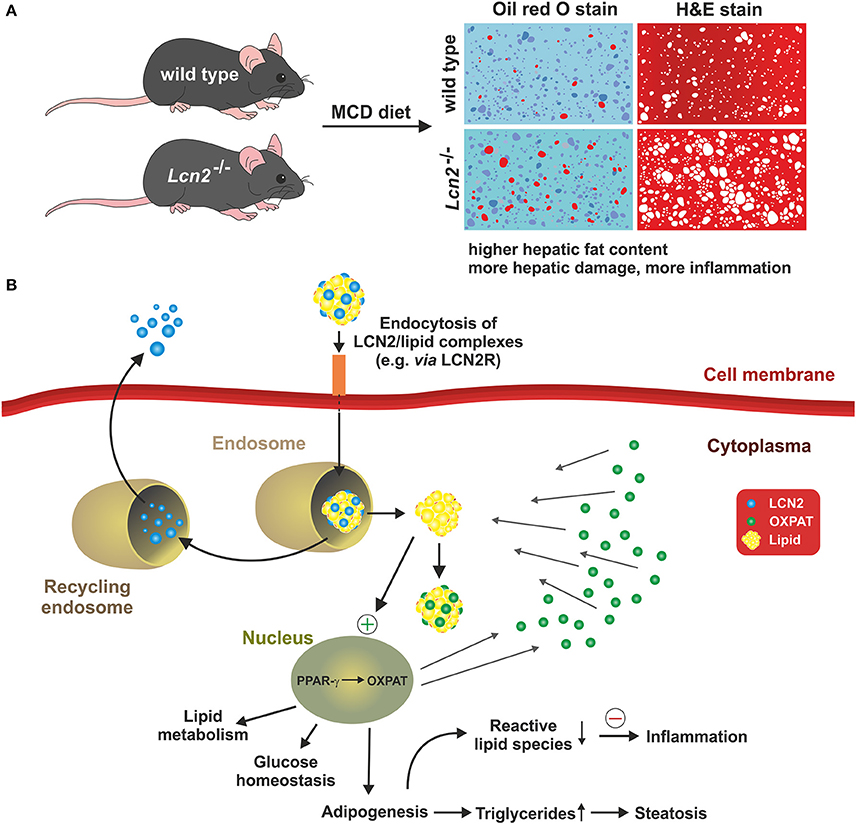
Figure 4. LCN2 and fat metabolism. A recent concept suggests that LCN2 is a key factor in controlling intracellular fat metabolisms in hepatocytes by regulating expression of the lipid droplet protein PLIN5/OXPAT. (A) The concept is majorly based on the finding that mice lacking LCN2 accumulate more lipids in the liver and show more hepatic damage and inflammation when fed a methionine-choline deficient diet (MCD) representing a nutritional model of NASH. (B) In the respective study, it was proposed that LCN2 imports lipids into hepatocytes either via specific receptors (e.g., LCN2R) or unknown endocytosis pathways. Within the cytoplasm, the LCN2/lipid complexes are first packed into endosomes whose slightly acidified microenvironment causes LCN2 to dissociate from the lipids. The lipids then move into the cytoplasm, where they are coated by PLIN5/OXPAT protecting them from intracellular degradation and oxidation. LCN2 may be recycled and secreted. PLIN5/OXPAT itself is up-regulated by PPAR-γ that is stimulated by the higher cytoplasmic fat content that is the consequence of facilitated lipid import by LCN2. Together, the suspected interaction of LCN2, PPAR-γ, and PLIN5/OXPAT presents a complex network that affects lipid metabolism, glucose homeostasis, and adipogenesis. In the presence of LCN2, intracellular concentrations of free reactive lipid species may be reduced and the overall inflammatory responses suppressed. Contrarily, in the absence of LCN2, the concentration of free fatty acids within the cytosol is increased predisposing for inflammation and steatosis.
Moreover, our study has shown that LCN2 is able to directly influence the expression of this lipid-droplet protein that is essential in maintaining the balance between lipogenesis and lipolysis and also of fundamental importance in fatty acid oxidation. Interestingly, Semba and colleagues found in the Fatty liver Shionogi (FLS) mice strain that are genetically programmed to develop NASH that LCN2 is directly linked to the pathogenesis of NASH. In addition, this study showed that LCN2 is primarily expressed by hepatocytes present in inflammatory cell clusters (Semba et al., 2013). Complementary data have suggested that the injury-induced upregulation of LCN2 is a kind of intrinsic “help me” signal (Figure 5) that might be relevant to recruit inflammatory cells into the injured tissue (Asimakopoulou et al., 2016).
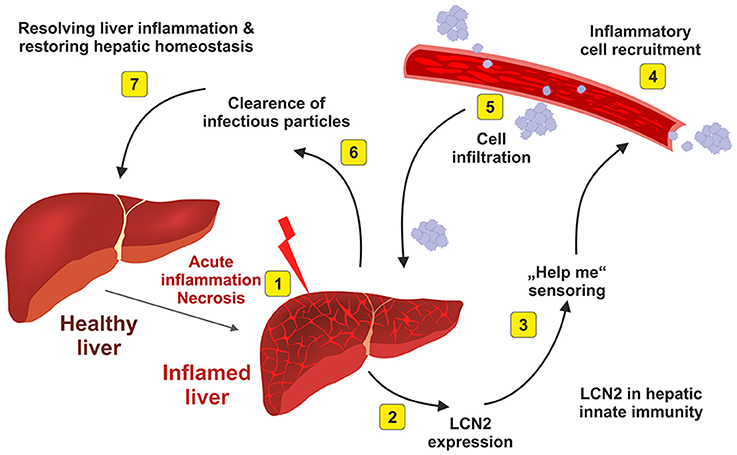
Figure 5. Elevated hepatic LCN2 expression is a physiological indicator of hepatic inflammation. After acute inflammatory stimulus (1), the liver synthesizes and secretes large quantities of LCN2 (2). This physiological response indicates that the liver is inflamed and needs help (3). As a consequence of the emitted “help me” signal, inflammatory blood cells that are necessary to begin the repair are recruited (4) and infiltrate the liver (5). The infiltrating cell populations help to destroy and clear infectious particles (6) and are helpful in restoring tissue homeostasis (7). For more details about this hypothesis are given elsewhere (Asimakopoulou et al., 2016).
After the finding that elevated LCN2 in circulation contributes to the progression of NAFLD (Ye et al., 2014), alcohol-related steatohepatitis was very recently shown also to closely relate to LCN2 levels (Wieser et al., 2016). In the respective study, the authors showed that Lcn2-deficient mice treated with ethanol presented less severe liver injury histologically and biochemically than the wild type mice. Reduced injury, according to ALT levels, was presented as well in wild type mice after LCN2 was neutralized before ethanol treatment with an LCN2 specific antibody. In addition, the control mice fed with ethanol showed increased LCN2 expression that was mainly localized to leukocytes, especially neutrophils and to a lower content in monocytes and Kupffer cells (Wieser et al., 2016). Most interestingly, adoptive transfers revealed that neutrophil-derived LCN2 is critically involved in hepatic neutrophil immigration chronic alcoholic exposure, suggesting that the neutralization of LCN2 in alcoholic liver disease might be a promising therapeutic option to interfere with hepatic inflammation (Wieser et al., 2016). In parallel to this, we showed previously that during hepatic inflammation, the recruitment of leukocytes/neutrophils as indicated by CD45 mRNA content and Myeloperoxidase activity were significantly lower in Lcn2 deficient mice compared to wild type mice (Asimakopoulou et al., 2016). In the same report we demonstrated that wild type mice after LPS treatment for 6 h recruited more Myeloperoxidase positive neutrophils when compared to LCN2 nulls, once again supporting the idea that LCN2 is critical for leukocyte and neutrophil recruitment (Asimakopoulou et al., 2016). The impact on migration and chemotaxis on polymorphonuclear neutrophils was previously demonstrated in mouse models in which the injection of LCN2 stimulated the mobilization of neutrophils (Schroll et al., 2012). All these data indicate that LCN2 acts as a paracrine chemoattractant that modulates neutrophil trafficking to the liver and other organs.
Hepatocellular Carcinoma and Hepatitis C
Hepatitis C virus (HCV) infection is a major cause of chronic liver disease worldwide, leading to end-stage cirrhosis, and development of hepatocellular carcinoma (HCC). In 2010 urinary LCN2 levels were correlated to hepatic fibrosis and cirrhosis in HCV patients (Kim et al., 2010). Shortly after, an additional investigation demonstrated that LCN2 levels was elevated in HCC tissues and that both higher LCN2 and LCN2R expression correlated with shorter overall survival in HCC patients (Figure 6; Zhang et al., 2012).
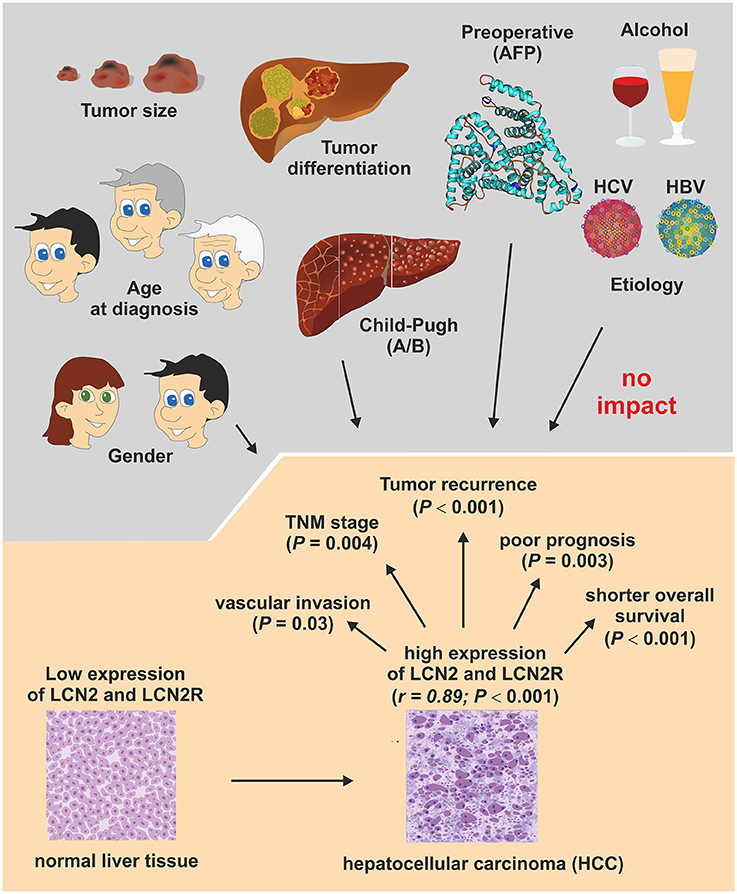
Figure 6. Association of LCN2 and LCN2R expression with conventional clinicopathological HCC parameters. An association study investigated the clinical significance of LCN2 and LCN2R in 138 patients who underwent curative resection of HCC. In conclusion, this study showed that the expression of both genes is correlated (r = 0.89; P < 0.001) and are up-regulated in HCC tissue and associated with vascular invasion status (P = 0.03) and TNM classification stage of malignant tumors (P = 0.004) that consider characteristics of the original primary tumor (T), the involved regional lymph nodes (N), and the occurrence of distant metastasis (M). In addition, the expression of both genes correlated well with tumor recurrence (P < 0.001), poor prognosis (P < 0.003), and overall survival rates (P < 0.001), suggesting that LCN2 and LCN2R expression are suitable prognostic factors and potential therapeutic targets in HCC. Details of this study are given elsewhere (Zhang et al., 2012).
Equally, LCN2 expression in patients with HCV and HCC is significantly increased (El Moety et al., 2013) proposing LCN2 as a reliable diagnostic marker for HCC progression. In this regard it should be mentioned that patients suffering from HCC are nowadays treated with hepatectomy, a surgical procedure of liver resection to remove hepatic tumors. This procedure is only possible because the liver is the only visceral organ that possesses a remarkable capacity to regenerate. In murine models of partial hepatectomy both Xu's and Kienzl-Wagner's teams demonstrated dramatic elevation of hepatic and serum LCN2 (Kienzl-Wagner et al., 2015; Xu et al., 2015). Xu et al. showed that the liver and specifically the hepatocytes are the main source of LCN2 responsible for the highly elevated expression of LCN2 after hepatectomy leading to a significant increase in circulating LCN2 levels for up to 24 h after hepatectomy (Xu et al., 2015). This finding indicates that LCN2 could serve as a biomarker for liver regeneration, however further experimentation is still necessary to elucidate the role of LCN2 in liver hepatectomy and regeneration.
Diagnostic Value of LCN2
In the reported findings described above, LCN2 is shown to be directly linked to several hepatic disorders in both humans and animals. Alterations in LCN2 expression are demonstrated in numerous models of hepatic pathogenesis conditions such as acute and chronic liver injury, inflammation, oxidative stress, mitochondrial dysfunction, bacterial infections, hepatitis C-induced fibrosis, liver failure, obesity, NAFLD, NASH, AFLD, HCC, and rejection after liver transplantarion (cf. Table 3).
Serum LCN2 could serve as a valuable biomarker of early stage hepatic damage (Borkham-Kamphorst et al., 2011, 2013). The measurement of LCN2 in urinary samples could be useful to predict the outcome of cirrhosis, liver transplantation rejection, and hepatic fibrosis (Kim et al., 2010; Wei et al., 2015; Ariza et al., 2016). Clinical data and in vivo models of FLD (NASH, NAFLD, AFLD) showed that elevated LCN2 levels are suitable to mirror hepatic lipid abnormalities, inflammatory response, mitochondrial malfunction, progress of FLD, and HCC (Guo et al., 2010; Zhang et al., 2012; El Moety et al., 2013; Semba et al., 2013; Asimakopoulou et al., 2014; Wieser et al., 2016). Since LCN2 is being proved to mediate hepatic function under multiple conditions, several studies during the last years have been focussed on the development of sensitive detection methods for LCN2. The mainly applied methods used for LCN2 detection include ELISA, Western blots and immunostainings. All these methods need specific LCN2 antibodies which have been available from a variety of pharmaceutical companies. We have previously listed the most common antibodies and systems used in research nowadays for the detection of LCN2 (Asimakopoulou and Weiskirchen, 2015). Most popular for quantification are diverse ELISA tests that have been proved to be the most sensitive and quick method to detect LCN2 elevation in early stages and conditions of experimental and clinical liver disease including FLD, NASH, and HCC.
However, there are actually many established biomarkers available for each of these diseases and it is too early to estimate the diagnostic and prognostic significance of LCN2 for daily clinical routine. It will be exciting to follow up if LCN2 will be added to the list of biomarkers suitable in HCC diagnosis and which superior insights in HCC development or progression will arise from LCN2 measurements that are not already disclosed by other serum markers such as α-fetoprotein (AFP), M39, M65, soluble CD163, or others that are already in use as tissue- or serum-associated HCC biomarkers (Waidmann et al., 2013; Morris et al., 2014; Chauhan and Lahiri, 2016).
LCN2 in Therapy
Many of the clinical conditions mentioned above that lead to hepatic malfunction or damage are associated with a significant upregulation of LCN2 expression in serum, urine, and/or liver tissue. Actually, there are many ongoing studies that analyse the precise molecular activities of LCN2 during disease progression. Recent studies performed in human kidney cells (i.e., HK-2) have shown that the application of recombinant LCN2 proteins induces excessive autophagy suggesting that LCN2 may have tremendous effects in AKI (Zhang W. et al., 2016). In contrast, protective effects of LCN2 were demonstrated in a mouse model of nephrotoxic serum nephritis (Eller et al., 2013). Likewise, a study that was conducted in myoblastic rat cardiomyocytes (i.e., H9c2) showed that the treatment with recombinant LCN2 inhibited autophagy (Chan et al., 2016). These contradictory findings indicate that LCN2 might have both protective as well as pathogenic activities. These double-edged biological properties may render therapeutic approaches complicated.
In regard to the liver, the chronic infusion of LCN2 exacerbated liver injury in two different models (i.e., MCD and high fat high cholesterol diet), while at the same time the observed hepatic injury in mice that were deficient for LCN2 was markedly reduced after feeding respective diets (Ye et al., 2016). Further analysis in this study showed that the inflammatory activities of LCN2 are mediated via induction of the chemokine receptor CXCR2 (formerly known as Interleukin 8 receptor, beta) that transduces its signals through a G-protein-activated second messenger system. Similarly, a pivotal and causal role of LCN2 was shown in cell models and animal models of AFLD (Cai et al., 2016). However, there are also other disease conditions in which this lipocalin protects the liver from acute liver injury (Borkham-Kamphorst et al., 2013), again demonstrating the versatile activities of LCN2.
Although it is now well-established that LCN2 has fundamental modulator activities during hepatic insult, there is still some work that remains to be done prior announcing LCN2 as a therapeutic target. Currently, initial studies that use anti-LCN2 antibodies (Tarín et al., 2016), siRNAs or shRNAs targeting LCN2 (Volpe et al., 2013; Miyamoto et al., 2016), specialized nanoparticle-based theranostic agents (Guo et al., 2016), or modulators that impacts LCN2 receptor expression (Cui et al., 2008) in different settings are initiated. In addition, the design of therapeutic small-molecule inhibitors for LCN2 was recently proposed (Suk, 2016). These molecules might be beneficial to target de novo LCN2 expression or its interaction with its cognitive receptors. Therapeutic strategies are nowadays tested in cell culture and preclinical models, but none of these has been tested in clinical studies so far.
Concluding Remarks
The lipocalin 2 protein was initially proposed to represent a secretory protein that is involved in the transportation of hydrophobic molecules, including steroids, retinoids, hormones, and lipids. Several years later, it has been promoted as a multifunctional siderophore that is involved in innate immunity by sequestering iron that in turn limits bacterial growth. A wide variety of studies have shown that the expression of this lipocalin is significantly altered in the pathogenesis of organ damage affecting kidney, heart, brain, pancreas, and lungs. In this review, we have summarized experimental and clinical findings linking LCN2 to liver disorders. It is obvious that LCN2 is significantly upregulated during phases of hepatic injury. In line with this assumption, numerous studies correlated LCN2 with general hepatic injury, inflammation, infection, cirrhosis, and fatty liver disorders. In all these pathological states LCN2 expression seems to be highly elevated, underpinning the notion that this lipocalin is a good biomarker candidate for a large variety of hepatic insults. Experimental findings have shown that the loss of LCN2 is correlated with a higher susceptibility to bacterial infections and more severe hepatic damage after challenge with appropriate hepatotoxins. Nowadays, several diagnostic test systems for detecting and quantification of LCN2 in urine, serum, plasma and tissue extracts are on the way to be incorporated into the routine diagnostics. Under some conditions, the measurement of urine LCN2 could more accurately reflect systemic inflammation or disease progression than serum LCN2. However, although the knowledge in LCN2 in liver biology has increased dramatically during the last years, there is still a mandatory need for studies that further characterized LCN2 and its pathways in the initiation, progression and potentially regression of hepatic lesions. These studies will potentially lead to novel therapeutic avenues or drugs that might be beneficial to interfere with progression or foster regression of inflammatory hepatic disease.
Author Contributions
RW and AA have written this review. SW prepared the figures. All authors agree to be accountable for the content of this work.
Funding
RW is supported by grants from the German Research Foundation (SFB/TRR 57, P13/Q3) and a grant from the Interdisciplinary Centre for Clinical Research within the Faculty of Medicine at the RWTH Aachen University (IZKF Aachen, Project E7-6). None of the funding sources exerted influence on content or decision to submit the article for publication.
Conflict of Interest Statement
The authors declare that the research was conducted in the absence of any commercial or financial relationships that could be construed as a potential conflict of interest.
Abbreviations
AFLD, alcoholic fatty liver disease; AKI, acute kidney injury; ER; endoplasmic reticulum; FLD, fatty liver disease; HCC, hepatocellular carcinoma; HCV, Hepatitis C virus; LCN2, lipocalin 2; LPS, lipopolysaccharide(s); MCI, mild cognitive impairment; MMP, matrix metalloproteinase; MS, multiple sclerosis; NAFLD, non-alcoholic fatty liver disease; NASH, non-alcoholic steatohepatitis; NF-κB, nuclear factor-κB; NGAL, neutrophil gelatinase-associated lipocalin.
References
Aigner, F., Maier, H. T., Schwelberger, H. G., Wallnöfer, E. A., Amberger, A., Obrist, P., et al. (2007). Lipocalin-2 regulates the inflammatory response during ischemia and reperfusion of the transplanted heart. Am. J. Transplant. 7, 779–788. doi: 10.1111/j.1600-6143.2006.01723.x
Alwahsh, S. M., Xu, M., Seyhan, H. A., Ahmad, S., Mihm, S., Ramadori, G., et al. (2014). Diet high in fructose leads to an overexpression of lipocalin-2 in rat fatty liver. World J. Gastroenterol. 20, 1807–1821. doi: 10.3748/wjg.v20.i7.1807
Ariza, X., Graupera, I., Coll, M., Solà, E., Barreto, R., García, E., et al. (2016). Neutrophil gelatinase-associated lipocalin is a biomarker of acute-on-chronic liver failure and prognosis in cirrhosis. J. Hepatol. 65, 57–65. doi: 10.1016/j.jhep.2016.03.002
Ashraf, M. I., Schwelberger, H. G., Brendel, K. A., Feurle, J., Andrassy, J., Kotsch, K., et al. (2016). Exogenous Lipocalin 2 ameliorates acute rejection in a mouse model of renal transplantation. Am. J. Transplant. 16, 808–820. doi: 10.1111/ajt.13521
Asimakopoulou, A., Borkham-Kamphorst, E., Henning, M., Yagmur, E., Gassler, N., Liedtke, C., et al. (2014). Lipocalin-2 (LCN2) regulates PLIN5 expression and intracellular lipid droplet formation in the liver. Biochim. Biophys. Acta 1842, 1513–1524. doi: 10.1016/j.bbalip.2014.07.017
Asimakopoulou, A., Borkham-Kamphorst, E., Tacke, F., and Weiskirchen, R. (2016). Lipocalin-2 (NGAL/LCN2), a “help-me” signal in organ inflammation. Hepatology 63, 669–671. doi: 10.1002/hep.27930
Asimakopoulou, A., and Weiskirchen, R. (2015). Lipocalin 2 in the pathogenesis of fatty liver disease and nonalcoholic steatohepatitis. Clin. Lipidol. 10, 47–67. doi: 10.2217/clp.14.65
Auguet, T., Terra, X., Quintero, Y., Martínez, S., Manresa, N., Porras, J. A., et al. (2013). Liver lipocalin 2 expression in severely obese women with non alcoholic fatty liver disease. Exp. Clin. Endocrinol. Diabetes 121, 119–124. doi: 10.1055/s-0032-1331696
Axelsson, L., Bergenfeldt, M., and Ohlsson, K. (1995). Studies of the release and turnover of a human neutrophil lipocalin. Scand. J. Clin. Lab. Invest. 55, 577–588. doi: 10.3109/00365519509110257
Barr, J., Vázquez-Chantada, M., Alonso, C., Pérez-Cormenzana, M., Mayo, R., Galán, A., et al. (2010). Liquid chromatography-mass spectrometry-based parallel metabolic profiling of human and mouse model serum reveals putative biomarkers associated with the progression of nonalcoholic fatty liver disease. J. Proteome Res. 9, 4501–4512. doi: 10.1021/pr1002593
Barresi, V., Ieni, A., Bolignano, D., Magno, C., Buemi, M., and Barresi, G. (2010). Neutrophil gelatinase-associated lipocalin immunoexpression in renal tumors: correlation with histotype and histological grade. Oncol. Rep. 24, 305–310. doi: 10.3892/or_00000860
Barresi, V., Leni, A., Tuccari, G., and Barresi, G. (2012b). Neutrophil gelatinase-associated lipocalin (NGAL) immunohistochemical expression in follicular cell-derived thyroid tumors: a novel diagnostic tool? Histol. Histopathol. 27, 329–336. doi: 10.3892/or_00000860
Barresi, V., Vitarelli, E., Reggiani Bonetti, L., Tuccari, G., and Barresi, G. (2012a). Diagnostic value of neutrophil gelatinase-associated lipocalin (NGAL) immunoexpression in follicular-patterned lesions of the thyroid gland. Virchows Arch. 460, 319–325. doi: 10.1007/s00428-012-1208-0
Barreto, R., Elia, C., Solà, E., Moreira, R., Ariza, X., Rodríguez, E., et al. (2014). Urinary neutrophil gelatinase-associated lipocalin predicts kidney outcome and death in patients with cirrhosis and bacterial infections. J. Hepatol. 61, 35–42. doi: 10.1016/j.jhep.2014.02.023
Baumeister, S. E., Völzke, H., Marschall, P., John, U., Schmidt, C. O., Flessa, S., et al. (2008). Impact of fatty liver disease on health care utilization and costs in a general population: a 5-year observation. Gastroenterology 134, 85–94. doi: 10.1053/j.gastro.2007.10.024
Belcher, J. M., Garcia-Tsao, G., Sanyal, A. J., Thiessen-Philbrook, H., Peixoto, A. J., Perazella, M. A., et al. (2014). Urinary biomarkers and progression of AKI in patients with cirrhosis. Clin. J. Am. Soc. Nephrol. 9, 1857–1867. doi: 10.2215/CJN.09430913
Bellentani, S., Scaglioni, F., Marino, M., and Bedogni, G. (2010). Epidemiology of non-alcoholic fatty liver disease. Dig. Dis. 28, 155–161. doi: 10.1159/000282080
Berger, T., Togawa, A., Duncan, G. S., Elia, A. J., You-Ten, A., Wakeham, A., et al. (2006). Lipocalin 2-deficient mice exhibit increased sensitivity to Escherichia coli infection but not to ischemia-reperfusion injury. Proc. Natl. Acad. Sci. U.S.A. 103, 1834–1839. doi: 10.1073/pnas.0510847103
Bläser, J., Triebel, S., and Tschesche, H. (1995). A sandwich enzyme immunoassay for the determination of neutrophil lipocalin in body fluids. Clin. Chim. Acta 235, 137–145. doi: 10.1016/0009-8981(95)06020-7
Bolignano, D., Coppolino, G., Romeo, A., De Paola, L., Buemi, A., Lacquaniti, A., et al. (2009). Neutrophil gelatinase-associated lipocalin (NGAL) reflects iron status in haemodialysis patients. Nephrol. Dial. Transplant. 24, 3398–3403. doi: 10.1093/ndt/gfp310
Borkham-Kamphorst, E., Drews, F., and Weiskirchen, R. (2011). Induction of lipocalin-2 expression in acute and chronic experimental liver injury moderated by pro-inflammatory cytokines interleukin-1β through nuclear factor-κB activation. Liver Int. 31, 656–665. doi: 10.1111/j.1478-3231.2011.02495.x
Borkham-Kamphorst, E., van de Leur, E., Zimmermann, H. W., Karlmark, K. R., Tihaa, L., Haas, U., et al. (2013). Protective effects of lipocalin-2 (LCN2) in acute liver injury suggest a novel function in liver homeostasis. Biochim. Biophys. Acta 1832, 660–673. doi: 10.1016/j.bbadis.2013.01.014
Bu, D. X., Hemdahl, A. L., Gabrielsen, A., Fuxe, J., Zhu, C., Eriksson, P., et al. (2006). Induction of neutrophil gelatinase-associated lipocalin in vascular injury via activation of nuclear factor-kappaB. Am. J. Pathol. 169, 2245–2253. doi: 10.2353/ajpath.2006.050706
Bundgaard, J. R., Sengeløv, H., Borregaard, N., and Kjeldsen, L. (1994). Molecular cloning and expression of a cDNA encoding NGAL: a lipocalin expressed in human neutrophils. Biochem. Biophys. Res. Commun. 202, 1468–1475. doi: 10.1006/bbrc.1994.2096
Cabedo Martinez, A. I., Weinhäupl, K., Lee, W. K., Wolff, N. A., Storch, B., Żerko, S., et al. (2016). Biochemical and structural characterization of the interaction between the siderocalin NGAL/LCN2 (Neutrophil Gelatinase-associated Lipocalin/Lipocalin 2) and the N-terminal domain of its endocytic receptor SLC22A17. J. Biol. Chem. 291, 2917–2930. doi: 10.1074/jbc.M115.685644
Cai, L., Rubin, J., Han, W., Venge, P., and Xu, S. (2010). The origin of multiple molecular forms in urine of HNL/NGAL. Clin. J. Am. Soc. Nephrol. 5, 2229–2235. doi: 10.2215/CJN.00980110
Cai, Y., Jogasuria, A., Yin, H., Xu, M.-J., Hu, X., Wang, J., et al. (2016). The detrimental role played by Lipocalin-2 in alcoholic fatty liver in mice. Am. J. Pathol. 186, 2417–2428. doi: 10.1016/j.ajpath.2016.05.006
Chan, P., Simon-Chazottes, D., Mattei, M. G., Guenet, J. L., and Salier, J. P. (1994). Comparative mapping of lipocalin genes in human and mouse: the four genes for complement C8 γ chain, prostaglandin-D-synthase, oncogene-24p3, and progestagen-associated endometrial protein map to HSA9 and MMU2. Genomics 23, 145–150.
Chan, Y. K., Sung, H. K., Jahng, J. W., Kim, G. H., Han, M., and Sweeney, G. (2016). Lipocalin-2 inhibits autophagy and induces insulin resistance in H9c2 cells. Mol. Cell Endocrinol. 430, 68–76. doi: 10.1016/j.mce.2016.04.006
Chan, Y. K., Sung, H. K., and Sweeney, G. (2015). Iron metabolism and regulation by neutrophil gelatinase - associated lipocalin in cardiomyopathy. Clin. Sci. (Lond) 129, 851–862. doi: 10.1042/CS20150075
Chan, Y. R., Liu, J. S., Pociask, D. A., Zheng, M., Mietzner, T. A., Berger, T., et al. (2009). Lipocalin 2 is required for pulmonary host defense against Klebsiella infection. J. Immunol. 182, 4947–4956. doi: 10.4049/jimmunol.0803282
Chauhan, R., and Lahiri, N. (2016). Tissue- and serum-associated biomarkers of hepatocellular carcinoma. Biomark Cancer 8(Suppl. 1), 37–55. doi: 10.4137/BIC.S34413
Choi, J., Lee, H. W., and Suk, K. (2011). Increased plasma levels of lipocalin 2 in mild cognitive impairment. J. Neurol. Sci. 305, 28–33. doi: 10.1016/j.jns.2011.03.023
Coca, S. G., Yalavarthy, R., Concato, J., and Parikh, C. R. (2008). Biomarkers for the diagnosis and risk stratification of acute kidney injury: a systematic review. Kidney Int. 73, 1008–1016. doi: 10.1038/sj.ki.5002729
Correnti, C., Richardson, V., Sia, A. K., Bandaranayake, A. D., Ruiz, M., Suryo Rahmanto, Y., et al. (2012). Siderocalin/Lcn2/NGAL/24p3 does not drive apoptosis through gentisic acid mediated iron withdrawal in hematopoietic cell lines. PLoS ONE 7:e43696. doi: 10.1371/journal.pone.0043696
Costa, D., Lazzarini, E., Canciani, B., Giuliani, A., Spanò, R., Marozzi, K., et al. (2013). Altered bone development and turnover in transgenic mice over-expressing lipocalin-2 in bone. J. Cell Physiol. 228, 2210–2221. doi: 10.1002/jcp.24391
Cowland, J. B., and Borregaard, N. (1997). Molecular characterization and pattern of tissue expression of the gene for neutrophil gelatinase-associated lipocalin from humans. Genomics 45, 17–23.
Cui, L., Xu, L. Y., Shen, Z. Y., Tao, Q., Gao, S. Y., Lv, Z., et al. (2008). NGALR is overexpressed and regulated by hypomethylation in esophageal squamous cell carcinoma. Clin. Cancer Res. 14, 7674–7681. doi: 10.1158/1078-0432.CCR-08-0420
Devireddy, L. R., Gazin, C., Zhu, X., and Green, M. R. (2005). A cell-surface receptor for lipocalin 24p3 selectively mediates apoptosis and iron uptake. Cell 123, 1293–1305. doi: 10.1016/j.cell.2005.10.027
Du, Z. P., Lv, Z., Wu, B. L., Wu, Z. Y., Shen, J. H., Wu, J. Y., et al. (2011). Neutrophil gelatinase-associated lipocalin and its receptor: independent prognostic factors of oesophageal squamous cell carcinoma. J. Clin. Pathol. 64, 69–74. doi: 10.1136/jcp.2010.083907
El Karoui, K., Viau, A., Dellis, O., Bagattin, A., Nguyen, C., Baron, W., et al. (2016). Endoplasmic reticulum stress drives proteinuria-induced kidney lesions via Lipocalin 2. Nat. Commun. 7:10330. doi: 10.1038/ncomms10330
Eller, K., Schroll, A., Banas, M., Kirsch, A. H., Huber, J. M., Nairz, M., et al. (2013). Lipocalin-2 expressed in innate immune cells is an endogenous inhibitor of inflammation in murine nephrotoxic serum nephritis. PLoS ONE 8:e67693. doi: 10.1371/journal.pone.0067693
El Moety, H. A., El Sharkawy, R. M., and El Menem Hussein, N. A. (2013). Lipocalin: a novel diagnostic marker for hepatocellular carcinoma in chronic liver disease patients in Egypt. Int. J. Clin. Med. 4, 440–450. doi: 10.4236/ijcm.2013.410079
Elsharawy, S., Raslan, L., Morsy, S., Hassan, B., and Khalifa, N. (2016). Plasma neutrophil gelatinase-associated lipocalin as a marker for the prediction of worsening renal function in children hospitalized for acute heart failure. Saudi J. Kidney Dis. Transpl. 27, 49–54. doi: 10.4103/1319-2442.174071
Fagundes, C., Pépin, M. N., Guevara, M., Barreto, R., Casals, G., Solà, E., et al. (2012). Urinary neutrophil gelatinase-associated lipocalin as biomarker in the differential diagnosis of impairment of kidney function in cirrhosis. J. Hepatol. 57, 267–273. doi: 10.1016/j.jhep.2012.03.015
Fierbinteanu-Braticevici, C., Dina, I., Petrisor, A., Tribus, L., Negreanu, L., and Carstoiu, C. (2010). Noninvasive investigations for non alcoholic fatty liver disease and liver fibrosis. World J. Gastroenterol. 16, 4784–4791. doi: 10.3748/wjg.v16.i38.4784
Flo, T. H., Smith, K. D., Sato, S., Rodriguez, D. J., Holmes, M. A., Strong, R. K., et al. (2004). Lipocalin 2 mediates an innate immune response to bacterial infection by sequestrating iron. Nature 432, 917–921. doi: 10.1038/nature03104
Fotbolcu, H., and Zorlu, E. (2016). Nonalcoholic fatty liver disease as a multi-systemic disease. World J. Gastroenterol. 22, 4079–4090. doi: 10.3748/wjg.v22.i16.4079
Friedl, A., Stoesz, S. P., Buckley, P., and Gould, M. N. (1999). Neutrophil gelatinase-associ-ated lipocalin in normal and neoplastic human tissues. Cell type-specific pattern of expression. Histochem. J. 31, 433–441. doi: 10.1023/A:1003708808934
Garay-Rojas, E., Harper, M., Hraba-Renevey, S., and Kress, M. (1996). An apparent autocrine mechanism amplifies the dexamethasone- and retinoic acid-induced expression of mouse lipocalin-encoding gene 24p3. Gene 170, 173–180.
Gerbes, A. L., Benesic, A., Vogeser, M., Krag, A., Bendtsen, F., and Mïller, S. (2011). Serum neutrophil gelatinase-associated lipocalin—a sensitive novel marker of renal impairment in liver cirrhosis? Digestion 84, 82–83. doi: 10.1159/000324881
Goetz, D. H., Holmes, M. A., Borregaard, N., Bluhm, M. E., Raymond, K. N., and Strong, R. K. (2002). The neutrophil lipocalin NGAL is a bacteriostatic agent that interferes with siderophore-mediated iron acquisition. Mol. Cell 10, 1033–1043. doi: 10.1016/S1097-2765(02)00708-6
Goetz, D. H., Willie, S. T., Armen, R. S., Bratt, T., Borregaard, N., and Strong, R. K. (2000). Ligand preference inferred from the structure of neutrophil gelatinase associated lipocalin. Biochemistry 39, 1935–1941. doi: 10.1021/bi992215v
Guo, H., Jin, D., Zhang, Y., Wright, W., Bazuine, M., Brockman, D. A., et al. (2010). Lipocalin-2 deficiency impairs thermogenesis and potentiates diet-induced insulin resistance in mice. Diabetes 59, 1376–1385. doi: 10.2337/db09-1735
Guo, P., Yang, J., Jia, D., Moses, M. A., and Auguste, D. T. (2016). ICAM-1-targeted, Lcn2 siRNA-encapsulating liposomes are potent anti-angiogenic agents for triple negative breast cancer. Theranostics 6, 1–13. doi: 10.7150/thno.12167
Hamzic, N., Blomqvist, A., and Nilsberth, C. (2013). Immune-induced expression of lipocalin-2 in brain endothelial cells: relationship with interleukin-6, cycloox-ygenase-2 and the febrile response. J. Neuroendocrinol. 25, 271–280. doi: 10.1111/jne.12000
Hau, C. S., Kanda, N., Tada, Y., Shibata, S., Uozaki, H., Fukusato, T., et al. (2016). Lipocalin-2 exacerbates psoriasiform skin inflammation by augmenting T-helper 17 response. J. Dermatol. 43, 785–794. doi: 10.1111/1346-8138.13227
Heyne, N., Kemmner, S., Schneider, C., Nadalin, S., Konigsrainer, A., and Häring, H. U. (2012). Urinary neutrophil gelatinase-associated lipocalin accurately detects acute allograft rejection among other causes of acute kidney injury in renal allograft recipients. Transplantation 93, 1252–1257. doi: 10.1097/TP.0b013e31824fd892
Holmes, M. A., Paulsene, W., Jide, X., Ratledge, C., and Strong, R. K. (2005). Siderocalin [Lcn 2] also binds carboxymycobactins, potentially defending against mycobacterial infections through iron sequestration. Structure 13, 29–41. doi: 10.1016/j.str.2004.10.009
Hvidberg, V., Jacobsen, C., Strong, R. K., Cowland, J. B., Moestrup, S. K., and Borregaard, N. (2005). The endocytic receptor megalin binds the iron transporting neutrophil-gelatinase-associated lipocalin with high affinity and mediates its cellular uptake. FEBS Lett. 579, 773–777. doi: 10.1016/j.febslet.2004.12.031
Iannetti, A., Pacifico, F., Acquaviva, R., Lavorgna, A., Crescenzi, E., Vascotto, C., et al. (2008). The neutrophil gelatinase-associated lipocalin (NGAL), a NF-κB-regulated gene, is a survival factor for thyroid neoplastic cells. Proc. Natl. Acad. Sci. U.S.A. 105, 14058–14063. doi: 10.1073/pnas.0710846105
Jiang, L., and Cui, H. (2015). Could blood Neutrophil Gelatinase-Associated Lipocalin (NGAL) be a diagnostic marker for acute kidney injury in neonates? A systemic review and meta-analysis. Clin. Lab. 61, 1815–1820. doi: 10.7754/Clin.Lab.2015.150532
Jin, D., Guo, H., Bu, S. Y., Zhang, Y., Hannaford, J., Mashek, D. G., et al. (2011). Lipocalin 2 is a selective modulator of peroxisome proliferator-activated receptor-γ activation and function in lipid homeostasis and energy expenditure. FASEB J. 25, 754–764. doi: 10.1096/fj.10-165175
Kangelaris, K. N., Prakash, A., Liu, K. D., Aouizerat, B., Woodruff, P. G., Erle, D. J., et al. (2015). Increased expression of neutrophil-related genes in patients with early sepsis-induced ARDS. Am. J. Physiol. Lung Cell Mol. Physiol. 308, L1102–L1113. doi: 10.1152/ajplung.00380.2014
Kaur, S., Baine, M. J., Guha, S., Ochi, N., Chakraborty, S., Mallya, K., et al. (2013). Neutrophil gelatinase-associated lipocalin, macrophage inhibitory cytokine 1, and carbohydrate antigen 19-9 in pancreatic juice: pathobiologic implications in diagnosing benign and malignant disease of the pancreas. Pancreas 42, 494–501. doi: 10.1097/MPA.0b013e31826a8597
Kienzl-Wagner, K., Moschen, A. R., Geiger, S., Bichler, A., Aigner, F., Brandacher, G., et al. (2015). The role of lipocalin-2 in liver regeneration. Liver Int. 35, 1195–1202. doi: 10.1111/liv.12634
Kim, J. W., Lee, S. H., Jeong, S. H., Kim, H., Ahn, K. S., Cho, J. Y., et al. (2010). Increased urinary lipocalin-2 reflects matrix metalloproteinase-9 activity in chronic hepatitis C with hepatic fibrosis. Tohoku J. Exp. Med. 222, 319–327. doi: 10.1620/tjem.222.319
Kjeldsen, L., Cowland, J. B., and Borregaard, N. (2000). Human neutrophil gelatinase-associated lipocalin and homologous proteins in rat and mouse. Biochim. Biophys. Acta 1482, 272–283. doi: 10.1016/S0167-4838(00)00152-7
Kjeldsen, L., Johnsen, A. H., Sengeløv, H., and Borregaard, N. (1993). Isolation and primary structure of NGAL, a novel protein associated with human neutrophil gelatinase. J. Biol. Chem. 268, 10425–10432.
Kotronen, A., Yki-Järvinen, H., Männistö, S., Saarikoski, L., Korpi-Hyövälti, E., Oksa, H., et al. (2010). Non-alcoholic and alcoholic fatty liver disease - two diseases of affluence associated with the metabolic syndrome and type 2 diabetes: the FIN-D2D survey. BMC Public Health 10:237. doi: 10.1186/1471-2458-10-237
Kubben, F. J., Sier, C. F., Hawinkels, L. J., Tschesche, H., van Duijn, W., Zuidwijk, K., et al. (2007). Clinical evidence for a protective role of lipocalin-2 against MMP-9 autodegradation and the impact for gastric cancer. Eur. J. Cancer 43, 1869–1876. doi: 10.1016/j.ejca.2007.05.013
Labbus, K., Henning, M., Borkham-Kamphorst, E., Geisler, C., Berger, T., Mak, T. W., et al. (2013). Proteomic profiling in Lipocalin 2 deficient mice under normal and inflammatory conditions. J. Proteomics 78, 188–196. doi: 10.1016/j.jprot.2012.11.021
Law, I. K., Xu, A., Lam, K. S., Berger, T., Mak, T. W., Vanhoutte, P. M., et al. (2010). Lipocalin-2 deficiency attenuates insulin resistance associated with aging and obesity. Diabetes 59, 872–882. doi: 10.2337/db09-1541
Lipinski, M., Rydzewska-Rosolowska, A., Rydzewski, A., and Rydzewska, G. (2015). Urinary neutrophil gelatinase-associated lipocalin as an early predictor of disease severity and mortality in acute pancreatitis. Pancreas 44, 448–452. doi: 10.1097/MPA.0000000000000282
Lívero, F. A., and Acco, A. (2016). Molecular basis of alcoholic fatty liver disease: from incidence to treatment. Hepatol. Res. 46, 111–123. doi: 10.1111/hepr.12594
Mallbris, L., O'Brien, K. P., Hulthén, A., Sandstedt, B., Cowland, J. B., Borregaard, N., et al. (2002). Neutrophil gelatinase-associated lipocalin is a marker for dysregulated keratinocyte differentiation in human skin. Exp. Dermatol. 11, 584–591. doi: 10.1034/j.1600-0625.2002.110611.x
Mannelqvist, M., Stefansson, I. M., Wik, E., Kusonmano, K., Raeder, M. B., Øyan, A. M., et al. (2012). Lipocalin 2 expression is associated with aggressive features of endometrial cancer. BMC Cancer 12:169. doi: 10.1186/1471-2407-12-169
Marques, F., Mesquita, S. D., Sousa, J. C., Coppola, G., Gao, F., Geschwind, D. H., et al. (2012). Lipocalin 2 is present in the EAE brain and is modulated by natalizumab. Front. Cell Neurosci. 6:33. doi: 10.3389/fncel.2012.00033
Matsa, R., Ashley, E., Sharma, V., Walden, A. P., and Keating, L. (2014). Plasma and urine neutrophil gelatinase-associated lipocalin in the diagnosis of new onset acute kidney injury in critically ill patients. Crit. Care 18, R137. doi: 10.1186/cc13958
Meheus, L. A., Fransen, L. M., Raymackers, J. G., Blockx, H. A., Van Beeumen, J. J., Van Bun, S. M., et al. (1993). Identification by microsequencing of lipopolysaccharide-induced proteins secreted by mouse macrophages. J. Immunol. 151, 1535–1547.
Miyamoto, T., Kashima, H., Yamada, Y., Kobara, H., Asaka, R., Ando, H., et al. (2016). Lipocalin 2 enhances migration and resistance against cisplatin in endometrial carcinoma cells. PLoS ONE 11:e0155220. doi: 10.1371/journal.pone.0155220
Moniaux, N., Chakraborty, S., Yalniz, M., Gonzalez, J., Shostrom, V. K., Standop, J., et al. (2008). Early diagnosis of pancreatic cancer: neutrophil gelatinase-associated lipocalin as a marker of pancreatic intraepithelial neoplasia. Br. J. Cancer 98, 1540–1547. doi: 10.1038/sj.bjc.6604329
Monier, F., Mollier, S., Guillot, M., Rambeaud, J. J., Morel, F., and Zaoui, P. (2002). Urinary release of 72 and 92 kDa gelatinases, TIMPs, N-GAL and conventional prognostic factors in urothelial carcinomas. Eur. Urol. 42, 356–363. doi: 10.1016/S0302-2838(02)00350-0
Monier, F., Surla, A., Guillot, M., and Morel, F. (2000). Gelatinase isoforms in urine from bladder cancer patients. Clin. Chim. Acta 299, 11–23. doi: 10.1016/S0009-8981(00)00271-0
Morris, K. L., Tugwood, J. D., Khoja, L., Lancashire, M., Sloane, R., Burt, D., et al. (2014). Circulating biomarkers in hepatocellular carcinoma. Cancer Chemother. Pharmacol. 4, 323–332. doi: 10.1007/s00280-014-2508-7
Musana, K. A., Yale, S. H., and Abdulkarim, A. S. (2004). Tests of liver injury. Clin. Med. Res. 2, 129–131. doi: 10.3121/cmr.2.2.129
Myers, R. P. (2009). Noninvasive diagnosis of nonalcoholic fatty liver disease. Ann. Hepatol. 1, S25–S33.
Naudé, P. J., Eisel, U. L., Comijs, H. C., Groenewold, N. A., De Deyn, P. P., Bosker, F. J., et al. (2013). Neutrophil gelatinase-associated lipocalin: a novel inflammatory marker associated with late-life depression. J. Psychosom. Res. 75, 444–450. doi: 10.1016/j.jpsychores.2013.08.023
Neuman, M. G., Voiculescu, M., Nanau, R. M., Maor, Y., Melzer, E., Cohen, L. B., et al. (2016). Non-Alcoholic steatohepatitis: clinical and translational research. J. Pharm. Pharm. Sci. 19, 8–24. doi: 10.18433/J3F61F
Ören, B., Urosevic, J., Mertens, C., Mora, J., Guiu, M., Gomis, R. R., et al. (2016). Tumour stroma-derived lipocalin-2 promotes breast cancer metastasis. J. Pathol. 239, 274–285. doi: 10.1002/path.4724
Paragas, N., Qiu, A., Hollmen, M., Nickolas, T. L., Devarajan, P., and Barasch, J. (2012). NGAL-siderocalin in kidney disease. Biochim. Biophys. Acta 1823, 1451–1458. doi: 10.1016/j.bbamcr.2012.06.014
Pawar, R. D., Pitashny, M., Gindea, S., Tieng, A. T., Levine, B., Goilav, B., et al. (2012). Neutrophil gelatinase-associated lipocalin is instrumental in the pathogenesis of antibody-mediated nephritis in mice. Arthritis Rheum. 64, 1620–1631. doi: 10.1002/art.33485
Reddy, J. K., and Rao, M. S. (2006). Lipid metabolism and liver inflammation. II. Fatty liver disease and fatty acid oxidation. Am. J. Physiol. Gastrointest. Liver Physiol. 290, 852–858. doi: 10.1152/ajpgi.00521.2005
Roth, G. A., Nickl, S., Lebherz-Eichinger, D., Schmidt, E. M., Ankersmit, H. J., Faybik, P., et al. (2013). Lipocalin-2 serum levels are increased in acute hepatic failure. Transplant. Proc. 45, 241–244. doi: 10.1016/j.transproceed.2012.02.047
Roy, R., Louis, G., Loughlin, K. R., Wiederschain, D., Kilroy, S. M., Lamb, C. C., et al. (2008). Tumor-specific urinary matrix metalloproteinase fingerprinting: identification of high molecular weight urinary matrix metalloproteinase species. Clin. Cancer Res. 14, 6610–6617. doi: 10.1158/1078-0432.CCR-08-1136
Schroll, A., Eller, K., Feistritzer, C., Nairz, M., Sonnweber, T., Moser, P. A., et al. (2012). Lipocalin-2 ameliorates granulocyte functionality. Eur. J. Immunol. 42, 3346–3357. doi: 10.1002/eji.201142351
Semba, T., Nishimura, M., Nishimura, S., Ohara, O., Ishige, T., Ohno, S., et al. (2013). The FLS (fatty liver Shionogi) mouse reveals local expressions of lipocalin-2, CXCL1 and CXCL9 in the liver with non-alcoholic steatohepatitis. BMC Gastroenterol. 13:120. doi: 10.1186/1471-230X-13-120
Seth, P., Porter, D., Lahti-Domenici, J., Geng, Y., Richardson, A., and Polyak, K. (2002). Cellular and molecular targets of estrogen in normal human breast tissue. Cancer Res. 62, 4540–4544.
Shah, B. N., and Greaves, K. (2010). The cardiorenal syndrome: a review. Int. J. Nephrol. 2011:920195. doi: 10.4061/2011/920195
Smith, E. R., Zurakowski, D., Saad, A., Scott, R. M., and Moses, M. A. (2008). Urinary biomarkers predict brain tumor presence and response to therapy. Clin. Cancer Res. 4, 2378–2386. doi: 10.1158/1078-0432.CCR-07-1253
Sørensen, O. E., Cowland, J. B., Theilgaard-Monch, K., Liu, L., Ganz, T., and Borregaard, N. (2003). Wound healing and expression of antimicrobial peptides/polypeptides in human keratinocytes, a consequence of common growth factors. J. Immunol. 170, 5583–5589. doi: 10.4049/jimmunol.170.11.5583
Suk, K. (2016). Lipocalin-2 as a therapeutic target for brain injury: an astrocentric perspective. Prog. Neurobiol. 144, 158–172. doi: 10.1016/j.pneurobio.2016.08.001
Sunil, V. R., Patel, K. J., Nilsen-Hamilton, M., Heck, D. E., Laskin, J. D., and Laskin, D. L. (2007). Acute endotoxemia is associated with upregulation of lipocalin 24p3/Lcn2 in lung and liver. Exp. Mol. Pathol. 83, 177–187. doi: 10.1016/j.yexmp.2007.03.004
Tan, B. K., Adya, R., Shan, X., Syed, F., Lewandowski, K. C., O'Hare, J. P., et al. (2009). Ex vivo and in vivo regulation of lipocalin-2, a novel adipokine, by insulin. Diabetes Care 32, 129–131. doi: 10.2337/dc08-1236
Tarín, C., Fernandez-Garcia, C. E., Burillo, E., Pastor-Vargas, C., Llamas-Granda, P., Castejón, B., et al. (2016). Lipocalin-2 deficiency or blockade protects against aortic abdominal aneurysm development in mice. Cardiovasc. Res. 111, 262–273. doi: 10.1093/cvr/cvw112
Tekkesin, N., Taga, Y., Ibrisim, D., and Gündogan, N. (2012). Urinary neutrophil gelatinase-associated lipocalin (uNGAL) levels in patients with nonalcoholic fatty liver disease. Interv. Med. Appl. Sci. 4, 132–138. doi: 10.1556/IMAS.4.2012.3.3
Triebel, S., Bläser, J., Reinke, H., and Tschesche, H. (1992). A 25 kDa α2-microglobulin-related protein is a component of the 125 kDa form of human gelatinase. FEBS Lett. 314, 386–388. doi: 10.1016/0014-5793(92)81511-J
Vazquez, D. E., Niño, D. F., De Maio, A., and Cauvi, D. M. (2015). Sustained expression of lipocalin-2 during polymicrobial sepsis. Innate Immun. 21, 477–489. doi: 10.1177/1753425914548491
Verna, E. C., Brown, R. S., Farrand, E., Pichardo, E. M., Forster, C. S., Sola-DelValle, D. A., et al. (2012). Urinary neutrophil gelatinase-associated lipocalin predicts mortality and identifies acute kidney injury in cirrhosis. Dig. Dis. Sci. 57, 2362–2370. doi: 10.1007/s10620-012-2180-x
Viau, A., Karoui, K. E., Laouari, D., Burtin, M., Nguyen, C., Mori, K., et al. (2010). Lipocalin 2 is essential for chronic kidney disease progression in mice and humans. J. Clin. Invest. 120, 4065–4076. doi: 10.1172/JCI42004
Volpe, V., Raia, Z., Sanguigno, L., Somma, D., Mastrovito, P., Moscato, F., et al. (2013). NGAL controls the metastatic potential of anaplastic thyroid carcinoma cells. J. Clin. Endocrinol. Metab. 98, 228–235. doi: 10.1210/jc.2012-2528
Waidmann, O., Köberle, V., Bettinger, D., Trojan, J., Zeuzem, S., Schultheiß, M., et al. (2013). Diagnostic and prognostic significance of cell death and macrophage activation markers in patients with hepatocellular carcinoma. J. Hepatol. 59, 769–779. doi: 10.1016/j.jhep.2013.06.008
Wei, W., Huang, X. H., Liang, D., Zeng, Y. Y., Ma, C., Wu, Y. B., et al. (2015). A proteomic analysis of transplanted liver in a rat model of chronic rejection. Clin. Res. Hepatol. Gastroenterol. 39, 340–350. doi: 10.1016/j.clinre.2014.10.005
Wieser, V., Tymoszuk, P., Adolph, T. E., Grander, C., Grabherr, F., Enrich, B., et al. (2016). Lipocalin 2 drives neutrophilic inflammation in alcoholic liver disease. J. Hepatol. 64, 872–880. doi: 10.1016/j.jhep.2015.11.037
Wilson, R., Belluoccio, D., Little, C. B., Fosang, A. J., and Bateman, J. F. (2008). Proteomic characterization of mouse cartilage degradation in vitro. Arthritis Rheum. 58, 3120–3131. doi: 10.1002/art.23789
Wong, F., and Murray, P. (2014). Kidney damage biomarkers: novel tools for the diagnostic assessment of acute kidney injury in cirrhosis. Hepatology 60, 455–457. doi: 10.1002/hep.27063
Xiong, H., Luo, T., He, W., Xi, D., Lu, H., Li, M., et al. (2016). Up-regulation of miR-138 inhibits hypoxia-induced cardiomyocyte apoptosis via down-regulating lipocalin-2 expression. Exp. Biol. Med. (Maywood) 241, 25–30. doi: 10.1177/1535370215591831
Xu, G., Ahn, J., Chang, S., Eguchi, M., Ogier, A., Han, S., et al. (2012). Lipocalin-2 induces cardiomyocyte apoptosis by increasing intracellular iron accumulation. J Biol. Chem. 287, 4808–4817. doi: 10.1074/jbc.M111.275719
Xu, M. J., Feng, D., Wu, H., Wang, H., Chan, Y., Kolls, J., et al. (2015). Liver is the major source of elevated serum lipocalin-2 levels after bacterial infection or partial hepatectomy: a critical role for IL-6/STAT3. Hepatology 61, 692–702. doi: 10.1002/hep.27447
Yan, Q. W., Yang, Q., Mody, N., Graham, T. E., Hsu, C. H., Xu, Z., et al. (2007). The adipokine lipocalin 2 is regulated by obesity and promotes insulin resistance. Diabetes 56, 2533–2540. doi: 10.2337/db07-0007
Yang, J., Bielenberg, D. R., Rodig, S. J., Doiron, R., Clifton, M. C., Kung, A. L., et al. (2009). Lipocalin 2 promotes breast cancer progression. Proc. Natl. Acad. Sci. U.S.A. 106, 3913–3918. doi: 10.1073/pnas.0810617106
Yang, J., Goetz, D., Li, J. Y., Wang, W., Mori, K., Setlik, D., et al. (2002). An iron delivery pathway mediated by a lipocalin. Mol. Cell 10, 1045–1056. doi: 10.1016/S1097-2765(02)00710-4
Ye, D., Yang, K., Zang, S., Lin, Z., Chau, H. T., Wang, Y., et al. (2016). Lipocalin-2 mediates non-alcoholic steatohepatitis by promoting neutrophil-macrophage crosstalk via the induction of CXCR2. J. Hepatol. doi: 10.1016/j.jhep.2016.05.041. [Epub ahead of print].
Ye, Z., Wang, S., Yang, Z., He, M., Zhang, S., Zhang, W., et al. (2014). Serum lipocalin-2, cathepsin S and chemerin levels and nonalcoholic fatty liver disease. Mol. Biol. Rep. 41, 1317–1323. doi: 10.1007/s11033-013-2977-5
Zeng, G., Jia, C. W., Liu, J., and Guo, S. B. (2014). Lipocalin-2 test in distinguishing acute lung injury cases from septic mice without acute lung injury. Chin. Med. Sci. J. 29, 65–77. doi: 10.1016/S1001-9294(14)60031-6
Zhang, H., Xu, L., Xiao, D., Xie, J., Zeng, H., Wang, Z., et al. (2007). Upregulation of neutrophil gelatinase-associated lipocalin in oesophageal squamous cell carcinoma: significant correlation with cell differentiation and tumour invasion. J. Clin. Pathol. 60, 555–561. doi: 10.1136/jcp.2006.039297
Zhang, W., Yang, S., Cui, L., and Zhang, J. (2016). Neutrophil gelatinase-associated lipocalin worsens ischemia/reperfusion damage of kidney cells by autophagy. Ren. Fail. 38, 1136–1140. doi: 10.3109/0886022X.2016.1158041
Zhang, Y., Fan, Y., and Mei, Z. (2012). NGAL and NGALR overexpression in human hepatocellular carcinoma toward a molecular prognostic classification. Cancer Epidemiol. 36, e294–e299. doi: 10.1016/j.canep.2012.05.012
Keywords: inflammation, liver, biomarker, alcoholic fatty liver disease, non-alcoholic steatohepatitis, matrix metalloproteinases, liver failure, hepatic disease
Citation: Asimakopoulou A, Weiskirchen S and Weiskirchen R (2016) Lipocalin 2 (LCN2) Expression in Hepatic Malfunction and Therapy. Front. Physiol. 7:430. doi: 10.3389/fphys.2016.00430
Received: 28 June 2016; Accepted: 09 September 2016;
Published: 27 September 2016.
Edited by:
Ghanshyam Upadhyay, City College of New York-CUNY, USAReviewed by:
Michael Hickey, Monash University, AustraliaHai Li, Shanghai Jiao Tong University, China
Deepak Gurbani, University of Texas Southwestern Medical Center, USA
Copyright © 2016 Asimakopoulou, Weiskirchen and Weiskirchen. This is an open-access article distributed under the terms of the Creative Commons Attribution License (CC BY). The use, distribution or reproduction in other forums is permitted, provided the original author(s) or licensor are credited and that the original publication in this journal is cited, in accordance with accepted academic practice. No use, distribution or reproduction is permitted which does not comply with these terms.
*Correspondence: Ralf Weiskirchen, rweiskirchen@ukaachen.de
†These authors have contributed equally to this work.