- 1Faculty of Physical Education, Tomsk State University, Tomsk, Russia
- 2Sports Coaching College, Beijing Sport University, Beijing, China
- 3Central Research Laboratory, Siberian State Medical University, Tomsk, Russia
Cold water immersion (CWI) is very popular as a method reducing post-exercise muscle stiffness, eliminating fatigue, decreasing exercise-induced muscle damage (EIMD), and recovering sports performance. However, there are conflicting opinions as to whether CWI functions positively or negatively. The mechanisms of CWI are still not clear. In this systematic review, we used meta-analysis aims to examine the effect of CWI on fatigue recovery after high-intensity exercise and exercise performance. A total of 20 studies were retrieved and included from PubMed, PEDro and Elsevier databases in this review. Publication years of articles ranged from 2002 to 2022. In selected studies including randomized controlled trials (RCTs) and Crossover design (COD). Analyses of subjective indicators such as delayed-onset muscle soreness (DOMS) and ratings of perceived exertion (RPE), and objective indicators such as countermovement jump (CMJ) and blood plasma markers including creatine kinase(CK), lactate/lactate dehydrogenase(LDH), C-reactive protein(CRP), and IL-6 were performed. Pooled data showed as follows: CWI resulted in a significant decline in subjective characteristics (delayed-onset muscle soreness and perceived exertion at 0 h); CWI reduced countermovement jump(CMJ) significantly at 0 h, creatine kinase(CK) was lowered at 24 h, and lactate at 24 and 48 h. There was no evidence that CWI affects C-reactive protein(CRP) and IL-6 during a 48-h recovery period. Subgroup analysis revealed that different CWI sites and water temperatures have no effect on post-exercise fatigue recovery. Recommended athletes immersed in cold water immediately after exercise, which can effectively reduce muscle soreness and accelerate fatigue recovery.
Introduction
Physiological disorders such as muscle damage, hyperthermia, dehydration, and glycogen depletion can occur after high-intensity training. Insufficient or unreasonable relaxation after long-term intensive training can adversely affect the subsequent training performance or competitions (Spencer et al., 2005; Rowsell et al., 2009). Fatigue can build up after unreasonable relaxation, which affects athletes’ physical health and decreased exercise performance (Gill et al., 2006). Exercise, training, or competing will not be affected after exercise if there is sufficient recovery time. However, when the post-exercise rest period is very short, the problem of reducing or eliminating fatigue and muscle damage, and maintaining or improving performance for the next exercise becomes very urgent.
In an attempt to alleviate fatigue and damage caused by exercise, it is imperative to fully warm up before training or competing, standardize movements during the process, and fully relax in time afterwards. Additionally, multiple external intervention methods have been adopted for athletes, including myoelectric stimulation, anti-inflammatory drugs, antioxidants, and cold water immersion(CWI) (Barnett, 2006). Among them, CWI is currently more popular in training and competing. Studies have shown that CWI reduces post-exercise muscle stiffness, eliminates fatigue, decreases exercise-induced muscle damage (EIMD), and improves athletic performance. Furthermore, athletes can accomplish more work in subsequent exercise after CWI (Roberts et al., 2014). Other evidence indicates that CWI negatively affects exercise performance (Paddon-Jones & Quigley, 1997; Sellwood et al., 2007), which may just be a placebo effect (Broatch et al., 2014; White et al., 2014). To determine the physiological mechanisms of CWI intervention and the possibility of it as an external relaxation tool during sports competitions, the effects of CWI intervention on sports fatigue and injury, as well as exercise performance after high-intensity exercise, were examined in this review.
Methods
Literature search strategies
According to the PRISMA statement, this systematic review was conducted from September 2021 and March 2022 in the PubMed, PEDro and Elsevier databases (Moher et al., 2015). The following search terms were used to find the relevant articles: 1) cold water immersion(CWI) OR cooling OR ice bath; 2) exercise performance OR sports performance; 3) fatigue OR recovery. The language restriction (articles published in English only) was adopted. Publication years of articles ranged from 2002 to 2022. In fact, articles after 2018 have not been included in present study. First of all, articles were not relevant, the control group of article did not use passive rest but other interventions. Secondly, even if the article was relevant, the researchers failed to contact the author of the article to obtain relating data.
Selection variables
Muscle soreness and fatigue recovery status, which can be characterized by delayed-onset muscle soreness (DOMS) and ratings of perceived exertion (RPE), are generally measured by subjective scales. Countermovement jump (CMJ) performance is considered an easy-to-implement and valuable indicator of muscle strength, a significant predictor of maximum speed and explosive force in sports, and a useful method for assessing mobility and functional capacity in fatigued athletes. The release of creatine kinase (CK), lactate, and lactate dehydrogenase (LDH) in plasma has been used as an indirect biochemical marker for exercise muscle injury to characterize muscle membrane disruption. IL-6 has traditionally been taken as a marker of post-exercise inflammation. C-reactive protein (CRP) is an indicator of infectious or inflammatory conditions (Clyne & Olshaker, 1999). Consequently, the indicators including DOMS, RPE, CMJ, CK, Lactate/LDH, IL-6, and CRP were included in this review to examine whether performing CWI after exercise can affect the above indicators, which were measured immediately(0 h) and/or 24 h and/or 48 h after exhaustive exercise. DOMS and RPE were defined as subjective recovery characteristics, and CMJ, CK, lactate/LDH, CRP, and IL-6 as objective recovery characteristics (Hohenauer et al., 2015). The systematic search strategy and process are shown in Figure 1.
Study inclusion and exclusion criteria
The present selected studies must include human subjects who received CWI intervention after exercise. CWI is defined as immersion in water at ≤15°C (Wilcock et al., 2006). In selected studies including randomized controlled trials (RCTs) and Crossover design (COD), the effect of post-exercise CWI on subsequent muscle soreness was examined. The inclusion criteria for studies were as follows: 1) RCTs and COD; 2) healthy humans; 3) at least one of the two outcome variables reported; 4) CWI performance at ≤15°C; 5) having performed baseline and post-training assessments of fatigue/soreness indicators; 6) the human volunteers could be of any athletic training status; 7) no sex-specific inclusion or exclusion criteria. Studies were excluded in the following cases: 1) not scrupulous experimental design; 2) duplicate publication; 3) animal experiments; 4) English language restrictions.
Data analysis
Meta-analysis calculations were performed by Revman 5.4 software. Two researchers (JL and ZH) independently extracted the general study features and specific study results. The disagreement between the two authors (JL and ZH) was resolved by consensus or third-party adjudication. The outcome indicators in the included literature were continuous variables. The mean difference (MD) was selected as the effect scale indicator for statistical analysis when the unit was unified, and standardized mean difference (SMD) was chosen when the unit or method for measurement was different. The I2 statistic was used to test the heterogeneity among the studies. There was no heterogeneity among studies when I2 = 0; there was heterogeneity among studies when I2 > 50%. Fixed-effects models were applied in case of no heterogeneity; random-effects models were implemented in case of heterogeneity. Subgroup and sensitivity analyses were performed if heterogeneity was observed, or meta-analyses were repeated after excluding studies with abnormal results. The publication bias analysis was performed by a funnel plot.
Risk of bias
Cochrane’s risk-of-bias tool was adopted to independently assess all included articles by two authors (Higgins et al., 2019). Each article was scored in the following aspects: random sequence generation, allocation concealment, blinding participants, blinding personnel, blinding outcome assessors, incomplete outcome data, and other sources of bias. They were rated ‘low’ (+) if the criteria for items at low risk of bias were met, or ‘high’ (-) if the criteria for items at high risk of bias were met. Additionally, risk of bias items were deemed “unclear” if insufficient information in the article or material led to doubtful interpretation (e.g. no symbols for indication).
Subgroup analysis
Subgroup analyses were conducted based on CWI of different body parts (shoulder, umbilicus), and the water temperature was <10°C or ≥10°C in performing CWI.
Results
Risk of bias
Blinding of participants and personnel, and blinding of outcome assessment demonstrated a high risk, whereas allocation concealment demonstrated an unclear risk. Random allocation of participants was used only in five studies (Rupp et al., 2012; Glasgow et al., 2014; Machado et al., 2016; Siqueira et al., 2018). Blinding of the participants and blinding of outcome assessment were only found in two studies (Glasgow et al., 2014; Machado et al., 2016). Detailed information about the risk of bias is shown in Figures 2, 3.
Research characteristics
The characteristics of the selected studies are shown in Table 1. A total of 20 studies were included in the analysis (Bosak et al., 2006; Halson et al., 2008; Ingram et al., 2009; Peiffer et al., 2010a; Parouty et al., 2010; Brophy-Williams et al., 2011; Pointon et al., 2012a; Pointon and Duffield, 2012; Rupp et al., 2012; Glasgow et al., 2014; Minett et al., 2014; Roberts et al., 2014; Takeda et al., 2014; Fonseca et al., 2016; Machado et al., 2016; Amir et al., 2017; Argus et al., 2017; Chow et al., 2018; Siqueira et al., 2018; Wiewelhove et al., 2018). And the trials were used as a primary data source for this review. The meta-analysis was adopted in the introduction and conclusion sections. There were a total of n = 419 healthy subjects including males (n = 360) and females (n = 59) in the selected articles, whereas only n = 357 healthy participants were involved in the experiments.
Characteristics of exercise protocols
The exercise protocols included football matches, rugby match simulations (Takeda et al., 2014), cycling (Halson et al., 2008; Peiffer et al., 2010a), swimming (Parouty et al., 2010), jumping (Amir et al., 2017; Siqueira et al., 2018), and running (Bosak et al., 2006; Ingram et al., 2009; Pointon et al., 2012a; Minett et al., 2014; Takeda et al., 2014; Chow et al., 2018; Wiewelhove et al., 2018). All running protocols were endurance running ones. Two authors adopted the YoYo intermittent recovery test (Brophy-Williams et al., 2011; Rupp et al., 2012). In one study, jiu-jitsu was used to ensure the pre-cooling load (Fonseca et al., 2016). In a total of two studies, the functional exercise was chosen (Roberts et al., 2014; Argus et al., 2017). The eccentric contraction was adopted in two articles (Glasgow et al., 2014; Machado et al., 2017). A detailed overview of the performed exercise protocols is shown in Table 1.
Characteristics of CWI
In the present included studies, the most common body parts of CWI were submerged to the iliac crest, the legs immersed, to ensure that the lower limbs were fully submerged in the tubs. The water temperature for immersion to the iliac crest ranged from 5°C to 15°C (Pointon et al., 2012a; Pointon and Duffield, 2012; Machado et al., 2016; Amir et al., 2017; Chow et al., 2018; Siqueira et al., 2018; Wiewelhove et al., 2018), including the water temperature for immersion to the umbilicus (Ingram et al., 2009; Rupp et al., 2012). In the other studies, it was ensured that participants were submerged in the water bath, with the water immersion to the mid-sternal level (Halson et al., 2008; Peiffer et al., 2010a; Brophy-Williams et al., 2011; Glasgow et al., 2014; Minett et al., 2014) and shoulders (Parouty et al., 2010; Roberts et al., 2014; Takeda et al., 2014; Fonseca et al., 2016; Argus et al., 2017). The water temperature was 6°C–15°C in both mid-sternal and shoulder immersion studies.
Characteristics of the passive Control group
Common passive CONs were sitting on a chair in a room with a normal temperature between 6°C and 32°C, and a relative humidity between 37% and 62%. Active cyclists were taken as the CON group because athletes usually perform low-intensity warm-ups to maintain their body temperature instead of passively waiting when resting between competitions (Roberts et al., 2014). It was decided that this article was not excluded in this review, because active recovery was chosen over passive recovery in it. Due to the low intensity of the active exercise, this interference could be negligible (subjects can self-select the intensity on an ergometer).
CMJ
Current studies indicate that CMJ immediately and 24 h after CWI are significantly different from that in the CON group (immediately: MD -2.71, 95%CL -5.03 to -0.39, 5 trials); (24 h: MD 4.77, 95%CL 2.12 to 7.42, 4 trials); but there was no significant different between the CWI and CON groups at 48 h (MD -1.60, 95%CL -4.98 to 1.79, two trials) (Figure 4). However, there was significant heterogeneity in the data at 24 h (I2 = 86%), so a random effects model was used. At this point, there was no significant difference in the CMJ between the CWI and CON groups at 24 h (SMD 5.72, 95%CL -1.83 to 13.27, 4 trials). And the CMJ results after the intervention were unchanged from those at 0 and 48 h, respectively.
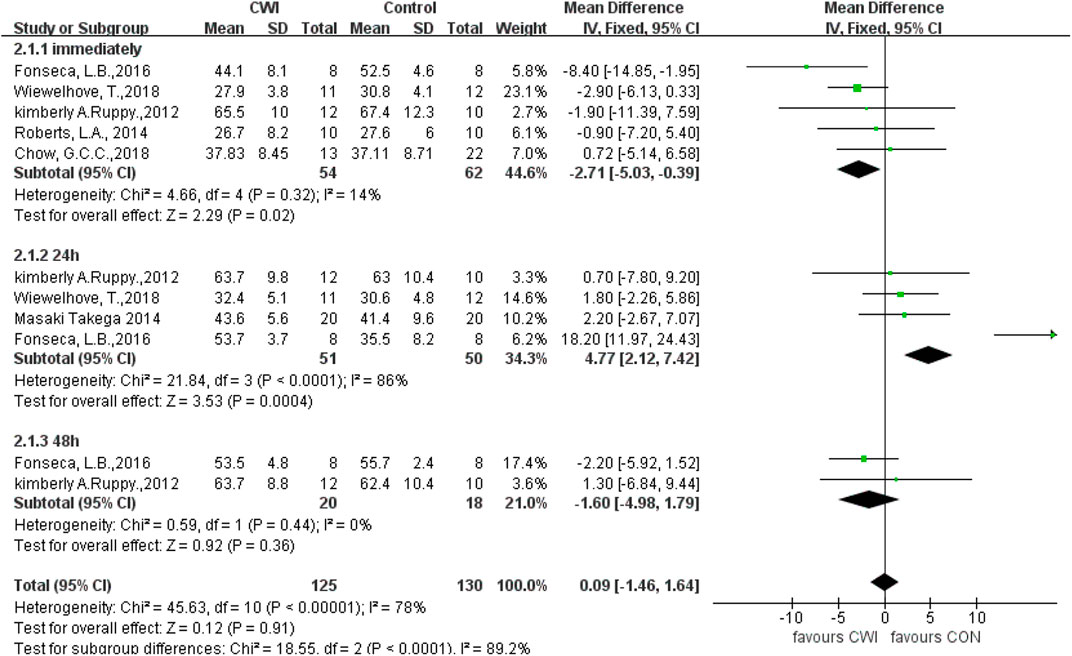
FIGURE 4. Forest plot of the comparison of CWI versus CON for measurement of CMJ. CWI = Cold water immersion, CON = Control, CMJ = countermovement jump.
A sensitivity analysis was performed to investigate whether heterogeneity among studies was caused by individual studies. At 24h, heterogeneity decreased after excluding the study by Fonseca, L, B (Chi2 = 0.09, df = 2 (p = 0.96), I2 = 0%), showing that this study was the source of heterogeneity, but with the effect size unchanging significantly at this time (MD 1.81 95%CL -1.11 to 4.74, 3 trails), suggesting stable study results.
Subgroup analysis was performed on potential moderator variables to further explore the sources of heterogeneity in CMJ at 24 h. The groups were divided into the navel and shoulder immersion ones according to CWI of different body parts, and subgroup analysis was performed, which revealed heterogeneity in the shoulder immersion group at 24 h (Chi2 = 15.71, df = 1 (p < 0.0001), I2 = 94%). And hence a random effects model was used, and the results showed no significant difference. There was no heterogeneity in the umbilicus immersion group at 24 h (Chi2 = 0.05, df = 1 (p = 0.82), I2 = 0%), so a fixed-effects model was used, and the results showed no significant differences. And there was no significant difference between the two subgroups of CWI to the shoulder and the umbilicus (Test for subgroup differences: Chi2 = 1.07, df = 1(p = 0.30), I2 = 6.2%), indicating that CWI of different body parts was not a source of heterogeneity and that CWI to different sites was ineffective in improving CMJ performance at 24 h after exercise.
CWI participants were divided into two groups at <10°C and ≥10°C, and subgroup analysis revealed significant differences between the two groups at 24 h (Test for subgroup differences: Chi2 = 21.75, df = 1 (p < 0.00001), I2 = 95.4%), suggesting that the difference in cold water temperature accounts for heterogeneity. Post-exercise immersion in cold water at a temperature <10°C can enhance CVJ at 24 h. However, since there was only 1 article involving a <10°C group, this may contribute to the uncertainty of the results.
DOMS
Among the DOMS indicators, a total of seven articles were selected by different scales, visual analogue scale/score(VAS) 1–100 mm, 1–10 cm, 0–10 cm, and therefore SMD was chosen for analysis (Figure 5). The DOMS was measured 0h, 24h, and 48 h after the experimental intervention. The results showed that DOMS levels in the CWI group were significantly lower than those in the CON group at 0h, 24 h (0 h: SMD -0.59, 95%CL -0.90 to -0.28, 6 trials); (24 h: SMD -0.34, 95%CL -0.65 to -0.04, 7 trials), and no significant difference was found between the CWI and CON groups at 48 h (48 h: SMD -0.25, 95%CL -0.58 to 0.07, 6 trials). Heterogeneity was found between the literature at 24 and 48 h (24 h: I2 = 67%; 48 h: I2 = 66%), and hence the random effects model was chosen. The DOMS results were unchanged at 0h, and there was no longer a significant difference at 24 h (24 h: SMD -0.50, 95%CL -1.05 to 0.04, 7 trials); (48 h: SMD -0.41, 95%CL -0.98 to 0.71, 6 trials). The results showed that post-exercise CWI intervention was effective in reducing DOMS immediately, but not for prolonged periods of time (24 h, 48 h).
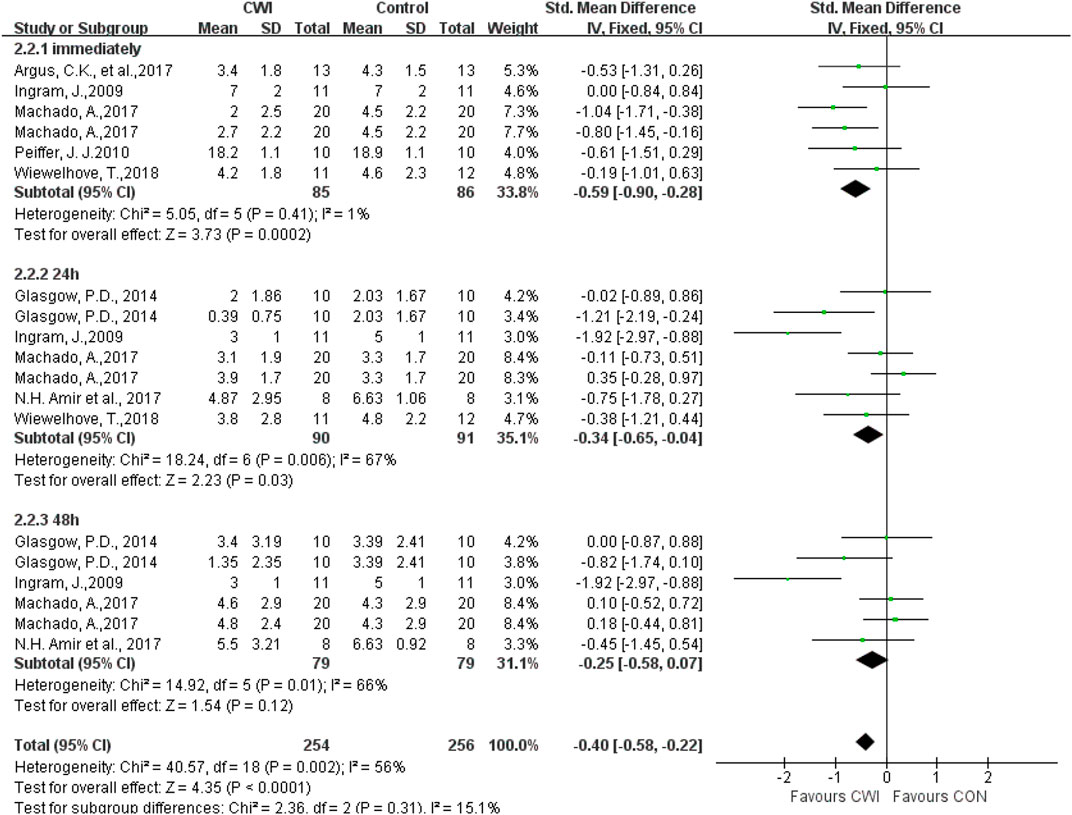
FIGURE 5. Forest plot of the comparison of CWI versus CON for measurement of DOMS. CWI = Cold water immersion, CON = Control, DOMS = delayed-onset muscle soreness.
A sensitivity analysis was performed by the one-by-one exclusion of literature to investigate whether the heterogeneity between studies was caused by individual studies. The heterogeneity was found to decrease after excluding the study by Ingram et al., 2009 at 24 and 48 h (24 h: Chi2 = 8.66, df = 5 (p = 0.12), I2 = 42%); (48 h: Chi2 = 4.08, df = 4(p = 0.40), I2 = 2%), showing that this study was the source of heterogeneity. Nevertheless, the effect size did not change significantly (24 h: SMD -0.26 95%CL -0.69 to 0.16, 6 trails); (48 h: SMD -0.08 95%CL -0.42 to 0.26, 5 trails), suggesting stable study results.
CWI subgroup analysis was performed based on CWI of different body parts to further explore potential sources of heterogeneity. Heterogeneity was observed in the shoulder immersion group at 24 h (Chi2 = 3.21, df = 1(p = 0.07), I2 = 69%). A random effects model was used and there was no significant difference in results. There was no heterogeneity at 48 h (Chi2 = 1.61, df = 1(p = 0.20), I2 = 38%), and the results were not significantly different when a fixed effects model was used, indicating that post-exercise CWI to the shoulder was ineffective in changing muscle soreness at 24 and 48 h. Heterogeneity also existed in the umbilicus immersion group at 24 and 48 h (24 h: Chi2 = 14.52, df = 4(p = 0.006), I2 = 72%); (48 h: Chi2 = 13.08, df = 3(p = 0.004), I2 = 77%), and none of the differences were statistically significant when a random effects model was used, indicating that post-exercise CWI to the umbilicus was ineffective in altering muscle soreness at 24 or 48 h. There were no significant differences between the two subgroups of CWI to the shoulder and the umbilicus (Test for subgroup differences: 24 h: Chi2 = 0.03, df = 1 (p = 0.87), I2 = 0%; 48 h: Chi2 = 0.01, df = 1 (p = 0.94), I2 = 0%), indicating that the difference in CWI sites is not a factor in DOMS heterogeneity.
Subgroup analysis was performed based on the difference in CWI temperature, revealing heterogeneity in the <10°C group at 24 and 48 h (24 h: Chi2 = 3.53, df = 1(p = 0.06), I2 = 72%), (48 h: Chi2 = 2.65, df = 1(p = 0.10), I2 = 62%). A random effects model was used and no significant effect on the results was found. Heterogeneity was found in the ≥10°C group at 24 and 48 h (24 h: Chi2 = 14.57, df = 4(p = 0.006), I2 = 73%), (48 h: Chi2 = 12.16, df = 3(p = 0.007), I2 = 75%). Then, a random effects model was used to show no significant effect on the results. Also, it was discovered that at <10°C or ≥10°C, post-exercise CWI was both ineffective in changing DOMS at 24 and 48 h. In addition, there was no significant difference between the two subgroups at different CWI temperatures (Test for subgroup differences: 24 h: Chi2 = 0.03, df = 1 (p = 0.86), I2 = 0%; 48 h: Chi2 = 0.09, df = 1 (p = 0.76), I2 = 0%). Therefore, the changes in CWI temperature do not contribute to heterogeneity between DOMS groups.
RPE
A total of five articles were selected to analyze the RPE in the CWI group versus the CON group. Different measurement tools were chosen for different articles, including 0–10 and 1–10 scales, and hence the SMD was selected (Figure 6). The data showed that there was a significant difference in RPE between the CWI and CON groups at 0 h (SMD -0.57, 95%CL -0.86 to -0.28, 6 trials), but no significant difference was found between the CWI and CON groups at 24 and 48 h (24 h: SMD -0.20, 95%CL -0.53 to 0.13,4 trials); (48 h: SMD 0.09, 95%CL -0.30 to 0.48, 3 trials). Compared with passive recovery, CWI reduced subjective fatigue immediately after long-term exercise but did not help prolonged fatigue.
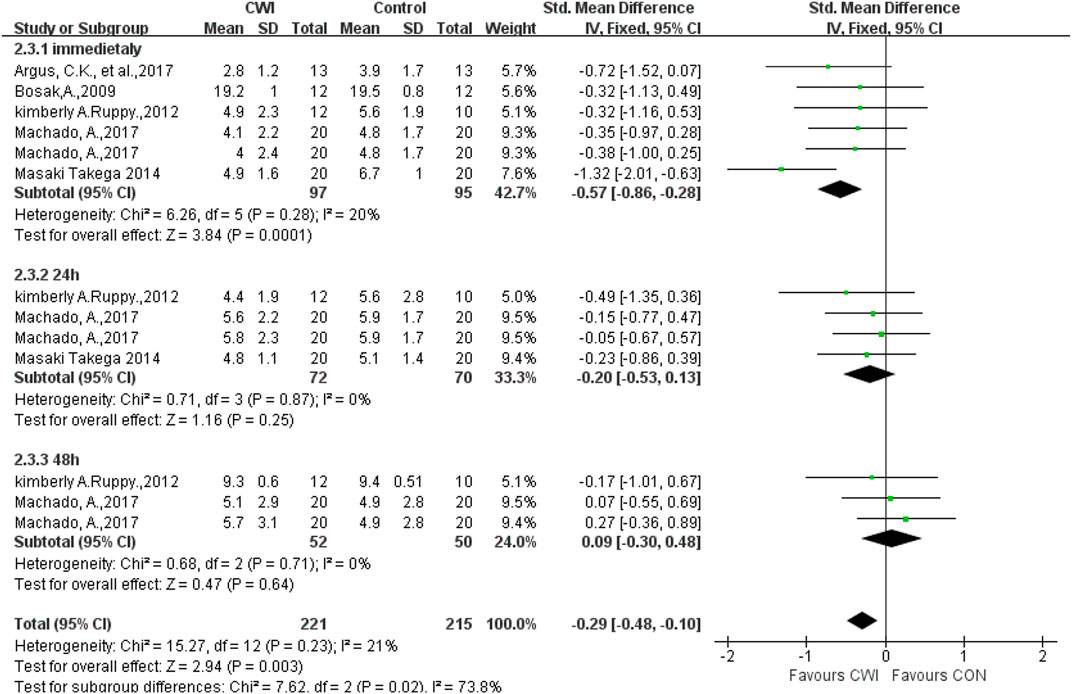
FIGURE 6. Forest plot of the comparison of CWI versus CON for measurement of RPE. CWI = Cold water immersion, CON = Control, RPE = rate of perceived exertion.
CK
Twelve articles were selected to determine whether post-exercise CWI affects CK at 0, 24, and 48 h (Figure 7). The results showed a significant difference between the CWI and the CON groups only at 24 h after the experimental intervention (MD -86.04 95% CL -153.88 to -18.21, 13 trials), with CK levels significantly lower at 24 h after the CWI intervention than after passive recovery. In contrast, there was no statistically significant difference between the CWI and CON groups at both 0 and 48 h (0 h: MD -2.63 95%CL -36.72 to -31.47, 7 trails); (48 h: MD -5.44 95%CL -62.93 to 52.04, 7 trials).
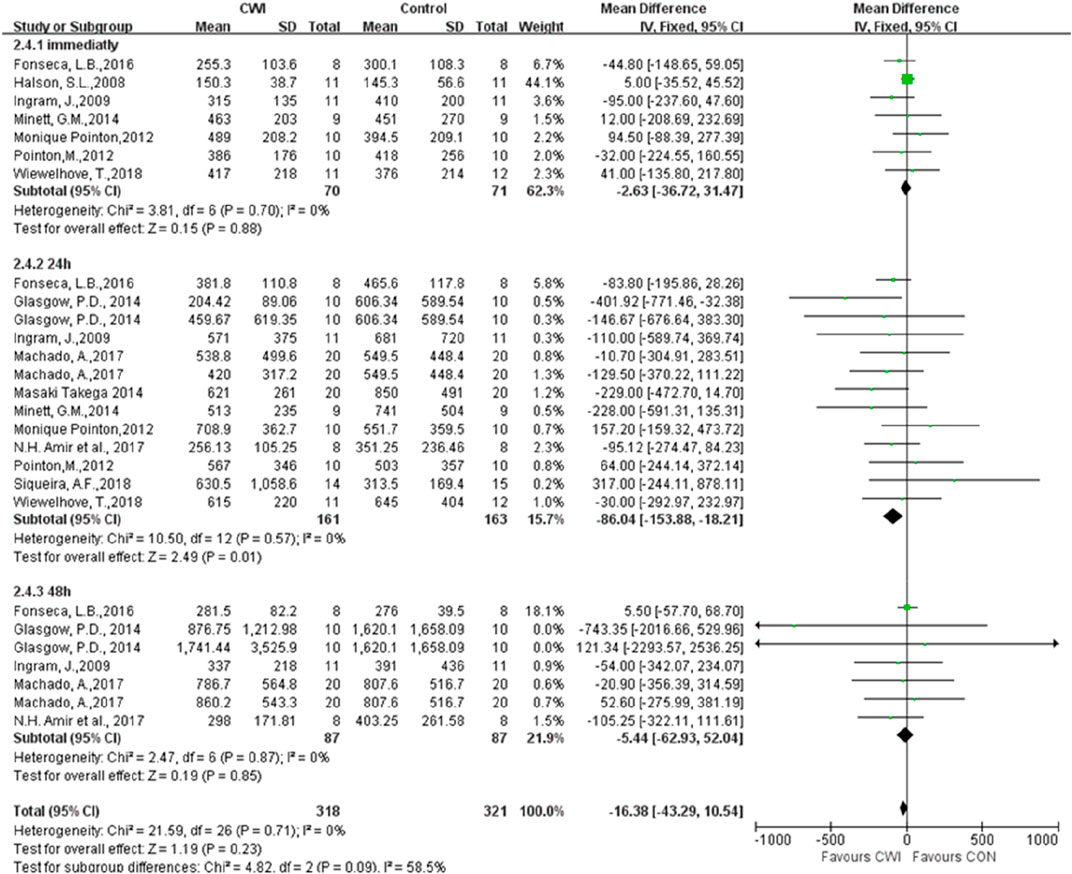
FIGURE 7. Forest plot of the comparison of CWI versus CON for measurement of CK. CWI = Cold water immersion, CON = Control, CK = creatine kinase.
Lactate
Analysis of the effects of post-exercise CWI and passive recovery on lactate revealed significant differences in lactate indicators at 24 and 48 h after intervention (24 h SMD -0.61 95%CL -1.08 to -0.13, 3 trials); (48 h SMD -0.82 95%CL -1.56 to -0.08, two trials) but no significant differences at 0 h (SMD 0.05 95%CL -0.37 to 0.47, 5 trials). Heterogeneity was found among the included literature at 0 h (Chi2 = 9.41, df = 4 (p = 0.05), I2 = 58%), and there was no statistical significance immediately after selecting the random effects model (SMD -0.01 95%CL -0.67 to 0.64, 5 trials) (Figure 8).
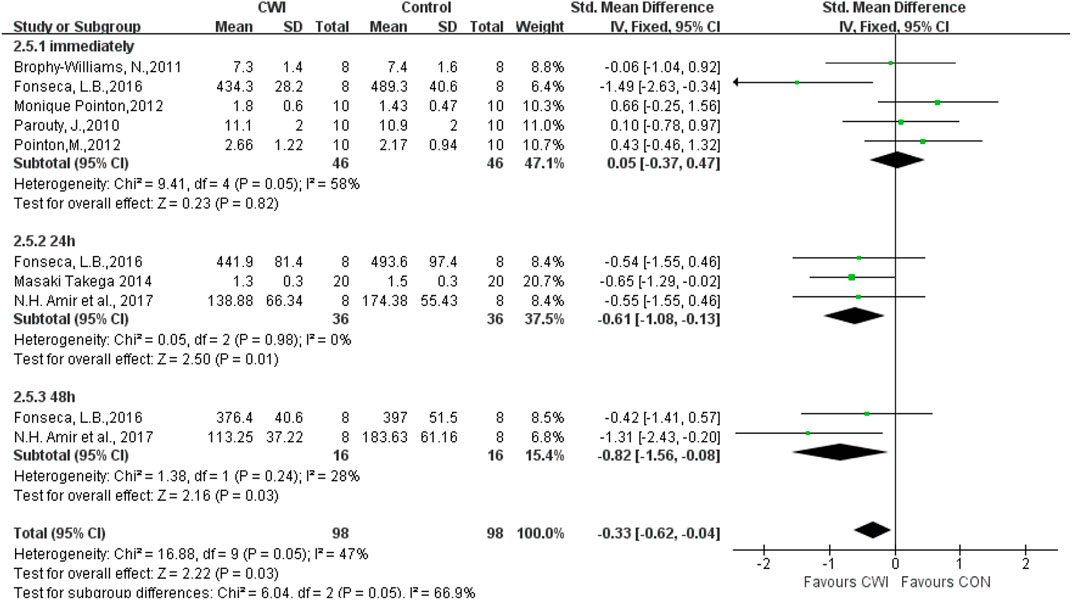
FIGURE 8. Forest plot of the comparison of CWI versus CON for measurement of Lactate. CWI = Cold water immersion, CON = Control.
A sensitivity analysis was conducted by the one-by-one exclusion method to investigate whether the heterogeneity among studies was caused by individual articles. It was found that heterogeneity decreased when Fonseca et al., 2016 was excluded at 0 h (Chi2 = 1.42, df = 3 (p = 0.70), I2 = 0%), indicating that this article contributes to heterogeneity in present included studies. However, the effect size did not change significantly after exclusion (SMD 0.29 95%CL -0.16 to 0.75, 4 trials), suggesting stable study results.
For purpose of further exploring potential sources of heterogeneity, subgroup analysis was performed based on CWI of different body parts. Heterogeneity was found in the shoulder immersion group at 0 h (Chi2 = 5.51, df = 2 (p = 0.08), I2 = 61%), and then a random effects model was chosen. The results of the shoulder immersion group were not significantly different (SMD -0.43 95%CL -1.35 to 0.49, 3 trials), indicating that CWI to the shoulder is ineffective in reducing exercise-induced lactic acid accumulation immediately. Moreover, there was no heterogeneity in the umbilicus immersion group (Chi2 = 0.12, df = 1 (p = 0.73), I2 = 0%), and thus a fixed-effects model was used to show no significantly different results (SMD 0.54 95%CL -0.09 to 1.18, 2 trials), indicating that CWI to the umbilicus does not change the lactate at 0 h. No significant differences between the two subgroups at 0 h (Test for subgroup differences at 0 h: Chi2 = 2.89, df = 1 (p = 0.09), I2 = 65.4%), indicating that CWI of different body parts does not cause heterogeneity in the lactate group at 0 h.
Subgroup analysis was performed based on the difference in CWI temperature. Heterogeneity was observed in the <10°C group at 0 h (Chi2 = 9.35, df = 2(p = 0.009), I2 = 79%). And therefore a random effects model was selected and the results were not significantly different (SMD -0.08 95%CL -1.30 to 1.13, 3 trials), suggesting that CWI at a temperature <10°C does not affect lactate accumulation at 0 h after intervention. There was no heterogeneity in the ≥10°C group (Chi2 = 0.06, df = 1 (p = 0.81), I2 = 0%), so a fixed effects model was chosen. And the results were not significantly different (SMD 0.03 95%CL -0.63 to 0.68, 2 trials), indicating that water temperature ≥10°C is ineffective in reducing lactate immediately after CWI. There was no significant difference between the two subgroups of CWI at different water temperatures (Test for subgroup differences at 0 h: Chi2 = 0.02, df = 1 (p = 0.88), I2 = 0%), which implied that different CWI temperatures are not a source of heterogeneity in the lactate at 0 h.
CRP
In a total of 7 studies, CRP levels were recorded after the intervention experiment. There was no significant difference in CRP at 0, 24, or 48 h (0 h MD -0.01 95%CL -0.06 to 0.04, 5 trials); (24 h MD 0.06 95%CL -0.45 to 0.57, 6 trials); (48 h MD -0.20 95%CL -0.96 to 0.56, 1trials). Only 1 article was included at 48h, and thus the data at 48 h were ignored (Figure 9).
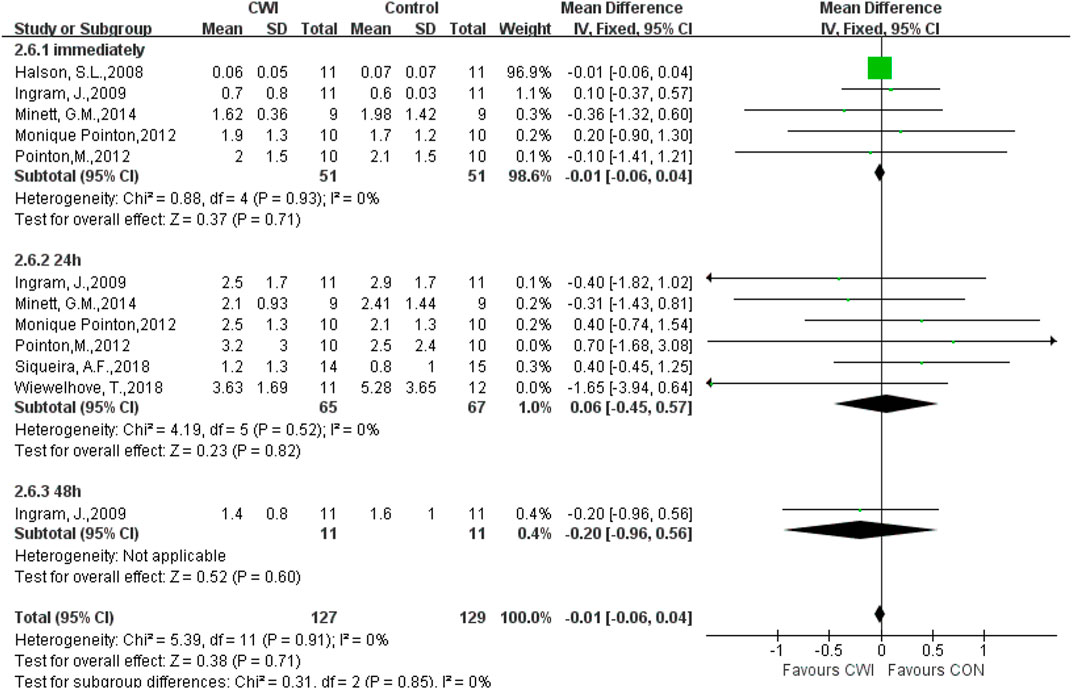
FIGURE 9. Forest plot of the comparison of CWI versus CON for measurement of CRP. CWI = Cold water immersion, CON = Control, CRP = C-Reactive Protein.
IL-6
Only a few articles were selected to analyze IL-6, rendering the results meaningless. Meanwhile, there were no significant differences in inflammatory markers (IL-6), which implied that performing CWI after exercise has no effect on the subsequent physical recovery of the subjects (Figure 10).
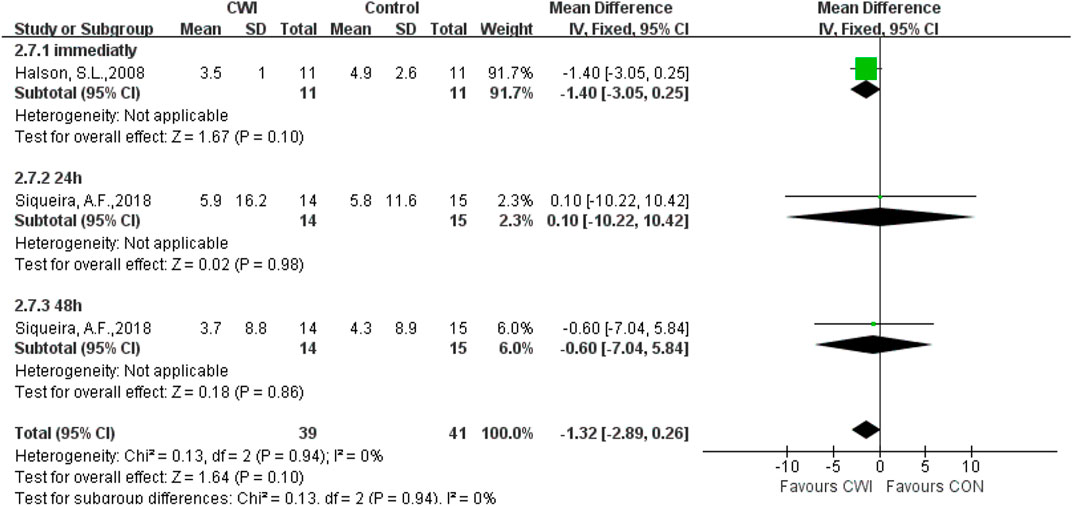
FIGURE 10. Forest plot of the comparison of CWI versus CON for measurement of IL-6.CWI = Cold water immersion, CON = Control, IL-6 = Interleukin 6.
Discussion
Methodological analysis of included literature
First, the majority of studies had a high or unclear risk of bias, rendering the validity of most results uncertain. The main bias was produced by unblinding in experiments, which is due to the limited possibility of blinding during CWI application. Second, in terms of the allocation concealment procedure, the random allocation of participants was used in only four studies; in two studies, the envelope was used to conceal distribution (Rupp et al., 2012; Glasgow et al., 2014) and in the others two, computers were adopted to ensure random allocation (Machado et al., 2016; Siqueira et al., 2018). Third, random-CON and crossover trials were separately used in different studies of this review. CODs can risk certain carry-over effects between treatment periods that are not present in random-CON designs. In order to improve methodological quality, more randomized controlled trials, allocation concealment, and blind assessors can be included in studies.
Subjective recovery characteristics
One of the main findings of this review was that post-exercise CWI reduced DOMS and RPE immediately, but with no significant effect on DOMS and RPE at 24 and 48 h (Table 2). It was found by sensitivity analysis that the heterogeneity between DOMS groups might be caused by the study by Ingram, J., in 2009. The reason for this may be the short duration of CWI intervention in this article, which was only 2 min × 5 times with 2.5 min apart. It has been suggested that the short duration of immersion is less effective in relieving exercise-induced muscle (Getto & Golden, 2013).
The physiological mechanisms by which CWI performance after high-intensity exercise can immediately reduce DOMS and RPE may be explained as follows. 1. CWI can reduce edema, pain, and metabolite accumulation by lowering body temperature and regulating central nervous system mechanisms (Vaile et al., 2011; Leeder et al., 2012). Immersion in cold water results in a decline in acetylcholine production, which in turn causes a decrease in neuronal transmission rate and the ability to reduce muscle spasm. Also, a 10°C–13°C decrease in the skin temperature can reduce nerve conduction velocity by 10%–33% (Algafly & George, 2007). 2. CWI causes vasoconstriction due to hydrostatic pressure, which increases venous blood return, thus reducing edema and pain, and accelerating metabolic elimination (Wilcock et al., 2006). 3. CWI has been reported to reduce lymphatic and capillary permeability (Wilcock et al., 2006), thereby reducing fluid diffusion, which may help reduce exercise-induced inflammation, as well as edema and pain (Wilcock et al., 2006). 4. CWI can provide a placebo effect on subjects to some extent. Double-blind bias during the experiment can affect the judgment of the subjects, causing a placebo effect.
Objective recovery characteristics
The main conclusion of this review is that CWI reduces CMJ at 0h, CK at 24h, and lactate at 24h and 48 h after intervention. However, post-exercise CWI does not produce any effect on CRP or IL-6 (Table 2). It was found by sensitivity analysis that the heterogeneity between CMJ studies may be caused by the study of Fonseca, L.B., in which the CWI group was immersed in cold water at 6°C ± 0.5°C for 16 min. One study indicated that immersion in cold water at 11°C–15°C for 11–15 min is the most appropriate and beneficial for fatigue recovery after exercise (Machado et al., 2016). In this review, studies involving a CWI group at 11°C–15°C were mainly selected. Hence, the great difference in water temperature between the study by Fonseca and the others may be the reason for the heterogeneity between them.
This review suggests that CMJ immediately decrease after CWI, which is consistent with the findings from several studies (Fischer et al., 2009; Nuhmani et al., 2012). The evaluation of the effect of cryotherapy on physical performance showed a 17.5% decline in vertical jump performance immediately after CWI (Patterson et al., 2008). A 37% reduction was observed in vertical jumps immediately when the objects underwent CWI (Didehdar and Sobhani, 2019). The jump height was discovered to decrease by 4.2% for every 1°C decrease in muscle temperature (Bergh & Ekblom, 1979). The reason is that cold muscles can result in lower nerve impulse frequency and longer relaxation time.
The reasons for the decrease in CMJ performance immediately caused by CWI are as follows. 1. CWI decreased the skeletal muscle temperature, resulting in an increase in skeletal muscle viscosity. The CMJ is a plyometric contraction exercise. When the muscle is elongated, elastic potential energy and stretch reflex contraction will be generated; when the elastic potential energy, stretch reflex contraction, and active concentric contraction form a resultant force, the muscle will produce a larger contraction force. Increasing the muscle viscosity can decrease muscle extension and elasticity, and impair elastic potential energy, which ultimately causes a decrease in CMJ performance. 2. CWI affects the exchange between Ca2+ and Na2+ in nerve cells, which may lead to a delay in action potential generation, contraction velocity, and force-producing capacity, thus reducing dynamic contraction force and the subsequent motor performance (Machado et al., 2016). Meanwhile, studies have shown a linear relationship among muscle temperature, nerve conduction rate, and muscle activation through electromyography (EMG) recordings. When the muscle temperature is low, the nerve conduction rate decreases, and the central nervous system becomes less excitable (Machado et al., 2017), with the maximum muscle strength declining.
Post-exercise CWI could decrease CK levels at 24 h. These results are similar to the findings of the study by Ascensao et al., in which it was concluded that performing CWI for athletes at 10°C for 10 min after a simulated soccer match reduces serum CK levels at 24 h (Ascensao et al., 2011). Also, they are similar to the results of the other meta-analyses (Higgins et al., 2017). CWI could reduce lactate levels at 24 and 48 h. However, CWI did not affect CRP or IL-6. An inverse relationship was observed between serum levels of LDH, with muscle soreness perceived (Júnior et al., 2014), which was consistent with the results of this review. Lactate levels did not change significantly but DOMS levels significantly decreased from 0 h by CWI application after exercise, compared with passive recovery. However, while CWI significantly decreased lactate levels, it did not change DOMS levels at 24 and 48 h after exercise.
As previously stated, local cooling may change the skeletal blood flow by reducing the capillary permeability (Eston & Peters, 1999; Vaile et al., 2011). Also, it may decrease the passive leakage of intracellular substances utilized as subliminal indicators of muscle injury, such as decreased CK efflux. Prolonged analgesia may result from intravascular fluid shifts due to vasoconstriction, thus facilitating nutrient and waste transport, as well as reducing muscle edema (Cheung et al., 2003; Ihsan et al., 2013; Yanagisawa et al., 2014). In addition, there were inadequate data on the other biochemical indicators such as CRP and IL-6, and thus meta-analyses were difficult to conduct.
Subgroup analysis characteristics
Differences in body parts and the water temperature in CWI application did not affect subjective fatigue recovery, nor did they affect objective recovery. This was different from the study result by Poppendieck W (Poppendieck et al., 2013), indicating that whole-body CWI is more effective than partial-body cooling. The reason is that whole-body CWI reduces the core body temperature effectively. Moreover, whole-body cooling is subjected to higher hydrostatic pressure than partial-body cooling.
Limitations
Limitations in study designs were also identified. First, we have chosen articles from 2002 to 2022, but in fact articles just to 2018. Second, blinding of participants in studies was problematic, especially with hydrotherapy, which left them vulnerable to the placebo effect. Thirdly, the subgroup analysis was not comprehensive. The subgroup analysis was based solely on different body parts that underwent CWI, and the different temperatures. There was a lack of comparisons of differences between eccentric and high-intensity exercise and between genders. Fourthly, grouping together the active population, recreational athletes and professional athletes limits the applicability of this present findings.
Conclusion
CWI is commonly used as a fatigue-recovery measure after exercise. In this review, the effect of CWI on post-exercise fatigue recovery was systematically analyzed. Subjective sensations seemed to recover immediately, as did objective biochemical markers such as CK and lactate after 24 h. CWL of different body parts and different water temperatures have no effect on fatigue recovery.
In future, a randomized controlled study design needs to be adopted as much as possible in studies to improve the methodological quality. Also, a double-blind design should be conducted for subjects and experimenters whenever possible to ensure that they are not affected by each other’s placebo. Additionally, it is necessary to perform multidimensional subgroup analyses such as different gender and exercise intensity. Furthermore, the dose of CWI should be reconsidered.
Data availability statement
The original contributions presented in the study are included in the article/supplementary material, further inquiries can be directed to the corresponding author.
Author contributions
FX was mainly responsible for data processing and writing the paper, while LJ and HZ were mainly responsible for literature search and data collection. KAV and KLV was mainly responsible for the methodological guidance and the erratum of statements.
Funding
This study was supported by the Tomsk State University Development Programme (Priority-2030).
Conflict of interest
The authors declare that the research was conducted in the absence of any commercial or financial relationships that could be construed as a potential conflict of interest.
Publisher’s note
All claims expressed in this article are solely those of the authors and do not necessarily represent those of their affiliated organizations, or those of the publisher, the editors and the reviewers. Any product that may be evaluated in this article, or claim that may be made by its manufacturer, is not guaranteed or endorsed by the publisher.
References
Algafly A. A., George K. P. (2007). The effect of cryotherapy on nerve conduction velocity, pain threshold and pain tolerance. Br. J. sports Med. 41, 365–369. doi:10.1136/bjsm.2006.031237
Amir N. H., Hashim H. A., Saha S. (2017). The effect of single bout of 15 minutes of 15-degree celsius cold water immersion on delayed-onset muscle soreness indicators. Singapore: Springer.
Argus C. K., Broatch J. R., Petersen A. C., Polman R., Bishop D. J., Halson S. (2017). Cold-water immersion and contrast water therapy: No improvement of short-term recovery after resistance training. Int. J. Sports Physiology Perform. 12, 886–892. doi:10.1123/ijspp.2016-0127
Ascensao A., Leite M., Rebelo A. N., Magalhaes S., Magalhaes J. (2011). Effects of cold water immersion on the recovery of physical performance and muscle damage following a one-off soccer match. J. Sports Sci. 29, 217–225. doi:10.1080/02640414.2010.526132
Barnett A. (2006). Using recovery modalities between training sessions in elite athletes. Sports Med. 36, 781–796. doi:10.2165/00007256-200636090-00005
Bergh U., Ekblom B. (1979). Influence of muscle temperature on maximal muscle strength and power output in human skeletal muscles. Acta physiol. Scand. 107, 33–37. doi:10.1111/j.1748-1716.1979.tb06439.x
Bosak A., Bishop P., Smith J., Green M., Richardson M., Iosia M. (2006). Impact of cold water immersion on 5km racing performance. Med. Sci. Sports Exerc. 38, S233. doi:10.1249/00005768-200605001-01901
Broatch J. R., Petersen A., Bishop D. J. (2014). Postexercise cold water immersion benefits are not greater than the placebo effect. Med. Sci. Sports Exerc 46, 2139–2147. doi:10.1249/MSS.0000000000000348
Brophy-Williams N., Landers G., Wallman K. (2011). Effect of immediate and delayed cold water immersion after a high intensity exercise session on subsequent run performance. J. Sports Sci. Med. 10, e114–e170. doi:10.1016/j.jsams.2011.11.238
Cheung K., Hume P. A., Maxwell L. (2003). Delayed onset muscle soreness: Treatment strategies and performance factors. Sports Med. 33, 145–164. doi:10.2165/00007256-200333020-00005
Chow G. C. C., Chung J. W. Y., Fong S. S. M. (2018). Differential effects of post-exercise ice water immersion and room temperature water immersion on muscular performance, vertical jump, and agility in amateur rugby players: A randomized controlled trial. Sci. Sports 33, E271–E279. doi:10.1016/j.scispo.2018.04.005
Clyne B., Olshaker J. S. (1999). The C-reactive protein. J. Emerg. Med. 17, 1019–1025. doi:10.1016/s0736-4679(99)00135-3
Didehdar D., Sobhani S. (2019). The effect of cold-water immersion on physical performance. J. Bodyw. Mov. Ther. 23, 258–261. doi:10.1016/j.jbmt.2018.05.001
Eston R., Peters D. (1999). Effects of cold water immersion on the symptoms of exercise-induced muscle damage. J. sports Sci. 17, 231–238. doi:10.1080/026404199366136
Fischer J., Van Lunen B. L., Branch J. D., Pirone J. L. (2009). Functional performance following an ice bag application to the hamstrings. J. Strength & Cond. Res. 23, 44–50. doi:10.1519/JSC.0b013e3181839e97
Fonseca L. B., Brito C. J., Silva R. J. S., Silva-Grigoletto M. E., Da Silva W. M., Franchini E. (2016). Use of cold-water immersion to reduce muscle damage and delayed-onset muscle soreness and preserve muscle power in jiu-jitsu athletes. J. Athl. Train. 51, 540–549. doi:10.4085/1062-6050-51.9.01
Getto C. N., Golden G. (2013). Comparison of active recovery in water and cold-water immersion after exhaustive exercise. Athl. Train. Sports Health Care 5, 169–176. doi:10.3928/19425864-20130702-03
Gill N. D., Beaven C., Cook C. (2006). Effectiveness of post-match recovery strategies in rugby players. Br. J. sports Med. 40, 260–263. doi:10.1136/bjsm.2005.022483
Glasgow P. D., Ferris R., Bleakley C. M. (2014). Cold water immersion in the management of delayed-onset muscle soreness: Is dose important? A randomised controlled trial. Phys. Ther. sport 15, 228–233. doi:10.1016/j.ptsp.2014.01.002
Halson S. L., Quod M. J., Martin D. T., Gardner A. S., Ebert T. R., Laursen P. B. (2008). Physiological responses to cold water immersion following cycling in the heat. Int. J. Sports Physiology Perform. 3, 331–346. doi:10.1123/ijspp.3.3.331
Higgins J. P., Thomas J., Chandler J., Cumpston M., Li T., Page M. J., et al. (2019). Cochrane handbook for systematic reviews of interventions. Hoboken, New Jersey, U.S: John Wiley & Sons.
Higgins T. R., Greene D. A., Baker M. K. (2017). Effects of cold water immersion and contrast water therapy for recovery from team sport: A systematic review and meta-analysis. J. Strength & Cond. Res. 31, 1443–1460. doi:10.1519/JSC.0000000000001559
Hohenauer E., Taeymans J., Baeyens J.-P., Clarys P., Clijsen R. (2015). The effect of post-exercise cryotherapy on recovery characteristics: A systematic review and meta-analysis. PloS one 10, e0139028. doi:10.1371/journal.pone.0139028
Ihsan M., Watson G., Lipski M., Abbiss C. R. (2013). Influence of postexercise cooling on muscle oxygenation and blood volume changes. Med. Sci. Sports Exerc 45, 876–882. doi:10.1249/MSS.0b013e31827e13a2
Ingram J., Dawson B., Goodman C., Wallman K., Beilby J. (2009). Effect of water immersion methods on post-exercise recovery from simulated team sport exercise. J. Sci. Med. sport 12, 417–421. doi:10.1016/j.jsams.2007.12.011
JúNIOR E. P., Brito C. J., Santos W. C., Valido C. N., Mendes E. L., Franchini E. (2014). Influence of cryotherapy on muscle damage markers in jiu-jitsu fighters after competition: A cross-over study. Rev. Andal. Med. del Deporte 7, 7–12. doi:10.1016/S1888-7546(14)70054-1
Leeder J., Gissane C., Van Someren K., Gregson W., Howatson G. (2012). Cold water immersion and recovery from strenuous exercise: A meta-analysis. Br. J. sports Med. 46, 233–240. doi:10.1136/bjsports-2011-090061
Machado A., Almeida A., Micheletti J., Vanderlei F., Tribst M., Netto Junior J., et al. (2017). Dosages of cold-water immersion post exercise on functional and clinical responses: A randomized controlled trial. Scand. J. Med. Sci. sports 27, 1356–1363. doi:10.1111/sms.12734
Machado A. F., Ferreira P. H., Micheletti J. K., De Almeida A. C., Lemes Í. R., Vanderlei F. M., et al. (2016). Can water temperature and immersion time influence the effect of cold water immersion on muscle soreness? A systematic review and meta-analysis. Sports Med. 46, 503–514. doi:10.1007/s40279-015-0431-7
Minett G. M., Duffield R., Billaut F., Cannon J., Portus M. R., Marino F. E. (2014). Cold-water immersion decreases cerebral oxygenation but improves recovery after intermittent-sprint exercise in the heat. Scand. J. Med. Sci. sports 24, 656–666. doi:10.1111/sms.12060
Moher D., Shamseer L., Clarke M., Ghersi D., Liberati A., Petticrew M., et al. (2015). Preferred reporting items for systematic review and meta-analysis protocols (PRISMA-P) 2015 statement. Syst. Rev. 4, 1–9. doi:10.1186/2046-4053-4-1
Nuhmani S., Kapoor G., Ahmad N., Agnihotri D. (2012). Effects of ice with active warmup and active warmup alone on performance in football player. Int. J. Biomed. Adv. Res. 3. doi:10.7439/ijbar.v3i11.778
Paddon-Jones D., Quigley B. (1997). Effect of cryotherapy on muscle soreness and strength following eccentric exercise. Int. J. sports Med. 18, 588–593. doi:10.1055/s-2007-972686
Parouty J., Al Haddad H., Quod M., Lepretre P. M., Ahmaidi S., Buchheit M. (2010). Effect of cold water immersion on 100-m sprint performance in well-trained swimmers. Eur. J. Appl. Physiology 109, 483–490. doi:10.1007/s00421-010-1381-2
Patterson S. M., Udermann B. E., Doberstein S. T., Reineke D. M. (2008). The effects of cold whirlpool on power, speed, agility, and range of motion. J. sports Sci. Med. 7, 387–394.
Peiffer J. J., Abbiss C. R., Watson G., Nosaka K., Laursen P. B. (2010a). Effect of cold water immersion on repeated 1-km cycling performance in the heat. J. Sci. Med. Sport 13, 112–116. doi:10.1016/j.jsams.2008.08.003
Pointon M., Duffield R., Cannon J., Marino F. E. (2012a). Cold water immersion recovery following intermittent-sprint exercise in the heat. Eur. J. Appl. physiology 112, 2483–2494. doi:10.1007/s00421-011-2218-3
Pointon M., Duffield R. (2012). Cold water immersion recovery after simulated collision sport exercise. Med. Sci. Sports Exerc. 44, 206–216. doi:10.1249/MSS.0b013e31822b0977
Poppendieck W., Faude O., Wegmann M., Meyer T. (2013). Cooling and performance recovery of trained athletes: A meta-analytical review. Int. J. sports physiology Perform. 8, 227–242. doi:10.1123/ijspp.8.3.227
Roberts L. A., Nosaka K., Coombes J. S., Peake J. M. (2014). Cold water immersion enhances recovery of submaximal muscle function after resistance exercise. Am. J. Physiology-Regulatory, Integr. Comp. Physiology 307, R998–R1008. doi:10.1152/ajpregu.00180.2014
Rowsell G. J., Coutts A. J., Reaburn P., Hill-Haas S. (2009). Effects of cold-water immersion on physical performance between successive matches in high-performance junior male soccer players. J. sports Sci. 27, 565–573. doi:10.1080/02640410802603855
Rupp K. A., Selkow N. M., Parente W. R., Ingersoll C. D., Weltman A. L., Saliba S. A. (2012). The effect of cold water immersion on 48-hour performance testing in collegiate soccer players. J. Strength Cond. Res. 26, 2043–2050. doi:10.1519/JSC.0b013e318239c3a1
Sellwood K. L., Brukner P., Williams D., Nicol A., Hinman R. (2007). Ice-water immersion and delayed-onset muscle soreness: A randomised controlled trial. Br. J. sports Med. 41, 392–397. doi:10.1136/bjsm.2006.033985
Siqueira A. F., Vieira A., Bottaro M., Ferreira-JúNIOR J. B., NóBREGA O. D. T., De Souza V. C., et al. (2018). Multiple cold-water immersions attenuate muscle damage but not alter systemic inflammation and muscle function recovery: A parallel randomized controlled trial. Sci. Rep. 8, 10961. doi:10.1038/s41598-018-28942-5
Spencer M., Rechichi C., Lawrence S., Dawson B., Bishop D., Goodman C. (2005). Time-motion analysis of elite field hockey during several games in succession: A tournament scenario. J. Sci. Med. Sport 8, 382–391. doi:10.1016/s1440-2440(05)80053-2
Takeda M., Sato T., Hasegawa T., Shintaku H., Kato H., Yamaguchi Y., et al. (2014). The effects of cold water immersion after rugby training on muscle power and biochemical markers. J. sports Sci. Med. 13, 616–623.
Vaile J., O'Hagan C., Stefanovic B., Walker M., Gill N., Askew C. D. (2011). Effect of cold water immersion on repeated cycling performance and limb blood flow. Br. J. sports Med. 45, 825–829. doi:10.1136/bjsm.2009.067272
White G. E., Rhind S. G., Wells G. D. (2014). The effect of various cold-water immersion protocols on exercise-induced inflammatory response and functional recovery from high-intensity sprint exercise. Eur. J. Appl. physiology 114, 2353–2367. doi:10.1007/s00421-014-2954-2
Wiewelhove T., Schneider C., DöWELING A., Hanakam F., Rasche C., Meyer T., et al. (2018). Effects of different recovery strategies following a half-marathon on fatigue markers in recreational runners. PLoS One 13, e0207313. doi:10.1371/journal.pone.0207313
Wilcock I. M., Cronin J. B., Hing W. A. (2006). Physiological response to water immersion. Sports Med. 36, 747–765. doi:10.2165/00007256-200636090-00003
Keywords: delayed-onset muscle soreness, cold water immersion, exercise fatigue, exercise-induced soreness, fatigue recovery measures
Citation: Xiao F, Kabachkova AV, Jiao L, Zhao H and Kapilevich LV (2023) Effects of cold water immersion after exercise on fatigue recovery and exercise performance--meta analysis. Front. Physiol. 14:1006512. doi: 10.3389/fphys.2023.1006512
Received: 29 July 2022; Accepted: 02 January 2023;
Published: 20 January 2023.
Edited by:
Antonella Muscella, University of Salento, ItalyReviewed by:
Ersan Arslan, Faculty of Sport Sciences, Tokat Gaziosmanpasa University, TürkiyeAttilio Parisi, Foro Italico University of Rome, Italy
Jozef Simenko, University of Hertfordshire, United Kingdom
Copyright © 2023 Xiao, Kabachkova, Jiao, Zhao and Kapilevich. This is an open-access article distributed under the terms of the Creative Commons Attribution License (CC BY). The use, distribution or reproduction in other forums is permitted, provided the original author(s) and the copyright owner(s) are credited and that the original publication in this journal is cited, in accordance with accepted academic practice. No use, distribution or reproduction is permitted which does not comply with these terms.
*Correspondence: Feiyan Xiao, xiaofeiyan1223@gmail.com