- 1Department of Internal Medicine, Hualien Armed Forces General Hospital, Hualien City, Taiwan
- 2Department of Medicine, Tri-Service General Hospital, National Defense Medical Center, Taipei, Taiwan
- 3Department of Stomatology of Periodontology, Mackay Memorial Hospital, Taipei, Taiwan
- 4Department of Exercise Science, Arnold School of Public Health, University of South Carolina, Columbia, SC, United States
- 5Ochsner Clinical School, John Ochsner Heart and Vascular Institute, The University of Queensland School of Medicine, New Orleans, LA, United States
Background: Both cardiopulmonary exercise testing (CPET) and run field tests are recommended by the American Heart Association for assessing the maximal oxygen uptake (VO2 max) of youth. Power output was highly correlated with VO2 max in CPET. However, it is unclear regarding the correlations of time and estimated power output (EPO) for a run field test with VO2 max obtained from CPET in young adults.
Methods: This study included 45 participants, aged 20–40 years, from a sample of 1,120 military personnel who completed a 3,000-m run field test in Taiwan in 2020. The participants subsequently received CPET using the Bruce protocol to assess VO2 max in the same year. According to the physics rule, EPO (watts) for the run field test was defined as the product of half body mass (kg) and [distance (3000-m)/time (s) for a run field test]. Pearson product–moment correlation analyses were performed.
Results: The Pearson correlation coefficient (r) of time against EPO for the run field test was estimated to be 0.708 (p <0.001). The correlation coefficient between the time for the run field test and VO2 max (L/min) in CPET was estimated to be 0.462 (p = 0.001). In contrast, the correlation coefficient between time for the run field test and VO2 max scaled to body mass in CPET was estimated to be 0.729 (p <0.001). The correlation coefficient of EPO for the run field test against VO2 max in CPET was estimated to be 0.813 (p <0.001).
Conclusion: In young adults, although the time for a run field test was a reliable estimate of VO2 max scaled to body mass, EPO proportional to the mean square velocity was found as a superior estimate of VO2 max.
Introduction
Cardiorespiratory fitness (CRF) is inversely associated with the risk of metabolic syndrome, diabetes mellitus, cardiovascular diseases (CVD), and mortality in the general population (Carnethon et al., 2009; Mehta et al., 2020; Wang et al., 2021; Lin et al., 2022; Sui et al., 2022). Obtaining greater CRF levels for sedentary individuals has been proposed as one of the major preventive measures to reduce the severity and burden of atherosclerosis in developed countries (Lavie et al., 2019; Sanchis-Gomar et al., 2022). The gold standard for CRF assessment is maximal oxygen uptake (VO2 max) with or without an adjustment for body mass from cardiopulmonary exercise testing (CPET). The main advantages of CPET include a strictly controlled environment, e.g., a maintained indoor temperature, atmospheric pressure, and calibration gas. However, the limitation to CPET is facility-dependent, and examinees require a tolerance to wear a mask during the graded exercise testing. Therefore, CPET may not be feasible for some specific populations, such as children and the elderly, and might not be practical at a large population level.
Based on the findings of previous studies, the American Heart Association (AHA) has recommended VO2 max and some alternative measures for the CRF levels of youth (Mayorga-Vega et al., 2015; Raghuveer et al., 2020). For instance, the performance of a field-based 20-m shuttle run test or distance run test has a moderate-to-high correlation with VO2 max obtained from CPET, whereas the performance of a field-based step test or 6-minute walk test has only a low-to-moderate correlation with VO2 max in children or adolescents (Raghuveer et al., 2020).
In addition, previous studies also revealed that the peak power output of the heart or body measured in CPET has a high correlation with VO2 max in patients with recovering heart failure and athletes (Hawley and Noakes, 1992; Jakovljevic et al., 2011). Physiologically, most oxygen uptake is translated to energy output during peak exercise. However, to the best of our knowledge, there have been no studies with regard to the correlation between estimated power output (EPO) for a run field test and VO2 max in CPET. The correlation between a run test performance and VO2 max in CPET was not clarified in adults and varied by study (Cooper, 1968; O’Gorman et al., 2000; Casajus and Castagna, 2007). The aim of this study was to investigate the correlations of time and EPO for a distance run field test with VO2 max measured from CPET in a group of young military adults.
Materials and methods
Study population
The study included 45 participants, aged 20–40 years, without any medication use, randomly selected from the cardiorespiratory fitness and health in eastern armed forces (CHIEF) study participants (N = 1,120) (Lin et al., 2021; Liu et al., 2021) in 2020, which aimed to carry out a preliminary study on the correlation of time for a 3000-m run field test with VO2 max from CPET. All participants received exercise training daily, e.g., a 3,000-m run within 25 min in the morning at the military base for over 6 months. A history of moderate physical activity, such as a limited-time 3,000-m run in the morning per week in the past half year, was obtained from each participant. Each participant underwent the 2020 annual health examinations for physical examinations (Hsu et al., 2022) in the Hualien Armed Forces General Hospital of Taiwan. Each participant also underwent the 2020 annual military exercise test for a 3,000-m run field test to assess endurance capacity. Within 2 weeks of the 3,000-run field test, the 45 volunteers were scheduled for CPET to objectively assess VO2 max.
Anthropometric and blood pressure (BP) measurements
Anthropometric parameters, i.e., body height and weight, were measured in a standing position by a medical staff member in the annual military exercise test. Body mass index was defined as the body weight divided by body height squared (kg/m2). Overweight or obesity was defined as a body mass index ≥27.5 kg/2 for Asians, according to the recommendations of the World Health Organization (Jih et al., 2014). The BP and pulse rate of each participant were automatically measured using the same device (FT201, Parama-Tech Co., Ltd., Fukuoka, Japan), which utilized the oscillometric method (Lin et al., 2016; Lin et al., 2020a; Lin et al., 2020b). The BP was measured once over the right arm in a sitting position after resting for longer than 15 min and was recorded by a medical staff member. If the pulse rate was ≥90 beats per minute, systolic BP ≥140 mmHg, or diastolic BP ≥90 mmHg was found, the participant would undergo another two rounds of hemodynamic parameter measurements, which were averaged as the final result.
The 3000-m run field test
The 3,000-m run field test was performed outdoors on a flat playground of the Military Physical Training and Testing Center in Hualien, Taiwan, at 16:00. Each participant wore sweat suits and did not carry any additional objects. The whole running process of each participant was video recorded and supervised by eight military sports officers. The 3,000-m run field test was carried out if there was no heavy rain, and the coefficient of the heat stroke risk formula, the product of relative humidity (%) and outdoor temperature (Celsius scale) x 0.1, was less than 40. The time for the 3,000-m run field test was utilized to evaluate the endurance capacity of each participant. EPO for the run field test was defined as “1/2 x body mass (kg) x square of mean velocity (m/s),” on the basis of the physics rule of the kinetic energy theorem (Serway and Jewett, 2004). The mean velocity was calculated by the formula “distance (3,000-m) divided by time (s) for the run field test.”
The performance of CPET
CPET was performed on a Trackmaster TMX-428 stress treadmill (SCHILLER, Baar, Switzerland) using the standard Bruce protocol. The same supervisor conducted all of the CPETs throughout the study. All participants were asked not to consume caffeine or alcohol for 12 h or longer before the CPET and exercised after a 2-h postprandial period. The room for the CPET used an air conditioning system to maintain a constant temperature of approximately 22 degrees Celsius. Throughout the CPET, electrocardiography and BP were monitored. The rates of oxygen uptake (VO2), production of carbon dioxide (VCO2), tidal volume (Vt), end-tidal partial pressure of carbon dioxide (PETCO2), and respiratory rate were recorded breath by breath using a Cardiovit CS-200 Excellence Ergo-Spiro analytic system (SCHILLER, Baar, Switzerland). VO2 max was defined as the average of VO2 during the last minute of maximal exercise.
Statistical analysis
Characteristics of overall participants for a 3,000-m run field test and those for both a 3,000-m run field test and CPET were presented as numbers (%) for categorical variables and mean ± standard deviation for continuous variables, respectively. Continuous variables were compared using analysis of variance if the Kolmogorov–Smirnov test for the normal distribution was met; otherwise, the Wilcoxon signed-rank test was used. Categorical variables were compared using Fisher’s exact test. The Pearson product–moment correlation coefficient was used to determine the association strength of time and EPO for a 3,000-m run field test with VO2 max scaled to body mass or not in CPET. The correlations of time and EPO for a 3,000-m run field test were performed for a comparison between selected participants for both a 3,000-m run field test and CPET and a sample of age-, sex-, body mass index-, and BP-matched participants from the overall study participants. Scatter plots between time and EPO for the 3,000-m run field test and VO2 max scaled for body mass or not in CPET were obtained. Internal validation was performed for those whose body mass index <27.5 kg/m2. A value of p <0.05 was considered significant. All analyses were carried out using SPSS version 25.0 for Windows (IBM Corp., Armonk, NY, United States). This study has been approved by the Institutional Review Board of the Clinical Ethics Committee of the Mennonite Christian Hospital (No. 16-05-008), Hualien City, Eastern Taiwan, R.O.C., and written informed consent was obtained from all participants.
Results
Clinical characteristics of the participants
Table 1 shows the clinical characteristics of the participants for a 3,000-m run field test (N = 1,120) and those for both a 3,000-m run field test and CPET (N = 45). The characteristics of the selected participants for CPET were similar to the original overall sample, except greater age, pulse rate, and BP levels were observed in participants for CPET. The mean age of the participants for CPET was approximately 30 years old, and over 90% of the participants were males. Since only one woman was included for the CPET, the characteristics of men were also compared between the original group (N = 911) and the selected group (N = 44), and the results are provided in Supplementary Table S1, which show consistent findings.
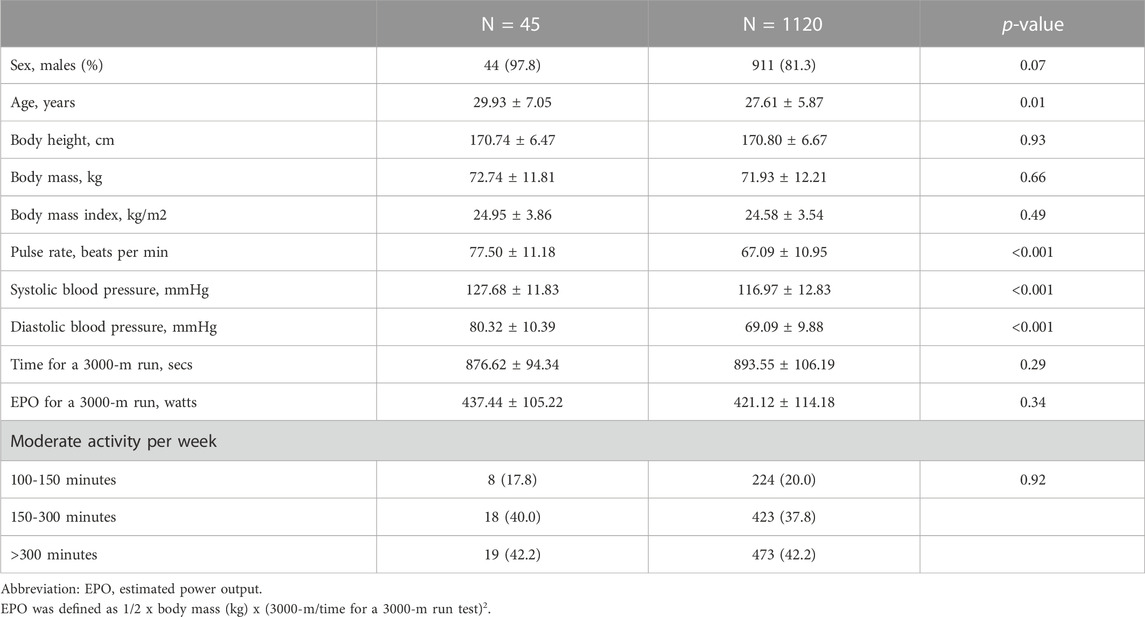
TABLE 1. Clinical Characteristics of the Overall Participants for a Run Field Test and the Selected Participants for a Cardiopulmonary Exercise Testing.
Correlations between time and EPO for a 3000-m run field test
The correlation coefficient (r) of time against EPO for a 3,000-m run field test was estimated to be 0.708 (p <0.001) in participants for both a 3,000-m run field test and CPET (Figure 1A), which was close to the correlation coefficient (r = 0.703 and p <0.001) in the age-, sex-, body mass index-, and BP-matched samples of 707 participants for a 3000-m run test (Figure 1B). The characteristics of the variable-matched population are provided in Supplementary Table S2. The correlation coefficients for men only were in line with the main findings, and the results are provided in Supplementary Figure S1.
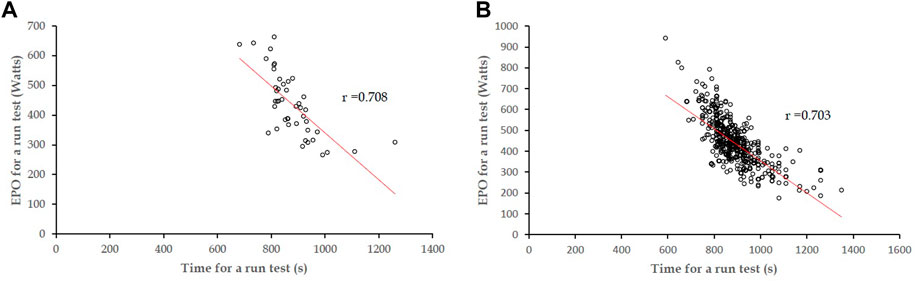
FIGURE 1. (A) Correlation coefficient (r) of time against EPO for a 3,000-m run field test, estimated to be 0.708 (p <0.001) in participants for both a 3,000-m run field test and CPET. (B) Correlation coefficient estimated to be 0.703 (p <0.001) in the variable-matched participants for a 3,000-m run field test.
Correlations of time for a 3000-m run field test against VO2 max in CPET
The correlation coefficient of time for a 3000-m run field test against VO2 max (L/min) in CPET was estimated to be 0.462 (p = 0.001) (Figure 2A). In contrast, the correlation coefficient between time for a 3000-m run field test and VO2 max scaled to body mass (kg) in CPET was estimated to be 0.729 (p <0.001) (Figure 2B). The correlation coefficients for men only were in line with the main findings, and the results are provided in Supplementary Figure S2.
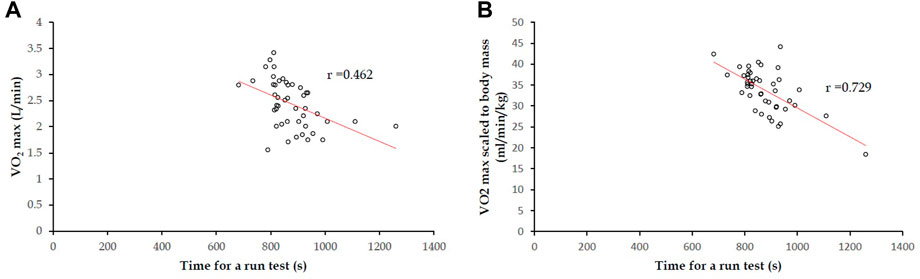
FIGURE 2. In 45 participants, for both a 3,000-m run field test and CPET: (A) correlation coefficient of time for a 3,000-m run field test against VO2 max (L/min) was 0.462 (p = 0.001); (B) correlation coefficient of time for a 3,000-m run field test with VO2 max scaled to body mass (kg) was 0.729 (p <0.001).
Correlations of EPO for a 3000-m run field test against VO2 max in CPET
The correlation coefficient of EPO for a 3,000-m run field test against VO2 max (L/min) in CPET was estimated to be 0.813 (p <0.001) (Figure 3A). However, the correlation coefficient between EPO for a 3,000-m run field test and VO2 max scaled to body mass (kg) in CPET was estimated to be only 0.364 (p <0.001) (Figure 3B). The correlation coefficients for men only were in line with the main findings, and the results are provided in Supplementary Figure S3.
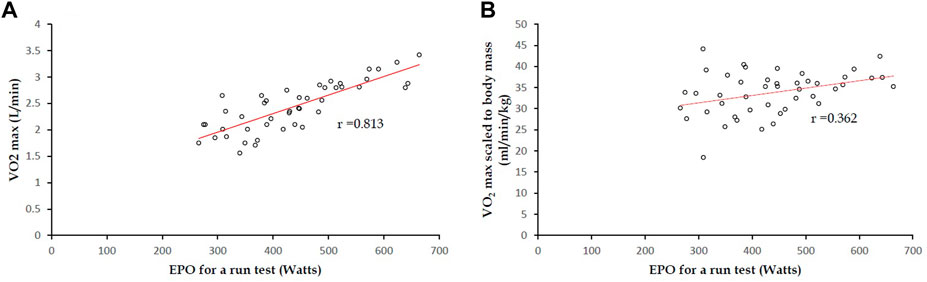
FIGURE 3. In 45 participants, for both a 3,000-m run field test and CPET: (A) correlation coefficient of EPO for a 3000-m run field test against VO2 max (L/min) was 0.813 (p <0.001); (B) correlation coefficient between EPO for a 3,000-m run field test and VO2 max scaled to body mass (kg) was estimated to be 0.364 (p <0.001).
Internal validation for non-obese participants
The results of interval validation for participants with body mass index <27.5 kg/m2 (N = 35) are revealed in Figure 4. The correlation coefficient of time for a 3,000-m run field test against VO2 max (L/min) was 0.453 (p = 0.006) (Figure 4A), and the correlation coefficient of time for a 3,000-m run field test with VO2 max scaled to body mass (kg) was 0.485 (p = 0.003) (Figure 4B). The correlation coefficient of EPO for a 3,000-m run field test with VO2 max (L/min) was 0.757 (p <0.001) (Figure 4C), and the correlation coefficient of EPO for a 3,000-m run field test against VO2 max scaled to body mass (kg) was 0.349 (p = 0.04) (Figure 4D). Although the correlation coefficients in the sample for interval validation were all lower than that in the overall sample (N = 45) receiving the CPET, all of the associations of time and EPO for a 3,000-m run with VO2 max in a CPET were significant, and the EPO association remained with the greatest strength.
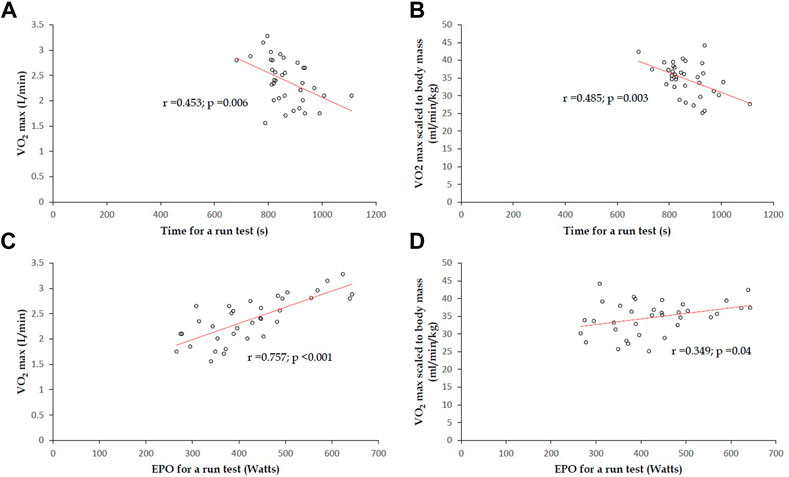
FIGURE 4. In 35 participants with body mass index <27.5 kg/m2, for both a 3000-m run field test and CPET: (A) correlation coefficient of time for a 3000-m run field test against VO2 max (L/min) was estimated to be 0.453 (p = 0.006); (B) correlation coefficient of time for a 3,000-m run field test against VO2 max scaled to body mass (kg) was estimated to be 0.485 (p = 0.003); (C) correlation coefficient of EPO for a 3,000-m run field test with VO2 max (L/min) was 0.757 (p <0.001). (D) correlation coefficient between EPO for a 3,000-m run field test and VO2 max (L/min) scaled to body mass was 0.349 (p = 0.04).
Estimation of VO2 max in CPET from time and EPO for a run field test
Based on the regression line in Figure 2B, formula 1, with regard to time for a run field test to estimate VO2 max scaled to body mass, can be derived as follows:
Formula 1
Y = −0.0386X + 67.151
X: Time for a 3,000-m field run (s)
Y: VO2 max scaled to body mass (mL/min/kg)
Based on the regression line in Figure 3A, formula 2, with regard to EPO for a run field test to estimate VO2 max, can be derived as follows:
Formula 2
Y = 3.7943X + 757.6
X: EPO for a 3000-m field run (watts)
Y: VO2 max (mL/min)
Discussion
The principal finding of this study was that in young adults, the time for a run field test can be an acceptable estimate of VO2 max scaled to body mass obtained in CPET. In addition, the EPO for a run field test, calculated according to the kinetic energy theorem, can be a more precise estimate of VO2 max (L/min) than the time for a run field test.
Numerous studies have investigated the correlation between distance- or time-based run field test performance and VO2 max in CPET in adults (Mayorga-Vega et al., 2016). However, the correlation coefficients were distributed widely, ranging from 0.60 to 0.90, among various run field tests (Cooper, 1968). In addition, no consensus has been reached in previous studies to unify the VO2 max unit, with or without an adjustment for body mass, when analyzing the correlation. Furthermore, the correlation coefficients might vary even in the same run field test among different studies (Cooper, 1968; O’Gorman et al., 2000; Casajus and Castagna, 2007). For instance, Cooper found a high correlation between running distance and VO2 max scaled to body mass in a 12-min run field test in a sample of military males, in whom the correlation coefficient was estimated to be 0.897 (Cooper, 1968). In the O’Gorman et al. (2000) study, there was a moderate correlation in a 12-min run field test and in a 3,000-m run field test using a sample of physically fit young males, in whom the correlation coefficient was estimated equally to be 0.67 and −0.67. In contrast, the Casajus and Castagna study revealed a relatively lower correlation in a 12-min run field test in a sample of elite soccer players, in whom the correlation coefficient was estimated to be only 0.46 (Casajus and Castagna, 2007). In the present study, we demonstrated a moderate correlation of time for a 3,000-m run field test with VO2 max scaled to body mass, whereas the results showed a relatively lower correlation with VO2 max, which was not scaled to body mass. These findings are in line with previous studies and a meta-analysis (Serway and Jewett, 2004) made by Mayorga-Vega et al., which showed the correlation coefficient between time for a 3,000-m run field test and VO2 max scaled to a body mass of 12 studies, including 951 young adults, was estimated to be 0.70. We further highlighted the importance of the VO2 max unit for examining the correlation. It is reasonable that the examinees’ running velocity in run field tests was inversely related to their body mass. The correlation between the running velocity in a run field test and VO2 max scaled to body mass in CPET would theoretically result in the optimal result, except that the examinees had a similar level of body mass at baseline.
Some reports have shown a moderate-to-high correlation between peak cardiac power output (Watts) and VO2 max (L/min) not scaled to body mass in CPET in patients with heart failure, in whom the greatest correlation coefficient was 0.85, observed in those with recovering heart function (Jakovljevic et al., 2011), and a high correlation of peak power output with VO2 max in cycling athletes (correlation coefficient greater than 0.90) (Hawley and Noakes, 1992). The present study is the first report using EPO for a run field test to estimate VO2 max in CPET in young adults. Oxygen consumption generates energy, heat, and waste. Accordingly, it is apt to use peak exercise power output to assess VO2 max not adjusted for body mass in CPET for adults. In the present study, EPO was calculated according to the kinetic energy theorem and proportional to the square of the mean velocity in a 3,000-m run field test. The use of the square of the mean velocity was better than the mean velocity in the run field test to correlate with VO2 max in CPET in adults. This finding is consistent with a previous study on CPET, where peak power output was superior to the cycling speed to estimate VO2 max in athletes (Hawley and Noakes, 1992).
The present study, however, has a few limitations. First, the limited number of enrolled subjects is the major limitation to this preliminary study, and further study is required to expand the sample size to obtain greater power. Second, this study included only one woman and lacked multi-ethnic/racial diversity, making generalization of the results difficult. Third, although the annual military exercise test was held with some restrictions of the weather, there could have been a bias for differences in outdoor temperature and humidity, which may affect the running performance and EPO estimation. Fourth, as the study included merely healthy subjects, the results may not be appropriately applied to those with a mismatch between heart and lung functions. External validation should be performed in further study. Finally, since we chose mean running velocity in the kinetic formula, the maximum actual power output during the run test might be underestimated, possibly leading to a bias for the correlation with VO2 max.
Conclusion
There have been no recommendations from the AHA regarding the role of time and EPO for a run field test to evaluate VO2 max in adults, and the VO2 max unit was not emphasized. Our findings suggest that in young adults, although the time for a distance run field test was an acceptable estimate of VO2 max scaled to body mass, EPO proportional to the square of the mean velocity in a run field test was found as a superior estimate of VO2 max than the time for a run field test in this population. Further studies are needed involving young women.
Data availability statement
The raw data supporting the conclusions of this article will be made available by the authors, without undue reservation.
Ethics statement
The studies involving human participants were reviewed and approved by the Institutional Review Board of the Clinical Ethics Committee of the Mennonite Christian Hospital (No 16-05-008) Hualien City, Eastern Taiwan. The patients/participants provided their written informed consent to participate in this study.
Author contributions
GL collected and interpreted the data and wrote the manuscript; KT analyzed the data; XS and CL raised critical comments and edited the text; GL was the principal investigator for the administration of the study.
Funding
The present study was supported by grants from the Hualien Armed Forces General Hospital (HAFGH-D-112004) and the Medical Affairs Bureau, Ministry of National Defense (MND-MAB-D-112182).
Conflict of interest
The authors declare that the research was conducted in the absence of any commercial or financial relationships that could be construed as a potential conflict of interest.
Publisher’s note
All claims expressed in this article are solely those of the authors and do not necessarily represent those of their affiliated organizations, or those of the publisher, the editors, and the reviewers. Any product that may be evaluated in this article, or claim that may be made by its manufacturer, is not guaranteed or endorsed by the publisher.
Supplementary material
The Supplementary Material for this article can be found online at: https://www.frontiersin.org/articles/10.3389/fphys.2023.1110802/full#supplementary-material
References
Carnethon M. R., Sternfeld B., Schreiner P. J., Jacobs D. R., Lewis C. E., Liu K., et al. (2009). Association of 20-year changes in cardiorespiratory fitness with incident type 2 diabetes: The coronary artery risk development in young adults (CARDIA) fitness study. Diabetes Care 32 (7), 1284–1288. doi:10.2337/dc08-1971
Casajus J. A., Castagna C. (2007). Aerobic fitness and field test performance in elite Spanish soccer referees of different ages. J. Sci. Med. Sport 10 (6), 382–389. doi:10.1016/j.jsams.2006.08.004
Cooper K. H. (1968). A means of assessing maximal oxygen intake. Correlation between field and treadmill testing. JAMA 203 (3), 201–204. doi:10.1001/jama.1968.03140030033008
Hawley J. A., Noakes T. D. (1992). Peak power output predicts maximal oxygen uptake and performance time in trained cyclists. Eur. J. Appl. Physiol. Occup. Physiol. 65 (1), 79–83. doi:10.1007/BF01466278
Hsu C. Y., Liu P. Y., Liu S. H., Kwon Y., Lavie C. J., Lin G. M. (2022). Machine learning for electrocardiographic features to identify left atrial enlargement in young adults: CHIEF heart study. Front. Cardiovasc Med. 9, 840585. doi:10.3389/fcvm.2022.840585
Jakovljevic D. G., Birks E. J., George R. S., Trenell M. I., Seferovic P. M., Yacoub M. H., et al. (2011). Relationship between peak cardiac pumping capability and selected exercise-derived prognostic indicators in patients treated with left ventricular assist devices. Eur. J. Heart Fail 13 (9), 992–999. doi:10.1093/eurjhf/hfr069
Jih J., Mukherjea A., Vittinghoff E., Nguyen T. T., Tsoh J. Y., Fukuoka Y., et al. (2014). Using appropriate body mass index cut points for overweight and obesity among Asian Americans. Prev. Med. 65, 1–6. doi:10.1016/j.ypmed.2014.04.010
Lavie C. J., Ozemek C., Carbone S., Katzmarzyk P. T., Blair S. N. (2019). Sedentary behavior, exercise, and cardiovascular health. Circ. Res. 124 (5), 799–815. doi:10.1161/CIRCRESAHA.118.312669
Lin G. M., Li Y. H., Lee C. J., Shiang J. C., Lin K. H., Chen K. W., et al. (2016). Rationale and design of the cardiorespiratory fitness and hospitalization events in armed forces study in Eastern Taiwan. World J. Cardiol. 8 (8), 464–471. doi:10.4330/wjc.v8.i8.464
Lin G. M., Liu P. Y., Tsai K. Z., Lin Y. K., Huang W. C., Lavie C. J. (2022). Cardiorespiratory fitness and carotid intima-media thickness in physically active young adults: CHIEF atherosclerosis study. J. Clin. Med. 11 (13), 3653. doi:10.3390/jcm11133653
Lin G. M., Tsai K. Z., Lin C. S., Han C. L. (2020). Physical fitness and long-term blood pressure variability in young male military personnel. Curr. Hypertens. Rev. 16 (2), 156–160. doi:10.2174/1573402115666191023111351
Lin Y. K., Tsai K. Z., Han C. L., Lin Y. P., Lee J. T., Lin G. M. (2021). Obesity phenotypes and electrocardiographic characteristics in physically active males: CHIEF study. Front. Cardiovasc Med. 8, 738575. doi:10.3389/fcvm.2021.738575
Lin Y. P., Fan C. H., Tsai K. Z., Lin K. H., Han C. L., Lin G. M. (2020). Psychological stress and long-term blood pressure variability of military young males: The cardiorespiratory fitness and hospitalization events in armed forces study. World J. Cardiol. 12 (12), 626–633. doi:10.4330/wjc.v12.i12.626
Liu P. Y., Tsai K. Z., Lima J. A. C., Lavie C. J., Lin G. M. (2021). Athlete's heart in asian military males: The CHIEF heart study. Front. Cardiovasc Med. 8, 725852. doi:10.3389/fcvm.2021.725852
Mayorga-Vega D., Aguilar-Soto P., Viciana J. (2015). Criterion-related validity of the 20-M shuttle run test for estimating cardiorespiratory fitness: A meta-analysis. J. Sports Sci. Med. 14 (3), 536–547.
Mayorga-Vega D., Bocanegra-Parrilla R., Ornelas M., Viciana J. (2016). Criterion-related validity of the distance- and time-based walk/run field tests for estimating cardiorespiratory fitness: A systematic review and meta-analysis. PLoS One 11, e0151671. doi:10.1371/journal.pone.0151671
Mehta A., Kondamudi N., Laukkanen J. A., Wisloff U., Franklin B. A., Arena R., et al. (2020). Running away from cardiovascular disease at the right speed: The impact of aerobic physical activity and cardiorespiratory fitness on cardiovascular disease risk and associated subclinical phenotypes. Prog. Cardiovasc Dis. 63 (6), 762–774. doi:10.1016/j.pcad.2020.11.004
O’Gorman D., Hunter A., MacDonnacha C., Kirwan J. P. (2000). Validity of field tests for evaluating endurance capacity in competitive and international-level sports participants. J. Strength Cond. Res. 14 (1), 62–67. doi:10.1519/00124278-200002000-00011
Raghuveer G., Hartz J., Lubans D. R., Takken T., Wiltz J. L., Mietus-Snyder M., et al. (2020). Cardiorespiratory fitness in youth: An important marker of health: A scientific statement from the American heart association. Circulation 142 (7), e101–e118. doi:10.1161/CIR.0000000000000866
Sanchis-Gomar F., Lavie C. J., Marín J., Perez-Quilis C., Eijsvogels T. M. H., O'Keefe J. H., et al. (2022). Exercise effects on cardiovascular disease: From basic aspects to clinical evidence. Cardiovasc Res. 118 (10), 2253–2266. doi:10.1093/cvr/cvab272
Serway R., Jewett J. W. (2004). Physics for scientists and engineers, 6th edition Books/Cole. 0-534-40842-7.
Sui X., Sarzynski M. A., Gribben N., Zhang J., Lavie C. J. (2022). Cardiorespiratory fitness and the risk of all-cause, cardiovascular and cancer mortality in men with hypercholesterolemia. J. Clin. Med. 11 (17), 5211. doi:10.3390/jcm11175211
Keywords: cardiopulmonary exercise testing, maximal oxygen uptake, estimated power output, run field test, velocity
Citation: Lin G-M, Tsai K-Z, Sui X and Lavie CJ (2023) Estimated power output for a distance run and maximal oxygen uptake in young adults. Front. Physiol. 14:1110802. doi: 10.3389/fphys.2023.1110802
Received: 05 December 2022; Accepted: 20 January 2023;
Published: 07 February 2023.
Edited by:
Elisabetta Salvioni, Monzino Cardiology Center (IRCCS), ItalyReviewed by:
Maurizio Bussotti, Scientific Clinical Institute Maugeri (ICS Maugeri), ItalyYaoshan Dun, Xiangya Hospital, Central South University, China
Copyright © 2023 Lin, Tsai, Sui and Lavie. This is an open-access article distributed under the terms of the Creative Commons Attribution License (CC BY). The use, distribution or reproduction in other forums is permitted, provided the original author(s) and the copyright owner(s) are credited and that the original publication in this journal is cited, in accordance with accepted academic practice. No use, distribution or reproduction is permitted which does not comply with these terms.
*Correspondence: Gen-Min Lin, farmer507@yahoo.com.tw; Carl J. Lavie, clavie@ochsner.org