- 1Innovation Design Office, Riken, Kobe, Japan
- 2Institute of Exact Sciences, Federal University of Alfenas, Alfenas, Brazil
- 3Laboratory of Physiological Sciences, Department of Structural Biology, Institute of Biological Sciences, University of Brasilia, Brasilia, Brazil
- 4Postgraduate Program in Environment, University CEUMA, São Luís, Brazil
- 5Laboratory of Biomathematics, Institute of Biomedical Sciences, Federal University of Alfenas, Alfenas, Brazil
Introduction: Bearded capuchins display a wide variety of manipulatory skills and make routine use of tools in both captivity and the wild. The efficient handling of objects in this genus has led several investigators to assume near-human thumb movements, despite a lack of anatomical studies.
Methods: Here, we performed an anatomical analysis of muscles and bones in the capuchin hand. Sapajus morphological traits were quantitatively compared with those of humans, chimpanzees, gorillas, and baboons.
Results: The comparative analysis indicated that the Sapajus hand is more similar to that of baboons and least similar to that of humans according to the muscles, bones, and three-dimensional data. Furthermore, these findings suggest that bearded capuchins lack true thumb opponency. Regarding manipulatory skills, they display rather primitive hand traits, with limited resources for precision grasping using the opponens pollicis.
Discussion: These findings suggest that bearded capuchins’ complex use of tools depends more heavily on their high cognitive abilities than on a versatile hand apparatus. These findings offer crucial insights into the evolution of primate cognition.
1 Introduction
Despite being a New World primate, bearded capuchins (Sapajus sp.) exhibit several evolutionary convergences with great apes and humans, including a long lifespan (Hakeem et al., 1996; Judge and Carey, 2000), a well-developed neocortex (Rilling and Insel, 1999), high degrees of gregariousness and social tolerance (Fragazy et al., 2004), and tool use in both captivity and the wild (Canale et al., 2009; Mannu and Ottoni, 2009; Spagnoletti et al., 2009; Souto et al., 2011). Nevertheless, in other anatomical characteristics, such as anatomical patterns of vessels, nerves, and muscles of the thoracic and pelvic limbs, capuchins are more similar to baboons (Aversi-Ferreira et al., 2013; Aversi-Ferreira et al., 2014c; Aversi-Ferreira et al., 2020; Aversi-Ferreira et al., 2021a; Abreu et al., 2021) and New World primates (Aversi-Ferreira et al., 2010).
A detailed study demonstrated that despite performing precision grip and high manual abilities, bearded capuchins present a primitive anatomical structure in their hands from the forearm muscles, as opposed to those found in great apes and humans (i.e., bearded capuchins do not realize true thumb opponency); therefore, they putatively use their large brain to generate strategies to contour over their primitive apparatus (Aversi-Ferreira et al., 2014a; Abreu et al., 2021). Indeed, the use of complex tools requires high motor and cognitive skills as well as an appropriate hand apparatus, in support of which Sapajus presents high indices of encephalization (Fragazy et al., 2004; Pereira-de-Paula et al., 2010; Borges et al., 2015; Aversi-Ferreira et al., 2021a; Abreu et al., 2021) and abundant corticospinal motor connections (Stephan and Andy, 1969; Rilling and Insel, 1999; Abreu et al., 2021) to support the planning and coordination involved in tool use.
Behavioral accounts of capuchins’ hand abilities have been asserted despite the lack of complete and/or detailed anatomical analyses of their hand and forearm structures. One exception to this was a study concerning bearded capuchins’ hand joints, bones, and thumb muscles (Aversi-Ferreira et al., 2014a). It demonstrated various strategies for the precision grip and lateral pinch of Sapajus and fine movements found in certain human performance, such as holding a pen or pencil to write. Moreover, studies on bearded capuchins’ forearms (Aversi-Ferreira et al., 2010; Aversi-Ferreira et al., 2011) have demonstrated the presence of a separated tendon of the extensor pollicis longus and extensor indicis in Sapajus, which until then had only been observed in modern humans (Aversi-Ferreira et al., 2010).
The aforementioned particularities of bearded capuchins concern similarities with great apes in cognitive and behavioral aspects as well as in certain muscles; on the other hand, morphological similarities exist with baboons and New World primates. Therefore, we performed dissections on bearded capuchin hands, describing the muscles involved in hand movements, as well as their origins, insertions, innervation, supply, and number. These data were compared with the anatomical descriptions of modern humans (Swindler and Wood, 1973; Gibbs, 1999; Standring, 2008), chimpanzees (Swindler and Wood, 1973; Gibbs, 1999), gorillas (Swindler and Wood, 1973; Gibbs, 1999), and baboons (Swindler and Wood, 1973), and the results were associated with behavioral and tool use aspects. All descriptions were quantified and compared using the statistical method for a comparative anatomy (Aversi-Ferreira, 2009; Aversi-Ferreira et al., 2011; Aversi-Ferreira et al., 2014a; Aversi-Ferreira et al., 2014b; Aversi-Ferreira et al., 2015; Aversi-Ferreira et al., 2016).
2 Materials and methods
2.1 Samples
Eight adult Sapajus specimens were used (six males and two females), weighing 1–3 kg. No animals were killed for the purpose of this study; four of them suffered accidental deaths in their natural habitats and were acquired from the anatomical collection of the Laboratory of Neurosciences and Behavior (LNB) at the Department of Physiological Sciences, Institute of Biology, University of Brasília. The remaining four specimens belonged to the Brazilian Institute of Environment and Renewable Natural Resources (IBAMA) archive and were donated to the University of Uberlândia in the 1970s before being donated to the LNB. This study was approved by the Institutional Ethical Committee (CoEP-UFG 81/2008, with authorization from IBAMA number 15275).
2.2 Preparation of animals for dissection
All procedures involving the animals were performed in accordance with the guidelines of the Brazilian Society of Animal Experimentation (COBEA). After trichotomy using a razor blade, the animals were incubated in water at room temperature for 10–12 h; then, they received perfusion through the femoral vein with 10% formaldehyde and 5% glycerin for fixation. The animals were conserved in 10% formaldehyde in covered opaque cubes to avoid light penetration and the evaporation of formaldehyde.
2.3 Dissection and documentation
Hand dissection was performed on six subjects to expose their muscles, while the other two were prepared to study their hand joints and bones. All materials were registered using a digital camera, schematic drawings, and anatomical descriptions. The nomenclature of the hand muscles follows, wherever possible, the guidelines used by Gibbs (1999). The data collected were analyzed and compared with the patterns described for human, chimpanzee, gorilla, and baboon species.
2.4 Statistical analysis
The statistical analysis was based on the previous methods described by Aversi-Ferreira and colleagues (Aversi-Ferreira, 2009; Aversi-Ferreira et al., 2011; Aversi-Ferreira et al., 2014a; Aversi-Ferreira et al., 2014b; Aversi-Ferreira et al., 2015; Aversi-Ferreira et al., 2016). The methods used to calculate the similarities in the hand muscles among different species are summarized in Table 1. In this work, the bearded capuchin was primarily chosen as the reference (control species) for comparison with other primates, although they were also analyzed among themselves (Tables 2, 3, 5).
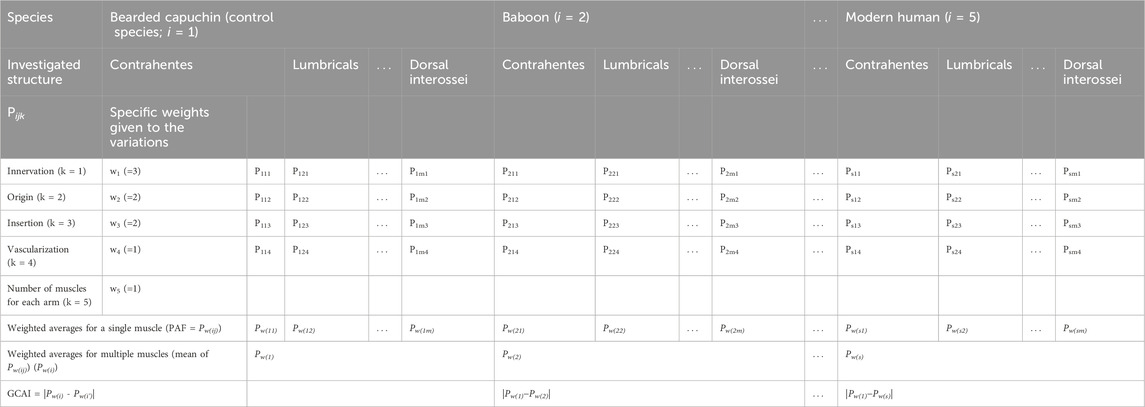
TABLE 1. General methods for calculating the similarities of muscles between Sapajus and other species (i.e., baboon, apes, and modern humans).
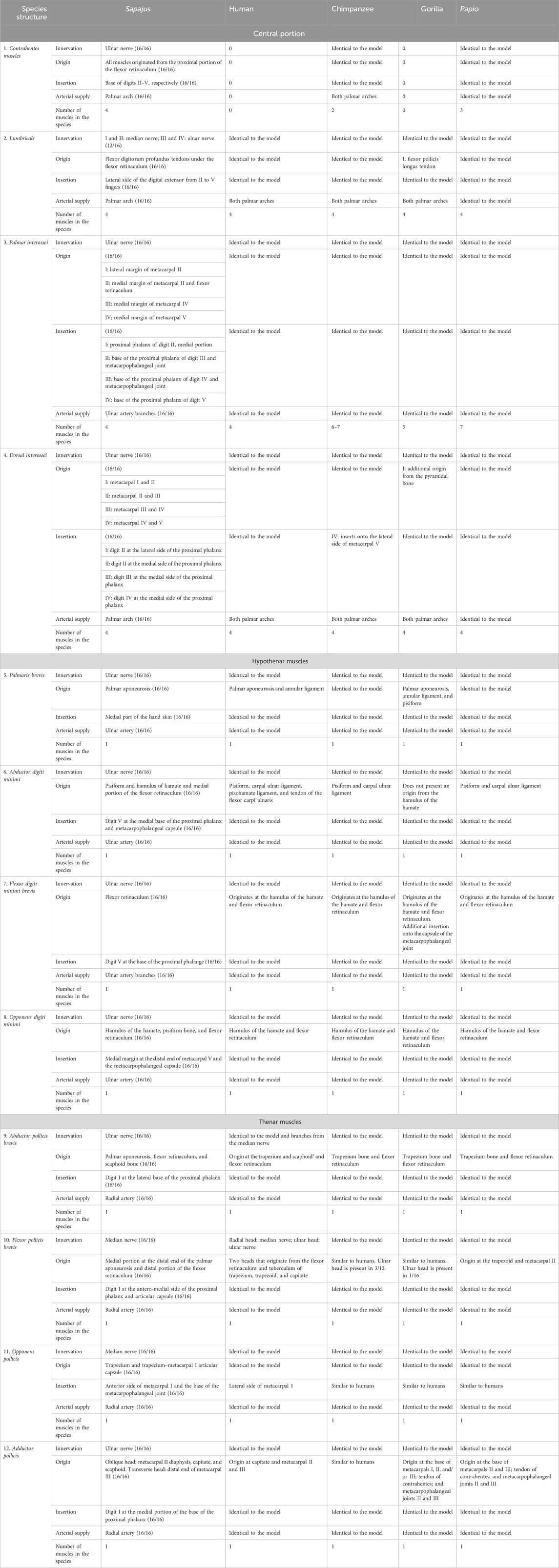
TABLE 2. Partial modal data of anatomical structures with some information about the structures that generate the data for CAI calculations.
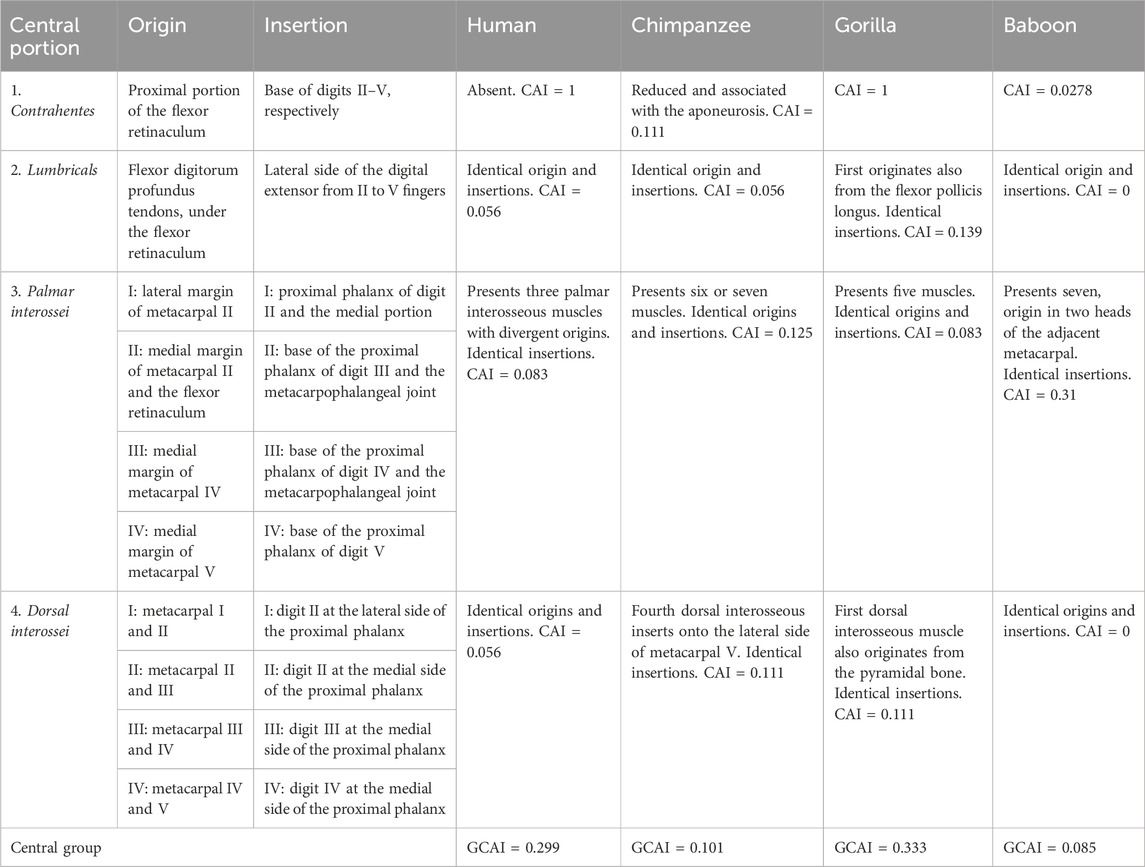
TABLE 3. Muscles of the central portion of the capuchin hand with origins and insertions compared with modern humans, apes, and baboons.
The values in Table 1 are calculated based on the following formula:
where P is the relative frequency, N is the total number of analyzed structures of the samples (Table 2), and rv is the number of normal structures.
To account for the influence of the weight on the relationship with the body size, Aversi-Ferreira et al. (2015) proposed the method of assigning weights based on the degree of evolutionary divergence. Accordingly, innervation was assigned a weight of 3, origin and insertion a weight of 2, vascularization a weight of 1, and the number of muscles per hand a weight of 1. This weighting scheme prioritizes features with less variation across the evolutionary scale, resulting in a CAI calculation that emphasizes phylogenetic relationships between the compared animals.
When the number of insertions, origins, and/or muscles differed from those of the bearded capuchin’s, the value of Pijk was calculated using the difference of 1 and a multiple of a ¼ of the number assigned to the control species (i.e., bearded capuchin). This multiple was the number of the structure greater or less than that ascribed to the control species. For instance, if the frequency Pijk of the control species was 1, then the origin of a muscle in bearded capuchins occurred from only one structure, and for other species, it occurred from three structures; the calculation of Pijk would be as follows:
for
This procedure was necessary because when it is not possible to obtain data directly from an animal, the consulted literature does not always supply information about the frequency of the muscles but rather provides the variations in the number, origin, or insertion:
Here,
where
where
Notably,
Here,
or
Here,
In general terms, to calculate CAI, the same pattern of innervation was considered for Sapajus, baboons, chimpanzees, and humans based on the study by Marin and colleagues (2009), while that for gorillas was based on Gibbs (1999). Vascularization was deemed identical between bearded capuchins and baboons and different between these and the remaining species considered, following Aversi-Ferreira (2009), Aversi-Ferreira and colleagues (2020), Gibbs (1999), and Swindler and Wood (1973). In this sense, baboons and bearded capuchins have only one palmar arch in the hand, whereas apes and modern humans have two; thus, the frequency of vascularization (Pijk) for bearded capuchins and baboons was 1, while it was ½ for the other species.
3 Results and discussion
3.1 Superficial description
The bearded capuchin hand presents an expressive proximal hypothenar eminence with the majority placed proximally to the line between the styloid process of the radius and ulna, with lesser distal hypothenar and thenar eminences (Figures 1A, B). After removing the skin, we observed much dense unmodeled connective tissue forming the eminences and also the palmar aponeurosis with bundles of collagen, which are associated with the tendons of muscles that insert on the hand, such as the palmaris longus (Figure 1C). On the medial side, it was possible to distinguish muscular fibers of the muscle palmaris brevis (Figure 1A). The size of the proximal hypothenar eminence indicated a place used to support locomotion-caused friction and also for prehension, as observed in other primates (Swindler and Wood, 1973).
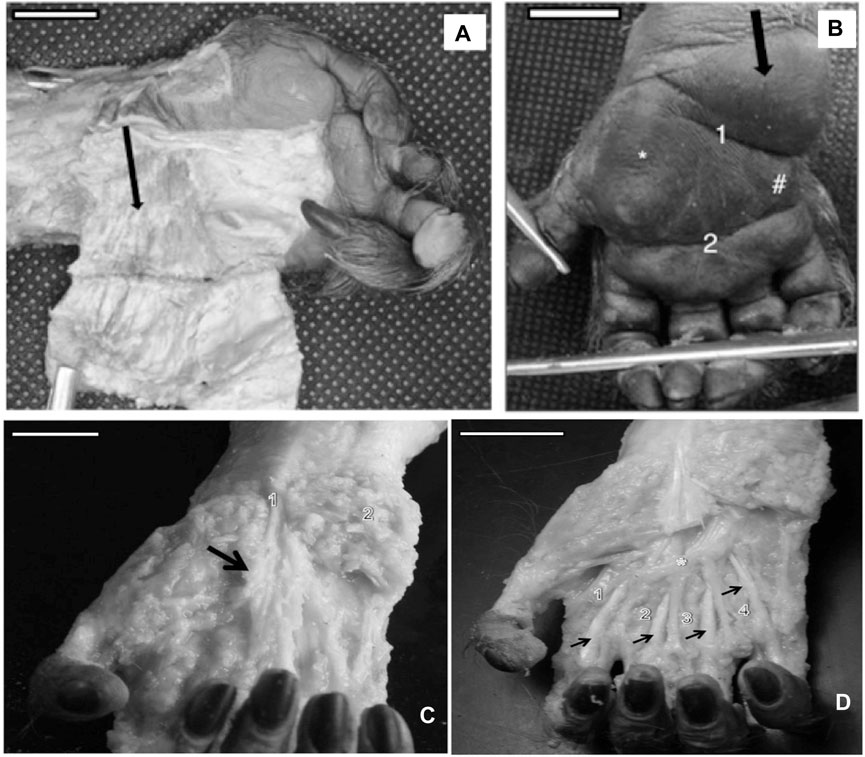
FIGURE 1. (A) Left hand of a bearded capuchin showing the dissected proximal hypothenar eminence (black arrow) and fibers of the muscle palmaris brevis (bar = 1.5 cm). (B) Black arrow indicates the nondissected proximal hypothenar eminence, * indicates the thenar eminence, # indicates the distal hypothenar eminence, 1 is the flexure proximal that separates the proximal from the distal eminence, and 2 indicates the simian flexure (bar = 1.3 cm). (C) Dissected right hand of a bearded capuchin exhibiting aponeurosis (arrow), a visible collagen bundle of the palmaris longus tendon (1), and the tissues of hypothenar eminence (2). Bar = 2.2 cm. (D) Dissected right hand of a bearded capuchin showing contrahentes (1, 2, 3, and 4) inserted in digits II–V and lying between the superficial and deep muscles (arrows). The asterisk (*) indicates the palmar arch unique to Sapajus (bar = 2.8 cm).
Furthermore, the thenar and distal hypothenar eminences were not as expressive as the proximal hypothenar eminences, presenting less connective tissues; however, it seemed to be associated with support for grabbing peanuts (Spinozzi et al., 2004).
Moreover, the flexure lines in the bearded capuchin hand displayed a different pattern, as cited by Swindler and Wood (1973), in relation to other primates; that is, the interdigital eminences observed in Papio were not present; the simian line did not terminate or take direction for the second interdigital space, as in modern humans, Pan, and Papio; and no deep longitudinal flexure lines were observed. Two transversal lines were evident in Sapajus hands, namely, a proximal line that separates the proximal from distal hypothenar eminences and terminates on the proximal part of the thenar eminence and a distal eminence—the simian line that crosses all palms.
Lastly, the primitive pattern of pentadactylism with a basic phalangeal formula (i.e., 3 >4 >2 >5 >1) observed in primates (Gibbs, 1999) was verified in the Sapajus hands.
3.2 Muscles
The intrinsic muscles of the hand are divided into the thenar, hypothenar, and central groups (Swindler and Wood, 1973). While the thenar and hypothenar muscles are associated with the thumb and digit V, respectively, the central group is associated with all fingers. Nevertheless, all muscle groups originate in the central proximal area of the hand.
3.2.1 Central muscles of the hand
The contrahentes muscles act as a superficial layer in the palm, immediately below the palmar aponeurosis level (Table 3). All bearded capuchin specimens analyzed in this study presented four contrahentes in their hands. They originated in the proximal portion of the retinaculum of the flexor muscles and were inserted in the base of digits II, III, IV, and V. Their position lays between the superficial and deep flexor muscles of the fingers (Figure 1D). They were innervated through the ulnar nerve.
Humans do not present contracting muscles, while baboons have three and chimpanzees have only two that are reduced and aponeurotic, lacking the digit II contrahens muscle; otherwise, all have the same origin in the species (Swindler and Wood, 1973). Gorillas, on the other hand, do not display distinct contrahens muscles (Gibbs, 1999). According to Swindler and Wood (1973), the contrahentes are innervated through the ulnar nerve, identical to the bearded capuchins investigated in the present study.
Notably, there are varying numbers of contrahens muscles across the species considered here: humans and gorillas present no such muscles; chimpanzees present two muscles; and baboons present three muscles. Therefore, Pijk was ascribed the values of 0, 0, ½, and ¾ for humans, gorillas, chimpanzees, and baboons, respectively, for the purpose of the CAI calculation.
The lumbrical group of muscles comprised four muscles elongated along the sagittal plane. They had a double origin, except the first, from the tendons of the flexor digitorum profundus muscle in all bearded capuchins analyzed in this study; they were inserted onto the extensor aponeurosis and onto the lateral side of the proximal phalanges of fingers II–V (Figure 2A). According to Swindler and Wood (1973), they are identical in all primates: the first and second lumbricals are innervated through the median nerve, while the third and fourth lumbricals are innervated through the ulnar nerve.31 In the bearded capuchins investigated in this study, this pattern of innervation was not observed in their four hands, while in the others, the branches were cut.
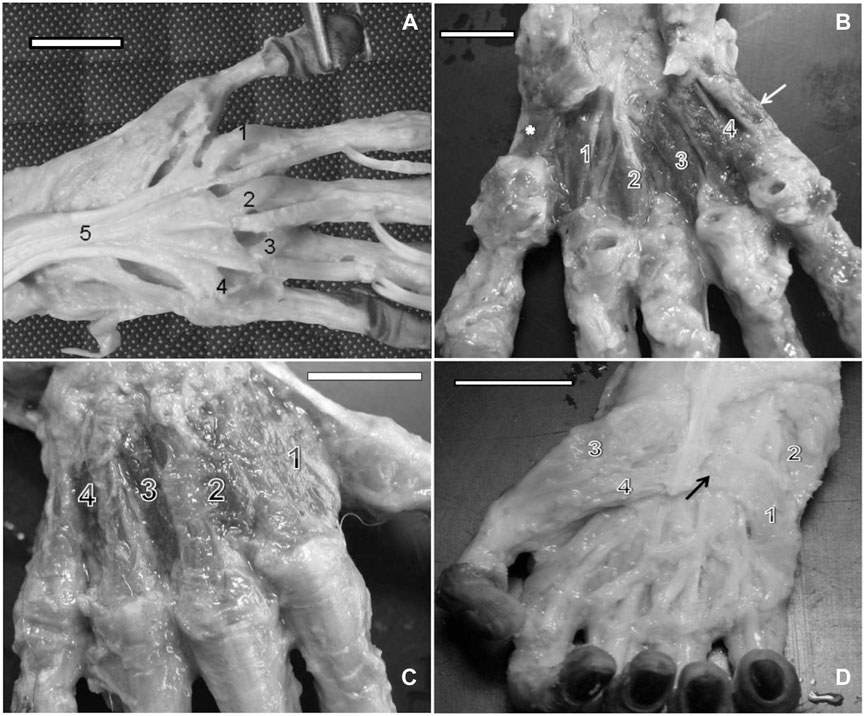
FIGURE 2. (A) Left hand of a bearded capuchin. Lumbrical muscles are numbered from 1 to 4. Number 5 indicates the tendon for the deep flexor muscle of the fingers (bar = 2.2 cm). (B) Left hand of a capuchin. Dissection revealed palmar interosseous muscles (1, 2, 3, and 4), the digit IV opposing muscle (arrow), and the thumb opposing muscle (*) (bar = 2.4 cm). (C) Right hand of a bearded capuchin. Dissection revealed dorsal interossei (1, 2, 3, and 4; bar = 3.2 cm). (D) Right hand of a capuchin. Dissection revealed the origin tendon of the flexor digiti minimi brevis (arrow) and its ventral portion (1), abductor digiti minimi (2), abductor pollicis brevis (3), and flexor pollicis brevis muscles (4) (bar = 2.8 cm).
Gibbs (1999) reported that all modern humans and apes present four lumbricals (Standring, 2008), which originate from the tendon of the flexor digitorum profundus, as observed here in the bearded capuchins; however, the first lumbrical originates from the tendon of the flexor pollicis longus only in gorillas.
Moreover, there were four palmar interosseous muscles located between two adjacent metacarpal bones. The first palmar interosseous muscle originated in the lateral margin of the metacarpal II and the retinaculum of the flexor muscles and was inserted into the base of the proximal phalanx of digit II, next to the insertion tendon of the first dorsal interosseous muscle. The second palmar interosseous muscle originated in the medial margin of metacarpal II and in the retinaculum of the flexor muscles and was also inserted at the base of the proximal phalanx of digit II but next to the metacarpophalangeal articulation capsule. The third muscle stemmed from the medial margin of metacarpal IV and was inserted into the base of the proximal phalanx of digit IV, associated with the corresponding metacarpophalangeal articulation capsule. Finally, the fourth muscle originated in the medial margin of metacarpal V and was inserted at the base of the proximal phalanx of digit V (Figure 2B). All muscles were innervated through the ulnar nerve and supplied by branches of the ulnar artery.
The palmar interosseous muscles range from three to seven among primates. Baboons have seven, with different origins (i.e., with two heads for all of them), with the same insertions in relation to Sapajus (Swindler and Wood, 1973).
Gibbs (1999) reported five such muscles in gorillas and from six to seven in chimpanzees, and in modern humans, Pan paniscus, and gorillas, those muscles are innervated through the deep ramus of the ulnar nerve; in great apes and modern humans, they have the same origin and were inserted into the proximal phalanges and dorsal aponeurosis of each finger, identical to what was observed in Sapajus; however, in gorillas, they are inserted onto the capsules of the metacarpophalangeal joints.
Dorsal interossei are the only intrinsic muscles in the dorsal portion of the hand. In bearded capuchins, they originated on the adjacent sides of two metacarpal bones. They were all feather-shaped muscles except for the first, which was flabelliform. No insertions in digits I and V were observed. Digits II and III received insertions in the lateral and medial sides of their proximal phalanges from the first, second, and third interosseous muscles, whereas digit IV received an insertion from the fourth interosseous muscle in its medial portion (Figure 2C). They were innervated through the ulnar nerve in bearded capuchins.
This group is identical in all of the studied primates considered here (Swindler and Wood, 1973; Gibbs, 1999); however, an additional origin of the first dorsal interosseous from the pyramidal bone was reported in gorillas, while in chimpanzees, the fourth dorsal interosseous is inserted onto the lateral side of the metacarpal V,31 different from what was observed in bearded capuchins. All dorsal interossei are innervated through the ulnar nerve in all primates studied here (Swindler and Wood, 1973; Gibbs, 1999).
Lastly, the central muscle group of bearded capuchins is more similar to those in baboons and chimpanzees and exhibits more differences when compared with modern humans and gorillas. The main differences between bearded capuchins and baboons in central muscles were observed in the palmar interossei in terms of the origin and number (Table 3).
3.2.2 Hypothenar muscles
The palmaris brevis in bearded capuchins exhibited a triangular form, originated from the palmar aponeurosis, and inserted onto the medial part of the hand skin (Figures 1A, B) (Table 3). Furthermore, it was innervated through the ulnar nerve. Data on the palmaris brevis muscle are scarce in primates, but it originates from the palmar fascia in modern humans and chimpanzees,32 from the annular ligament in modern humans and gorillas and also from the pisiform in gorillas; furthermore, it is inserted onto the skin of the ulnar border of the palm and is innervated through the ulnar nerve in all primates cited here (Swindler and Wood, 1973; Gibbs, 1999).
The palmaris brevis of bearded capuchins was identical to that of baboons, very similar to that of chimpanzees, and presented some differences from those of humans and gorillas in that order (Table 4).
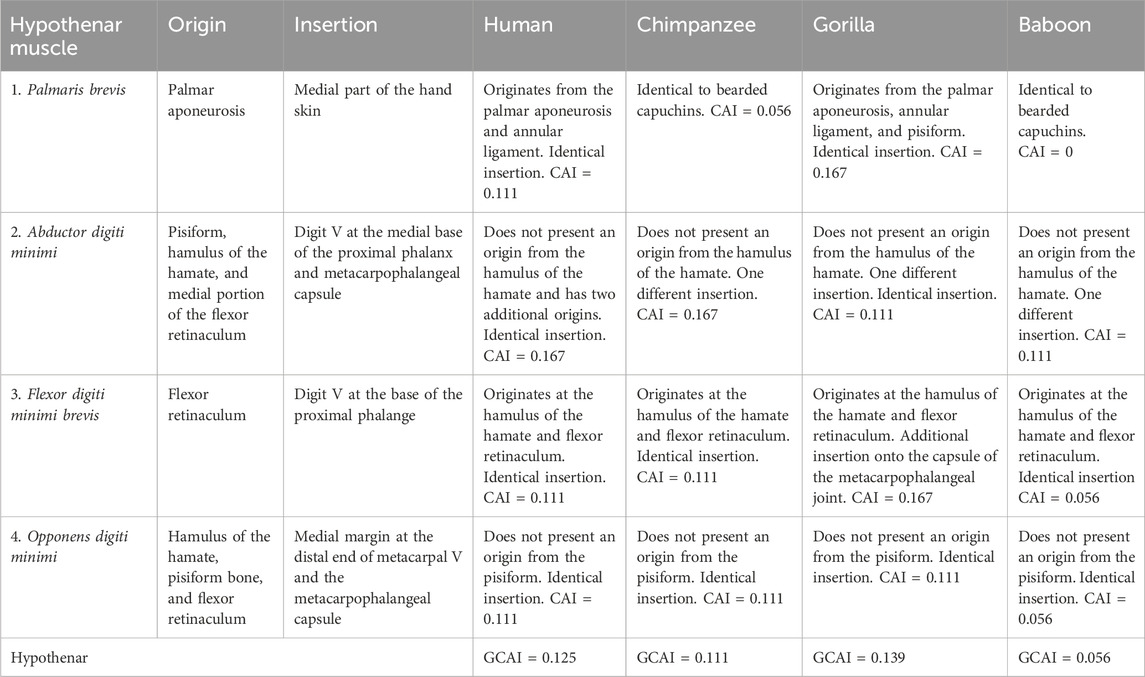
TABLE 4. Hypothenar muscle of capuchins with the origins and insertions compared with modern humans, apes, and baboons.
The abductor digiti minimi is located at the superficial medial portion of the hand, originated from the pisiform, hamulus of the hamate, and medial portion of the flexor retinaculum (Figures 2D, 3A, B). It is inserted into the medial portion of the metacarpophalangeal articulation capsule of digit V, extending to the base of the proximal phalanx blended with the flexor digiti minimi brevis (Figures 2D, 3A, B). It is innervated through the ulnar nerve.
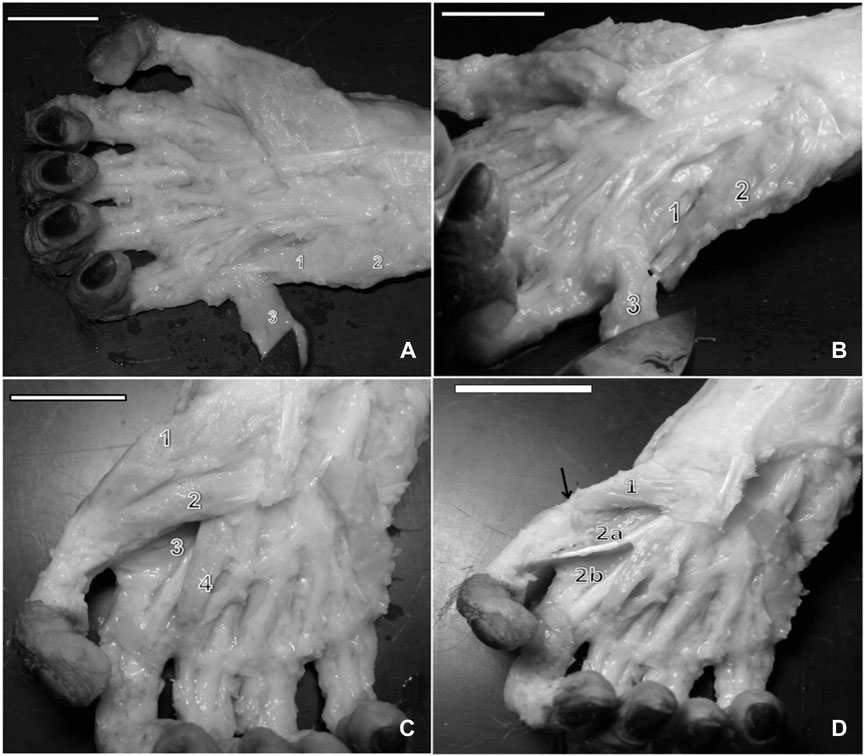
FIGURE 3. (A) Right hand of a capuchin. Numbers 1 and 2 indicate the venter of the abductor digiti minimi muscle, and number 3 indicates the center of the flexor digiti minimi brevis, opened (bar = 2.8 cm). (B) Right hand of a capuchin Dissection revealed the opponens digiti minimi (1), (2) abductor digiti minimi muscle, cut, and (3) flexor digiti minimi brevis, opened (bar = 2.8 cm). (C) Right hand of a capuchin. Dissection revealed digit I’s (1) abductor pollicis brevis, (2) flexor pollicis brevis; (3) adductor muscle, transverse head, and (4) the first lateral contracting muscle, cut (bar = 2.8 cm). (D) Right hand of a capuchin. Dissection revealed (arrow) the tendon of the insertion of (1) the opponens pollicis muscle and the adductor muscle and the (2a) oblique head and (2b) the transverse head (bar = 3.0 cm).
In humans, chimpanzees, baboons (Swindler and Wood, 1973), and gorillas (Gibbs, 1999), the muscle originates from the pisiform bone and carpal ulnar ligament, although it originates from the pisohamate ligament and the tendon of the flexor carpi ulnaris in humans (Gibbs, 1999). None of the primates exhibited an origin from the hamulus of the hamate, as verified in Sapajus. The insertion for this muscle in these species is identical to that in bearded capuchins, namely, onto the medial base of the proximal phalanx32 and blended with the flexor digiti minimi brevis. As a variation in modern humans, however, it is also inserted onto the extensor aponeurosis of digit V in modern humans as well as in chimpanzees and onto the capsule of the metacarpophalangeal joint in gorillas (Gibbs, 1999), similar to bearded capuchins. In all primates studied here, innervation takes place through the ulnar nerve (Swindler and Wood, 1973; Gibbs, 1999).
According to the CAI calculation, the abductor digiti minimi was found to be similar among baboons, gorillas, and bearded capuchins.
In bearded capuchins, the flexor digiti minimi brevis muscle is a flat muscle originating from the flexor muscle retinaculum in the medial portion of the hand (Figures 2D, 3A, B). It lies obliquely and is inserted into the medial part of the digit V proximal phalanx base; it is distally located at the insertion of the abductor digiti minimi muscle, with which it shares its fibers, and is innervated through the ulnar nerve.
The origin at the hamate’s hamulus and flexors’ retinaculum, insertion onto the base of the proximal phalange of digit V, which is in common with the abductor digiti minimi, and innervation through the ulnar nerve have been reported for all other primates (Swindler and Wood, 1973; Gibbs, 1999). However, Gibbs (1999) reported additional insertions in gorillas onto the metacarpophalangeal joint and the extensor tendon on the dorsal surface. The flexor digiti minimi brevis is more similar to that of baboons, whereas the additional two insertions are more different from those of gorillas.
The opponens digit minimi is located beneath the abductor digiti minimi. In capuchins, it originates from the hamate’s hamulus, pisiform bone, and flexor muscle retinaculum. It is inserted onto the distal end of metacarpal V, at the medial margin, and in the articulation capsule of the metacarpophalangeal joint (Figures 3C, D).
In all other primates, it originates from the hamate bone and the flexor muscle retinaculum, the insertion occurs on the ulnar margin of metacarpal V, and innervation takes place through the ulnar nerve (Gibbs, 1999; Standring, 2008). The origin of the opponens digiti minimi in the pisiform in primates other than the Sapajus was not mentioned in any of the articles analyzed here, but other features of the opponens digiti minimi were identical among all primates.
The difference in the calculated CAI for baboons compared to Sapajus stems from the origin of the pisiform muscle in the latter. The variations in CAI for Pan, gorillas, and humans arise due to the presence of two palmaris arcus muscles in their hands. Specifically, the CAI values were 0.056 for baboons and 0.111 for the other three species (Table 3).
The hypothenar muscles in capuchins exhibit distinct origins compared with the other primates considered here, but differences exist in insertions, mainly in relation to gorillas. Notwithstanding, according to Swindler and Wood (1973), the abductor digiti minimi occasionally exhibits an origin in the hamate’s hamulus in humans. However, Standring (2008) reported a consistent origin in the pisohamate ligament for humans.
In the CAI calculation, the differences in bearded capuchins in terms of origins and insertions were largest compared to modern humans and gorillas.
3.2.3 Thenar muscles
Swindler and Wood (1973) found that the abductor pollicis brevis muscle is the main component of the thenar eminence in chimpanzees, baboons, and humans (Table 5). It also seems to be the case in gorillas, although this was not directly mentioned by Gibbs (1999). In capuchins, it overlays digit V’s opponent muscle and the lateral part of the short flexor muscle. It originates from the palmar aponeurosis, flexor muscle retinaculum, and scaphoid bone and is inserted into the lateral portion of the proximal phalanx base, associated with the corresponding articulation capsule (Figures 3C, D).
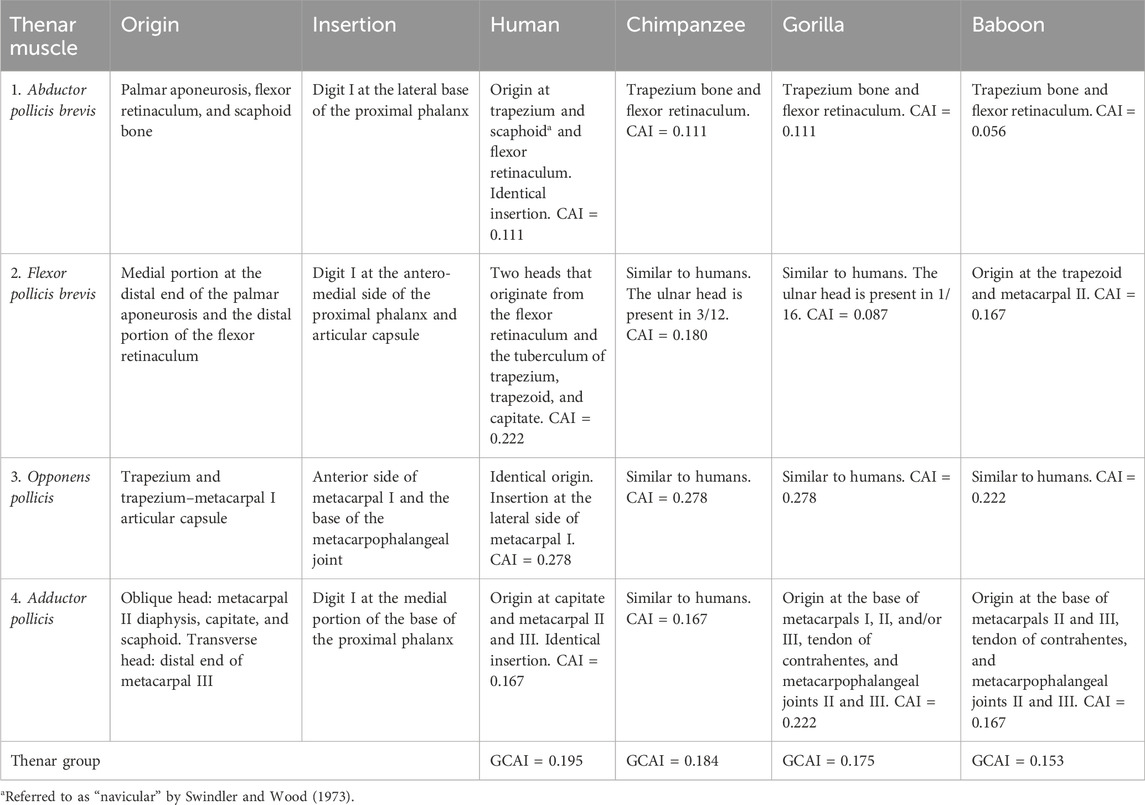
TABLE 5. Thenar muscles of capuchins with the origins and insertions compared with modern humans, apes, and baboons.
The abductor pollicis brevis was observed to originate from the scaphoid bone in all Sapajus specimens dissected in this study and was innervated through the ulnar nerve. This is without precedent in comparison with primate species. In humans, discrepancies exist regarding its origin from the navicular bone (Swindler and Wood, 1973) and scaphoid bone (Gibbs, 1999), but studies are in agreement regarding its origin from the trapezium bone. Napier (1955) indicated the flexor retinaculum, tuberculum of the scaphoid, and the crest of the trapezium to be the common origins for this muscle in humans and that it shares fibers with the flexor pollicis brevis. In gorillas and baboons, the bone origin starts from the trapezium bone (Swindler and Wood, 1973; Gibbs, 1999). Once again, insertion and innervation through the ulnar nerve are identical in all primates considered here; however, branches from the median nerve were cited for humans (Gibbs, 1999).
The flexor pollicis brevis originates from the medio-distal portion of the palmar aponeurosis and distal portion of the flexor muscle retinaculum; furthermore, it is inserted into the anterior-medial margin of the proximal phalanx, associated with the articulation capsule (Figures 3C, D). The deep head of the short flexor muscle was not observed in the bearded capuchin specimens. It shares fibers with the abductor pollicis brevis. The tendon of insertion of the long flexor muscle is located deep and medially relative to the distal portion of the flexor pollicis brevis. It is innervated through the median nerve.
In apes and baboons, the flexor pollicis brevis presents two heads, namely, the radial or superficial and the ulnar or deep head (Swindler and Wood, 1973; Gibbs, 1999). The radial head originates from the flexor retinaculum in modern humans, all apes (Gibbs, 1999), and baboons (Swindler and Wood, 1973) and from the tubercle of the trapezium in chimpanzees and modern humans (Swindler and Wood, 1973; Gibbs, 1999) and is innervated through the median nerve. The ulnar head originates from the trapezoid and capitate in modern humans and chimpanzees when present (Swindler and Wood, 1973; Gibbs, 1999; Standring, 2008) and from the trapezoid and base of metacarpal II in baboons, which is always present (Gibbs, 1999). Moreover, it is innervated through the ulnar nerve. Insertion is identical in all primates (Swindler and Wood, 1973; Gibbs, 1999).
Gibbs (1999) claimed that the ulnar head is present in 3/12 of Pan and 1/16 of gorillas; therefore, the frequency (Pijk) of innervation, origin, and the number for the calculation of CAI in each of these species was ½ (1–3/4 × 3/12) and ½ (1–3/4 × 1/16), respectively.
Due to doubts about the presence of the ulnar head in modern humans, Day and Napier (1963) analyzed the heads of the flexor pollicis brevis muscle in 65 human corpses. They concluded that it presents two heads at the origin, thus settling the controversy surrounding this issue.
According to Gibbs (1999), an ulnar head is found in all primates, in which the thumb performs true opponency. A detailed anatomical study (Aversi-Ferreira et al., 2014c) demonstrated that bearded capuchins do not perform opponency, despite their high cognitive capacity for tool use, but they use precision grip as a lateral pinch.
The flexor pollicis brevis is more similar between bearded capuchins and gorillas, mainly because of the similarity of the frequency of the number.
The opponens pollicis originates from the trapezium bone and the trapezium–metacarpal articulation capsule. It is inserted onto the anterior margin of metacarpal I and the base of the metacarpophalangeal capsule, and it is innervated through the median nerve. It is located underneath the abductor pollicis brevis, with which it shares fibers (Figure 3C).
In chimpanzees, baboons, and modern humans, the opponens pollicis is innervated through the median nerve, originating from the radial transverse carpal ligament and trapezium (Swindler and Wood, 1973). According to Gibbs (1999), it is absent in Pan. The insertion is similar to the primates studied here, namely, onto the radial margin of metacarpal I; however, in bearded capuchins, the position of the insertion is anteriorly placed, which is an important aspect in relation to the angle of the movement of the pollicis. In Sapajus, it is more similar to baboons because of the supplying pattern.
This muscle exists in all primates, with its development peaking in humans according to Swindler and Wood (1973). Napier (1955) defined it as a rotatory muscle located in a privileged position for acting on the trapezium–metacarpal articulation. The different insertions found in capuchins indicate more of a flexing rather than a rotating action (Aversi-Ferreira et al., 2014c). It does not allow, for instance, the cushion of the thumb distal phalanx to rotate toward the cushion of the other digits. In fact, the flexing action of digit I’s opponent muscle is more consistent with the phylogenetic history since in lower mammalians, it is fused with the flexor pollicis brevis in digit I.
The adductor pollicis of capuchins has two heads: oblique and transverse. The transverse head originates from the distal end of the third metacarpal and is inserted into the medial portion of the proximal phalanx base of digit I (Figures 3C, D). The oblique head has an identical insertion but a different origin, originating from metacarpal II’s diaphysis, capitate, and scaphoid bones. This last origin was not reported in the compared primates. The adductor pollicis is innervated through the ulnar nerve in bearded capuchins.
Moreover, humans and chimpanzees exhibit an identical origin for this head in the capitate bone and bases of metacarpal II and III; however, in baboons (Swindler and Wood, 1973) and gorillas (Gibbs, 1999), the origins from the capitate and scaphoid bones have not been reported. There are four origins for the two muscle heads in bearded capuchins with common insertion in three instances (namely, metacarpal II, capitate bone, and metacarpal III for the transverse head) in chimpanzees and modern humans; by contrast, in gorillas and baboons, they present five insertions (Swindler and Wood, 1973; Gibbs, 1999), but only two of them are identical in bearded capuchins. The insertion is identical in bearded capuchins and other primates, namely, onto the base of the first pollical phalanx, and the tendon of the distal phalanx has been reported in baboons, chimpanzees (Swindler and Wood, 1973), and gorillas (Gibbs, 1999), but it was not observed in Sapajus. For the primates studied here, the innervation of the adductor pollicis takes place through the ulnar nerve. Taken together, the capuchin’s thenar group is more similar to that of baboons.
According to the descriptive analysis and GCAI results (Tables 2, 3, 5), Sapajus hand muscles are similar to those of baboons. It is crucial to note that the vascularization of the hand is identical between capuchins and baboons but is different compared with the other primates (Aversi-Ferreira et al., 2007a; Aversi-Ferreira et al., 2007b; Aversi-Ferreira et al., 2007c; Aversi-Ferreira, 2009; Abreu et al., 2021; Aversi-Ferreira et al., 2021b), while innervation is identical in all of them (Marin et al., 2009), except for the presence of the second head of the flexor pollicis brevis, which is innervated through the ulnar nerve. A higher similarity with baboons was also reported for other parts of the thoracic limb, as cited in the Introduction section.
In addition, the thenar muscles in the human hand are stronger and bulkier than those in other primates (Young, 2003), even chimpanzees (Ogihara et al., 2005), whose hands are used as an evolutionary model for hominid tool use. Bearded capuchin thenar muscles are not as strong and bulky as those of chimpanzees and do not seem to be more adapted for tool use than those of baboons in terms of the muscular structure, corroborating the data of Aversi-Ferreira and colleagues (2014a).
According to Young (2003), the evolution of the human hand is associated with tool use and bipedalism, as bipedalism was necessary to permit the free use of their hands. Tocheri and colleagues (2008) suggested a correspondence between cognitive evolution and hand morphology in primates. Our analysis of Sapajus hand muscles does not support tool-use behavior in the way that it is credited to this genus. The hand of the Sapajus monkey is more similar to that of baboons in terms of the central, hypothenar, and thenar muscles as well as other structures observed here (Tables 2, 3, 5; Figure 4), while baboons do not exhibit the muscle requirements for the hand skills associated with fine tool use; nevertheless, capuchins perform tool use and demonstrate high cognition abilities (Lacreuse and Fragazy, 1997; Panger, 1998; Fragazy et al., 2004; Abreu et al., 2021). Other data regarding Sapajus hands have also demonstrated a primitive characteristic compared with great apes and modern humans (Aversi-Ferreira et al., 2010; Aversi-Ferreira et al., 2011; Aversi-Ferreira et al., 2011b; Aversi-Ferreira et al., 2014c).
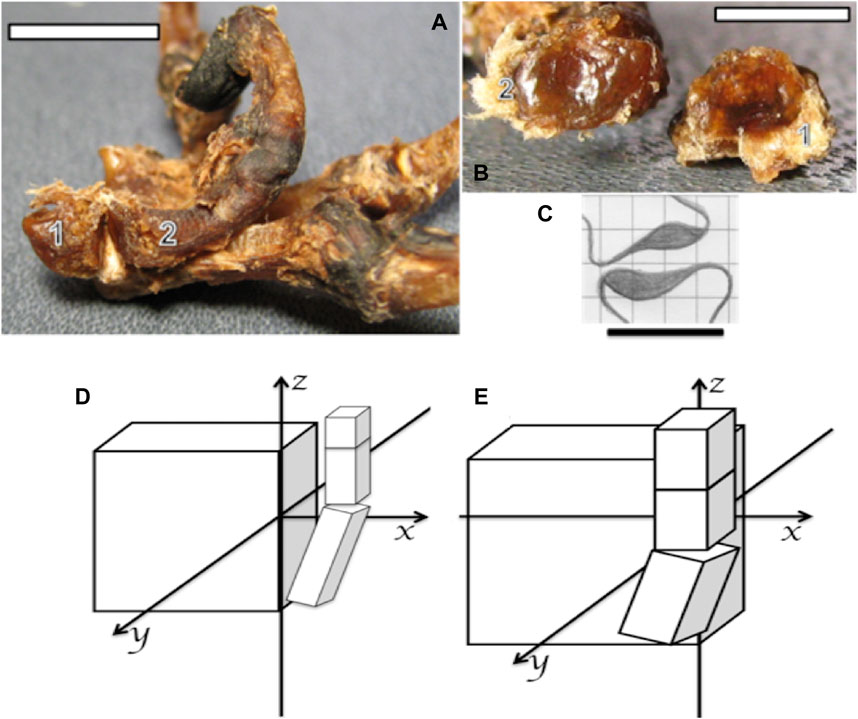
FIGURE 4. (A,B) Photographs of the bones and ligaments of the right hand of a capuchin. Number 1 indicates the trapezium and number 2 the metacarpal I. (A) Position of bones 1 and 2 in the formation of the trapezium–metacarpal joint (bar = 16 cm). (B) Articular faces of bones 1 and 2 showing concave (bone 2) and convex (bone 1) surfaces (bar = 32 cm). (C) Schematic association between the trapezium (down) and metacarpal I (up) to form a saddle joint, proportionally obtained from bearded capuchin bones (bar = 25 cm). (D) Schematic representation of a Sapajus hand, in which the projection of the thumb over the plane of the hand is limited to the z-axis; that is, the carpus aligns in a flat plate or a few centimeters past it in the latero-medial direction. (E) Schematic representation of a human hand, indicating the projection of the thumb over the plane of the hand; that is, the curved carpus and crossing parallel to the z-axis due to the anterior position of the trapezium bone (based on Aversi-Ferreira et al., 2014a; Aversi-Ferreira et al., 2014c).
The muscle anatomical data from this work expands upon previous bone studies of a Sapajus hand (Aversi-Ferreira et al., 2014c). Those studies demonstrated, through general measures of the carpus and digits, that these primates lack the capacity for true thumb opponency. Our findings further support this conclusion by revealing that the Sapajus carpus is straighter than the human carpus due to the absence of a central bone in the former. Consequently, the first metacarpus originates in the same line as the carpus plane in capuchins, hindering the movement of the cushion of the pollicis toward the base of the first phalanx of other digits. In fact, the opponens pollicis action observed in this work more closely resembles the flexor action rather than true opponency. This, along with the saddle trapezium–metacarpal joint observed in Sapajus (Aversi-Ferreira et al., 2014c), confirms that capuchins lack the capacity for human-like thumb opponency.
In contrast, while great apes possess the ability for thumb opponency, their thumbs are generally small and weak, limiting their object manipulation skills. Notwithstanding, despite lacking true opponency, Sapajus exhibits remarkable skills in manipulating objects (Fragaszy et al., 2004; Fragaszy et al., 2004). This dexterity relies on the combined strength of various hand movements, primarily involving the second and third digits, the first digit in conjunction with the second metacarpal/second phalanx base (Aversi-Ferreira et al., 2014c). Therefore, bearded capuchins leverage powerful hand muscles to achieve great hand skills in manipulating objects, deviating from the expectations set by Darwin’s theory of human evolution (Darwin, 1871). The combined insights into linear hand measures, bone anatomical analyses (Aversi-Ferreira et al., 2014c), and the data from this present work unveil an alternative pathway for achieving advanced hand manipulation in Sapajus, distinct from both great apes and modern humans.
4 Final considerations
Considering other works on hand manipulation and anatomy, the Sapajus trapeziometacarpal joint exhibits a latero-laterally concave part (metacarpal I) and latero-laterally convex part (trapezium), generating a saddle joint-like arrangement (Aversi-Ferreira et al., 2014a). This differs significantly from the configuration observed in great apes and modern humans (Figures 4A–C).
Such a joint restricts the medial rotation of thumbs, hindering true opponency (Napier, 1961; Napier, 1980), as shown in Figure 4. Aversi-Ferreira et al. (2006) reported that capuchins possess nine carpal bones, one more than adult great apes and humans. This central bone contributes to a flat carpal plane, indicating a relatively primitive hand structure. Conversely, great apes and modern humans exhibit a curved carpus capable of true opponency (Aversi-Ferreira et al., 2011). Therefore, the capuchin hand pattern positions the thumb closer to the carpal plane, limiting opponency, while the curved human carpus allows the thumb to move above the hand plane and across the additional axes, namely, the y- and x-axes, and parallel to the z-axis, as indicated in Figures 4D, E.
These analyses reveal that the Sapajus hand more closely resemble those of arboreal primates (Aversi-Ferreira et al., 2014a), consistent with the findings of the current study and other studies comparing the primate blood supply (Aversi-Ferreira et al., 2020; Aversi-Ferreira et al., 2021b). However, linear hand proportion measurements suggest that capuchin hands are closer to those of humans than other primates (Almecíja et al., 2015). Despite that, a three-dimensional analysis (Aversi-Ferreira et al., 2014c) demonstrated that the flat plane hand of Sapajus is more similar to baboons than humans.
Through precise analyses of anatomical structures rather than behavioral observations, we derived propositions for generating a deductive corollary regarding the tool use and high manual abilities of capuchins. Subsequently, we employed inductive reasoning to generate new hypotheses for subsequent works exploring thumb opponency, tool use associated with encephalon characteristics (Borges et al., 2015; Aversi-Ferreira et al., 2021a; Abreu et al., 2021), and primate evolution.
According to our comparative analysis of hand muscles, the bearded capuchin hand exhibits a closer similarity to that of baboons than modern humans and great apes based on both the CAI calculations and gross anatomical observations. This is primarily due to the absence of the ulnar head of the flexor pollicis brevis and the flexion action of the opponens pollicis, which are the feature characteristics of primates lacking true opponency (Gibbs, 1999). We propose this primitive characteristic of bearded capuchin hand muscles as proposition 1.
Furthermore, Aversi-Ferreira et al. (2014c) concluded that capuchins possess a relatively primitive hand based on the articular surfaces of the carpus–thumb joint and carpal dimensions, indicating limited thumb movement. This finding reinforces the distance between capuchins and humans/great apes and suggests a greater affinity with arboreal specialist primate hands. However, in terms of hand proportions, using the intrinsic hand proportion method (Almécija et al., 2015), Sapajus was found to be closer to modern humans due to its relative thumb length. Notably, Almécija et al.’s (2015) analysis was linear, while Aversi-Ferreira et al. (2014c) considered the three-dimensional shape of the carpal bones, including the features of the hand palm (i.e., less arched carpus and less tridimensionality compared to apes and humans). Therefore, based on both our muscle data and the bone structures described by Aversi-Ferreira et al. (2014c), we propose that the bearded capuchin hand exhibits primitive characteristics, constituting proposition 2.
Indeed, the corollary derived from propositions 1 and 2 suggests that the Sapajus hand lacks the morphological characteristics necessary for enabling true thumb opponency or advanced tool use. This makes it a relative primitive hand among primates, supported by the analyses of both bones (Aversi-Ferreira et al., 2014c) and muscles in this work. This conclusion is further corroborated by the evolutionary distance between Sapajus and humans.
The association between thumb opponency, tool use, and high cognitive skills in primates is well established, supported by various studies focusing on apes (Napier, 1952; Napier, 1961; Ogihara et al., 2005; Tocheri et al., 2008). Given the high cognitive and manipulative abilities of capuchins, exploring their evolutionary path toward these characteristics becomes intriguing (Bortoff and Strick, 1993). The standard evolutionary model for these skills in apes emphasizes the pivotal role of adaptation to bipedalism and tool use (Young, 2003; Tocheri et al., 2008). While bearded capuchins exhibit only occasional and limited bipedalism (Demes, 2011), making free hand movements challenging during locomotion, they utilize their hands freely during intermittent bipedal phases, such as when foraging. Phylogenetic studies based on DNA analysis indicated that the Sapajus genus is relatively distant from apes (Goodman et al., 1998). Nevertheless, bearded capuchins present cognitive abilities that parallel those of great apes (Reader et al., 2011). In light of their thoracic limb morphology (Aversi-Ferreira et al., 2005; Aversi-Ferreira et al., 2010; Aversi-Ferreira et al., 2014c), especially the form of their hand, capuchins seem to have undergone a divergent evolutionary process to achieve manipulation, indicating that behavioral convergence did not follow anatomical convergence with the apes.
It has recently been argued that the adoption of incremental terrestriality by arboreal primate species may have been crucial to the development of tool use in primates (van Schaik et al., 1999). This account is not necessarily in opposition to ours. First, terrestrialism does not precisely equate to bipedalism in terms of the conditions for complex manipulation. Although bipedalism substantially spares the upper limbs from the locomotory function, the use of complex tools is generally a stationary activity. Furthermore, terrestriality without bipedalism could still be advantageous for arboreal species in the handling of objects since less effort, and consequently, fewer limbs are necessary for maintaining balance on the ground. Second, reports exist on complex tool use in the arboreal habitats of wild capuchins and chimpanzees (Sugiyama, 1997; Boesch et al., 2009; Sanz and Morgan, 2009; Souto et al., 2011). This suggests that arborealism does not prevent the complex manipulation of objects completely, even if terrestriality seems to favor more complex interactions with tools (Meulman et al., 2012). Regardless, the present findings support the hypothesis that refined manipulatory skills may emerge in the absence of erect bipedalism.
According to Darwin (1871) and Washburn (1963), bipedalism, large brains, manipulative hands, tool use, and language are the interconnected traits associated with progressive hominid evolution.
The present investigation agrees with the view expressed by Fleagle (1988) that “the cluster of features characterizing living humans are not necessarily linked but are rather evolved one by one.” We have demonstrated that the morphological basis of the manipulatory behavior in Sapajus sp. is based on different hand traits (especially in the thenar sector) than those found in catarrhines (selected Old World monkeys, great apes, and Hominidae). In the latter infraorder, precision grips facilitated by a hypermobile trapezium–metacarpal joint permitted fine manipulation, leading to tool use and manufacture; that is, Sapajus sp. does not present this model. It uses most of its pliable carpal and metacarpal joints to “fold in” the palm and fingers around the object to be manipulated. Several Old and New World primates indicate that a habitual arboreal locomotory behavior provides an alternative pathway for manipulatory behavior and neocortical growth (Abreu et al., 2021) as well as elaboration from the one demonstrated by advanced hominids. Further studies employing detailed analyses of hand bone proportions using dimensional analysis would be valuable in further elucidating the evolutionary trajectory of the primate hand morphology and its relationship with manipulative and cognitive abilities. In summary, the morphological and phylogenetical findings derived from DNA analyses place capuchins as distantly related to apes. Therefore, the obligatory relationship between erect bipedalism, manipulatory hands centered on the hypermobile trapezium–metacarpal joint, elaboration of the neocortex leading to tool use/manufacture, and language that requires fresh scrutiny. Overall, convergent encephalization indices and cognitive capacities seem to allow capuchins to use their divergent and relatively limited morphological hand apparatus to a high degree of skill.
Data availability statement
All relevant data is contained within the article. The source specimens for the measured data are available at the University of Tocantins, Brazil. Further inquiries can be directed to the corresponding author.
Ethics statement
The animal study was approved by CoEP-UFG 81/2008, with authorization from IBAMA number 15275. The study was conducted in accordance with the local legislation and institutional requirements.
Author contributions
RB: formal analysis, funding acquisition, project administration, and writing–review and editing. EF-F: investigation, validation, visualization, and writing–review and editing. RM: visualization and writing–review and editing. CT: conceptualization, formal analysis, supervision, validation, visualization, and writing–review and editing. MG-M: Writing–review and editing. TA-F: conceptualization, data curation, formal analysis, investigation, methodology, project administration, resources, supervision, validation, visualization, writing–original draft, and writing–review and editing.
Funding
The author(s) declare that financial support was received for the research, authorship, and/or publication of this article. The publication of this research was supported by the Innovation Design Office, Riken, Kobe, Japan.
Acknowledgments
RB thanks the Riken Innovation Design Office for the financial support for the publication of this study; EF, RM, CB, and TA-F thank CNPq, Brazil, for providing scholarships (Scientific Initiation and Productivity in Research) and supporting the research into the postgraduation programs.
Conflict of interest
The authors declare that the research was conducted in the absence of any commercial or financial relationships that could be construed as a potential conflict of interest.
Publisher’s note
All claims expressed in this article are solely those of the authors and do not necessarily represent those of their affiliated organizations, or those of the publisher, the editors, and the reviewers. Any product that may be evaluated in this article, or claim that may be made by its manufacturer, is not guaranteed or endorsed by the publisher.
References
Abreu T., Tavares M. C., Vieira R. B., Rodrigues R., Pissinati A., Aversi-Ferreira T. A. (2021). Comparative anatomy of the encephalon of new world primates with emphasis for the Sapajus sp. PLoS One 16, e0256309. doi:10.1371/journal.pone.0256309
Almécija S., Smaers J., Jungers W. (2015). The evolution of human and ape hand proportions. Nat. Commun. 6, 7717. doi:10.1038/ncomms8717
Aversi-Ferreira R. A., Nishijo H., Aversi-Ferreira T. A (2015) Reexamination of statistical methods for comparative anatomy: examples of its application and comparisons with other parametric and nonparametric statistics. Biomed. Res. Int 2015;:902534. doi:10.1155/2015/902534
Aversi-Ferreira RAGMF, Abreu T., Pfrimer G. A., Silva S. F., Ziermann J. M., Carneiro-e-Silva F. O., et al. (2013) Comparative anatomy of the hind limb vessels of the bearded capuchins (Sapajus libidinosus) with apes, baboons, and Cebus capucinus: with comments on the vessels' role in bipedalism. BioMed Res. Int 2013:737358. doi:10.1155/2013/737358
Aversi-Ferreira RAGMF, Bretas R. V., Maior R. S., Davaasuren M., Paraguassú-Chaves C. A., Nishijo H., et al. (2014a) Morphometric and statistical analysis of the palmaris longus muscle in human and non-human primates. BioMed Res. Int 2014:178906–6. doi:10.1155/2014/178906
Aversi-Ferreira RAGMF, Maior S. R., Aziz A., Ziermann J. M., Nishijo H., Tomaz C., et al. (2014c). Anatomical analysis of thumb opponency movement in the capuchin monkey (Sapajus sp). PLos One 9 (2), e87288. doi:10.1371/journal.pone.0087288
Aversi-Ferreira RAGMF, Marin K. A., Silva F. O. C., Aversi-Ferreira T. A. (2011). Comparative anatomy of the thigh nerve of Cebus libidinosus (Rylands et al., 2000). Braz. J. Veterinary Res. 31, 261–266. doi:10.1590/S0100-736X2011000300013
Aversi-Ferreira RAGMF, Vieira S. V., Tomaz C., Aversi-Ferreira T. A. (2014b). Comparative anatomy of the pelvic vessels in the bearded capuchin (Sapajus libidinosus) and baboons, apes and modern humans. Folia Primatol. (Basel) 85 (4), 252–264. doi:10.1159/000366061
Aversi-Ferreira T. A. (2009). Comparative anatomical description of forearm and hand arteries of Cebus libidinosus. Int. J. Morphol. 27 (1), 219–226. doi:10.4067/S0717-95022009000100037
Aversi-Ferreira T. A., Aversi-Ferreira RAGMF, Bretas R. V., Nishimura H., Nishijo H. (2016). Comparative anatomy of the arm muscles of the Japanese monkey (Macaca fuscata) with some comments on locomotor mechanics and behavior. J. Med. Primatol. 45 (4), 165–179. doi:10.1111/jmp.12222
Aversi-Ferreira T. A., Borges K. M., Goncalves-Mendes M. T., Caixeta L. F. (2021a). Gross anatomy of the longitudinal fascicle of Sapajus sp. PLoS One 16 (6), e0252178. doi:10.1371/journal.pone.0252178
Aversi-Ferreira T. A., Diogo R., Potau J. M., Bello G., Pastor J. F., Aziz M. A. (2010). ComparativeAnatomical Study of the Forearm Extensor Muscles of Cebus libidinosus (Rylands et al., 2000; Primates, Cebidae), Modern Humans, and Other Primates, With Comments on Primate Evolution, Phylogeny, and Manipulatory Behavior. Anatomical Rec. 293 (12), 2056–2070. doi:10.1002/ar.21275
Aversi-Ferreira T. A., Freitas-Ferreira E., Aversi-Ferreira H. (2021b). Differences among the forelimb arteries in groups of primates and a mathematical model explanation. J. Med. Primatol. 50 (1), 21–28. doi:10.1111/jmp.12498
Aversi-Ferreira T. A., Freitas-Ferreira E., Aversi-Ferreira H., Cordeiro-de-Oliveira K., Lopes-de-Freitas G., Trevisan K., et al. (2020). Comparative gross anatomy of the forelimb arteries of the Japanese monkey (Macaca fuscata) and a comparative pattern of forelimb arterial distribution in primates. BioMed Res. Int. 2020, 8635917–8636016. doi:10.1155/2020/8635917
Aversi-Ferreira T. A., Lima-e-Silva M. S., Pereira-de-Paula J., Gouvea-e-Silva L. F., Penha-Silva N. (2005). Anatomia comparativa dos nervos do braço de Cebus apella. Descrição do músculo dorso epitroclear. Acta Sci. Biol. Sci. 27 (3), 291–296. doi:10.4025/actascibiolsci.v27i3.1338
Aversi-Ferreira T. A., Maior R. S., Carneiro-E-Silva F. O., Aversi-Ferreira RAGMF, Tavares M. C., Nishijo H., et al. (2011). Comparative anatomical analyses of the forearm muscles of Cebus libidinosus (Rylands et al. 2000): manipulatory behavior and tool use. PloS One 6 (7), e22165. doi:10.1371/journal.pone.0022165
Aversi-Ferreira T. A., Pereira-de-Paula J., Lima-e-Silva M. S., Prado Y. C. L., Silva Z. (2007b). Estudo anatômico das artérias do ombro de Cebus libidinosus (RYLANDS, 2000; PRIMATES – CEBIDAE). Ciência Anim. Bras. 8 (2), 273–284. doi:10.5216/cab.v8i2.1352
Aversi-Ferreira T. A., Pereira-de-Paula J., Lima-e-Silva M. S., Silva Z. (2007a). Anatomy of the arteries of the arm of Cebus libidinosus (Rylands et al., 2000) monkeys. Acta Sci. Biol. Sci. 29 (3), 247–254. doi:10.4025/actascibiolsci.v29i3.473
Aversi-Ferreira T. A., Pereira-de-Paula J., Prado Y. C. L., Lima-e-Silva M. S., Mata J. R. (2007c). Anatomy of the shoulder and arm muscles of Cebus libidinosus. Braz. J. Morphol. Sci. 24 (2), 63–74.
Aversi-Ferreira T. A., Vieira L. G., Pires R. M., Silva Z., Penha-Silva N. (2006). Estudo anatômico dos músculos flexores superficiais do antebraço no macaco Cebus apella. Biosci. J. 22 (1), 139–144.
Boesch C., Head J., Robbins M. M. (2009). Complex tool sets for honey extraction among chimpanzees in Loango National Park, Gabon. J. Hum. Evol. 56 (6), 560–569. doi:10.1016/j.jhevol.2009.04.001
Borges K. C., Nishijo H., Aversi-Ferreira T. A., Ferreira J. R., Caixeta L. F (2015) Anatomical study of intrahemispheric association fibers in the brains of capuchin monkeys (Sapajus sp.). BioMed Res. Int 2015, 648128. doi:10.1155/2015/648128
Bortoff G. A., Strick P. L. (1993). Corticospinal terminations in two new-world primates: further evidence that corticomotoneuronal connections provide part of the neural substrate for manual dexterity. J. Neurosci. 13 (12), 5105–5118. doi:10.1523/JNEUROSCI.13-12-05105.1993
Canale G. R., Guidorizzi C. E., Kierulff M. C., Gatto C. A. (2009). First record of tool use by wild populations of the yellow-breasted capuchin monkey (Cebus xanthosternos) and new records for the bearded capuchin (Cebus libidinosus). Am. J. Primatology 71 (5), 366–372. doi:10.1002/ajp.20648
Day M. H., Napier J. R. (1963). The functional significance of the deep head of flexor pollicis brevis in primates. Folia Primatol. 1 (2), 122–134. doi:10.1159/000165787
Demes B. (2011). Three-dimensional kinematics of capuchin monkey bipedalism. Am. J. Phys. Anthropol. 145 (1), 147–155. doi:10.1002/ajpa.21484
Fragaszy D. M., Izar P., Visalberghi E., Ottoni E. B., De Oliveira M. G. (2004). Wild capuchin monkeys (Cebus libidinosus) use anvils and stone pounding tools. Am. J. Primatology 64 (4), 359–366. doi:10.1002/ajp.20085
Fragaszy D. M., Visalberghi E., Fedigan L. M. (2004). The Complete Capuchin: the biology of the genus Cebus. Cambridge: Cambridge University Press.
Gibbs S. (1999). Comparative soft tissue morphology of the extant hominoidea, including man. Liverpool: University of Liverpool Press.
Goodman M., Porter C. A., Czelusniak J., Page S. L., Schneider H., Shoshani J., et al. (1998). Toward a phylogenetic classification of primates based on DNA evidence complemented by fossil evidence. Mol. Phylogenetics Evol. 9 (3), 585–598. doi:10.1006/mpev.1998.0495
Hakeem A., Sandoval R. G., Jones M., Allman J. (1996). “Brain and life span in primates,” in Handbook of the psychology of aging Editors J. E. Birren, and K. W. Schaie ed 4th. (San Diego: Academic Press), 78–104.
Judge D. S., Carey J. R. (2000). Postreproductive life predicted by primate patterns. Journals Gerontology Ser. a-Biological Sci. Med. Sci. 55 (4), B201–B209. doi:10.1093/gerona/55.4.b201
Lacreuse A., Fragaszy D. (1997). Manual exploratory procedures and asymmetries for a haptic search task: a comparison between capuchin monkeys (Cebus apella) and humans. Laterality 2 (3-4), 247–266. doi:10.1080/713754275
Mannu M., Ottoni E. B. (2009). The enhanced tool-kit of two groups of wild bearded capuchin monkeys in the Caatinga: tool making, associative use, and secondary tools. Am. J. Primatology 71 (3), 242–251. doi:10.1002/ajp.20642
Marin K. A., Carneiro-e-Silva F. O., Carvalho A. V., Nascimento G. N. L., Prado Y. C. L., Aversi-Ferreira T. A. (2009). Anatomy of the nervous of forearm and hand of Cebus libidinosus (Rylands, 2000). Int. J. Morphol. 27 (3), 635–642. doi:10.4067/S0717-95022009000300003
Meulman E. J. M., Sanz C. M., Visalberghi E., van Schaik C. P. (2012). The role of terrestriality in promoting primate technology. Evol. Anthropol. 21 (2), 58–68. doi:10.1002/evan.21304
Napier J. R. (1952). The attachments and function of the abductor pollicis brevis. J. Anat. 86 (4), 335–341.
Napier J. R. (1955). The form and function of the carpo-metacarpal joint of the thumb. J. Anat. 89 (3), 362–369.
Napier J. R. (1961).Prehensibility and opposability in the hands of primates. Symposia of the Zoological Society of London, 5, 115–132.
Ogihara N., Kunai T., Masato N. (2005). Muscle dimensions in the chimpanzee hand. Primates 46 (4), 275–280. doi:10.1007/s10329-005-0136-x
Panger M. A. (1998). Object-use in free-ranging white-faced capuchins (Cebus capucinus) in Costa Rica. Am. J. Phys. Anthropol. 106 (3), 311–321. doi:10.1002/(SICI)1096-8644
Pereira-de-Paula J., Prado Y. C. L., Tomaz C., Aversi-Ferreira T. A. (2010). Anatomical study of the main sulci and gyri of the Cebus libidinosus brain (rylands). Neurobiologia 73 (2), 65–78.
Reader S. M., Hager Y., Laland K. N. (2011). The evolution of primate general and cultural intelligence. Philosofical Trans. R. Soc. 366, 1017–1027. doi:10.1098/rstb.2010.0342
Rilling J. K., Insel T. R. (1999). The primate neocortex in comparative perspective using magnetic resonance imaging. J. Hum. Evol. 37, 191–223. doi:10.1006/jhev.1999.0313
Sanz C. M., Morgan D. B. (2009). Flexible and persistent tool-using strategies in honey-gathering by wild chimpanzees. Int. J. Primatology 30, 411–427. doi:10.1007/s10764-009-9350-5
Souto A., Bione C. B. C., Bastos M., Bezerra B. M., Fragaszy D., Schiel N. (2011). Critically endangered blonde capuchins fish for termites and use new techniques to accomplish the task. Biol. Lett. 7, 532–535. doi:10.1098/rsbl.2011.0034
Spagnoletti N., Izar P., Visalberghi E. (2009). Tool use and terrestriality in wild bearded capuchin monkey (Cebus libidinosus). Folia Primatol. 80, 142.
Spinozzi G., Truppa V., Lagana T. (2004). Grasping behavior in tufted capuchin monkeys (Cebus apella): grip types and manual laterality for picking up a small food item. Am. J. Phys. Anthropol. 125, 30–41. doi:10.1002/ajpa.10362
Standring S. (2008). Gray’s anatomy: the anatomical basis of clinical practice. London: Churchill Livingstone.
Stephan H., Andy O. J. (1969). Quantitative comparative neuroanatomy of primates - an attempt at a phylogenetic interpretation. Ann. N. Y. Acad. Sci. 167, 370–387. doi:10.1111/j.1749-6632.1969.tb20457.x
Sugiyama Y. (1997) Social tradition and the use of tool-composites by wild chimpanzees. Evol. Anthropol. 6:23–27. doi:10.1002/(sici)1520-6505(1997)6:1<23::aid-evan7>3.0.co;2-x
Swindler D. R., Wood C. D. (1973). An atlas of primates gross anatomy. Washington: University of Washington Press.
Tocheri M. W., Orr C. M., Jacofsky M. C., Marzke M. W. (2008). The evolutionary history of the hominin hand since the last common ancestor of Pan and Homo. J. Anat. 212, 544–562. doi:10.1111/j.1469-7580.2008.00865.x
van Schaik C. P., Deaner R. O., Merrill M. Y. (1999). The conditions for tool use in primates: implications for the evolution of material culture. J. Hum. Evol. 36, 719–741. doi:10.1006/jhev.1999.0304
Keywords: Sapajus, primate hand, morphology of recent groups, biomathematics, mathematical models, evolution, primates
Citation: Bretas R, Freitas-Ferreira E, Souto Maior R, Tomaz C, Gonçalves-Mendes MT and Aversi-Ferreira TA (2024) Comparative anatomy of the Sapajus sp. (bearded capuchin) hand with comments on tool use in a parallel evolution with the hominid pathway. Front. Physiol. 15:1292035. doi: 10.3389/fphys.2024.1292035
Received: 10 September 2023; Accepted: 05 January 2024;
Published: 09 February 2024.
Edited by:
Andrea Maria Chiariello, University of Naples Federico II, ItalyReviewed by:
Emmanuel Paul Gilissen, Royal Museum for Central Africa, BelgiumKevin Flaherty, University of Missouri, United States
Copyright © 2024 Bretas, Freitas-Ferreira, Souto Maior, Tomaz, Gonçalves-Mendes and Aversi-Ferreira. This is an open-access article distributed under the terms of the Creative Commons Attribution License (CC BY). The use, distribution or reproduction in other forums is permitted, provided the original author(s) and the copyright owner(s) are credited and that the original publication in this journal is cited, in accordance with accepted academic practice. No use, distribution or reproduction is permitted which does not comply with these terms.
*Correspondence: Tales Alexandre Aversi-Ferreira, aversiferreira@gmail.com