- Division of Gastroenterology, Department of Pediatrics, McGovern Medical School, The University of Texas Health Science Center at Houston, Houston, TX, United States
The lack of a functional Foxp3 transcription factor and regulatory T (Treg) cells causes lethal, CD4+ T cell-driven autoimmune diseases in scurfy (SF) mice and humans. Recent studies have shown that adenosine A2A receptor activation limits inflammation and tissue damage, thereby playing an anti-inflammatory role. However, the role of the adenosine A2A receptor in the development of disease in SF mice remains unclear. Using a genetic approach, we found that adenosine A2A receptor deletion in SF mice (SF) does not affect early life events, the development of a lymphoproliferative disorder, or hyper-production of pro-inflammatory cytokines seen in the Treg-deficiency state. As shown previously, Lactobacillus reuteri DSM 17938 treatment prolonged survival and reduced multiorgan inflammation in SF mice. In marked contrast, A2A receptor deletion completely blocked these beneficial effects of L. reuteri in SF mice. Altogether, these results suggest that although absence of the adenosine A2A receptor does not affect the development of disease in SF mice, it plays a critical role in the immunomodulation by L. reuteri in Treg-deficiency disease. The adenosine A2A receptor and its activation may have a role in treating other Treg dysfunction-mediated autoimmune diseases.
Introduction
Foxp3+ regulatory T (Treg) cells play a pivotal role in the phenomenon of self-tolerance. In humans, Foxp3 mutations result in immunodysregulation, polyendocrinopathy, and enteropathy, with X-linked inheritance (called IPEX syndrome). Newborn boys with IPEX syndrome have severe enteropathy, eczema, type I diabetes, thyroiditis, hemolytic anemia, and thrombocytopenia; and they die within the first years of life if left untreated (1, 2). In the mouse model, Foxp3-deficient scurfy (SF) mice develop a lethal autoimmune disease which closely resembles the IPEX syndrome (3, 4). SF mice develop early-onset dermatitis, progressive multiorgan inflammation, and early death within the first month of life due to a lymphoproliferative syndrome. This lethal lymphoproliferative syndrome is predominately mediated by CD4+ T cells in humans and mice (5, 6). Consequently, the SF mouse is a valuable model for studying novel therapies for human IPEX syndrome and other autoimmune diseases associated with Treg deficiency. These include IPEX-like syndromes induced by mutations or deficiency in Itchy E3 ubiquitin protein ligase (ITCH), the α-chain of the IL-2 receptor (CD25), signal transducer and activator of transcription 5b, STAT1, or cytotoxic T-lymphocyte-associated protein 4 (7, 8).
High levels of the adenosine A2A receptor are found in the brain, thymus, and spleen, as well as in circulating platelets and leukocytes (9). On the cell membrane of murine T lymphocytes, the adenosine A2A receptor is highly expressed and is increased by T-cell receptor (TCR) stimulation (10, 11). In humans, the A2A receptor is more highly expressed in CD4+ compared to CD8+ T cells (12). Moreover, numerous studies have highlighted the anti-inflammatory role of the adenosine A2A receptor (13, 14). There have been observations of anti-inflammatory effects of A2A receptor agonists in vivo and, conversely, enhanced inflammation in A2A receptor knockout mice (14). However, the function of adenosine A2A receptor in the development and control of autoimmune diseases remains unclear.
Recently, probiotics have emerged as relatively safe and inexpensive treatments for a number of gastrointestinal conditions. Lactobacillus reuteri strain DSM 17938 (L. reuteri) is a probiotic originally isolated from a Peruvian mother’s breast milk (15). This probiotic has been shown to prevent necrotizing enterocolitis (NEC) in newborn animals (16, 17) by inhibiting the toll-like receptor 4-mediated NF-κB pathway, facilitating the induction of immune-modulating Foxp3+ Tregs, and lowering the number of pro-inflammatory effector-memory T-cells in the intestinal mucosa. In humans, L. reuteri has been shown to reduce the severity of acute infant diarrhea (18–20), to prevent NEC in premature infants (21–23), and to decrease crying time in infants with colic (24, 25).
In addition, our recent studies demonstrated that L. reuteri significantly prolongs the survival rate of the SF mouse (from less than 30 days to greater than 4 months of age) by suppression of inflammatory T cells (mainly TH1 and TH2) extensively activated in multiple organs of SF mice (7). Mechanistically, L. reuteri modulates the abnormal microbial communities associated with these diseases, stimulating the production of bioactive metabolites involved in immune modulation. We observed that inosine, a downstream metabolite of adenosine, was decreased in the plasma of SF mice compared to wild-type (WT) mice, but was increased by oral administration of L. reuteri to SF mice. Oral administration of inosine by itself prolonged the survival and decreased autoimmunity of SF mice. Inosine was found to be a critical effector molecule of L. reuteri treatment, altering TH1/TH2 cell differentiation by activating A2A receptors, predominately expressed on T cells. Blocking A2A receptors by an A2A antagonist reversed the anti-inflammatory effects of both inosine and L. reuteri, indicating that A2A receptor appears to play a critical role in the beneficial effects of L. reuteri in the SF model (7).
In this study, we produced SF mice with genetically deleted adenosine A2A receptor (SF) to conclusively provide evidence of a central role of A2A receptor in the actions of L. reuteri. We demonstrate that A2A receptor gene deletion in SF mice did not accentuate the development of disease, but prevented the inhibitory effects of L. reuteri on autoimmunity. Our study highlights the A2A receptor as a key mediator of the immunomodulatory mechanism of this probiotic.
Materials and Methods
Animals
Wild-type C57BL/6, heterozygous B6.Cg-Foxp3sf/J and adora2atm1Jfc/J mice were purchased from Jackson Laboratories and allowed to acclimatize for 2 weeks before experimentation. SF mice were bred with adora2atm1Jfc/J mice to generate adenosine A2A receptor-deficient SF mice ( SF mice, SF). All males were either SF/SF double knockouts, the experimental group, or WT/ littermates, used as controls. All mice were housed in the animal facility at UT Health Science Center at Houston. This study was carried out in accordance with the recommendations of the Guide for the Care and Use of Laboratory Animals (NIH) and The Institutional Animal Care and Use Committee (IACUC). The protocol was approved by the IACUC (protocol numbers: AWC-14-056 and AWC-17-0045).
L. reuteri Treatment of SF Mice
Lactobacillus reuteri DSM17938 (L. reuteri), originally isolated from human breast milk, was provided by BioGaia AB (Stockholm, Sweden) and prepared as described previously (7). Each mouse was given either De Man, Rogosa, and Sharpe agar (MRS) media as a control or L. reuteri (SF + LR or SF + LR) which was given by daily gavage in cultured media (107 CFU/day), starting from 8 to 20 days of age for tissue analysis or to infinity for survival.
Histopathology
All tissues of WT, SF, SF + LR, , SF, and SF + LR mice were fixed and stained with hematoxylin and eosin (H&E) for histological evaluation by the Cellular and Molecular Morphology Core Lab (The Texas Medical Center Digestive Diseases Center, Houston, TX, USA). The area of lymphocyte infiltration in liver and lung was assessed in a blinded fashion using Image J morphometry software (NIH, USA).
In vitro Tissue Preparation and Stimulation for Flow Cytometry Analysis
Single-cell suspensions from the spleen were prepared by gently fragmenting and filtering the tissues through 40-μm cell strainers (BD Bioscience) into MACS buffer (1× PBS, 0.5% bovine BSA, and 2 mM EDTA). For in vitro stimulation of splenocytes, cells were stimulated with 50 ng/mL of phorbol 12-myristate 13-acetate (PMA) and 1 μg/mL of ionomycin in the presence of brefeldin A (5 μ/mL) for 4 h to analyze IFN-γ-producing (TH1) and IL-4-producing (TH2) CD4+ T cells by flow cytometry.
Staining Cells for Flow Cytometry Analysis
For evaluation of TH1 and TH2 cells, cells were surface stained by fluorescein-labeled CD4. Intracellular staining was performed with a fixation/permeabilization kit, according to the manufacturer’s protocol (eBioscience) and stained with IFN-γ and IL-4 for TH1 and TH2 cells, respectively. The data from all samples were acquired on BD FACSCalibur and analyzed using FlowJo software (TreeStar, Inc.).
Plasma Cytokine Assays
Plasma cytokine levels of IFN-γ, IL-1β, IL-2, IL-4, IL-5, IL-10, and IL-12p70 were assessed using a mouse multi-spot pro-inflammatory panel kit, and signals were detected by Imager 2400 from Meso Scale Discovery, according to the manufacturer’s protocol.
Statistical Analysis
Data are presented as mean ± SEM. Statistical significance was determined using one-way ANOVA corrected for multiple comparisons with Tukey and Dunnett’s posttests. The statistical analysis was performed using Prism version 4.0 (GraphPad Software). A p-value <0.05 was considered to indicate statistical significance.
Results
Adenosine A2A Receptor Deletion Does Not Affect Early Death in SF Mice
To determine the role of the adenosine A2A receptor in the pathogenesis of autoimmunity in the SF mouse, we bred female (Foxp3sf/+) mice with adora2a gene knockout mice. The male adenosine A2A receptor-deficient SF (SF) mice died between 21 and 25 days of age (Figure 1A). Our data show that A2A receptor deletion does not enhance or reverse the effect of the lethal autoimmune disease as it relates to lifespan in the SF mouse.
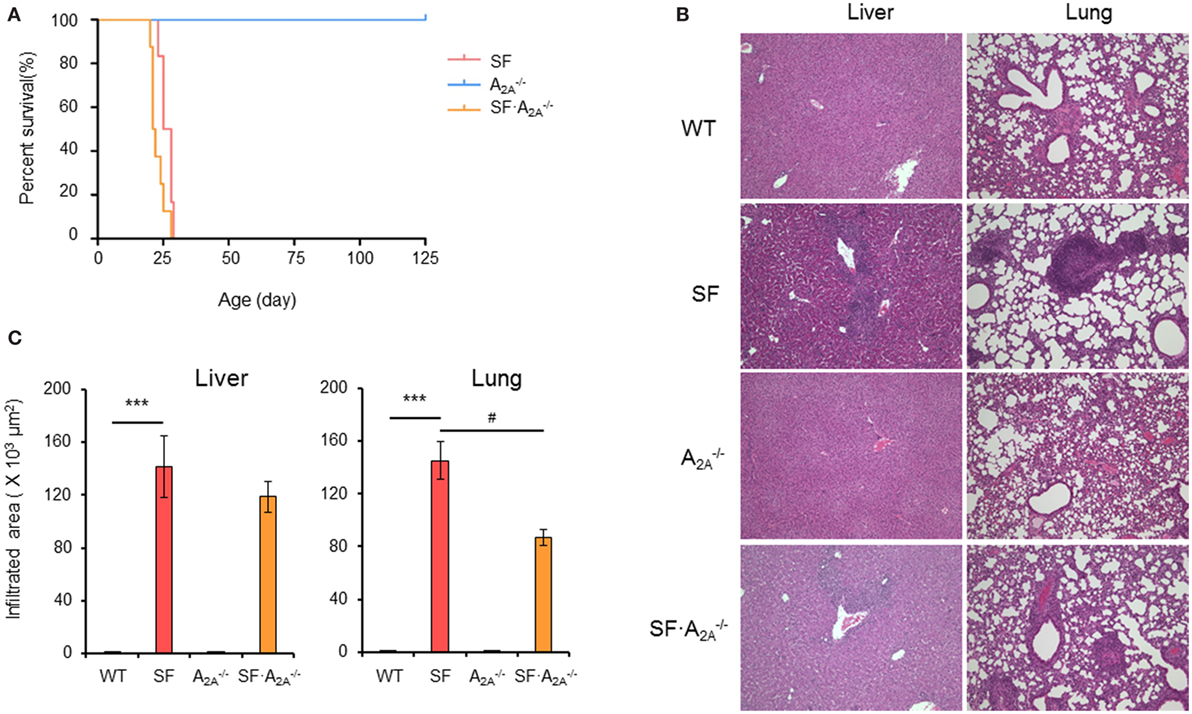
Figure 1. Effect of adenosine A2A receptor deletion on the development of diseases in scurfy (SF) mice. (A) Survival curves of , SF, and SF mice (n = 6–9). (B) H&E staining of representative sections of liver and lung of wild-type (WT), SF, , and SF mice (n = 6–9). (C) Quantitation of inflammatory infiltrates in liver and lung of WT, SF, , and SF mice (n = 6–9). Data are presented as mean ± SEM. ***p < 0.001. SF vs. WT. #p < 0.05. SF vs. SF.
Adenosine A2A Receptor Deletion Regulates Organ-Specific Inflammation in SF Mice
Scurfy mice develop severe inflammation in several tissues, including liver, lung, ear, tail, intestine, and colon (26). To examine whether adenosine A2A receptor deletion alters the autoimmune damage in these tissues, we measured the area of inflammatory cell infiltration in H&E-stained tissues sections from WT, SF, , and SF mice at the 20 days of age. There were no inflammatory infiltrates in the liver, lung, ear, tail, and intestine in mice (Figures 1B,C; Figure S1 in Supplementary Material). Indeed, the area of inflammatory cell infiltration in most organs studied (liver, ear, tail, and intestine) in SF mice was similar to the inflammatory cell infiltrate in SF mice (Figures 1B,C; Figure S1 in Supplementary Material). However, the inflammatory cell infiltration of the lung was slightly reduced in SF mice compared to SF mice. These results demonstrate that the A2A receptor deletion does not have a major impact on inflammation in SF mice.
Adenosine A2A Receptor Deletion Does Not Reduce TH1/TH2 Cells in SF Mice
The lethal lymphoproliferative syndrome in SF mice is predominantly caused by CD4+ T cell-induced pathology (27, 28). To evaluate the effect of A2A receptor deletion on TH1/TH2 cells in SF mice, we measured the percentage of IFN-γ-producing CD4+ T (TH1) cells and IL-4-producing CD4+ T (TH2) cells in the spleen of WT, SF, , and SF mice. A2A receptor deletion did not change the frequency of TH1 or TH2 cells in WT or SF mice (WT or SF) mice, respectively (Figure 2). Our findings suggest that A2A receptor deletion does not inhibit TH1/TH2 cell proliferation in SF mice.
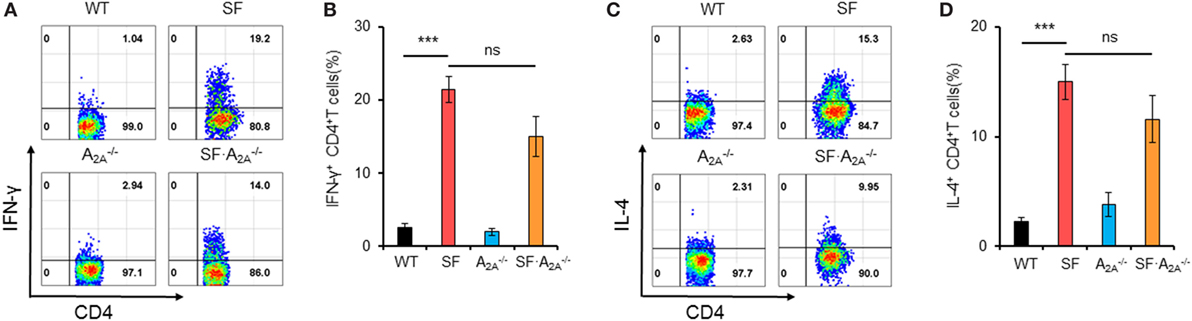
Figure 2. Effect of adenosine A2A receptor deletion on TH1/TH2 cells in spleen of scurfy (SF) mice. (A) Representative FACS plots of IFN-γ-producing CD4+ T (TH1) cells in spleen of wild-type (WT), SF, , and SF mice. (B) Percentage of TH1 cells in spleen of WT, SF, , and SF mice (n = 6–9). (C) Representative FACS plots of IL-4-producing CD4+ T (TH2) cells in spleen of WT, SF, , and SF mice. (D) Percentage of TH2 cells in spleen of WT, SF, , and SF mice (n = 6–9). Data are presented as mean ± SEM. ***p < 0.001. SF vs. WT. ns, non-significance.
Adenosine A2A Receptor Deletion Alters the Majority of Pro-inflammatory Cytokines in SF Mice
After TCR stimulation, CD4+ T cells from SF mice produce high levels of cytokines, including IFN-γ, IL-2, IL-4, IL-10, and TNF-α (29, 30). To examine whether these pro-inflammatory cytokines reached higher levels in SF mice compared to SF mice, we measured the concentration of pro-inflammatory cytokines in plasma (Figure 3; Figure S2 in Supplementary Material). Our results demonstrated that the levels of IFN-γ, IL-2, IL-4, IL-5, and IL-10 were increased in SF mice compared to WT mice. Conversely, the levels of IL-1β and IL-12p70 were not increased in SF mice compared to WT mice. However, A2A receptor deletion increased the levels of pro-inflammatory IL-1β and anti-inflammatory cytokine IL-10 in SF mice compared to SF mice. Together, our results show that the majority of pro-inflammatory cytokines contribute to the development of disease in SF mice.
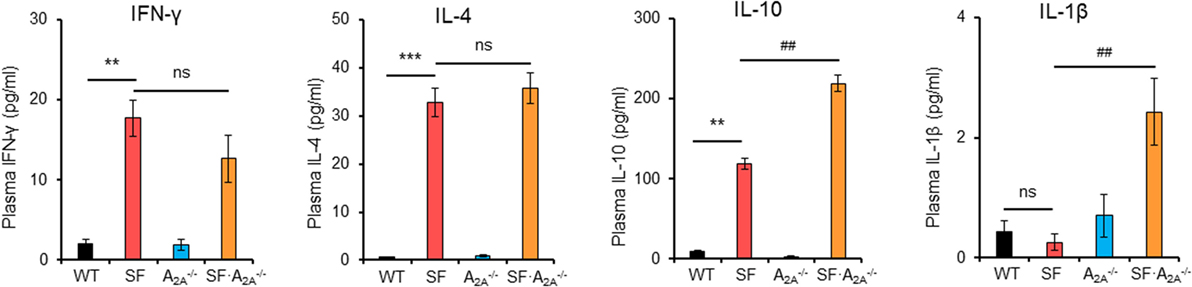
Figure 3. Effect of adenosine A2A receptor deletion on pro-inflammatory cytokines in scurfy (SF) mice. Plasma levels of IFN-γ, IL-1β, IL-4, and IL-10 in wild-type (WT), SF, , and SF mice were quantified by a mouse multi-spot pro-inflammatory panel kit (n = 6–9). Data are presented as mean ± SEM. **p < 0.01, ***p < 0.001. SF vs. WT. #p < 0.05, ##p < 0.01. SF vs. SF. ns, non-significance.
Adenosine A2A Receptor Deletion Reverses the Effect of L. reuteri on Lifespan in SF Mice
Previous studies have suggested that L. reuteri increases survival in SF mice by restoring plasma levels of the nucleotide inosine, which is an adenosine A2A receptor agonist (7), which represents a novel mechanism of action of probiotics. However, the effect of targeted genetic deletion of A2A receptor on the beneficial effects of L. reuteri in SF mice is unknown. To examine this effect, we fed SF with L. reuteri (SF + LR) and SF mice with L. reuteri (SF + LR). The median lifespan of the SF mouse was significantly increased by LR feeding (SF + LR mice), from 26.5 to 92 days (p < 0.001). However, the median lifespan of SF mice with L. reuteri treatment (SF + LR) was 22.5 days (Figure 4A). These data demonstrate that A2A receptor plays a critical role in the effect of L. reuteri to prolong the lifespan of the SF mouse.
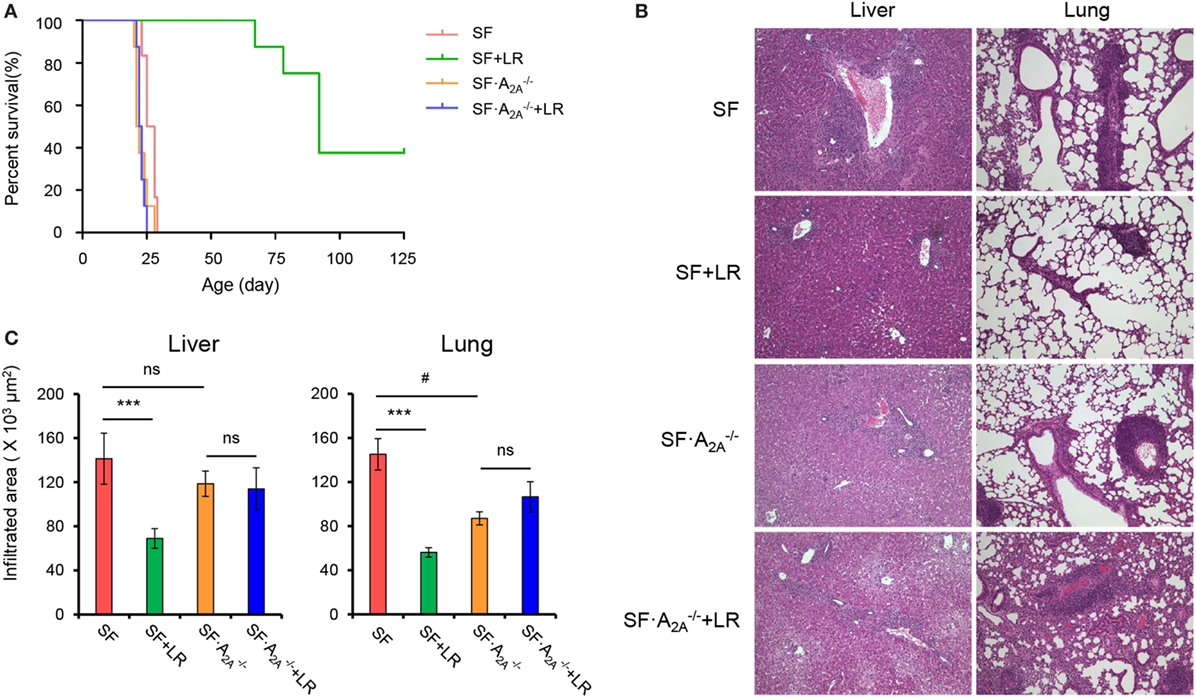
Figure 4. Adenosine A2A receptor deletion blocks effects of Lactobacillus reuteri on scurfy (SF) mice. (A) Survival curves of SF, SF + LR, SF, and SF + LR mice (n = 6–9). (B) H&E staining of representative sections of liver and lung of SF, SF + LR, SF, and SF + LR mice (n = 6–9). (C) Quantitation of inflammatory infiltrates in liver and lung of SF, SF + LR, SF, and SF + LR mice (n = 6–9). Data are presented as mean ± SEM. ***p < 0.001. SF + LR vs. SF. ns, non-significance. #p < 0.05.
Adenosine A2A Receptor Deletion Negates the Effect of L. reuteri on Inflammation in SF Mice
We next asked whether A2A receptor deletion could inhibit the beneficial effect of L. reuteri on multiorgan inflammation in living SF mice. Therefore, we fed SF and SF mice with a daily dose of L. reuteri, starting from 8 to 20 days. H&E-stained tissue sections from SF, SF + LR, SF, and SF + LR groups were scored. Inflammatory cell infiltration of liver and lung was reduced in SF + LR mice compared to SF mice. However, this infiltration was not reduced in SF + LR mice compared to SF mice (Figures 4B,C). These results demonstrate that A2A receptor activation contributes to the inhibition by L. reuteri of inflammation in the SF mouse.
Adenosine A2A Receptor Deletion Inhibits L. reuteri-Mediated Reduction of TH1/TH2 Splenocytes in SF Mice
Our studies have shown that L. reuteri reduces TH1/TH2 cells in SF mice (7). To explore whether genetic deletion of A2A receptor contributes to the inhibition of L. reuteri of TH1/TH2 cell differentiation in SF mice, we measured the frequency of TH1/TH2 cells in the spleen from SF, SF + LR, SF, and SF + LR mice (Figure 5). L. reuteri-treated SF mice had reduced TH1/TH2 cells when compared to SF mice at 20 days of age, consistent with our previous studies (7). Interestingly, L. reuteri treatment did not reduce the percentage of TH1/TH2 cells in SF mice, demonstrating that the activated A2A receptor plays an important role in L. reuteri-mediated immunoregulation in SF mice.
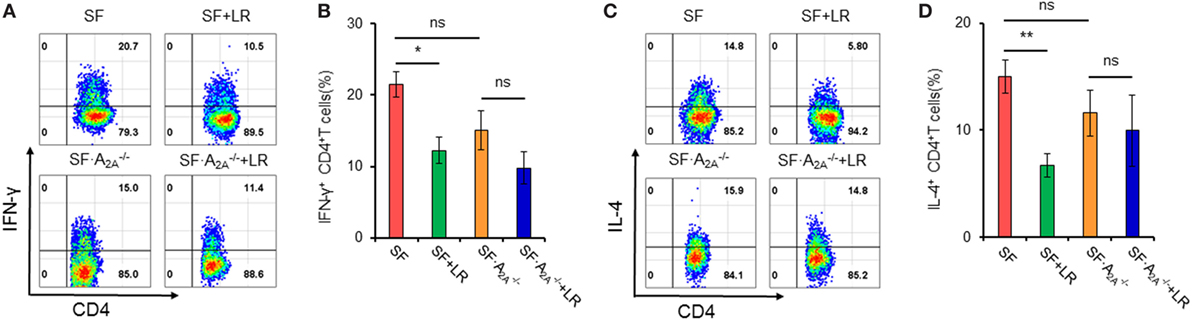
Figure 5. Effect of Lactobacillus reuteri on TH1/TH2 cells in spleen of scurfy (SF) and SF mice. (A) Representative FACS plots of IFN-γ-producing CD4+ T (TH1) cells in spleen of SF, SF + LR, SF, and SF + LR mice. (B) Percentage of TH1 cells in spleen of SF, SF + LR, SF, and SF + LR mice (n = 6–9). (C) Representative FACS plots of IL-4-producing CD4+ T (TH2) cells in spleen of SF, SF + LR, SF, and SF + LR mice. (D) Percentage of TH2 cells in spleen of SF, SF + LR, SF, and SF + LR mice (n = 6–9). Data are presented as mean ± SEM. *p < 0.05, **p < 0.01. SF + LR vs. SF. ns, non-significance.
Adenosine A2A Receptor Deletion Reverses the Effect of L. reuteri on Pro-inflammatory Cytokines in SF Mice
To test whether cytokine production regulated by L. reuteri treatment depends on the A2A receptor in SF mice, we examined plasma cytokines from SF, SF + LR, SF, and SF + LR mice (Figure 6; Figure S3 in Supplementary Material). L. reuteri-treated SF mice had reduced levels of IFN-γ and IL-4 and increased the levels of IL-12p70, but they had no changes in the levels of IL-1β, IL-2, IL-5, and IL-10, when compared to SF mice. Notably, A2A receptor deletion reversed the effects of L. reuteri on IFN-γ, IL-4, and IL-12p70. These findings further substantiate that A2A receptor activation contributes to the inhibitory effects of L. reuteri on inflammation in the SF mouse.
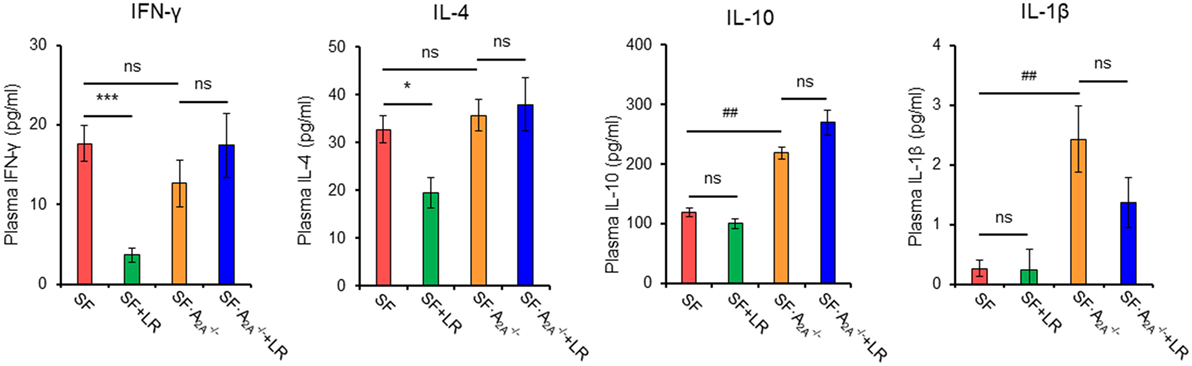
Figure 6. Effect of Lactobacillus reuteri on pro-inflammatory cytokines in scurfy (SF) and SF mice. Plasma levels of IFN-γ, IL-4, IL-1β, and IL-10 in SF, SF + LR, SF, and SF + LR mice were quantified by a mouse multi-spot pro-inflammatory panel kit (n = 6–9). Data are presented as mean ± SEM. *p < 0.05, ***p < 0.001. SF + LR vs. SF. ##p < 0.01. SF vs. SF. ns, non-significance.
Discussion
This study demonstrated a central role of the adenosine A2A receptor in mediating the protection of probiotic L. reuteri against inflammation in the Treg-deficient SF mouse (a model of human IPEX syndrome), evidenced by the observation that SF mice with an A2A receptor deletion continued to have systemic inflammation which was unresponsive to L. reuteri treatment.
It is well known that the lethal lymphoproliferative syndrome characterizing SF mice is predominately mediated by TH1 and TH2 cell-induced pathology (27, 28). The key to Treg suppression of T effector cells (TH1/TH2/TH17) is an interaction between adenosine produced by Tregs (mediated by a CD39–CD73 pathway) and the A2A receptor expressed on nearby T effector cells (31). Lymphocytes predominately express A2A receptors (10–12, 32, 33). However, during Treg deficiency in SF mice or human IPEX syndrome, TH1 and TH2 cells lose their regulation by adenosine A2A-mediated signaling, resulting in TH1 and TH2 cell-induced pathology. Studies by Csoka et al. showed that an agonist of A2A receptors inhibited the proliferation and effector functions of CD4+ T cells isolated from WT mice but failed to block these of cells obtained from A2A knockout mice (33), indicating that the activated adenosine A2A receptor plays a critical role in the suppression of TH1 and TH2 cells.
Our previous study demonstrated that Treg deficiency induces gut microbial dysbiosis dynamically over the first 22 days of life, an effect which could be reprogrammed by oral administration of L. reuteri. L. reuteri suppressed TH1 and TH2 cells in SF mice, as evidenced by lower circulating levels of IFN-γ (TH1) and IL-4 (TH2) and reduced numbers of IFN-γ and IL-4-expressing lymphocytes in spleen and mesenteric lymph nodes of SF mice. Metabolites produced by L. reuteri or L. reuteri-modulated bacteria are known to promote or suppress immune cell function (34–36). We discovered that the purine metabolite inosine, a metabolite of adenosine, is severely decreased in SF mice, while increased after oral administration of L. reuteri (7). Inosine has been proved to be a functional agonist of the A2A receptor which has an anti-inflammatory effect (37–43). Our previous experiments by using adenosine receptor knockout mice to study the suppression of inosine on naïve CD4+ T cell differentiation into TH1 and TH2 in vitro strongly suggested that the effects of inosine are dependent on the A2A receptor on T cells (7). In addition, an in vivo study showed that an A2A receptor antagonist blocks the anti-inflammatory effects of both inosine and (L. reuteri DSM 17938) on TH1 and TH2 suppression and multiorgan lymphocyte infiltration in SF mice (7). In summary, the A2A receptor mediates the beneficial biological effects of L. reuteri and inosine in SF mice. In this study, we further confirmed a critical role of A2A receptor-mediated effects by genetic deletion of A2A in SF mice (SF mice).
Mechanistically, how L. reuteri results in increased serum level of inosine is not fully understood. When we compared L. reuteri cultures to MRS broth (culture media without L. reuteri) after 16 h of anaerobic growth, L. reuteri did not generate significant amounts of purines or inosine in culture. Our previous studies indicated that enterally feeding L. reuteri is associated with recovery of the plasma levels of inosine and hypoxanthine to levels similar to WT, at the same level that inosine levels decreased in the stool of these mice (7). We hypothesized that, most likely, L. reuteri promotes inosine absorption in the intestine by improving overall gut health through multiple mechanisms (for example, by improving villus length) and/or by modulating the gut microbial community. We measured the small intestinal villi in SF mice compare with SF mice after oral feeding L. reuteri and showed that orally feeding L. reuteri improves the length of villi and depth of crypts. Furthermore, an increased expression of equilibrative nucleoside transporter transporters after L. reuteri feeding was found, which could contribute to produce improved absorption. The best method to confirm enhanced absorption would be to orally feed labeled inosine after administration of L. reuteri and quantify the labeled inosine in the circulation. However, the labeling approach for small molecules like inosine is much more difficult than for amino acid or proteins. In the meantime, we could not rule out that in vivo the gut environment could activate the enzymes such as adenosine deaminase (ADA) and 5′-nucleotidase generated by L. reuteri to produce inosine. But it is difficult to distinguish the ADA activity in the intestinal tissue lysates from the activity of L. reuteri or other microbes, because ADA activity is very high in the intestine (44). The direct links between L. reuteri and the metabolites required further exploration.
We also noticed that A2A receptor appears to be expressed in other organs besides lymphocytes (11, 45). In liver, the A2A receptor is expressed in Kupffer cells, hepatocytes, and hepatic stellate cells (46–48). Some studies suggested that the A2A receptor plays a role not only in regulating inflammation but also in maintaining liver function in general (39). Previous studies also revealed that it is more highly expressed in spleen, lymph nodes, liver, and lung than that in the small intestine or adrenal gland, supporting a functional role of this receptor in the regulation of the immune response in peripheral lymphoid tissues (11). It has been reported that A2A receptor activation confers tissue protection in peripheral organs (49, 50). While the mechanism of L. reuteri in regulating inflammation in SF mice clearly involves T cell modulation, we cannot rule out that A2A receptor expression in these organs may also contribute to the beneficial effects of L. reuteri in SF mice. Therefore, A2A receptor expression on both immune cells and other cells and their interaction may determine the overall impact of A2A receptor deletion on beneficial effects of L. reuteri.
The role of the T cell and its expression of A2A modulated by L. reuteri or highly related metabolites such as inosine could be further studied by using a T cell knockout mouse model by adoptive transfer of CD4+ T cells isolated from WT, SF, , or SF with/without L. reuteri or inosine treatment, which is currently under investigation.
In summary, our study demonstrates that adenosine A2A receptor deletion does not inhibit the development of autoimmune disease in the SF mouse. However, adenosine A2A receptor deletion reverses the inhibition of L. reuteri on autoimmunity induced by Treg-deficiency in SF mice. Our results support the concept that activated adenosine A2A receptors are linked to L. reuteri effects in vivo. They also suggest that the activated A2A receptor by L. reuteri or other agonists may represent a useful therapeutic strategy for preventing lethal outcomes in Foxp3 deficency- or dysfunction-induced autoimmune diseases.
Ethics Statement
This study was carried out in accordance with the recommendations of the Guide for the Care and Use of Laboratory Animals (NIH) and The Institutional Animal Care and Use Committee (IACUC). The protocol was approved by the IACUC (Protocol number: AWC-14-056 and AWC-17-0045).
Author Contributions
BH, YL, and JR conceived and designed the experiments. BH, TH, and YL performed all experiments and analyzed the data. BH, YL, DT, and JR wrote the paper and edited the manuscript. All authors read and approved the final manuscript.
Conflict of Interest Statement
The authors declare that the research was conducted in the absence of any commercial or financial relationships that could be construed as a potential conflict of interest.
Acknowledgments
The authors thank Pamela Parsons (Cellular and Morphology Core Lab at Texas Medical Center Digestive Diseases Center) for histological technical assistance and Dr. Eammon Connolly (Biogaia AB, Stockholm, Sweden) for providing Lactobacillus reuteri DSM 17938.
Funding
This work was supported by National Institutes of Health/National Center for Complementary and Integrative Health (NIH/NCCIH) grant R01AT007083, and, in part, by BioGaia AB (Sweden) Investigator Research Grant.
Supplementary Material
The Supplementary Material for this article can be found online at http://www.frontiersin.org/article/10.3389/fimmu.2017.01680/full#supplementary-material.
References
1. Barzaghi F, Passerini L, Bacchetta R. Immune dysregulation, polyendocrinopathy, enteropathy, X-linked syndrome: a paradigm of immunodeficiency with autoimmunity. Front Immunol (2012) 3:211. doi:10.3389/fimmu.2012.00211
2. d’Hennezel E, Bin DK, Torgerson T, Piccirillo CA. The immunogenetics of immune dysregulation, polyendocrinopathy, enteropathy, X linked (IPEX) syndrome. J Med Genet (2012) 49:291–302. doi:10.1136/jmedgenet-2012-100759
3. Bennett CL, Christie J, Ramsdell F, Brunkow ME, Ferguson PJ, Whitesell L, et al. The immune dysregulation, polyendocrinopathy, enteropathy, X-linked syndrome (IPEX) is caused by mutations of FOXP3. Nat Genet (2001) 27:20–1. doi:10.1038/83713
4. Brunkow ME, Jeffery EW, Hjerrild KA, Paeper B, Clark LB, Yasayko SA, et al. Disruption of a new forkhead/winged-helix protein, scurfin, results in the fatal lymphoproliferative disorder of the scurfy mouse. Nat Genet (2001) 27:68–73. doi:10.1038/83784
5. Baris S, Schulze I, Ozen A, Aydiner EK, Altuncu E, Karasu GT, et al. Clinical heterogeneity of immunodysregulation, polyendocrinopathy, enteropathy, X-linked: pulmonary involvement as a non-classical disease manifestation. J Clin Immunol (2014) 34:601–6. doi:10.1007/s10875-014-0059-7
6. Zennaro D, Scala E, Pomponi D, Caprini E, Arcelli D, Gambineri E, et al. Proteomics plus genomics approaches in primary immunodeficiency: the case of immune dysregulation, polyendocrinopathy, enteropathy, X-linked (IPEX) syndrome. Clin Exp Immunol (2012) 167:120–8. doi:10.1111/j.1365-2249.2011.04492.x
7. He B, Hoang TK, Wang T, Ferris M, Taylor CM, Tian X, et al. Resetting microbiota by Lactobacillus reuteri inhibits Treg deficiency-induced autoimmunity via adenosine A2A receptors. J Exp Med (2017) 214:107–23. doi:10.1084/jem.20160961
8. Verbsky JW, Chatila TA. Immune dysregulation, polyendocrinopathy, enteropathy, X-linked (IPEX) and IPEX-related disorders: an evolving web of heritable autoimmune diseases. Curr Opin Pediatr (2013) 25:708–14. doi:10.1097/MOP.0000000000000029
9. Fredholm BB, IJzerman AP, Jacobson KA, Klotz KN, Linden J. International union of pharmacology. XXV. Nomenclature and classification of adenosine receptors. Pharmacol Rev (2001) 53:527–52.
10. Lappas CM, Rieger JM, Linden J. A2A adenosine receptor induction inhibits IFN-gamma production in murine CD4+ T cells. J Immunol (2005) 174:1073–80. doi:10.4049/jimmunol.174.2.1073
11. Lukashev DE, Smith PT, Caldwell CC, Ohta A, Apasov SG, Sitkovsky MV. Analysis of A2A receptor-deficient mice reveals no significant compensatory increases in the expression of A2B, A1, and A3 adenosine receptors in lymphoid organs. Biochem Pharmacol (2003) 65:2081–90. doi:10.1016/S0006-2952(03)00158-8
12. Koshiba M, Rosin DL, Hayashi N, Linden J, Sitkovsky MV. Patterns of A2A extracellular adenosine receptor expression in different functional subsets of human peripheral T cells. Flow cytometry studies with anti-A2A receptor monoclonal antibodies. Mol Pharmacol (1999) 55:614–24.
13. Hasko G, Cronstein BN. Adenosine: an endogenous regulator of innate immunity. Trends Immunol (2004) 25:33–9. doi:10.1016/j.it.2003.11.003
14. Sitkovsky MV. Use of the A(2A) adenosine receptor as a physiological immunosuppressor and to engineer inflammation in vivo. Biochem Pharmacol (2003) 65:493–501. doi:10.1016/S0006-2952(02)01548-4
15. Walter J, Britton RA, Roos S. Host-microbial symbiosis in the vertebrate gastrointestinal tract and the Lactobacillus reuteri paradigm. Proc Natl Acad Sci U S A (2011) 108(Suppl 1):4645–52. doi:10.1073/pnas.1000099107
16. Liu Y, Fatheree NY, Mangalat N, Rhoads JM. Lactobacillus reuteri strains reduce incidence and severity of experimental necrotizing enterocolitis via modulation of TLR4 and NF-kappaB signaling in the intestine. Am J Physiol Gastrointest Liver Physiol (2012) 302:G608–17. doi:10.1152/ajpgi.00266.2011
17. Liu Y, Tran DQ, Fatheree NY, Marc RJ. Lactobacillus reuteri DSM 17938 differentially modulates effector memory T cells and Foxp3+ regulatory T cells in a mouse model of necrotizing enterocolitis. Am J Physiol Gastrointest Liver Physiol (2014) 307:G177–86. doi:10.1152/ajpgi.00038.2014
18. Urbanska M, Gieruszczak-Bialek D, Szajewska H. Systematic review with meta-analysis: Lactobacillus reuteri DSM 17938 for diarrhoeal diseases in children. Aliment Pharmacol Ther (2016) 43:1025–34. doi:10.1111/apt.13590
19. Francavilla R, Lionetti E, Castellaneta S, Ciruzzi F, Indrio F, Masciale A, et al. Randomised clinical trial: Lactobacillus reuteri DSM 17938 vs. placebo in children with acute diarrhoea – a double-blind study. Aliment Pharmacol Ther (2012) 36:363–9. doi:10.1111/j.1365-2036.2012.05180.x
20. Shornikova AV, Casas IA, Isolauri E, Mykkanen H, Vesikari T. Lactobacillus reuteri as a therapeutic agent in acute diarrhea in young children. J Pediatr Gastroenterol Nutr (1997) 24:399–404. doi:10.1097/00005176-199704000-00008
21. Oncel MY, Sari FN, Arayici S, Guzoglu N, Erdeve O, Uras N, et al. Lactobacillus reuteri for the prevention of necrotising enterocolitis in very low birthweight infants: a randomised controlled trial. Arch Dis Child Fetal Neonatal Ed (2014) 99:F110–5. doi:10.1136/archdischild-2013-304745
22. Rojas MA, Lozano JM, Rojas MX, Rodriguez VA, Rondon MA, Bastidas JA, et al. Prophylactic probiotics to prevent death and nosocomial infection in preterm infants. Pediatrics (2012) 130:e1113–20. doi:10.1542/peds.2011-3584
23. Athalye-Jape G, Rao S, Patole S. Lactobacillus reuteri DSM 17938 as a probiotic for preterm neonates: a strain-specific systematic review. JPEN J Parenter Enteral Nutr (2016) 40:783–94. doi:10.1177/0148607115588113
24. Urbanska M, Szajewska H. The efficacy of Lactobacillus reuteri DSM 17938 in infants and children: a review of the current evidence. Eur J Pediatr (2014) 173:1327–37. doi:10.1007/s00431-014-2328-0
25. Sung V, Collett S, de GT, Hiscock H, Tang M, Wake M. Probiotics to prevent or treat excessive infant crying: systematic review and meta-analysis. JAMA Pediatr (2013) 167:1150–7. doi:10.1001/jamapediatrics.2013.2572
26. Sharma R, Deshmukh US, Zheng L, Fu SM, Ju ST. X-linked Foxp3 (Scurfy) mutation dominantly inhibits submandibular gland development and inflammation respectively through adaptive and innate immune mechanisms. J Immunol (2009) 183:3212–8. doi:10.4049/jimmunol.0804355
27. Sharma R, Sharma PR, Kim YC, Leitinger N, Lee JK, Fu SM, et al. IL-2-controlled expression of multiple T cell trafficking genes and Th2 cytokines in the regulatory T cell-deficient scurfy mice: implication to multiorgan inflammation and control of skin and lung inflammation. J Immunol (2011) 186:1268–78. doi:10.4049/jimmunol.1002677
28. Suscovich TJ, Perdue NR, Campbell DJ. Type-1 immunity drives early lethality in scurfy mice. Eur J Immunol (2012) 42:2305–10. doi:10.1002/eji.201242391
29. Kanangat S, Blair P, Reddy R, Daheshia M, Godfrey V, Rouse BT, et al. Disease in the scurfy (sf) mouse is associated with overexpression of cytokine genes. Eur J Immunol (1996) 26:161–5. doi:10.1002/eji.1830260125
30. Singh N, Chandler PR, Seki Y, Baban B, Takezaki M, Kahler DJ, et al. Role of CD28 in fatal autoimmune disorder in scurfy mice. Blood (2007) 110:1199–206. doi:10.1182/blood-2006-10-054585
31. Antonioli L, Pacher P, Vizi ES, Hasko G. CD39 and CD73 in immunity and inflammation. Trends Mol Med (2013) 19:355–67. doi:10.1016/j.molmed.2013.03.005
32. Gomez G, Sitkovsky MV. Differential requirement for A2A and A3 adenosine receptors for the protective effect of inosine in vivo. Blood (2003) 102:4472–8. doi:10.1182/blood-2002-11-3624
33. Csoka B, Himer L, Selmeczy Z, Vizi ES, Pacher P, Ledent C, et al. Adenosine A2A receptor activation inhibits T helper 1 and T helper 2 cell development and effector function. FASEB J (2008) 22:3491–9. doi:10.1096/fj.08-107458
34. Arpaia N, Campbell C, Fan X, Dikiy S, van der VJ, DeRoos P, et al. Metabolites produced by commensal bacteria promote peripheral regulatory T-cell generation. Nature (2013) 504:451–5. doi:10.1038/nature12726
35. Furusawa Y, Obata Y, Fukuda S, Endo TA, Nakato G, Takahashi D, et al. Commensal microbe-derived butyrate induces the differentiation of colonic regulatory T cells. Nature (2013) 504:446–50. doi:10.1038/nature12721
36. Roelofsen H, Priebe MG, Vonk RJ. The interaction of short-chain fatty acids with adipose tissue: relevance for prevention of type 2 diabetes. Benef Microbes (2010) 1:433–7. doi:10.3920/BM2010.0028
37. da Rocha LF, da Silva MD, de Almeida CD, Santos AR. Anti-inflammatory effects of purine nucleosides, adenosine and inosine, in a mouse model of pleurisy: evidence for the role of adenosine A2 receptors. Purinergic Signal (2012) 8:693–704. doi:10.1007/s11302-012-9299-2
38. da Rocha LF, de Oliveira AP, Accetturi BG, de OM I, Domingos HV, de Almeida CD, et al. Anti-inflammatory effects of inosine in allergic lung inflammation in mice: evidence for the participation of adenosine A2A and A 3 receptors. Purinergic Signal (2013) 9:325–36. doi:10.1007/s11302-013-9351-x
39. Gomez G, Sitkovsky MV. Targeting G protein-coupled A2A adenosine receptors to engineer inflammation in vivo. Int J Biochem Cell Biol (2003) 35:410–4. doi:10.1016/S1357-2725(02)00177-2
40. Mabley JG, Pacher P, Murthy KG, Williams W, Southan GJ, Salzman AL, et al. The novel inosine analogue INO-2002 exerts an anti-inflammatory effect in a murine model of acute lung injury. Shock (2009) 32:258–62. doi:10.1097/SHK.0b013e31819c3414
41. Muto J, Lee H, Lee H, Uwaya A, Park J, Nakajima S, et al. Oral administration of inosine produces antidepressant-like effects in mice. Sci Rep (2014) 4:4199. doi:10.1038/srep04199
42. Rahimian R, Fakhfouri G, Daneshmand A, Mohammadi H, Bahremand A, Rasouli MR, et al. Adenosine A2A receptors and uric acid mediate protective effects of inosine against TNBS-induced colitis in rats. Eur J Pharmacol (2010) 649:376–81. doi:10.1016/j.ejphar.2010.09.044
43. Welihinda AA, Kaur M, Greene K, Zhai Y, Amento EP. The adenosine metabolite inosine is a functional agonist of the adenosine A receptor with a unique signaling bias. Cell Signal (2016) 28:552–60. doi:10.1016/j.cellsig.2016.02.010
45. Hasko G, Linden J, Cronstein B, Pacher P. Adenosine receptors: therapeutic aspects for inflammatory and immune diseases. Nat Rev Drug Discov (2008) 7:759–70. doi:10.1038/nrd2638
46. Carini R, Grazia De CM, Splendore R, Baldanzi G, Nitti MP, Alchera E, et al. Role of phosphatidylinositol 3-kinase in the development of hepatocyte preconditioning. Gastroenterology (2004) 127:914–23. doi:10.1053/j.gastro.2004.06.018
47. Chan ES, Montesinos MC, Fernandez P, Desai A, Delano DL, Yee H, et al. Adenosine A(2A) receptors play a role in the pathogenesis of hepatic cirrhosis. Br J Pharmacol (2006) 148:1144–55. doi:10.1038/sj.bjp.0706812
48. Reinstein LJ, Lichtman SN, Currin RT, Wang J, Thurman RG, Lemasters JJ. Suppression of lipopolysaccharide-stimulated release of tumor necrosis factor by adenosine: evidence for A2 receptors on rat Kupffer cells. Hepatology (1994) 19:1445–52. doi:10.1016/0270-9139(94)90241-0
49. Day YJ, Huang L, McDuffie MJ, Rosin DL, Ye H, Chen JF, et al. Renal protection from ischemia mediated by A2A adenosine receptors on bone marrow-derived cells. J Clin Invest (2003) 112:883–91. doi:10.1172/JCI15483
Keywords: regulatory T deficiency, autoimmunity, adenosine A2A receptor, Lactobacillus reuteri, cytokines, IPEX, scurfy, probiotic
Citation: He B, Hoang TK, Tran DQ, Rhoads JM and Liu Y (2017) Adenosine A2A Receptor Deletion Blocks the Beneficial Effects of Lactobacillus reuteri in Regulatory T-Deficient Scurfy Mice. Front. Immunol. 8:1680. doi: 10.3389/fimmu.2017.01680
Received: 22 August 2017; Accepted: 15 November 2017;
Published: 06 December 2017
Edited by:
Sudhir Gupta, University of California, Irvine, United StatesReviewed by:
Tomohiro Morio, Tokyo Medical and Dental University, JapanManish Butte, University of California, Los Angeles, United States
Copyright: © 2017 He, Hoang, Tran, Rhoads and Liu. This is an open-access article distributed under the terms of the Creative Commons Attribution License (CC BY). The use, distribution or reproduction in other forums is permitted, provided the original author(s) or licensor are credited and that the original publication in this journal is cited, in accordance with accepted academic practice. No use, distribution or reproduction is permitted which does not comply with these terms.
*Correspondence: Jon Marc Rhoads, ai5tYXJjLnJob2Fkc0B1dGgudG1jLmVkdQ==;
Yuying Liu, WXV5aW5nLkxpdUB1dGgudG1jLmVkdQ==