- 1Neuromodulation Center, Spaulding Rehabilitation Hospital, Department of Physical Medicine and Rehabilitation, Harvard Medical School, Boston, MA, United States
- 2Coma Science Group, GIGA-Research, University and University Hospital of Liege, Liege, Belgium
- 3Department of Psychology, Milan Center for Neuroscience-NeuroMi, University of Milano-Bicocca, Milano, Italy
- 4Laboratory for Neuropsychiatry and Neuromodulation, Transcranial Magnetic Stimulation Clinical Service, Department of Psychiatry, Massachusetts General Hospital, Boston, MA, United States
- 5Center for Integrative Medicine Research, Beth Israel Deaconess Medical Center, Harvard Medical School, Boston, MA, United States
- 6Post-Graduate Program in Medical Sciences, School of Medicine, Universidade Federal do Rio Grande do Sul (UFRGS), Porto Alegre, Brazil
- 7Laboratory of Pain and Neuromodulation, Hospital de Clínicas de Porto Alegre (HCPA), Porto Alegre, Brazil
- 8Department of Gynecology, Massachusetts General Hospital, Harvard Medical School, Boston, MA, United States
- 9Division of Translational Research, Beth Israel Deaconess Medical Center, Boston, MA, United States
Objective: Chronic visceral pain (CVP) syndromes are persistently painful disorders with a remarkable lack of effective treatment options. This study aimed at evaluating the effects of different neuromodulation techniques in patients with CVP on cortical activity, through electreocephalography (EEG) and on pain perception, through clinical tests.
Design: A pilot crossover randomized controlled study.
Settings: Out-patient.
Subjects: Adults with CVP (>3 months).
Methods: Participants received four interventions in a randomized order: (1) transcranial pulsed current stimulation (tPCS) and active transcranial direct current stimulation (tDCS) combined, (2) tPCS alone, (3) tDCS alone, and (4) sham condition. Resting state quantitative electroencephalography (qEEG) and pain assessments were performed before and after each intervention. Results were compared with a cohort of 47 healthy controls.
Results: We enrolled six patients with CVP for a total of 21 visits completed. Compared with healthy participants, patients with CVP showed altered cortical activity characterized by increased power in theta, alpha and beta bands, and a significant reduction in the alpha/beta ratio. Regarding tES, the combination of tDCS with tPCS had no effect on power in any of the bandwidths, nor brain regions. Comparing tPCS with tDCS alone, we found that tPCS induced higher increase in power within the theta and alpha bandwidths.
Conclusion: This study confirms that patients with CVP present abnormal EEG-indexed cortical activity compared with healthy controls. Moreover, we showed that combining two types of neurostimulation techniques had no effect, whereas the two interventions, when applied individually, have different neural signatures.
Introduction
Visceral pain results from nociceptor activation in thoracic, pelvic, or abdominal visceral organs (1). While acute pain has the vital role of preventing tissue damage, maladaptive processes may convert it into chronic (2), leading to chronic visceral pain (CVP), a condition featured by several maladaptive neural changes, one of them being central sensitization (3). The neural mechanisms of central sensitization in chronic neuropathic conditions are partially understood (4, 5). For instance, Simis et al. showed that patients with pelvic pain had significantly lower levels of N-acetylaspartate (NAA) and creatinophosphocreatine (Cr), reflecting loss of neuronal integrity in the primary motor cortex compared with healthy participants. This evidence also highlights the process of maladaptive plasticity in neural circuits involved in pain modulation (6). Notwithstanding, there is limited evidence showing neural correlates of pain-induced central sensitization in CVP. In addition, the investigation of cortical oscillation patterns by means of electroencephalography (EEG) is still at its infancy in patients with CVP.
This paucity of knowledge regarding neural correlates of CVP further undermines treatment interventions. CVP is characterized by high level of disability and discomfort (7–9) which affect patients’ quality of life and represent an important clinical problem and socioeconomic burden for societies (9–13). The lack of effective treatments, in turn, favors the excessive use of opioids (and their side effects), potentially leading to abuse (14, 15). Therefore, it is essential to develop new therapeutic options for CVP. Preliminary studies have shown that transcranial electrical stimulation (tES), in particular transcranial direct current stimulation (tDCS), may help managing pain. tDCS, in fact, by influencing neuronal cortical activity (16), has been proven capable of inducing clinical improvements in several chronic conditions (17, 18), such as fibromyalgia (19), abdominal (20), and refractory pelvic (21) pain. Recently, another tES technique, namely, transcranial pulsed current stimulation (tPCS) has gained increasing attention in experimental settings (22–24). tPCS, using randomly pulsed alternating current within a determined frequency range, is thought to reach cortical and subcortical brain regions such as the midbrain, pons, thalamus, insula, and the hypothalamus (25). Therefore, also tPCS, thanks to its mechanisms of action, could represent a promising tool for the treatment of pain, as it is potentially able to influence deeper structures involved in chronic pain.
In this scenario, we conducted a pilot study with a twofold aim: first, we wanted to assess baseline cortical activity of CVP patients; results are compared with those obtained by a normative sample of neurological healthy participants, published elsewhere (26). Second, we sought to assess the effects of two tES interventions, on cortical activity by means of EEG. We also provided preliminary data regarding the effects of such intervention on clinical pain assessments.
Materials and Methods
Participants
The inclusion criteria were as follows: (1) age between 18 and 65; (2) history of visceral pain for at least 3 months; (3) an average pain ≥4 in the past 3 months, as measured by the visual analog scale (VAS); (4) no history of neurologic or psychiatric conditions and no current unstable medical conditions; (5) no contraindications to tES; and (6) no current pregnancy. The study was approved by the Institutional Review Board of Spaulding Rehabilitation Hospital and performed in accordance with the Declaration of Helsinki. Healthy subjects’ cohort data have been published elsewhere (26).
Study Design
We conducted a pilot randomized, double-blind, sham-controlled crossover trial. Participants received the following four interventions in a randomized order separated by a minimum of 5 days: (1) active tPCS/active tDCS; (2) active tPCS/sham tDCS; (3) sham tPCS/active tDCS, and (4) sham tPCS/sham tDCS. Each stimulation condition was preceded and followed by EEG and clinical assessments.
Transcranial Direct Current Stimulation
Transcranial direct current stimulation was delivered with the anode electrode positioned over the left primary motor cortex (M1) and the cathode electrode over the contralateral right supra orbital region (Soterix Medical, NY, USA). Stimulation parameters were as follows: 20 min at 2 mA, 30-s fade-in and fade-out.
Transcranial Pulsed Current Stimulation
We used an investigational, custom-made, battery-powered and high-frequency tPCS device (Lab 8Tron AG) that delivered a quadratic biphasic alternating current using periauricular ear-clip electrodes. Stimulation parameters for tPCS were as follows: 20 min of stimulation at a fixed current intensity of 2 mA and a random noise frequency of 6–10 Hz [as previously described (23)]. For sham conditions (both tDCS and tPCS), stimulation parameters were the same, but the device turned off automatically after 30 s (27).
Quantitative Electroencephalography (qEEG) Recording, Analysis of Power and Interhemispheric Coherence
We used a 64-channel, high-density Electrical Geodesic Incorporated EEG device (Electrical Geodesics, OR, USA). EEG was recorded for 6 min eyes-closed. Data were sampled at 250 Hz, amplified and filtered using a bandpass of 0.1–70 Hz. For offline analysis, we used a low-pass filter of 40 Hz and high-pass of 1 Hz, followed by manual artifact detection and rejection by a blind assessor. Power and coherence were calculated using EEGLab (28) and MATLAB (MATLAB R2012a; The MathWorks Inc., Natick, MA, USA). Fast Fourier transformation (averaged windows of 5 s with 50% overlap) was used to calculate power (μV2) for the EEG bands theta (4–8 Hz), alpha (8–13 Hz) and beta (13–30 Hz), and the sub-bands low-alpha (8–10 Hz) and high-alpha (10–13 Hz). We also determined the alpha/beta power ratio. The signal from adjacent electrodes was averaged to represent frontal, central, parietal, temporal, and occipital areas.
We calculated interhemispheric coherence for these bands and sub-bands using two different electrode pairs: E19–E56 and E14–E57, located in the frontotemporal junction and including their reciprocal location in the contralateral hemisphere. Welch’s averaged modified periodogram method was used to find the estimated coherence of signal x and y, representing each electrode site.
Clinical Assessments
Visual analog scale for pain, anxiety, depression, stress, and sleepiness was collected. Von Frey Hair Assessment (North Coast Medical, Inc., Morgan Hill, CA, USA), comprised of monofilaments (0.008–300 g) was used to determine subjects’ perception threshold. This assessment was performed on the patient’s most painful region and over the ipsilateral hand to serve as a control. Pressure pain threshold (PPT) was also performed to measure subjects’ pain threshold (Commander Algometer, JTECH Medical, UT, USA). PPT was measured over the thenar eminence of both hands, namely, both ipsi- and contralateral to the most painful body area. For the evaluation of conditioned pain modulation (CPM), the same test applied for the PPT (test-stimulus) was repeated while the subject’s contralateral hand was immersed in cold water for 30 s (conditioned-stimulus).
Statistical Analyses
To compare neurophysiological and behavioral data of healthy controls (HCs) with that of CVP patients, we used baseline data of the first visit for each participant. Continuous variables (i.e., age and years of education) were compared using a t-test, while gender was analyzed using a χ2 test. Regarding EEG, baseline rhythms for each frequency band were compared between patients and HC (26), using a Mann–Whitney U test.
For patients only, Kruskal–Wallis test was used to evaluate the effects of stimulation on power and coherence variables (difference between pre- and post-stimulation values) with tES (tPCS/tDCS, tPCS, tDCS, and Sham) as independent fixed variable. Mann–Whitney tests were applied for post hoc comparisons. For each clinical assessment, a Friedman’s ANOVA was performed, with tES (tPCS/tDCS, tPCS, tDCS, and Sham) as the main factor.
Results
Six patients (2 males and 4 females) were included in the present study (see Table 1 for demographic and clinical data) for a total of 21 visits completed (5 patients completed the tPCS/tDCS session, 5 completed the tPCS session, 6 completed the tDCS session, and 5 completed the sham session). One patient drop out after the first visit (tDCS session) due to scheduling issues.
Baseline Characteristics of Patients Compared with HC
Chronic visceral pain patients and HC were similar for age (t = 0.885; p = 0.432), gender (χ2 = 0.24; p = 0.622), and level of education (t = 2.47; p = 0.122). At baseline, CVP patients had significantly more power in theta (p < 0.001), alpha (p < 0.0001), low-alpha (p < 0.001), and beta (p < 0.001) bandwidths, compared with HC. On the other hand, alpha/beta ratio was significantly reduced in patients with CVP (p < 0.001) (Table 2).
EEG Power Analysis in Patients
tES Effect
There were no differences in the baseline power spectrum in the frontal, central, parietal, temporal, or occipital brain regions (all ps > 0.05). After tES, there was a significant effect over all areas combined (i.e., global) for the theta bandwidth (p < 0.001), for the low-alpha (p = 0.016), high-alpha (p = 0.027) bandwidths, and for alpha/beta ratio (p < 0.001); see Figure 1. We also found a tES effect over the central and the parietal regions for the alpha/beta ratio (p = 0.037 and p = 0.028, respectively) and over the occipital region for the theta bandwidth (p = 0.002). No other effect was significant. Figure 2 represents the different topomaps of a representative patient (patient 1).
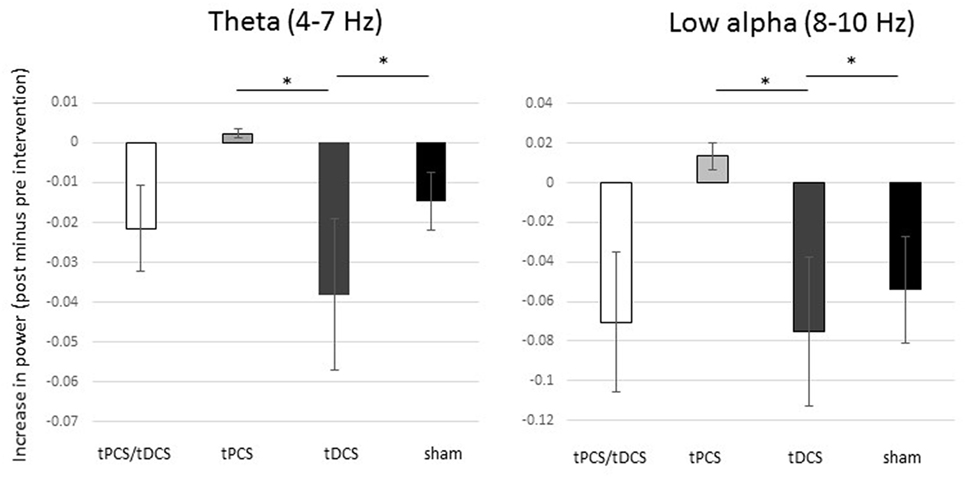
Figure 1. Differences in absolute power between groups. Mean differences (pre- versus post-intervention) of power for theta and low-alpha bandwidths. The bars represent the standard error. * Significant difference between groups.
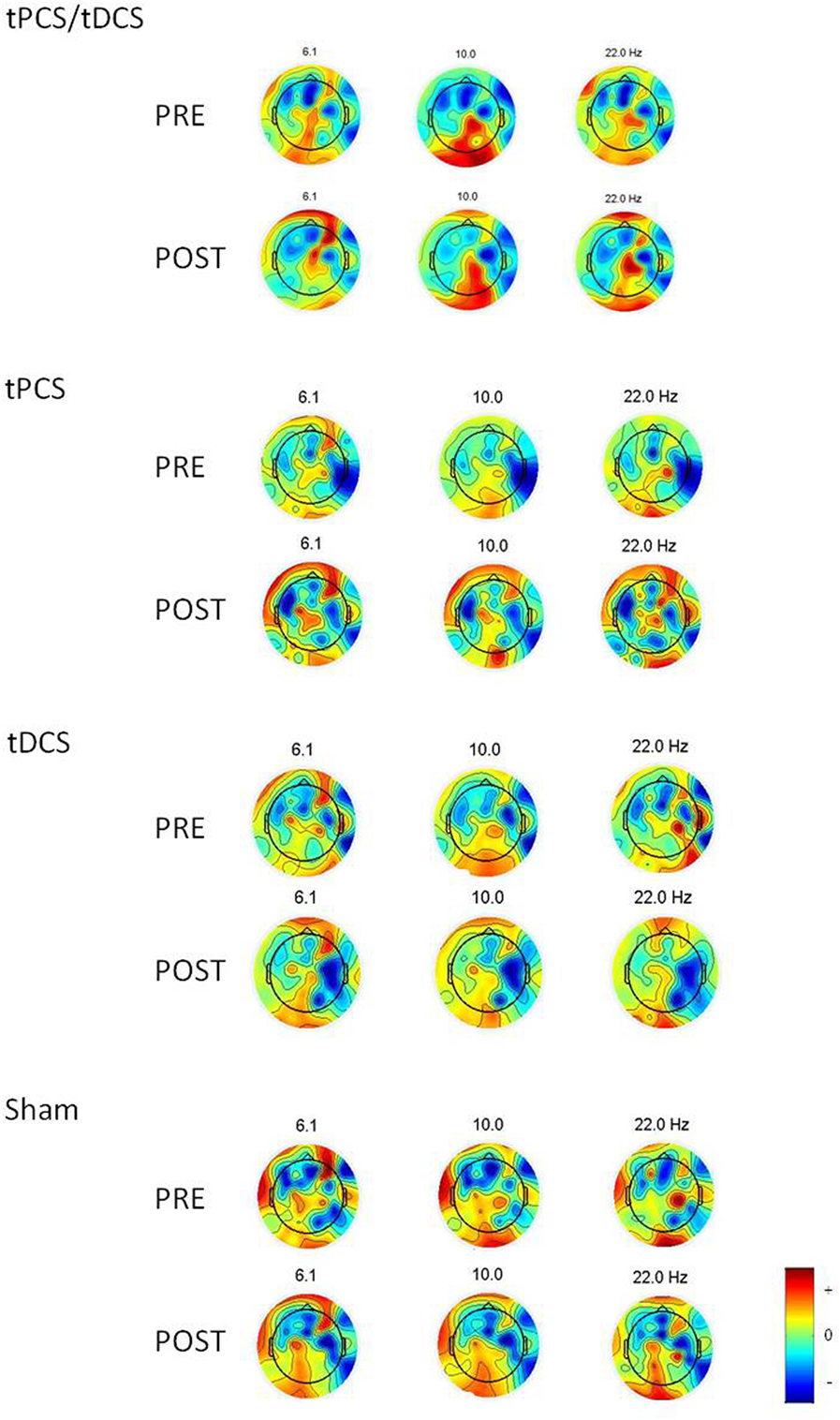
Figure 2. Topoplots showing the topographic distribution of the different bandwidths for a representative individual before and after each intervention. Red areas represent higher activity, while blue areas represent lower activity.
Post Hoc Analysis
As shown in Figure 1, combined tPCS with tDCS was not different as compared with sham for any of the frequency bands or brain regions (all ps > 0.05). In contrast to tDCS, tPCS induced significantly higher increase in power, in theta (p < 0.001) and low-alpha (p = 0.004) bandwidths, as well as for the alpha/beta ratio (p = 0.011) for global. In addition, tPCS induced a significantly higher increase in theta power over the occipital region, as compared with tDCS (p < 0.001).
Transcranial direct current stimulation induced higher reduction in power of theta (p = 0.002) and low-alpha (p = 0.01) bandwidths as compared with sham, as well as a reduction of alpha/beta ratio (p < 0.001) for global. In addition, tDCS induced a reduction of the alpha/beta ratio over the frontal region, but this decrease was significantly less important than sham (p = 0.023).
Transcranial pulsed current stimulation induced a decrease in power in the high-alpha bandwidth for global (p = 0.033) as compared with sham. Despite a significant group effect for alpha/beta ratio over the parietal region, none of the post hoc tests reached the significance level.
Coherence
No group effect for any bandwidths or brain regions was found (all ps > 0.05).
Individual power and coherence data can be found in Supplementary Material.
Clinical Assessments
No differences were found in either depression VAS (χ2 = 3.86; p = 0.27), anxiety VAS (χ2:1.5, p = 0.68), stress VAS (χ2:4.87, p = 0.18), sleepiness VAS (χ2 = 55.7, p = 0.12), or pain VAS (χ2 = 5.87, p = 0.12). No significant effect was found for Von Frey assessment over the painful- (χ2 = 6.6, p = 0.08), nor over the non-painful (thenar) region (χ2 = 2.6, p = 0.46) region.
Similarly, no significant differences were found for PPT (χ2 = 0.4; p = 0.94) or CPM (χ2 = 3.5; p = 0.32).
Individual clinical data can be found in Supplementary Material.
Discussion
The present study yielded interesting findings: (1) patients with CVP display abnormal neural activity compared with healthy controls, as indexed by qEEG; (2) EEG captured cortical changes following tES, similar to what was observed in healthy controls, while no clinical improvement was noticed; (3) combining tDCS with tPCS does not induce specific changes in neural activity; (4) tDCS and tPCS have different neural signature.
So far, only few studies have investigated the neurophysiological patterns of patients with CVP. For instance, EEG measures in patients with chronic pancreatitis show an increase in power in the theta and alpha frequency bands as well (29). Present findings substantiate this altered cortical activity in patients with CVP. Our results, in fact, show an increased power in different frequencies bands (i.e., theta, low alpha, and beta) and a decrease in low versus high frequencies ratio, as compared with healthy participants (26). Therefore, this feature seems to represent a reproducible pattern in patients with chronic pain, as pointed out by a recent systematic review (30).
Baseline cortical activity could serve as a biomarker for treatment effects and as a predictor of response when using tES and other neuromodulatory techniques. As seen in patients with spinal cord injury and chronic pain, increased theta power at baseline was associated with greater response to analgesic treatment; in this case, hypnosis (31). We also noticed that the alpha/beta ratio was significantly reduced in patients with CVP, compared with healthy participants (26). Even though our sample size is small to define a neurophysiological biomarker that serves as a predictor of response to tES, EEG may represent a valuable tool for this purpose in chronic pain (29, 32, 33). For instance, it has been shown that peak alpha frequency may represent valuable biomarker for chronic pain, as this measure is not only greatly decreased in patients, compared with healthy participants, but it is also correlated with the duration of pain (29). In this context, this pilot study provides the initial data to support further studies investigating changes in brain oscillations and maladaptive plasticity in patients with CVP.
An important mechanism that deserves further investigation in the context of these results is the interaction between pain, immune system and electrical stimulation. It has been discussed before that pain may trigger also immune mechanisms in a two-way brain response to injury (34) and electrical stimulation may modulate this interaction (35). This relationship between immune system and pain may also be used to find useful biomarkers and responders. For instance, the relationship between gene expression and pain has been demonstrated in mice (36). Specifically, it has been shown that cholecystokinin is implicated in the modulation of pain sensitivity and the development of neuropathic pain in mice and, more interestingly, when the cholecystokinin receptor gene is removed, the pain sensitivity is reduced and the development of hyperalgesia is abolished (37). In this context, a combination of electrophysiology and immune system gene expression study could open new doors and new treatments options in the field of chronic pain.
While no changes were observed clinically, neurophysiologically, the combination of tDCS with tPCS did not lead to any changes in brain activity, while the application of the two techniques separately induced different cortical modifications, as already demonstrated (23, 24, 38, 39). Therefore, it is becoming increasingly apparent that the effects of tDCS and tPCS are not mediated by the same neurophysiological mechanisms (40). By reaching deeper structures, it seems feasible that tPCS could stimulate bottom–up connectivity trough thalamo-cortical circuits (40). On the other hand, tDCS seems to target a top–down cortico-thalamic pathway (41) and increases cortical excitability under the stimulating electrodes and associated cortical networks (38). It is possible that tPCS and tDCS, when applied simultaneously, would eliminate their respective effects on brain activity. This important result underscores the need of careful consideration before associating different tES techniques.
Considering each technique individually, tPCS has shown promising and reproducible results as a neuromodulatory tool given the reported effects on coherence and power (22, 23, 40). In particular, in healthy volunteers, 6–10 Hz tPCS seems to modulate brain oscillation within the spectrum contained in these frequencies (i.e., high theta and low alpha). While for tDCS, an increased power has been observed in the high beta bandwidth over temporal and parietal regions, as compared with sham.
Our results showed that tPCS induced a higher increase in power within these two specific frequency bands compared with tDCS. We also identified a decrease in power for high-alpha bandwidth after tPCS and a decrease in power for theta and low-alpha bandwidths after tDCS, as compared with sham. This reduction in EEG power may represent a cortical modulation reducing a putative pathological over-excitability in patients suffering from chronic forms of pain. In fact, baseline EEG power of the main frequency bands is increased in CVP as compared with HC, suggesting a pathological over-activity in patients, especially over the sensorimotor cortex (i.e., central region). Therefore, the decrease here found can be interpreted as a normalization of the cortical oscillations, leading to a pattern more similar to healthy conditions.
No clinical changes were observed after tDCS, tPCS, or both interventions combined. Evidence shows that repeated tES sessions, and tDCS in particular, are required to induce long-lasting and significant clinical effects in psychiatric (42), motor (43), and pain conditions (44). Particularly, a significant decrease in chronic abdominal pain was registered after 5 days of anodal tDCS over the left motor cortex and persisted 1 week later (20). Similarly, a modest reduction of pain was reported by a small group of patients after two tDCS sessions (21). Moreover, a mean pain response of 58% compared with sham was registered after 5 days of anodal stimulation of the left motor cortex in neuropathic pain (45). It is hypothesized that recurring stimulation sessions are needed to produce perceptible and lasting clinical effects due to mechanisms resembling long-term potentiation (LTP) and long-term depression (LTD). Therefore, it is quite possible that a single-stimulation session was not sufficient to induce clinical effects in this experiment. Furthermore, the EEG montage and recording, often taking up to 20 min, was performed after the end of the stimulation. This represents a gap between the end of stimulation and clinical assessments, potentially allowing for any measurable effect to fade out by the time of collection.
Present findings, although preliminary, appear promising regarding the potentiality of tES techniques in modulating pathological cortical activity in CVP. Notwithstanding, they should be considered cautiously. When studying patients suffering from chronic pain, the effect of medications on spontaneous brain oscillations should be considered. In the present study, all patients but one were under one or more opioids drugs (see Table 1). Opioid-induced slowing of the EEG has been demonstrated with different morphine derivatives such as fentanyl (46) and oxycodone (47) under controlled infusions, while tramadol an opioid-like agent favors fast EEG power spectrum responses (48), including spikes generation. Still, prolonged use of oral or topic administration of these agents might induce modulation of spontaneous brain activity measured on scalp EEG, especially, shifting the background rhythm toward the low frequencies of the spectrum. For the results presented here, medication can be considered a confounding or interacting variable which deserves better control in future studies. However, as every patient received the four conditions (except for one), we can consider that pain medication influenced brain activity identically for the four types of tES.
Regarding the sham intervention, an important placebo component was identified (i.e., increase in theta and alpha and a decrease in beta bandwidths following the sham intervention). This could be interpreted in two different ways: (1) the observed increase in theta and alpha power and decrease in high-frequency bands could be related to relaxation. A similar pattern has been observed, for instance, under meditation (49–51). Here, active tES could enhance patients’ attention and therefore does not lead to an increase in drowsiness related rhythms. Therefore, the observed modification after the sham stimulation could be due to the other active conditions that blocked the occurrence of these modifications. (2) In this population of patients, the placebo effect and expectations are important components to take into account placebo effect and is often observed in patients with chronic pain (52) and also after electrical stimulation (53). This placebo effect could also partially explain our findings following the sham stimulation. Another important limitation to consider is that this study was a pilot exploratory study, and thus the lack of significant results in some of the analyses may simply indicate lack of power for that analysis. The goal was to inform feasibility and also provide effect estimates and discuss preliminary findings to design further studies.
Conclusion and Future Directions
With respect to tES techniques, our preliminary data suggest that the combination of tDCS with tPCS, applied simultaneously, does not lead to improvements in cortical oscillations, similarly to what has been observed in healthy population (26). We also highlighted that tDCS and tPCS influence brain activity differently, and therefore may be underlined by different neural mechanisms as previously shown (26, 40). While there are a limited amount of data on the neurophysiological reorganization in patients with CVP, our results could help designing future trials using tES as an intervention and neurophysiological assessment to evaluate its effects. EEG could represent a suitable biomarker to characterize neural states of chronic pain and to capture the immediate effects of tES (single or short-term protocol). In this scenario, EEG could further be used as a predictor of response (i.e., to differentiate treatment responders from non-responders based on their neural signature), and to better understand the neurophysiological mechanisms of tES on pain relief. The combination of clinical assessments and EEG may become a useful tool to tailor customized treatment options and improve the efficacy of tES in alleviating pain.
Ethics Statement
The study was approved by the Institutional Review Board of Spaulding Rehabilitation Hospital and performed in accordance with the Declaration of Helsinki. All subjects gave written informed consent in accordance with the Declaration of Helsinki. The protocol was approved by the local institutional review board. Healthy subjects’ cohort data have been published elsewhere.
Author Contributions
AT, CR, AD, and AH collected data. AT and CR analyzed the data, interpreted the results, and drafted the manuscript. FF designed the study, interpreted the results, and critically reviewed the manuscript. AD, AH, JM-Q, JP, and SF critically reviewed the manuscript and had substantial intellectual contributions. All authors approved the last version of the manuscript.
Conflict of Interest Statement
The authors declare that the research was conducted in the absence of any commercial or financial relationships that could be construed as a potential conflict of interest.
Acknowledgments
This research was supported by the Labuschagne Foundation; the Belgian American Educational Foundation (BAEF) and the Fonds Leon Fredericq Foundation (to AT); Institutional National Research Service Award from the National Center for Complementary and Integrative Health (grant number T32AT000051 to JM-Q); the Ryoichi Sasakawa Fellowship Fund; the Program in Placebo Studies at Beth Israel Deaconess Medical Center; the Coordination for the Improvement of Higher Education Personnel––CAPES; International Cooperation General Program––PGCI (grant number 023/11 to AD); and NIH RO1 (grant number 1R01HD082302-01A1 to FF).
Supplementary Material
The Supplementary Material for this article can be found online at http://www.frontiersin.org/article/10.3389/fneur.2017.00576/full#supplementary-material.
References
1. Kansal A, Hughes J. Visceral pain. Anaesth Intensive Care Med (2016) 17:543–7. doi:10.1016/j.mpaic.2016.08.013
2. Kuner R, Flor H. Structural plasticity and reorganisation in chronic pain. Nat Rev Neurosci (2016) 18:20–30. doi:10.1038/nrn.2016.162
3. Sengupta JN. Visceral pain: the neurophysiological mechanism. In: Canning B, Spina D, editors. Sensory Nerves. Berlin, Heidelberg: Springer. vol. 194 (2009). p. 31–74. doi:10.1007/978-3-540-79090-7_2
4. Latremoliere A, Woolf CJ. Central sensitization: a generator of pain hypersensitivity by central neural plasticity. J Pain (2009) 10:895–926. doi:10.1016/j.jpain.2009.06.012
5. Woolf CJ. Central sensitization: implications for the diagnosis and treatment of pain. Pain (2012) 152:1–31. doi:10.1016/j.pain.2010.09.030.Central
6. Simis M, Reidler JS, Duarte Macea D, Moreno Duarte I, Wang X, Lenkinski R, et al. Investigation of central nervous system dysfunction in chronic pelvic pain using magnetic resonance spectroscopy and noninvasive brain stimulation. Pain Pract (2015) 15:423–32. doi:10.1111/papr.12202
7. Hungin APS, Whorwell PJ, Tack J, Mearin F. The prevalence, patterns and impact of irritable bowel syndrome: an international survey of 40 000 subjects. Aliment Pharmacol Ther (2003) 17:643–50. doi:10.1046/j.1365-2036.2003.01456.x
8. Hungin APS, Chang L, Locke GR, Dennis EH, Barghout V. Irritable bowel syndrome in the United States: prevalence, symptom patterns and impact. Aliment Pharmacol Ther (2005) 21:1365–75. doi:10.1111/j.1365-2036.2005.02463.x
9. Paré P, Gray J, Lam S, Balshaw R, Khorasheh S, Barbeau M, et al. Health-related quality of life, work productivity, and health care resource utilization of subjects with irritable bowel syndrome: baseline results from logic (longitudinal outcomes study of gastrointestinal symptoms in Canada), a naturalistic study. Clin Ther (2006) 28:1726–35. doi:10.1016/j.clinthera.2006.10.010
10. Dean BB, Aguilar D, Barghout V, Kahler KH, Frech F, Groves D, et al. Impairment in work productivity and health-related quality of life in patients with IBS. Am J Manag Care (2005) 11:S17–26.
11. Leong SA, Barghout V, Birnbaum HG, Thibeault CE, Ben-Hamadi R, Frech F, et al. The economic consequences of irritable bowel syndrome: a US employer perspective. Arch Intern Med (2003) 163:929–35. doi:10.1001/archinte.163.8.929
12. Mathias SD, Kuppermann M, Liberman RF, Lipschutz RC, Steege JF. Chronic pelvic pain: prevalence, health-related quality of life, and economic correlates. Obstet Gynecol (1996) 87:321–7. doi:10.1016/0029-7844(95)00458-0
13. Zondervan K, Barlow DH. Epidemiology of chronic pelvic pain. Best Pract Res Clin Obstet Gynaecol (2000) 14:403–14. doi:10.1053/beog.1999.0083
14. Manchikanti L, Abdi S, Atluri S, Balog CC, Benyamin RM, Boswell MV, et al. American Society of Interventional Pain Physicians (ASIPP) guidelines for responsible opioid prescribing in chronic non-cancer pain: part I – evidence assessment. Pain Physician (2012) 15:S1–65.
15. Manchikanti L, Atluri S, Hansen H, Benyamin RM, Falco FJE, Helm S, et al. Opioids in chronic noncancer pain: have we reached a boiling point yet? Pain Physician (2014) 17:E1–10.
16. Nitsche MA, Paulus W. Excitability changes induced in the human motor cortex by weak transcranial direct current stimulation. J Physiol (2000) 527(Pt 3):633–9. doi:10.1111/j.1469-7793.2000.t01-1-00633.x
17. Lefaucheur J. Cortical neurostimulation for neuropathic pain: state of the art and perspectives. Pain (2016) 157(Suppl):S81–9. doi:10.1097/j.pain.0000000000000401
18. Lefaucheur JP, Antal A, Ayache SS, Benninger DH, Brunelin J, Cogiamanian F, et al. Evidence-based guidelines on the therapeutic use of transcranial direct current stimulation (tDCS). Clin Neurophysiol (2017) 128:56–92. doi:10.1016/j.clinph.2016.10.087
19. Fregni F, Gimenes R, Valle AC, Ferreira MJL, Rocha RR, Natalle L, et al. A randomized, sham-controlled, proof of principle study of transcranial direct current stimulation for the treatment of pain in fibromyalgia. Arthritis Rheum (2006) 54:3988–98. doi:10.1002/art.22195
20. Volz M, Farmer A, Siegmund B. Reduction of chronic abdominal pain in patients with inflammatory bowel disease through transcranial direct current stimulation: a randomized controlled trial. Pain (2016) 157(2):429–37. doi:10.1097/j.pain.0000000000000386
21. Fenton BW, Palmieri PA, Boggio P, Fanning J, Fregni F. A preliminary study of transcranial direct current stimulation for the treatment of refractory chronic pelvic pain. Brain Stimul (2009) 2:103–7. doi:10.1016/j.brs.2008.09.009
22. Morales-Quezada L, Saavedra LC, Rozisky J, Hadlington L, Fregni F. Intensity-dependent effects of transcranial pulsed current stimulation on interhemispheric connectivity: a high-resolution qEEG, sham-controlled study. Neuroreport (2014) 25:1054–8. doi:10.1097/WNR.0000000000000228
23. Morales-Quezada L, Castillo-Saavedra L, Cosmo C, Doruk D, Sharaf I, Malavera A, et al. Optimal random frequency range in transcranial pulsed current stimulation indexed by quantitative electroencephalography. Neuroreport (2015) 26:747–52. doi:10.1097/WNR.0000000000000415
24. Vasquez A, Malavera A, Doruk D, Morales-Quezada L, Carvalho S, Leite J, et al. Duration dependent effects of transcranial pulsed current stimulation (tPCS) indexed by electroencephalography. Neuromodulation (2016) 19(7):679–88. doi:10.1111/ner.12457
25. Datta A, Dmochowski JP, Guleyupoglu B, Bikson M, Fregni F. Cranial electrotherapy stimulation and transcranial pulsed current stimulation: a computer based high-resolution modeling study. Neuroimage (2013) 65:280–7. doi:10.1016/j.neuroimage.2012.09.062
26. Thibaut A, Russo C, Morales-Quezada L, Hurtado-Puerto A, Deitos A, Freedman S, et al. Neural signature of tDCS, tPCS and their combination: comparing the effects on neural plasticity. Neurosci Lett (2017) 637:207–14. doi:10.1016/j.neulet.2016.10.026
27. Gandiga PC, Hummel FC, Cohen LG. Transcranial DC stimulation (tDCS): a tool for double-blind sham-controlled clinical studies in brain stimulation. Clin Neurophysiol (2006) 117:845–50. doi:10.1016/j.clinph.2005.12.003
28. Delorme A, Makeig S. EEGLAB: an open source toolbox for analysis of single-trial EEG dynamics including independent component analysis. J Neurosci Methods (2004) 134:9–21. doi:10.1016/j.jneumeth.2003.10.009
29. de Vries M, Wilder-Smith OHG, Jongsma MLA, van den Broeke EN, Arns M, van Goor H, et al. Altered resting state EEG in chronic pancreatitis patients: toward a marker for chronic pain. J Pain Res (2013) 6:815–24. doi:10.2147/JPR.S50919
30. Pinheiro ESDS, de Queiros FC, Montoya P, Santos CL, do Nascimento MA, Ito CH, et al. Electroencephalographic patterns in chronic pain: a systematic review of the literature. PLoS One (2016) 11:e0149085. doi:10.1371/journal.pone.0149085
31. Jensen MP, Sherlin LH, Fregni F, Gianas A, Howe JD, Hakimian S. Baseline brain activity predicts response to neuromodulatory pain treatment. Pain Med (2014) 15:2055–63. doi:10.1111/pme.12546
32. Camfferman D, Moseley G, Gertz K, Pettet M, Jensen M. Waking EEG cortical markers of chronic pain and sleepiness. Pain Med (2017) 18(10):1921–31. doi:10.1093/pm/pnw294
33. Jensen MP, Day MA, Miró J. Neuromodulatory treatments for chronic pain: efficacy and mechanisms. Nat Rev Neurol (2014) 10:167–78. doi:10.1038/nrneurol.2014.12
34. Fregni F, Pascual-Leone A, Freedman SD. Pain in chronic pancreatitis: a salutogenic mechanism or a maladaptive brain response? Pancreatology (2007) 7:411–22. doi:10.1159/000108958
35. Li G, Li S, Sun L, Lin F, Wang B. A comparison study of immune-inflammatory response in electroacupuncture and transcutaneous electrical nerve stimulation for patients undergoing supratentorial craniotomy. Int J Clin Exp Med (2015) 8:1156–61.
36. Kõks S, Fernandes C, Kurrikoff K, Vasar E, Schalkwyk LC. Gene expression profiling reveals upregulation of Tlr4 receptors in Cckb receptor deficient mice. Behav Brain Res (2008) 188:62–70. doi:10.1016/j.bbr.2007.10.020
37. Kurrikoff K, Kõks S, Matsui T, Bourin M, Arend A, Aunapuu M, et al. Deletion of the CCK2 receptor gene reduces mechanical sensitivity and abolishes the development of hyperalgesia in mononeuropathic mice. Eur J Neurosci (2004) 20:1577–86. doi:10.1111/j.1460-9568.2004.03619.x
38. Keeser D, Padberg F, Reisinger E, Pogarell O, Kirsch V, Palm U, et al. Prefrontal direct current stimulation modulates resting EEG and event-related potentials in healthy subjects: a standardized low resolution tomography (sLORETA) study. Neuroimage (2011) 55:644–57. doi:10.1016/j.neuroimage.2010.12.004
39. Polania R, Nitsche MA, Paulus W. Modulating functional connectivity patterns and topological functional organization of the human brain with transcranial direct current stimulation. Hum Brain Mapp (2011) 32:1236–49. doi:10.1002/hbm.21104
40. Vasquez AC, Thibaut A, Morales-Quezada L, Leite J, Fregni F. Patterns of brain oscillations across different electrode montages in transcranial pulsed current stimulation. Neuroreport (2017) 28:421–5. doi:10.1097/WNR.0000000000000772
41. Frase L, Piosczyk H, Zittel S, Jahn F, Selhausen P, Krone L, et al. Modulation of total sleep time by transcranial direct current stimulation (tDCS). Neuropsychopharmacology (2016) 41(10):2577–86. doi:10.1038/npp.2016.65
42. Brunoni AR, Valiengo L, Baccaro A, Zanão TA, de Oliveira JF, Goulart A, et al. The sertraline vs. electrical current therapy for treating depression clinical study: results from a factorial, randomized, controlled trial. JAMA Psychiatry (2013) 70:383–91. doi:10.1001/2013.jamapsychiatry.32
43. Boggio PS, Nunes A, Rigonatti SP, Nitsche MA, Pascual-Leone A, Fregni F. Repeated sessions of noninvasive brain DC stimulation is associated with motor function improvement in stroke patients. Restor Neurol Neurosci (2007) 25:123–9.
44. Castillo-Saavedra L, Gebodh N, Bikson M, Diaz-Cruz C, Brandao R, Coutinho L, et al. Clinically effective treatment of fibromyalgia pain with high-definition transcranial direct current stimulation: phase II open-label dose optimization. J Pain (2016) 17:14–26. doi:10.1016/j.jpain.2015.09.009
45. Fregni F, Boggio PS, Lima MC, Ferreira MJ, Wagner T, Rigonatti SP, et al. A sham-controlled, phase II trial of transcranial direct current stimulation for the treatment of central pain in traumatic spinal cord injury. Pain (2006) 122:197–209. doi:10.1016/j.pain.2006.02.023
46. Scott JC, Cooke JE, Stanski DR. Electroencephalographic quantitation of opioid effect: comparative pharmacodynamics of fentanyl and sufentanil. Anesthesiology (1991) 74:34–42. doi:10.1097/00000542-199101000-00007
47. Pöyhiä R, Hynynen M, Seppälä T, Roine RO, Verkkala K, Olkkola KT. Pharmacodynamics and pharmacokinetics of high-dose oxycodone infusion during and after coronary artery bypass grafting. J Cardiothorac Vasc Anesth (2004) 18:748–54. doi:10.1053/j.jvca.2004.08.013
48. Freye E, Levy JV. The effects of tramadol on pain relief, fast EEG-power spectrum and cognitive function in elderly patients with chronic osteoarthritis (OA). Acute Pain (2006) 8:55–61. doi:10.1016/j.acpain.2006.03.001
49. Baijal S, Srinivasan N. Theta activity and meditative states: spectral changes during concentrative meditation. Cogn Process (2010) 11:31–8. doi:10.1007/s10339-009-0272-0
50. Jensen MP, Sherlin LH, Askew RL, Fregni F, Witkop G, Gianas A, et al. Effects of non-pharmacological pain treatments on brain states. Clin Neurophysiol (2013) 124:2016–24. doi:10.1016/j.clinph.2013.04.009
51. Lagopoulos J, Xu J, Rasmussen I, Vik A, Malhi GS, Eliassen CF, et al. Increased theta and alpha EEG activity during nondirective meditation. J Altern Complement Med (2009) 15:1187–92. doi:10.1089/acm.2009.0113
52. Reicherts P, Gerdes ABM, Pauli P, Wieser MJ. Psychological placebo and nocebo effects on pain rely on expectation and previous experience. J Pain (2016) 17:203–14. doi:10.1016/j.jpain.2015.10.010
Keywords: chronic visceral pain, pain, transcranial direct current stimulation, transcranial pulsed current stimulation, non-invasive brain stimulation, electroencephalogram
Citation: Thibaut A, Russo C, Hurtado-Puerto AM, Morales-Quezada JL, Deitos A, Petrozza JC, Freedman S and Fregni F (2017) Effects of Transcranial Direct Current Stimulation, Transcranial Pulsed Current Stimulation, and Their Combination on Brain Oscillations in Patients with Chronic Visceral Pain: A Pilot Crossover Randomized Controlled Study. Front. Neurol. 8:576. doi: 10.3389/fneur.2017.00576
Received: 17 August 2017; Accepted: 13 October 2017;
Published: 01 November 2017
Edited by:
Francisco R. Nieto, University of Granada, SpainReviewed by:
Sulev Kõks, University of Tartu, EstoniaBin Gu, University of North Carolina at Chapel Hill, United States
Copyright: © 2017 Thibaut, Russo, Hurtado-Puerto, Morales-Quezada, Deitos, Petrozza, Freedman and Fregni. This is an open-access article distributed under the terms of the Creative Commons Attribution License (CC BY). The use, distribution or reproduction in other forums is permitted, provided the original author(s) or licensor are credited and that the original publication in this journal is cited, in accordance with accepted academic practice. No use, distribution or reproduction is permitted which does not comply with these terms.
*Correspondence: Aurore Thibaut, YXRoaWJhdXRAbmV1cm9tb2R1bGF0aW9ubGFiLm9yZw==;
Felipe Fregni, ZmVsaXBlLmZyZWduaUBtZ2guaGFydmFyZC5lZHU=
†These authors share the first position.