- 1Arctic and Marine Biology, UiT – The Arctic University of Norway, Tromsø, Norway
- 2Bioscience, Fishery and Economy, UiT – The Arctic University of Norway, Tromsø, Norway
- 3Division of Wildlife Conservation, Alaska Department of Fish and Game, Fairbanks, AK, United States
Brucella pinnipedialis was first isolated from true seals in 1994 and from eared seals in 2008. Although few pathological findings have been associated with infection in true seals, reproductive pathology including abortions, and the isolation of the zoonotic strain type 27 have been documented in eared seals. In this study, a Brucella enzyme-linked immunosorbent assay (ELISA) and the Rose Bengal test (RBT) were initially compared for 206 serum samples and a discrepancy between the tests was found. Following removal of lipids from the serum samples, ELISA results were unaltered while the agreement between the tests was improved, indicating that serum lipids affected the initial RBT outcome. For the remaining screening, we used ELISA to investigate the presence of Brucella antibodies in sera of 231 eared and 1,412 true seals from Alaskan waters sampled between 1975 and 2011. In eared seals, Brucella antibodies were found in two Steller sea lions (Eumetopias jubatus) (2%) and none of the 107 Northern fur seals (Callorhinus ursinus). The low seroprevalence in eared seals indicate a low level of exposure or lack of susceptibility to infection. Alternatively, mortality due to the Brucella infection may remove seropositive animals from the population. Brucella antibodies were detected in all true seal species investigated; harbor seals (Phoca vitulina) (25%), spotted seals (Phoca largha) (19%), ribbon seals (Histriophoca fasciata) (16%), and ringed seals (Pusa hispida hispida) (14%). There was a low seroprevalence among pups, a higher seroprevalence among juveniles, and a subsequent decreasing probability of seropositivity with age in harbor seals. Similar patterns were present for the other true seal species; however, solid conclusions could not be made due to sample size. This pattern is in accordance with previous reports on B. pinnipedialis infections in true seals and may suggest environmental exposure to B. pinnipedialis at the juvenile stage, with a following clearance of infection. Furthermore, analyses by region showed minor differences in the probability of being seropositive for harbor seals from different regions regardless of the local seal population trend, signifying that the Brucella infection may not cause significant mortality in these populations. In conclusion, the Brucella infection pattern is very different for eared and true seals.
Introduction
Alaskan waters accommodate a number of pinniped species, both true seals (family Phocidae) including Eastern North Pacific harbor seals (Phoca vitulina richardsii), spotted seals (Phoca largha), ribbon seals (Histriophoca fasciata), Arctic ringed seals (Pusa hispida hispida), and bearded seals (Erignathus barbatus), as well as eared seals (family Otariidae) including Steller sea lions (Eumetopias jubatus) and Northern fur seals (Callorhinus ursinus) (1). Currently, bearded seals of the Bering Sea distinct population segment, and Steller sea lions in the western distinct population segment have been listed as threatened (“likely to become an endangered species within the foreseeable future”) under the Endangered Species Act (ESA). Although the Northern fur seal Pribilof Island stock has not been listed under the ESA, they have been deemed depleted (“below its optimum sustainable population”) under the Marine Mammal Protection Act (MMPA) (2). Listings for seals were based on their predicted negative responses to climate change, while for sea lions and fur seals it is due to population declines for unknown reasons. Furthermore, the International Union for Conservation of Nature and Natural Resources (IUCN) Red List of Threatened Species considers several of these species as “Data deficient” (“inadequate information to make a direct, or indirect, assessment of its risk of extinction based on its distribution and/or population status”) (3). Clearly there are concerns about how well these species and populations are able to adapt to future climate change scenarios where disease prevalence is predicted to increase as new species with novel pathogens appear to exploit warmer waters and longer open water seasons, and the host–pathogen balance may be altered (4). Thus, health and disease status of these animal populations are of prime importance for the purpose of management and conservation.
Brucella spp. was first reported in true seals in 1994 (5) and Brucella pinnipedialis has since been isolated from numerous true seal species (6). Persistence in macrophages—causing chronic infections—is the hallmark of brucellosis (7). However, B. pinnipedialis isolated from harbor (Phoca vitulina vitulina) and hooded seals (Cystophora cristata), both true seal species, did not multiply in vitro in human, murine or hooded seal macrophages, or in human and hooded seal epithelial cells (8–10) suggesting it may be a less virulent Brucella subspecies with a lower zoonotic potential. Furthermore, hooded and harbor seal brucellae are attenuated in the BALB/c mouse model (11, 12), in contrast to virulent pathogenic terrestrial brucellae, such as Brucella suis, which show a great ability to multiply and persist in this model (12). Brucella infections are further characterized by bacterial replication in the reproductive system of primary hosts, associated with pathology in the reproductive organs, causing abortion and sterility (7). Interestingly, although B. pinnipedialis has often been detected, pathology associated with it in true seals is virtually absent (6).
Previous studies on hooded seals from the east side of Greenland (13) and harbor seals from Alaska and the East coast of the USA (14, 15) have shown an age-dependent serological pattern, with a low probability of being seropositive for pups, a higher probability for yearlings, followed by a decreasing probability with age. This indicates that exposure occurs during the first year of life rather than in utero with a subsequent clearing of the infection (13). However, whether a similar age-dependent serological pattern is present in other Alaskan seal species and populations had not been documented.
In contrast to the situation in true seals, brucellae are rarely isolated or detected by PCR in eared seals, making it difficult to evaluate the presence or absence of Brucella-associated pathology in these species. However, it is worth noticing that the few cases reported in eared seals have been associated with reproductive pathology (16–18) and that transplacental transmission has been suggested (16). Furthermore, certain eared seal species are able to host infections with the zoonotic strain type (ST) 27 (16) and terrestrial brucellae (19), and could hence pose a zoonotic risk.
Serologic tests for detecting antibodies against a specific etiologic agent are the first screening tools for wildlife. The Rose Bengal test (RBT) is a simple and reliable test recommended by the World Organization for Animal Health (OIE) for the detection of Brucella antibodies. However, when using it in marine mammals, fat globules being wrongly identified as agglutinates may interfere with the interpretation of the results (20, 21). Serum lipids may be partly removed by chloroform cleanup (20) and has previously been shown to greatly improve the agreement between RBT and an enzyme-linked immunosorbent assay (ELISA) when detecting Brucella antibodies (21). The first objective of the present study was to compare the results of RBT and ELISA in a subset of serum samples before and after chloroform cleanup. Thereafter, the second objective was to use the best technique to determine the seroprevalence of Brucella antibodies adjusting by other potential covariates.
For the remaining screening, we used ELISA to investigate the seroprevalence of Brucella antibodies in a large number of harbor seals, spotted seals, ribbon seals, ringed seals, Steller sea lions, and Northern fur seals from Alaskan waters, sampled between 1975 and 2011. The aim of the present study was to analyze how the likelihood of seropositivity varied between species, sex, age, sampling year, and regions in order to draw further conclusions on whether a Brucella infection may be negatively impacting the health and population dynamics of these species. Knowledge about to what degree these species harbor the infection is also of importance as many of these species are subsistence harvested and hence may pose a zoonotic threat.
Materials and Methods
Sampling
Samples were collected (1975–2011) from Alaskan pinnipeds by biologists during live/capture release studies, scientific collections or Alaska native subsistence harvested animals and stored at −40 to −80°C at the Alaska Department of Fish and Game (ADF&G) in Fairbanks, Alaska until subsampled for this study (n = 1,643). Sample sizes and distribution by sex and age category (pups; <1 year, juveniles; 1–3 years, adults; >3 years) are depicted in Table 1. Age category was known for 1,420 seals (86%); 1,039 harbor seals (93%), 73 spotted seals (86%), 50 ribbon seals (91%), 75 ringed seals (50%), 46 Steller sea lions from the Western distinct population segment (61%), 31 Steller sea lions from the Eastern distinct population segment (65%), and 106 Northern fur seals (99%). Age by year was determined by assessing morphometric measurements (22), tooth annuli [e.g., (23)] or claw annuli [e.g., (24)] as validated for each species. Age by year was determined for 916 seals (56%): 599 harbor seals (53%, 0–30 years), 58 spotted seals (68%, 0–25 years), 49 ribbon seals (89%, 1–25 years), 75 ringed seals (50%, 0–16 years), 28 Steller sea lions from the Western distinct population segment (37%, 0 – 10 years), 15 Steller sea lions from the Eastern distinct population segment (31%, all pups), and 93 Northern fur seals (87%, all pups). The animals included in the study were from Alaskan waters (Figure 1) and seven ringed seals were from Argo Bay, Canada.
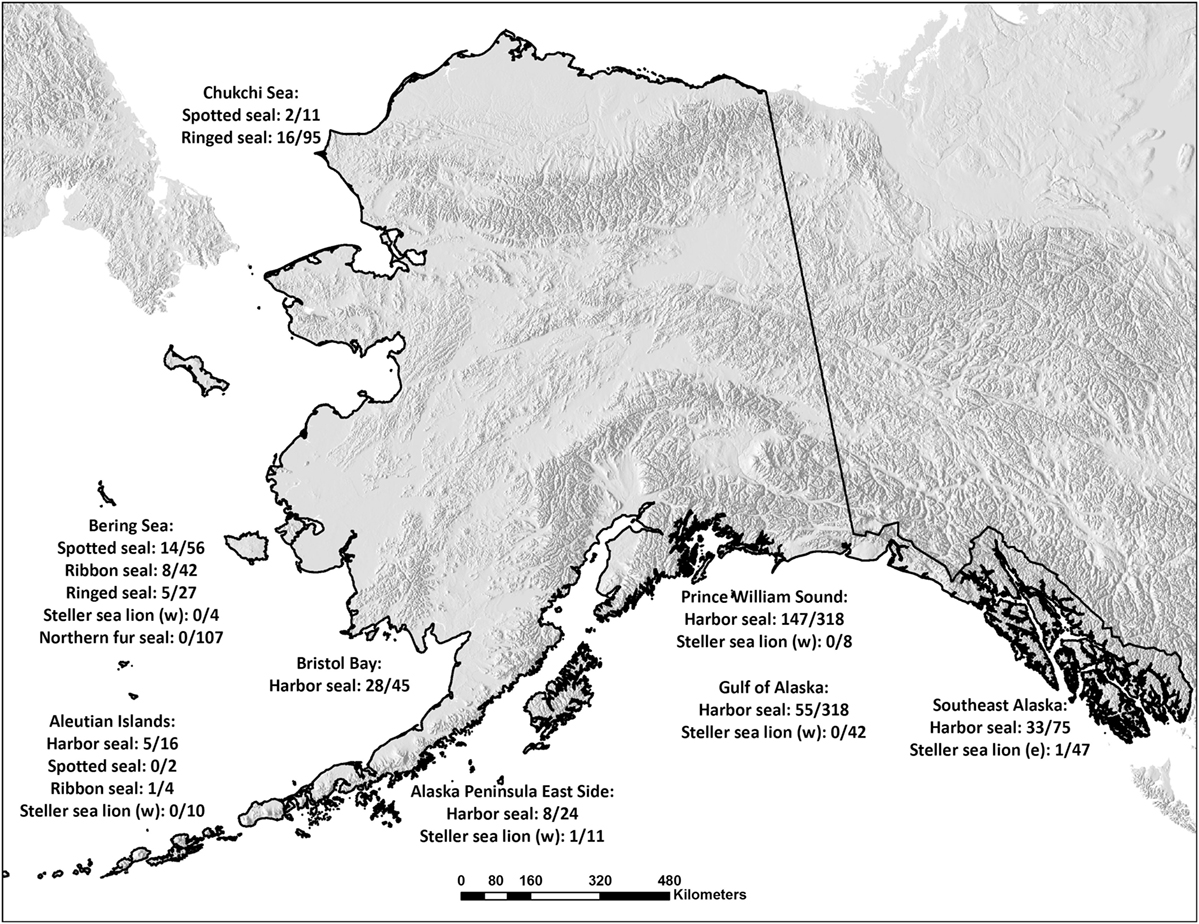
Figure 1. Number of Brucella-seropositive seals per species and sampling area. Serum samples obtained from Alaskan harbor seals, spotted seals, ribbon seals, ringed seals, Steller sea lions from the Western (w) and Eastern (e) distinct population segments, and Northern fur seals in the Chukchi and Bering Seas, in Bristol Bay, around the Aleutian Islands, on the Eastern side of the Alaska Peninsula, in Prince William Sound, in the Gulf of Alaska, on the coast of Southeast Alaska and in Argo Bay, Canada. The samples were investigated for the presence of Brucella antibodies and the numbers of positives per species and sampling spot are given.
Antibody Detection
Serum samples were analyzed for Brucella antibodies using a Protein A/G ELISA, as previously described (25). A subsample of sera was also tested using RBT (IDEXX Laboratories, Pourquier, Hoofddorp, the Netherlands) (20 ELISA-negatives/species, n = 140, and up to 20 ELISA-positives/species, n = 66). These sera (n = 206) were cleaned with chloroform to remove lipids (20, 21) and re-analyzed by ELISA (ELISAchl) and RBT (RBTchl).
Statistical Analysis
Pairwise agreement among the serological tests before and after chloroform cleanup was assessed (Cohen’s kappa, κ) (26, 27). RBT and RBTchl results were categorized as negative, positive, or impossible to interpret. ELISA and ELISAchl results were categorized as negative or positive. The remaining statistical analyses were performed using ELISA results.
Differences in seroprevalence between sex and age groups were estimated using generalized linear models with a binomial error distribution and a logit link. To account for the possibility that age effects may differ between males and females, the interaction age × sex was included in the models. Influence of age was analyzed in two ways: first, age was treated as a categorical variable with three age categories: pups, juveniles, and adults. Second, for animals older than pups, we used age as a continuous variable. Each species was modeled separately instead of using “species” as a covariate to reduce the number of model parameters.
We limited the examination of how seropositivity varied spatially to harbor seals because this was the only species with a sufficient sample size from all sampling regions (Figure 1), with exception of the Aleutian Islands, which was excluded from the analysis. Region was modeled as a categorical variable. Age category and sex, as well as their interactions, were included as covariates in the full model. Not all regions, age categories, sex, and species, were sampled all years. Hence, we chose not to include sampling year as a covariate as preliminary analysis indicated that this could bias estimates, in particular for species with small sample sizes. However, a univariate analysis of trends in seroprevalence over time did not reveal any overall trend (logit regression, slope = 0.00, 95% CI = −0.02, 0.02).
The most parsimonious models where selected using Akaikes information criterion corrected for small sample sizes (AICc), using an all possible regression approach [library MuMIn in R (28)] on the sample size for all possible combinations (i.e., a fixed minimum sample size for all models ranked). Estimates are considered significant if their 95% confidence intervals do not include zero. All statistical analyses were performed in the program R, version 3.3.0 [R (29)].
Results
Results from testing sera without chloroform cleanup (i.e., containing lipids), revealed moderate agreement between ELISA and RBT (κ: 0.66, SE: 0.04). Chloroform cleanup greatly improved agreement between the ELISA/ELISAchl (identical results) and RBTchl (κ: 0.90, SE: 0.03), while the agreement between RBT and RBTchl was only moderate (κ: 0.68, SE: 0.04). Detailed information about how the chloroform cleanup affected the results are available in Table 2. The remaining results are ELISA results.
Brucella antibodies were detected in 276/1,122 harbor seals (24.6%), 16/85 spotted seals (18.8%), 9/55 ribbon seals (16.4%), and 21/150 ringed seals (14.0%). Brucella antibodies were detected in 2/124 Steller sea lions, both of unknown age, one from the Western distinct population segment (1.3%) and one from the Eastern distinct population segment (2.1%). All 107 Northern fur seals were seronegative.
For harbor and ringed seals, juveniles had a higher probability of being seropositive than pups, and adults for harbor seals (45.3%, 95% CI = 40.0, 50.9 versus 7%, 95% CI = 4.2, 10.6 and 19.4%, 95% CI = 16.1, 23.1, and 56.3%, 95% CI = 32.3, 78.2 versus 14.3%, 95% CI = 0.9, 49.4 and 3.8%, 95% CI = 0.6, 11.4), though for the ringed seals the contrast to pups was not significant (Figure 2; Table 3; Table S1 in Supplementary Material). There was no such significant age category pattern evident for spotted or ribbon seals in the best approximating models (Figure 2; Table 3). While lower ranked models for both species included age category (δAICc < 2, Table S1 in Supplementary Material), these estimates did not significantly differ (95% CI for contrasts of pups and adults versus juveniles for spotted seal: [−4.71, 0.19] and [−2.75, 0.27], and for adults versus juveniles for ribbon seal: [−1.17, 1.95], all logit-transformed).
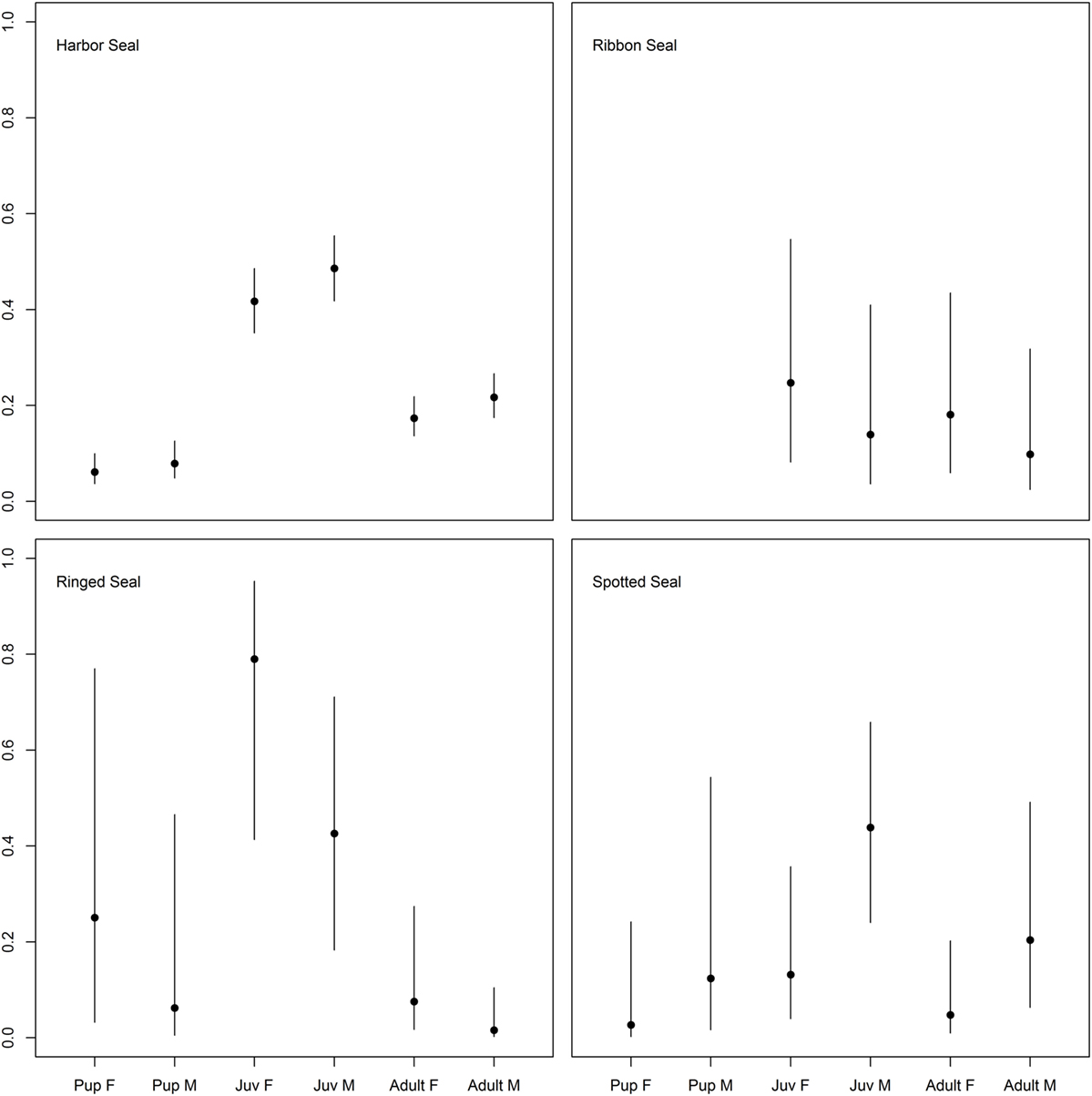
Figure 2. Differences in probability of being seropositive between age categories in true seals. Differences in probability of being Brucella spp. seropositive among age categories (pups < 1 year, juveniles 1–3 years, adults > 3 years) and sexes (males; M and females; F) for the four true seal species. Points indicate predicted mean, while error bars indicate 95% confidence intervals.
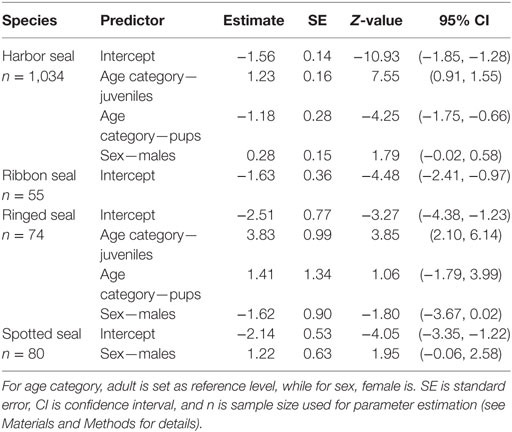
Table 3. Parameter estimates (logit-transformed) for best approximating models, with age is included as a categorical predictor.
When splitting up age categories into age by year, there was an overall significant decreasing probability of being seropositive with age from the age of 1 year for all true seals except ribbon seals (Figure 3; Table 4; Table S2 in Supplementary Material). While seropositive animals were absent among individuals older than 5 years in spotted seals and 6 years in ringed seals, they were present among harbor seals until the age of 16, though at a very low prevalence for older ages (Figure 3).
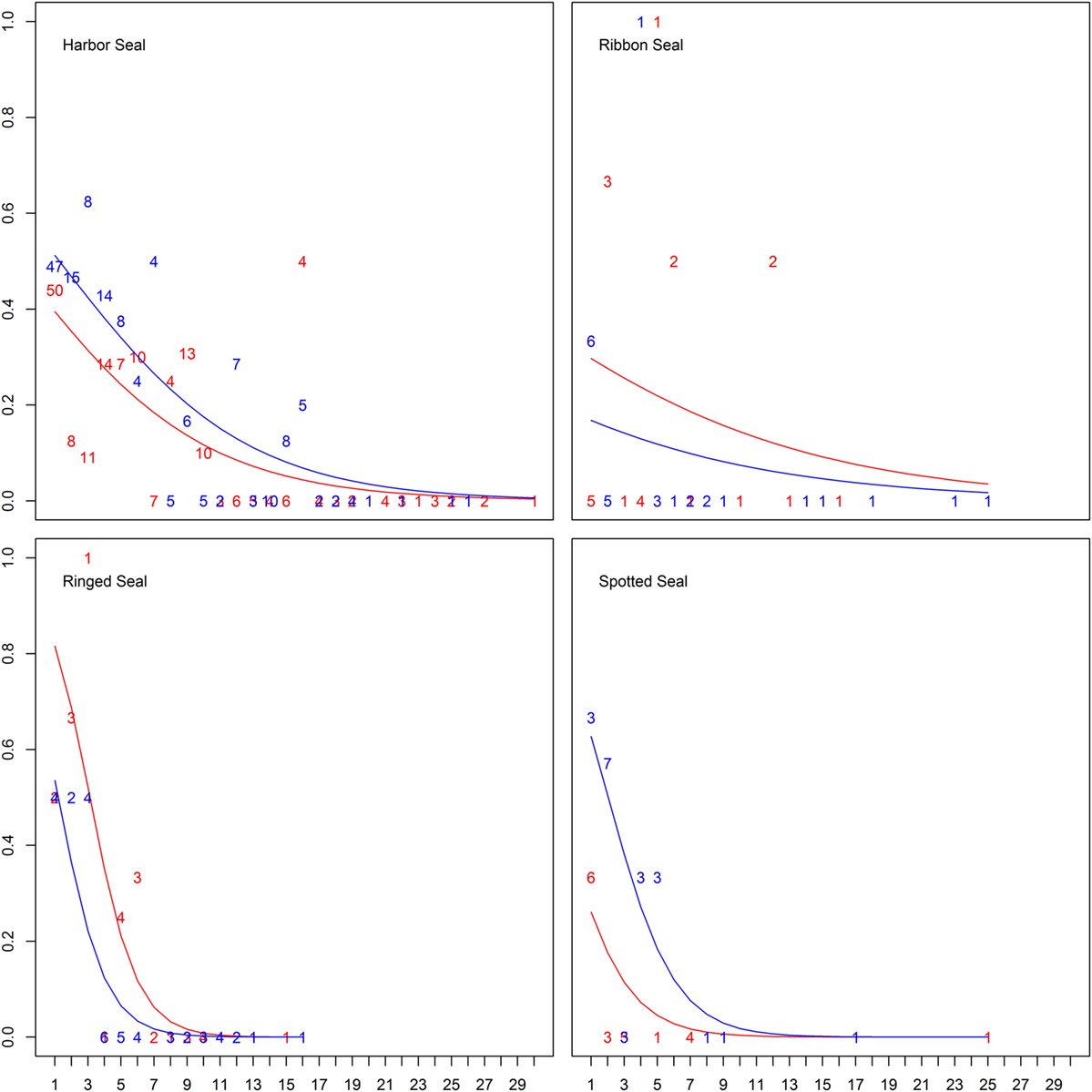
Figure 3. Differences in probability of being seropositive between ages in years for true seals. Differences in probability of being Brucella spp. seropositive among four true seal species at different age and sex (males in blue, females in red). Lines show the predicted probabilities, while numbers indicate empirical frequencies for different ages.
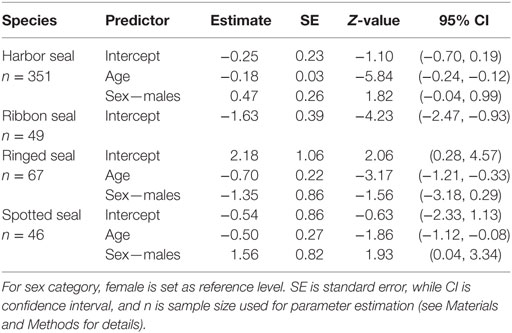
Table 4. Parameter estimates (logit-transformed) best approximating models, when age is included as a continuous predictor (for animal equal to, or older than 1 year).
There was an inconsistent pattern for difference in probability of seropositivity between sexes (Tables S1 and S2 in Supplementary Material). Spotted seal males had a significant and harbor seal males a near significant higher probability of being seropositive, while the pattern was the opposite for ringed seals (Figures 2 and 3; Tables 3 and 4). Like ringed seals, ribbon seal showed higher probability for seropositivity among females, though not significant [95% CI = [−0.66, 2.54] and [−0.78, 2.44], for lower ranked age category (δAICc = 1.4) and age models (δAICc = 1.2), respectively].
Differences were found in seropositivity among regions (δAIC = 9.2 to the best model not including region, controlled for age category and sex), mainly due to a significant lower probability of being seropositive for harbor seals in the Gulf of Alaska (Figures 1 and 4).
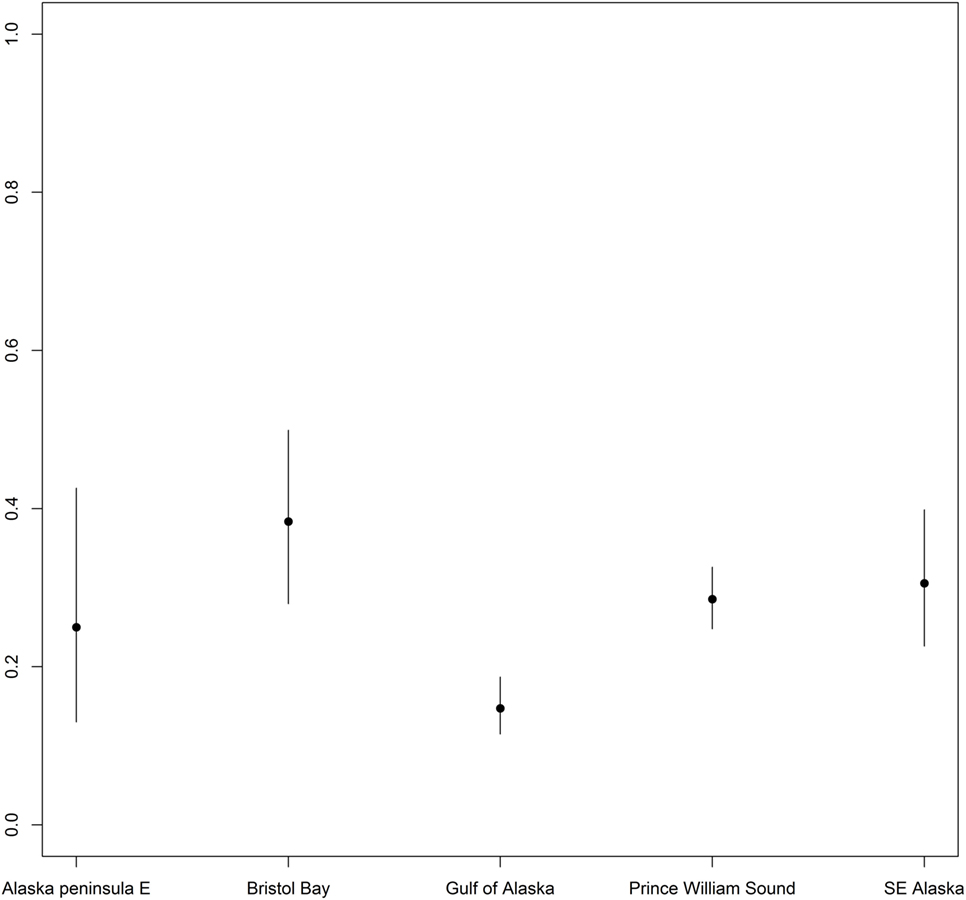
Figure 4. Differences in probability of being seropositive between harbor seals sampled at various regions. Differences in probability of being Brucella spp. seropositive between harbor seals sampled at various regions in Alaska. Points indicate predicted mean for all age groups, while error bars indicate 95% confidence intervals.
Discussion
Past evaluations of Brucella infection status in marine mammals have likely been inaccurate if the results were based solely on agglutination tests. RBT is a much used, simple, cheap, and reliable test recommended by the OIE (30); however, the results may be influenced by the presence of hemolysis and globules of fat (21), often present in marine mammal sera. For marine mammals, it is necessary to run a combination of tests to determine if discrepancies are found between RBT and other serological tests. A chloroform cleanup may be required, followed by repetition of the tests, as shown in the present study where the agreement between ELISA and RBT was moderate, while after chloroform cleanup, the coherence between ELISA and RBTchl was greatly improved. The improved agreement between the tests and the reduced number of non-interpretable RBT-results following chloroform cleanup indicate that the original serum quality likely contained lipids that reduced the accuracy of the initial RBT-outcome.
Even with serological techniques that are less sensitive to poor serum quality and lipemic serum (e.g., ELISA), positive serological results presume exposure (31), as serological cross-reactions and false positives bias (i.e., inflate) the results. Several potential agents cross-reacting with Brucella are identified (32), however, little is known about their presence in wildlife. Therefore, the gold standard in brucellosis diagnostics is bacterial isolation or detection of Brucella spp. specific DNA-fragments. Ideally serological tests should be used to screen sera and seropositives should be further tested by bacterial isolation and PCR to address Brucella status among Alaskan pinnipeds in the future.
An age-dependent serological pattern, with a low probability of being seropositive for pups, a higher probability for yearlings, followed by a decreasing probability with age, previously identified in hooded (13) and harbor seals (14, 15) was identified in harbor seals in the present study, and similar patterns were present also for the other true seal species, however, solid conclusions could not be made due to sample size. Harbor and ringed seal juveniles had a higher probability of being seropositive than pups and adults. Likewise, when analyzing juveniles and adults by exact ages, there was a falling probability of seropositivity with age for harbor, spotted and ringed seals. Seropositive animals were lacking among individuals older than 5 years in spotted seals and 6 years in ringed seals, but were present among harbor seals until the age of 16, though at a very low prevalence. Almost all seropositive ribbon seals were between 1 and 6 years, though one was 12 years. The lack of significant results for ribbon seals, although the same trend was present, is likely due to the small number of animals sampled, and the lack of pups among the sampled animals. These results suggest that the investigated true seal species may be clearing the infection with increasing age.
Seals have an endotheliochorial placenta where 5–10% of the maternal antibodies are transferred to the fetus in utero and the rest are transferred through the colostrum. The immunity transmitted is determined by the level of systemic immunity in the mother (33). The low numbers of seropositive harbor, spotted, and ringed seal pups in this study indicates that these (1) have not received maternal antibodies against Brucella and (2) are not exposed to brucellae and hence have not mounted an antibody response yet. The low seroprevalence among pups is consistent with our finding that the majority of the females had no detectable levels of Brucella antibodies by the time they reached sexual maturity.
Brucella spp. in terrestrial animals causes reproductive pathology and may be transmitted during breeding and lactation or by crossing the placenta from mother to offspring (34). However, reproductive pathology is not associated with B. pinnipedialis infections in true seals and vertical transmission of B. pinnipedialis has never been described in a true seal species (6). The limited number of serologically and bacteriologically positive true seals, of reproductive age, in previous studies (13, 15, 35, 36), and the herein presented age-dependent serological patterns, further indicates that maternal transmission is unlikely as females have become seronegative for Brucella by the time they reach sexual maturity. Furthermore, the mean probability of being seropositive increased from pups to juveniles in previous studies (13–15) as well as in this study, suggesting that exposure to B. pinnipedialis is primarily during the post-weaning period and during the first few years of life, and is not transmitted in utero or to neonates.
Although underlying reason for this age-related serological pattern is unknown, it may be related to changes in diet. Stable isotopes and mercury biomarkers have indicated that in general, adult harbor and ringed seals feed at a higher trophic level than pups (37). Stable isotope analysis has shown that ribbon and spotted seals also feed at increasing trophic levels with age (38). Hence, there may be a general shift in diet composition toward higher trophic levels with increasing age, which coincides with the age at which seropositive juveniles start to appear, indicating a possible reservoir of B. pinnipedialis in one or more lower trophic level prey species.
Analysis by region showed a decrease in the probability of being seropositive for harbor seals in the Gulf of Alaska compared to the other regions. At Nanvak Bay, the largest haul-out in northern Bristol Bay, harbor seals declined in abundance between 1975 and 1990, but have increased since (2). Samples from harbor seals were from the time period 1975 to 2001, however, as different sites were sampled different years, we chose not to include year as a covariate in the statistical analysis. Still, univariate analysis of trends in seroprevalence over time did not reveal any overall trend suggesting that the Brucella infection in the harbor seal population in Bristol Bay may not contribute to higher mortality rates.
Considering the lack of impact on the harbor seal population trends, the age-dependent serological and bacteriological patterns (13–15, 35, 36), the lack of Brucella-associated pathology in true seals (6) and the lack of multiplication in established in vitro (8–10) and in vivo models (11, 12), it is possible that true seals may not be the primary hosts of B. pinnipedialis, but rather a spillover host. B. pinnipedialis has been isolated from lungworms in seals (35) and a recent experimental infection showed that a B. pinnipedialis hooded seal strain survived in Atlantic cod (Gadus morhua) (39). Moreover, a novel Brucella strain has been isolated from a fish, a bluespotted ribbontail ray (Taeniura lymma) (40). In addition, Brucella microti has been isolated from soil (41) and novel brucellae strains have been isolated from frogs (42–44), indicating an extended ecological niche of brucellae. Further investigation of marine sources for exposure to B. pinnipedialis should be performed in order to further reveal the epizootiology of Brucella infection in true seals.
The infection pattern in eared seals seems to be very different from that found in true seals. We detected Brucella antibodies in only two Steller sea lions, and none of the Northern fur seals. These findings are consistent with the low number of Brucella isolates obtained from eared seals in other studies; four bacteriology positive California sea lion placentas (16, 17), of which two showed signs of inflammation and multifocal acute necrosis (17). Additionally, transplacental transmission of brucellae in California sea lions has been indicated when brucellae strains belonging to the zoonotic ST27 were detected by PCR in three placentas and multiple fetal tissues in parallel (16). Terrestrial brucellae of unknown origin have also been detected by PCR in blood and milk from two apparently clinically healthy wild California sea lions, and marine mammal brucellae were detected in blood and milk from one animal (19). B. pinnipedialis has also been detected by PCR in six Northern fur seal placentas, of which one had severe placentitis (18), and in one Northern fur seal spleen with no pathology associated (45). The low number of isolates and PCR-positive cases obtained from eared seals make drawing any conclusions regarding the presence or absence of pathology in these species difficult, however, it is worth noticing that the few cases reported have often been associated with pathology in the reproductive organs (16–18) and that transplacental transmission has been suggested (16). The low seroprevalences detected in eared seals of all ages in the present study suggests a low level of exposure due to possibly a different diet or a greater resistance toward the infection. Considering the reports of pathology in eared seals, morbidity and/or mortality due to infection is also possible. Further studies, including samples suited for bacterial isolation and/or PCR and from a higher number of individuals from different age groups, are needed to determine to what degree the infection is a threat to the Alaskan eared seal populations.
Certain eared seal species are able to host infections with the zoonotic ST27 (16) and terrestrial brucellae (19). There have been three cases of naturally acquired infections in humans with ST27, none of which had been in contact with marine mammals; however, they had been at the coast, eaten raw shellfish (46) or been in contact with raw fish bait (47). Further studies on both marine mammals and other species from the Arctic marine ecosystem are warranted in order to address this important issue, especially as marine mammals and other marine species are used for human consumption. Whether the zoonotic ST27 is present in Alaskan waters is currently unknown and warrants further investigation; however given the ample opportunities for transfer from marine mammals to humans, it appears that if ST27 were present more cases would be known.
In conclusion, the Brucella serological pattern is very different for true and eared seals. The infection in true seals seems to be relatively common, yet shown in the present study to be transient and decreasing with increasing age for harbor seals, becoming virtually absent at the age of sexually maturity. Similar patterns were present also for the other true seal species; however, firm conclusions could not be made due to sample size. This suggests that true seals may not be the primary hosts of B. pinnipedialis, but rather a spillover host susceptible to infection from other sources in the marine environment. In eared seals, we detected only two seropositive animals which could be explained by a low level of exposure or lack of susceptibility to infection; however, it could also be explained by high susceptibility to Brucella infection with mortality removing infected animals from the population. Comparison of true and eared seal Brucella isolates with established bacteriological and molecular methods (6) could provide new information about their potential differences and similarities. Furthermore, the pathogenicity of isolates should be compared to already characterized terrestrial Brucella strains in established in vitro cell (7) and in vivo mouse (12) Brucella models.
Ethics Statement
This study was carried out in accordance with the recommendations of four Institutional Animal Care and Use protocols approved by two separate committees. These protocols were approved by the Alaska Department of Fish and Game’s Institutional Animal Care and Use Committee (protocols #06-16, 0921, #03-0014, 09-08, and #2010-13R) and by the University of Alaska Fairbanks’ committee (protocol #98-23).
Author Contributions
Acquisition of samples: LQ and KB. Design of study: IN, KB, and JG. Laboratory testing: IN. Statistical analysis: RR. Interpretation of data: IN and RR. Drafting the work or revising it critically for important intellectual content: IN, RR, AL, MT, LQ, KB, and JG. All authors have read and approved the final manuscript.
Conflict of Interest Statement
The authors declare that the research was conducted in the absence of any commercial or financial relationships that could be construed as a potential conflict of interest.
Acknowledgments
We are grateful for the willingness of the marine mammal subsistence hunters of Alaska to share samples with us for studies like this and for the many field biologists and collaborators for collecting the samples including K. Frost, G. Sheffield, M. Nelson, R. Merrick, J. Sease, and R. Zarnke. S. Crawford and A. Bryan facilitated the permitting and international shipping to get the samples to Norway. E. M. Breines and E. Hareide, Arctic Infection Biology, UiT – The Arctic University of Norway, are thanked for excellent laboratory support and N. Pamperin, ADF&G and S. Hansen for graphical assistance. We thank S. E. Scotter, Arctic Infection Biology, UiT – The Arctic University of Norway, for language editing. All research and sample collection was conducted under the auspices of the Marine Mammal Protection Act permits issued by the National Marine Fisheries Service Office of Protected Resources. Live animal captures were conducted by methods approved by the Institutional Animal Care and Use Committees of ADF&G (protocol #06-16, 0921, #03-0014, 09-08, and #2010-13R) and University of Alaska Fairbanks (protocol #98-23).
Funding
Funding to collect, manage, and analyze the samples was provided by ADF&G, the National Marine Fisheries Service, U.S. Fish and Wildlife Service Tribal Grants, National Science Foundation, and the North Pacific Research Board.
Supplementary Material
The Supplementary Material for this article can be found online at http://www.frontiersin.org/articles/10.3389/fvets.2018.00008/full#supplementary-material.
References
1. Alaska Department of Fish and Game (ADF&G). Mammals. (2015). Available from: http://www.adfg.alaska.gov/index.cfm?adfg=animals.listmammals
2. Allen BM, Angliss RP; U.S. Department Commerce. Alaska Marine Mammal Stock Assessments, 2014. NOAA Technical Memorandum NMFS-AFSC-301. Seattle: National Oceanic and Atmospheric Administration, National Marine Fisheries Service, Alaska Fisheries Science Center (2015). p. 1–313. doi:10.7289/V5NS0RTS
4. Burek KA, Gulland FMD, O’Hara TM. Effects of climate change on Arctic marine mammal health. Ecol Appl (2008) 18(2):126–34. doi:10.1890/06-0553.1
5. Ross HM, Foster G, Reid RJ, Jahans KL, MacMillan AP. Brucella species infection in sea-mammals. Vet Rec (1994) 134(14):359–359. doi:10.1136/vr.134.14.359-b
6. Nymo IH, Tryland M, Godfroid J. A review of Brucella infection in marine mammals, with special emphasis on Brucella pinnipedialis in hooded seal (Cystophora cristata). Vet Res (2011) 42(93):42–93. doi:10.1186/1297-9716-42-93
7. von Bargen K, Gorvel JP, Salcedo SP. Internal affairs: investigating the Brucella intracellular lifestyle. FEMS Microbiol Rev (2012) 36(3):533–62. doi:10.1111/j.1574-6976.2012.00334.x
8. Larsen AK, Nymo IH, Boysen P, Tryland M, Godfroid J. Entry and elimination of marine mammal Brucella spp. by hooded seal (Cystophora cristata) alveolar macrophages in vitro. PLoS One (2013) 8:e70186. doi:10.1371/journal.pone.0070186
9. Larsen AK, Nymo IH, Briquemont B, Sørensen KK, Godfroid J. Entrance and survival of Brucella pinnipedialis hooded seal strain in human macrophages and epithelial cells. PLoS One (2013) 8:e84861. doi:10.1371/journal.pone.0084861
10. Larsen A, Godfroid J, Nymo IH. Brucella pinnipedialis in hooded seal (Cystophora cristata) primary epithelial cells. Acta Vet Scand (2016) 58:9. doi:10.1186/s13028-016-0188-5
11. Nymo IH, das Neves CG, Tryland M, Bårdsen B-J, Santos RL, Turchetti AP, et al. Brucella pinnipedialis hooded seal (Cystophora cristata) strain in the mouse model with concurrent exposure to PCB 153. Comp Immunol Microbiol Infect Dis (2014) 37(3):195–204. doi:10.1016/j.cimid.2014.01.005
12. Nymo IH, Arias MA, Pardo J, Álvarez MP, Alcaraz A, Godfroid J, et al. Marine mammal Brucella reference strains are attenuated in a BALB/c mouse model. PLoS One (2016) 11(3):e0150432. doi:10.1371/journal.pone.0150432
13. Nymo IH, Tryland M, Frie AH, Haug T, Foster G, Rødven R, et al. Age-dependent prevalence of anti-Brucella antibodies in hooded seals (Cystophora cristata). Dis Aquat Organ (2013) 106(3):187–96. doi:10.3354/dao02659
14. Zarnke RL, Saliki JT, MacMillan AP, Brew SD, Dawson CE, Hoef JMV, et al. Serologic survey for Brucella spp., phocid herpesvirus-1, phocid herpesvirus-2, and phocine distemper virus in harbor seals from Alaska, 1976-1999. J Wildl Dis (2006) 42(2):290–300. doi:10.7589/0090-3558-42.2.290
15. Lambourn DM, Garner M, Ewalt D, Raverty S, Sidor I, Jeffries SJ, et al. Brucella pinnipedialis infections in Pacific harbor seals (Phoca vitulina richardsi) from Washington state, USA. J Wildl Dis (2013) 49(4):802–15. doi:10.7589/2012-05-137
16. Sidor I, Goldstein T, Whatmore AM, Zabka TS, Frasca S, Gulland FMD, et al. Transplacental infection with Brucella in California sea lion (Zalophus californianus) fetus. Brucellosis 2008 International Research Conference (2008).
17. Goldstein T, Zabka TS, Delong RL, Wheeler EA, Ylitalo G, Bargu S, et al. The role of domoic acid in abortion and premature parturition of California sea lions (Zalophus Californianus) on San Miguel Island, California. J Wildl Dis (2009) 45(1):91–108. doi:10.7589/0090-3558-45.1.91
18. Duncan CG, Tiller R, Mathis D, Stoddard R, Kersh GJ, Dickerson B, et al. Brucella placentitis and seroprevalence in northern fur seals (Callorhinus ursinus) of the Pribilof Islands, Alaska. J Vet Diagn Invest (2014) 26(4):507–12. doi:10.1177/1040638714532647
19. Avalos-Tellez R, Ramirez-Pfeiffer C, Hernandez-Castro R, Diaz-Aparicio E, Sanchez-Dominguez C, Zavala-Norzagaray A, et al. Infection of California sea lions (Zalophus californianus) with terrestrial Brucella spp. Vet J (2014) 202(1):198–200. doi:10.1016/j.tvjl.2014.06.021
20. Castro AR, Morrill WE, Pope V. Lipid removal from human serum samples. Clin Diagn Lab Immunol (2000) 7(2):197–9. doi:10.1128/CDLI.7.2.197-199.2000
21. Godfroid J, Beckmen K, Nymo IH. Removal of lipid from serum increases coherence between brucellosis rapid agglutination test and enzyme-linked immunosorbent assay in bears in Alaska, USA. J Wildl Dis (2016) 52(4):912–5. doi:10.7589/2015-11-298
22. King JC, Gelatt TS, Pendleton GW, Pitcher KW. A field-based method for estimating age in free-ranging juvenile Steller sea lions (Eumetopias jubatus). Mar Mammal Sci (2007) 23(2):262–71. doi:10.1111/j.1748-7692.2007.00108.x
23. Stewart REA, Stewart BE, Stirling I, Street E. Counts of growth layer groups in cementum and dentine in ringed seals (Phoca hispida). Mar Mamm Sci (1996) 12(3):383–401. doi:10.1111/j.1748-7692.1996.tb00591.x
24. McLaren IA. The biology of the ringed seal (Phoca hispida Schreber) in the Eastern Canadian Arctic. J Fish Res Board Can (1958) 118:12.
25. Nymo IH, Godfroid J, Åsbakk K, Larsen AK, das Neves CG, Rødven R, et al. A protein A/G indirect enzyme-linked immunosorbent assay for the detection of anti-Brucella antibodies in Arctic wildlife. J Vet Diagn Invest (2013) 25(3):369–75. doi:10.1177/1040638713485073
26. Cohen J. A coefficient of agreement for nominal scales. Educ Psychol Meas (1960) 20(1):37–46. doi:10.1177/001316446002000104
27. Cohen J. Weighted kappa: nominal scale agreement with provision for scaled disagreement or partial credit. Psychol Bull (1968) 70(4):213–20. doi:10.1037/h0026256
28. Kamil Barton. MuMIn: Multi-Model Inference. R Package Version 1.15.6. (2016). Available from: https://CRAN.R-project.org/package=MuMIn.
29. Core Team R. R: a language and environment for statistical computing. R Foundation for Statistical Computing. Vienna, Austria: (2017). Available from: https://www.R-project.org/
30. OIE. Bovine Brucellosis. In: Linnana S, Pearson JE, editors. OIE Manual of Diagnostic Tests and Vaccines for Terrestrial Animals. 6 ed. Paris, France: Office International des Epizooties (2009). p. 1–35.
31. Poester P, Nielsen K, Samartino LE, Yu WL. Diagnosis of Brucellosis. Open Vet Sci J (2010) 4:46–60. doi:10.2174/1874318801004010046
32. Alton GG, Jones LM, Angus RD, Verger JM. Techniques for the Brucellosis Laboratory. Paris, France: Institut National de la Recherche Agronomique (1988).
33. Stewart REA, Stewart BE. Female reproductive systems. 2nd ed. In: Perrin WF, Wursig B, Thewissen JGM, editors. Encyclopedia of Marina Mammals. Amsterdam, Boston, Heidelberg, London, New York, Oxford, Paris, San Diego, San Francisco, Singapore, Sydney, Tokyo: Elsevier (2009). p. 423–9.
34. Corbel MJ, Banai M. Genus Brucella Meyer and Shaw 1920. 2nd ed. In: Garrity GM, Krieg NR, Staley JT, James T, editors. Bergey’s Manual of Systematic Bacteriology. Volume two: the Proteobacteria (Part C). New York: Springer (2005). p. 370–85.
35. Garner MM, Lambourn DM, Jeffries SJ, Hall PB, Rhyan JC, Ewalt DR, et al. Evidence of Brucella infection in Parafilaroides lungworms in a Pacific harbor seal (Phoca vitulina richardsi). J Vet Diagn Invest (1997) 9(3):298–303. doi:10.1177/104063879700900311
36. Tryland M, Sørensen KK, Godfroid J. Prevalence of Brucella pinnipediae in healthy hooded seals (Cystophora cristata) from the North Atlantic ocean and ringed seals (Phoca hispida) from Svalbard. Vet Microbiol (2005) 105(2):103–11. doi:10.1016/j.vetmic.2004.11.001
37. Young BG, Loseto LL, Ferguson SH. Diet differences among age classes of Arctic seals: evidence from stable isotope and mercury biomarkers. Polar Biol (2010) 33(2):153–62. doi:10.1007/s00300-009-0693-3
38. Dehn LA, Sheffield GG, Follmann EH, Duffy LK, Thomas DL, O’Hara TM. Feeding ecology of phocid seals and some walrus in the Alaskan and Canadian Arctic as determined by stomach contents and stable isotope analysis. Polar Biol (2007) 30(2):167–81. doi:10.1007/s00300-006-0171-0
39. Nymo IH, Seppola M, Al Dahouk S, Bakkemo KR, Jimenez de Bagues MP, Godfroid J, et al. Experimental challenge of Atlantic cod (Gadus morhua) with a Brucella pinnipedialis hooded seal (Cystophora cristata) strain. PLoS One (2016) 1(1/7):e0159272. doi:10.1371/journal.pone.0159272
40. Eisenberg T, Risse K, Schauerte N, Geiger C, Blom J, Scholz HC. Isolation of a novel ’atypical’ Brucella strain from a bluespotted ribbontail ray (Taeniura lymma). Antonie Van Leeuwenhoek (2017) 110(2):221–34. doi:10.1007/s10482-016-0792-4
41. Scholz HC, Hubalek Z, Nesvadbova J, Tomaso H, Vergnaud G, Le Fleche P, et al. Isolation of Brucella microti from soil. Emerg Infect Dis (2008) 14(8):1316–7. doi:10.3201/eid1408.080286
42. Eisenberg T, Hamann HP, Kaim U, Schlez K, Seeger H, Schauerte N, et al. Isolation of potentially novel Brucella spp. from frogs. Appl Environ Microbiol (2012) 78(10):3753–5. doi:10.1128/AEM.07509-11
43. Fischer D, Lorenz N, Heuser W, Kampfer P, Scholz HC, Lierz M. Abscesses associated with Brucella inopinata-like bacterium in a big-eyed tree frog (Leptopelis varmiculatus). J Zoo Wildl Med (2012) 43(3):625–8. doi:10.1638/2011-0005R2.1
44. Whatmore A, Dale E-J, Stubberfield E, Muchowski J, Koylass M, Dawson C, et al. Isolation of Brucella from a White’s tree frog (Litoria caerulea). JMM Case Rep (2015) 2:1–5. doi:10.1099/jmmcr.0.000017
45. Duncan C, Dickerson B, Pabilonia K, Miller A, Gelatt T. Prevalence of Coxiella burnetii and Brucella spp. in tissues from subsistence harvested northern fur seals (Callorhinus ursinus) of St. Paul Island, Alaska. Acta Vet Scand (2014) 56(1):67. doi:10.1186/s13028-014-0067-x
46. Sohn AH, Probert WS, Glaser CA, Gupta N, Bollen AW, Wong JD, et al. Human neurobrucellosis with intracerebral granuloma caused by a marine mammal Brucella spp. Emerg Infect Dis (2003) 9(4):485–8. doi:10.3201/eid0904.020576
Keywords: harbor seal, ribbon seal, ringed seal, serology, spotted seal, Steller sea lion, Northern fur seal, disease
Citation: Nymo IH, Rødven R, Beckmen K, Larsen AK, Tryland M, Quakenbush L and Godfroid J (2018) Brucella Antibodies in Alaskan True Seals and Eared Seals—Two Different Stories. Front. Vet. Sci. 5:8. doi: 10.3389/fvets.2018.00008
Received: 10 November 2017; Accepted: 12 January 2018;
Published: 31 January 2018
Edited by:
Scott Napper, University of Saskatchewan, CanadaReviewed by:
Balamurugan Vinayagamurthy, National Institute of Veterinary Epidemiology and Disease Informatics (ICAR), IndiaJulio Alvarez, University of Minnesota, United States
Copyright: © 2018 Nymo, Rødven, Beckmen, Larsen, Tryland, Quakenbush and Godfroid. This is an open-access article distributed under the terms of the Creative Commons Attribution License (CC BY). The use, distribution or reproduction in other forums is permitted, provided the original author(s) and the copyright owner are credited and that the original publication in this journal is cited, in accordance with accepted academic practice. No use, distribution or reproduction is permitted which does not comply with these terms.
*Correspondence: Ingebjørg H. Nymo, aW5nZWJqb3JnLm55bW9AdmV0aW5zdC5ubw==