- Department of Oncology, Brighton and Sussex University Hospitals, Brighton, United Kingdom
Cytotoxic DNA damaging chemotherapy brings clinical benefits in the treatment of many metastatic malignancies. However routine curative treatment remains restricted to a small number of malignancies including acute leukemia, high grade lymphoma, germ cell tumors, gestational malignancies and some of the rare childhood cancers. The detailed explanation for this dramatic divergence in outcomes remains to be elucidated. However, we have previously argued that there is a strong correlation between presence of the unique genetic events of immunoglobulin gene variable/diversity/joining (VDJ) recombination, somatic hypermutation (SHM), meiosis, nuclear fusion and gastrulation occurring in cells of origin of these malignancies and their high sensitivity to DNA damaging chemotherapy. In this study we have reviewed some of the basic physiological information relating to the specialized activity and sensitivity to DNA damage mediated apoptosis of normal cells undergoing these processes. In each of unique genetic events there are dramatic changes in apoptotic sensitivity. In VDJ recombination and somatic hypermutation over 95% of the cells involved undergo apoptosis, whilst in meiosis and nuclear fusion there are dramatic short term increases in the apoptotic sensitivity to DNA damage. It is apparent that each of the malignancies arising during these processes retains some of the unique phenotype associated with it. The impact of the physiological differences is most clearly seen in the two non-mutational malignancies. Gestational choriocarcinoma which arises shortly after nuclear fusion is routinely curable with chemotherapy whilst CIMP-positive ependymomas which is not linked to any of the unique genetic events is highly resistant. A similar pattern is found in a pair of malignancies driven by a single driver mutation. Infantile acute lymphoblastic leukemia (ALL) arises in a cell undergoing the early stages of VDJ recombination and has a 40% cure rate in contrast pediatric rhabdoid malignancy which is not linked to a unique genetic event responds very poorly to chemotherapy treatment. The physiological changes occurring in cancer cells at the time of the malignant transformation appear to have a major impact on the subsequent sensitivity to chemotherapy and curability. New therapies that impact on these pathways may be of therapeutic value.
Introduction and Hypothesis
The additional genetic changes that can occur in tumors after the development of malignancy have been extensively studied and considerable data indicates that tumor genetic heterogeneity is an important factor in resistance to many cancer therapies (Gerlinger et al., 2012; Alizadeh et al., 2015).
Whilst the majority of metastatic malignancies remain resistant to curative drug treatment, a number of rarer malignancies, comprising gestational choriocarcinoma, testicular and ovarian germ cell tumors, acute leukemia, high grade lymphoma, Hodgkin’s disease and some of the rare childhood malignancies have been routinely curable with cytotoxic chemotherapy drugs for more than 60 years (Hertz et al., 1956; Chabner and Roberts, 2005; DeVita and Chu, 2008).
The explanation for this dramatic divergence in curability between these relatively rare malignancies and the more common incurable metastatic malignancies has long been a subject of great interest and scientific debate (Savage et al., 2009; Pritchard et al., 2013; Housman et al., 2014; Romano et al., 2016).
Historically the rate of cell division and the development of mutations within the tumor have been regarded as two of the key features in determining sensitivity and resistance to cytotoxic chemotherapy (Dean et al., 2005; Mitchison, 2012; Holohan et al., 2013). More recently we have suggested that a further central component determines heightened chemotherapy sensitivity and cancer curability. The overview of the hypothesis is shown in Figure 1. This shows the persistence in the malignant cells of at least part of the unique phenotype that their transient cells of origin hold at the time of their malignant transformation (Savage, 2015). The result of this is to produce extreme sensitivity to DNA damaging chemotherapy and radiation in the resultant malignancies.
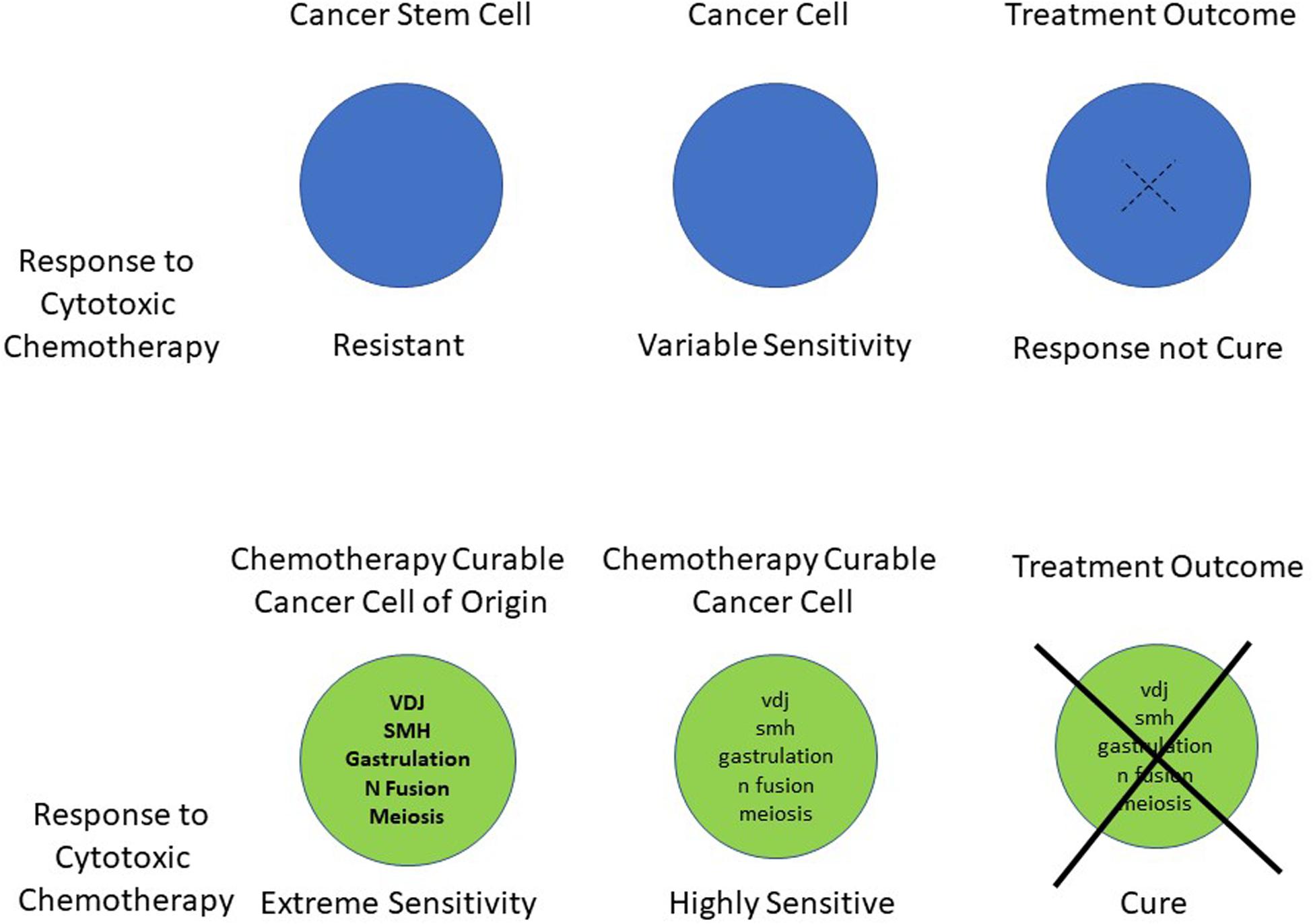
Figure 1. Schematic summary of the hypothesis indicating the unique genetic processes, which are associated with dramatic changes in apoptotic sensitivity. These occur in the cancer cells of origin and a remnant of this activity persists in their subsequent malignant cells. This ongoing phenotype leads to a heightened apoptotic response to DNA damaging chemotherapy.
Whilst the genetic event of mitosis is common to all human cells, the chemotherapy curable malignancies each appear to have a very close temporal association between their cell of origin and the physiological specialized unique genetic events. As shown in more detail in Table 1 one of the events of nuclear fusion, immunoglobulin VDJ gene recombination, immunoglobulin gene somatic hypermutation, meiosis or gastrulation is closely linked to the cell of origin of the each of the chemotherapy curable malignancies (Savage, 2015).
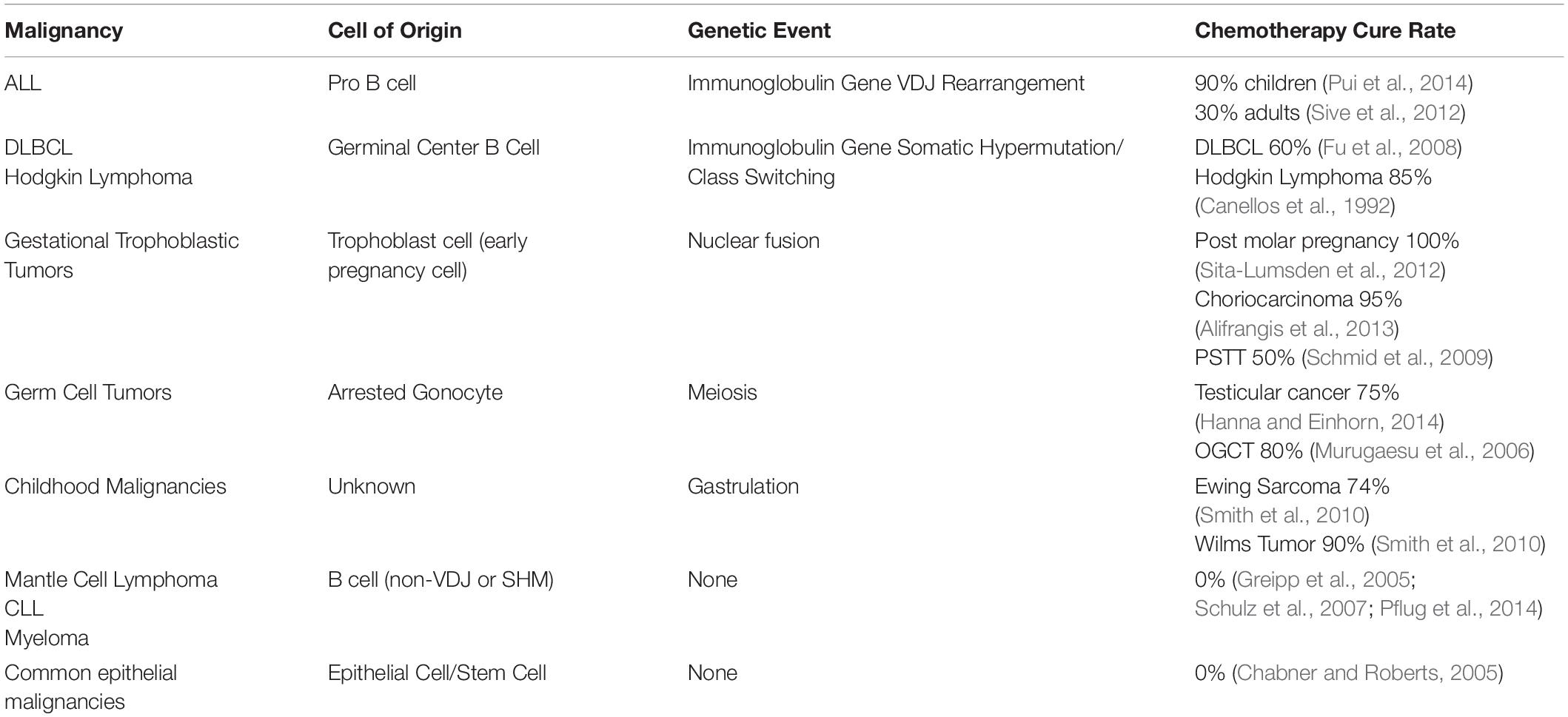
Table 1. Comparison of the chemotherapy curability for varying malignancies and their relationship with the unique genetic events.
There is increasing data to support the hypothesis that these malignant cells, retain components of the normally transient physiological changes occurring in their parent cells. As we will discuss in this review, it is apparent that the cells undergoing these unique genetic events transiently gain extremely high sensitivity to DNA damage mediated apoptosis. Retention of these profiles within the malignant cells appears to lead to heightened sensitivity to DNA damage induced apoptosis and hence curative cytotoxic chemotherapy treatment.
Cell of Origin, Malignant Transformation and Frozen Development
The concept that a malignant cell retains much of the characteristics of the cell of origin from which it has arisen is well established and the impact of this is most clearly illustrated in the lymphoid malignancies (Küppers et al., 1999). The most apparent feature of this developmental freezing in B cell malignancies is the static morphology and fixed antigenic markers that define the appearance and cell surface marker phenotype of the differing B cell malignancies (Shaffer et al., 2002).
As can be seen in Table 2, it is apparent that the malignancies arising along the B cell developmental pathway include a wide range of differing cell types and physiologies. These include acute undifferentiated leukemia, acute lymphoblastic leukemia, mantle cell lymphoma, diffuse large B cell lymphoma, Hodgkin’s lymphoma, chronic lymphocytic leukemia and myeloma. Each of these malignancies has very differing morphology, cell surface antigens, typical mutational rates, physiological characteristics and cytotoxic chemotherapy cure rates despite their common B cell ancestry. In addition to the fixed antigenic phenotype corresponding to the cell of origin, the malignant B cells also retain gene expression profiles that are very similar to that of the corresponding normal B cell parent (Andersson et al., 2005, 2010).
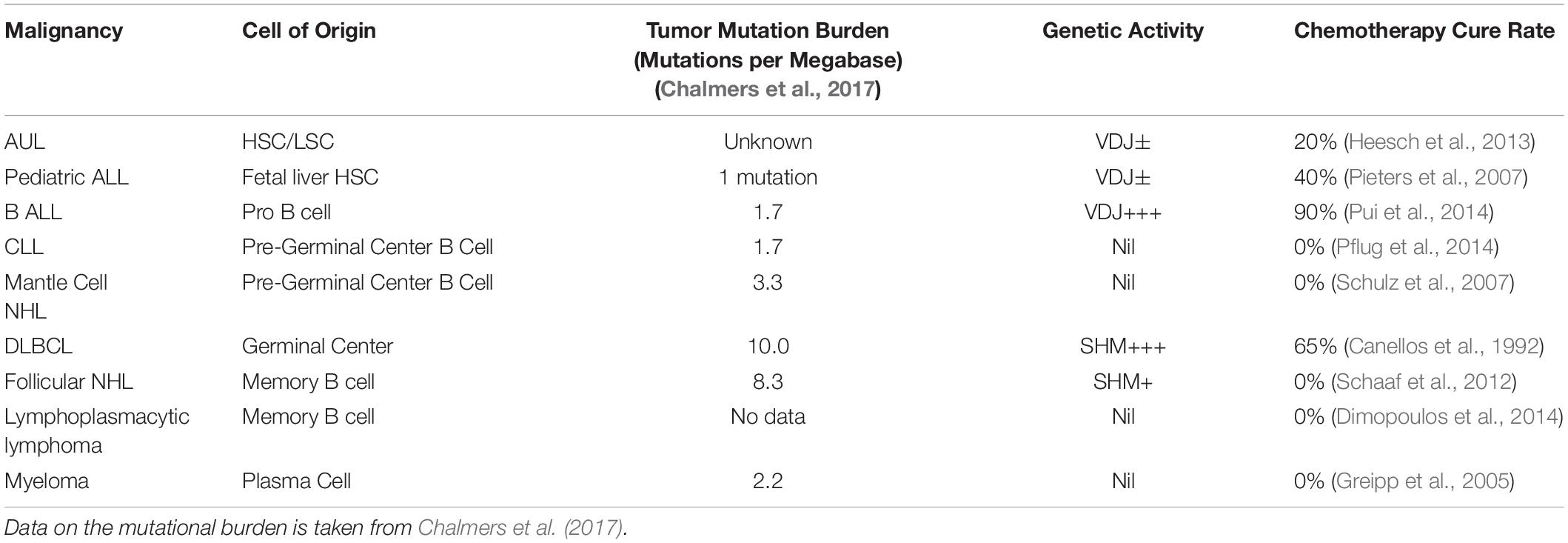
Table 2. Comparison of the cell of origin, mutational burden, genetic activity and chemotherapy cure rate for selected B cell malignancies.
A parallel pattern of frozen development is also seen in other malignancies that have linear developmental pathways. In the rarer diagnoses of T cell malignancies, acute T cell leukemia arise from cells at the earliest developmental point from the hematopoietic stem cell (De Bie et al., 2018), whilst the more indolent malignancies of Sezary syndrome and mycoses fungoides arise from mature effector T cells (Campbell et al., 2010).
Similarly, in the gestational trophoblastic malignancies, choriocarcinoma retains the phenotypic and methylation characteristics of a very early trophoblast cell (Mao et al., 2007; Savage et al., 2019). Whilst the less chemotherapy sensitive rarer malignancies of placental site trophoblastic tumor (PSTT) and epithelioid trophoblast tumor arise from more developmentally mature cells (Kurman et al., 1984).
Unique Genetic Events, Natural Physiological Changes, Impact on Apoptotic Sensitivity and Chemotherapy Curability
Acute B Cell Leukemia and VDJ Recombination
During the development pathway of normal B cells, the inherent sensitivity of the transient cells and their related malignancies to the induction of apoptosis via DNA damage varies dramatically. Within a short period of time developing B cells move from hematopoietic stem cells, which are inherently very resistant to DNA damage mediated apoptosis (Mohrin et al., 2010; Biechonski et al., 2018) to pro-B cells that can give rise to B-ALL.
The process of VDJ recombination of the immunoglobulin genes is the key defining feature of the early development phase of B cells and is the initial mechanism that allows the production of the width of antibody response from the limited pool of germ line immunoglobulin genes (Tonegawa, 1983).
The VDJ recombination process includes the cutting and re-joining of the immunoglobulin genes in a process involving the VDJ recombinase system (Oettinger et al., 1990). Within this process, the expression and activation of the key RAG1 and RAG2 enzymes is tightly controlled, occurring at significant levels only in B and T cells and is restricted to just a brief time in their overall cellular development pathway (Kuo and Schlissel, 2009).
The initiation of the VDJ phenotype and end of the VDJ process occur as a result of epigenetic changes very early in B cell and T cell development. The key components of the VDJ process, including the expression of RAG1, RAG2, DNTT (TdT) and ADA, are switched on early as the cells move from hemopoietic stem cell to common lymphocyte progenitor (CLP) and then are increased in width and intensity as cells move through the pro-B cell stage (Hystad et al., 2007).
Alongside the changes in gene expression there are also changes in the physical structure of the DNA encoding the immunoglobulin genes and their recognition sequences. These changes occur by alterations in the placement of nucleosomes that produce enhancement to the accessibility of the RAG recombinase to the immunoglobulin genes (Pulivarthy et al., 2016). These processes combine to focus VDJ activity predominantly to the immunoglobulin genes, although it is apparent that the process still retains significant risk of off target mutation and adverse oncogenic outcome (Tsujimoto et al., 1985; Schlissel et al., 2006).
In normal B cell development, the activity associated with the VDJ phenotype is then lost as the VDJ recombinase system is switched off as the cells move through to the stage of the immature B cell (Llorian et al., 2007). During the process of VDJ recombination, the B cell phenotype is characterized by an acquired balance between enhanced apoptotic pressures and competing survival signals. It is estimated, in murine studies, that cells undergoing VDJ recombination are dividing every 16 h (Opstelten and Osmond, 1983) and that 97% of these B cells die an apoptotic death at this stage (Liu et al., 1989; Osmond, 1991).
B-ALL is characteristically associated with a number of key mutations, including PAX5, IKZF1, TCF3 and EBF1. These mutations impact on the activity of genes associated with the regulation of normal B cell development (Inaba et al., 2013). Disruption of the activity of these genes can lead to a block in B cell differentiation and maturation and result in developmental arrest (Liu et al., 2014). The impact of this is to prevent the B cell either dying an apoptotic death or successfully maturing and moving through to the next stage in development.
There is appreciable data to indicate that the B cell malignancies arising at this part in their development also retain an ongoing degree of activity of the VDJ phenotype. A number of studies have reported that in B-ALL there is detectable ongoing activity of the VDJ system in the malignant cells (Bird et al., 1988; Yoneda et al., 1993; Beishuizen et al., 1994; Li et al., 2004; Gawad et al., 2012). As a result, it appears the pro B cell as it becomes frozen in its developmental phenotype by the onset of malignancy retains at some of the activity of the VDJ phenotype and its associated mechanisms including heighten apoptotic sensitivity.
Physiological Changes in Apoptotic Sensitivity During VDJ Recombination
There is a large amount of clinical data from the 1940s onward documenting the extreme sensitivity (Farber and Diamond, 1948) and subsequent routine curability for B-ALL to DNA damaging cytotoxic chemotherapy (Pinkel, 1971). However, there is relatively little work exploring the sensitivity to the induction of apoptosis by DNA damage in the cell of origin.
Whilst hemopoietic stem cells appear able to tolerate DNA damage and have a high threshold for the induction of apoptosis (Durdik et al., 2017) developing lymphocytes vary significantly in their sensitivity to DNA damage during the B cell development pathway.
An insight into the dramatic changes in the apoptotic threshold to DNA damage during B cell development is seen in a paper published in 1993 by Griffiths et al. (1994a). In this study the authors compared the in vitro sensitivity of IL-7 dependent B cells, which equate to pro-B cells, and that of other earlier and later developmental lymphoid lines to cytotoxic chemotherapy drugs. As shown in Figure 2 the cells at these differing developmental stages had markedly differing sensitivity to chemotherapy drugs. This is most clearly shown with etoposide where the 50% inhibitory dose (ID50) dose varies from 108 ng/ml for CFU, falls to 3.2 ng/ml for pre-B cells and then rises to 95 ng/ml for mature B cells (Griffiths et al., 1994a).
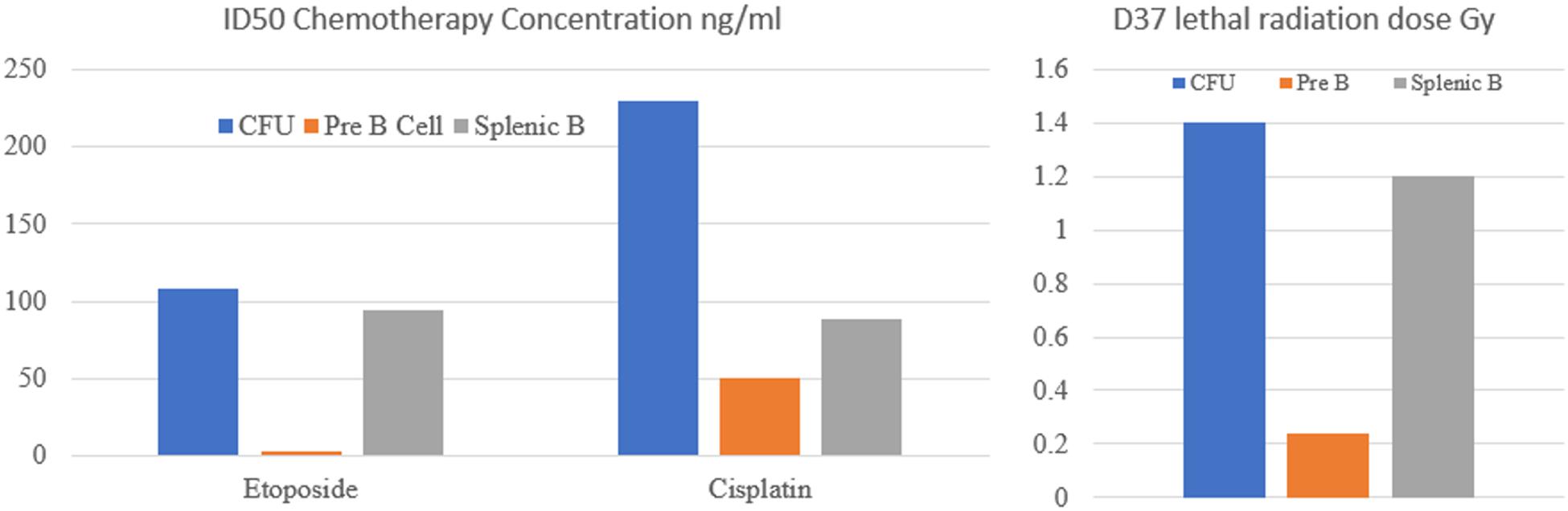
Figure 2. Sensitivity of colony forming units (CFU), pre-B cells and mature splenic B cells to cytotoxic chemotherapy drugs and radiation. ID50 is the drug concentration required to kill 50% of cells. D37 is the radiation dose (Gy) required to kill 37% of cells. It is apparent that Pre B cells are significantly more sensitive to DNA damaging agents than CFU or splenic B cells.
A parallel study examined the sensitivity of these B cells to radiotherapy also showed a similar pattern. The lethal radiation dose permitting 37% survival (D37) was 1.4Gy for the CFU, 0.24Gy for the pre-B cells and 1.2Gy for mature B cells (Griffiths et al., 1994b). Similar results were also reported examining the radio sensitivity of B cell lines of different developmental stages. The greatest sensitivity was seen in the pro B cell lines with greater resistance seen in both lymphocyte progenitor cells and also in B cells that have completed VDJ recombination (Uckun et al., 1991).
The Impact of the Timing of Oncogenesis on B ALL Chemotherapy Sensitivity Characteristics
Within the overall diagnosis of B-ALL, a number of key sub-types arise at slightly differing points in the early stages of B cell development. These malignancies have significantly differing clinical characteristics and also differing outcomes to cytotoxic chemotherapy treatment. The relationship of these diagnoses and their chemotherapy sensitivity appears to have a potential relationship to the developmental timing and the activity of the VDJ system at the time of malignant transformation.
Malignant transformation occurring very early in the development of the B cell pathway prior to the point at which B-ALL would arise can lead to the development of two relatively rare forms of leukemia, infantile ALL and acute undifferentiated leukemia.
The rare diagnosis of infantile ALL arises from the stem/progenitor cells present in the fetal liver (Agraz-Doblas et al., 2019) and has a chemotherapy cure rate of approximately 40% (Pieters et al., 2007). This malignancy is unusual in that it generally occurs as a result of a single mutation in the mixed lineage leukemia (MLL) gene (Biondi et al., 2000). Aside from this mutation the rest of the malignant cell’s genome appears otherwise mutationally bland (Dobbins et al., 2013; Andersson et al., 2015).
Review of the activity of the VDJ system in t(4;11) infant acute lymphoblastic leukemia indicates that whilst there is generally expression of TdT, a key enzyme in the VDJ system (Liu et al., 2004) but there is a low frequency of completed immunoglobulin and T-cell receptor gene rearrangements (Peham et al., 2002). As a result, it appears that this malignancy is likely to arise during the very earliest phase of VDJ recombination, when the VDJ phenotype associated apoptotic sensitivity is still evolving.
Acute undifferentiated leukemia is also rare and is characterized by an absence of lymphoid or myeloid lineage-specific antigens. Overall early genetic assessment of acute undifferentiated leukemia indicates a malignancy characterized by a stem-cell-driven gene expression pattern that lacks any of the common recurrent genotype aberrations (Heesch et al., 2013). More recent information indicates that mutations of genes linked to lymphocyte development are common and that there is expression of TdT, an early component of the VDJ process (Weinberg et al., 2019).
Clinically this malignancy carries a much poorer response to treatment and lower cure rate than the more frequent cases of B-ALL, with a reported cure rate in the region of 20% (Heesch et al., 2013). Genetic assessment of the VDJ status of this malignancy indicates that acute undifferentiated leukemia cells do not have clonal rearrangements of the B cell receptor indicating that these malignant cells arise prior to the commencement of effective VDJ recombination (Lao et al., 2019).
Conventional B-ALL in children has high cure rate approaching 90% (Pui et al., 2014) however in adults the cure rates are much lower, and the overall biology appears significantly different (Rowe, 2010). There are a number of possible explanations for this divergence in outcome including better tolerability of intensive chemotherapy in younger patients and the high prevalence of adverse prognostic factors in older patients. However, it is possible that differences in the underlying reduced intensity of the activity of the VDJ system in adults compared to children may also play an important role (Jensen et al., 2013a, b).
Whilst the full mechanisms for the enhanced sensitivity to chemotherapy treatment tracking in parallel with the VDJ phenotype is not yet detailed, it appears to be a strong association. In contrast the relationship between curability and the mutational load of these differing types of B cell malignancies appears much less strong as shown in Table 2.
Post VDJ Pre Somatic Hypermutation B Cell Malignancies
After the VDJ process is completed, the healthy B cell that has successfully recombined its immunoglobulin genes moves from a pro-B cell to an immature B cell. The VDJ process is completed and the phenotype moves away from the previously seen finely balanced apoptosis/survival pressures.
At this point in B cell development if malignancy occurs the resultant diagnosis can include mantle cell lymphoma or CLL (without hypermutated antibody variable regions) each of these whilst responsive to chemotherapy are not currently curable (Crespo et al., 2003; Fernàndez et al., 2010).
Germinal Center Derived B Cell Malignancies and Somatic Hypermutation
The B cells malignancies arising later at the germinal center stage of development include diffuse large B cell lymphoma, Hodgkin’s disease and Burkitt’s lymphoma. Each of these have high cure rates with cytotoxic chemotherapy (Sehn et al., 2007; Viviani et al., 2011; Todeschini et al., 2012).
Similarly, to the pro B cells undergoing VDJ recombination, the germinal center B cells are physiologically highly specialized and are undergoing a differing unique genetic event. At this developmental point B cells further alter the DNA sequence and structure of their immunoglobulin genes by undertaking somatic hypermutation of the antibody variable regions. Additionally, the cells also perform class switching in their immunoglobulin heavy chains (Jacob et al., 1991). These processes result primarily from the action of the enzyme activation-induced cytidine deaminase (AID), which deaminates cytidines to uridines within the immunoglobulin gene. These changes are then targeted by DNA repair pathways leading to either point mutations or class switching (Muramatsu et al., 2000; Maul and Gearhart, 2010).
The onset of the activity of this usually highly restricted enzyme is associated with significant genotoxic stress and a lowering of the apoptotic threshold (Zaheen et al., 2009). Similarly, to cells undergoing VDJ recombination the germinal center B cell have a fine balance between negative and positive apoptosis regulators with increased expression of Bax, p53, and c-myc and over expression of the pro-apoptotic receptors including CD95 (Koncz and Hueber, 2012). Overall this balance within the germinal center B cell leads to a programmed apoptotic death unless the B cell is rescued by interaction with antigen or CD40L (Inman and Allday, 2000). As a result, B cells that fail to successfully refine their antibody genes and form functional B cell receptors are destined to die an apoptotic death.
In parallel to the cells undergoing VDJ rearrangement, the B cells in the germinal centers also have dramatic changes to their physiology. Estimates from kinetic studies of germinal center B cells indicate that approximately 50% of these cells die via an apoptotic death every 6 h (Victora et al., 2010) and less than 2% of B cells successfully exit the lymph node (Mayer et al., 2017).
In Hodgkin lymphoma it is apparent that the infection of germinal center B cell with the Epstein Barr virus produces a number of pro-survival mechanisms that allow the infected B cells to resist the normal pro-apoptotic pressures and stimuli that normally remove the large majority of non-functional germinal center B cells (Spender and Inman, 2011). As a result, the Hodgkin lymphoma cells appears to be held suspended in a pro-apoptotic state that is relatively easy to destabilize with additional DNA damage from either cytotoxic drugs or radiation.
In a similar finding to that of ALL where the VDJ recombination process remains on going after the malignant transformation, there is also significant evidence to indicate that B cell malignancies arising from germinal center B cells also have on going activity of aspects of the somatic hypermutation process (Lossos et al., 2000; Pasqualucci et al., 2004; Lenz et al., 2007; Xu-Monette et al., 2019).
Follicular Lymphoma
The exception to the routine curability of malignancies linked to the somatic hypermutation process in the germinal center is follicular lymphoma. Follicular lymphoma is characteristically highly sensitive to DNA damaging cytotoxic chemotherapy treatment, but despite high response rates and long progression free intervals cure does not occur (Federico et al., 2009; Kahl and Yang, 2016). Whilst the full explanation of this divergence in outcome is awaited, it is likely that the characteristic t(14;18)(q32;q21) translocation occurring in follicular lymphoma (Tsujimoto et al., 1985) has a central role on the cells phenotype and apoptotic sensitivity. The impact of this translocation is to greatly enhance expression of the BCL-2 protein which is a powerful inhibitor of the normal apoptotic mechanisms in the germinal center (Huet et al., 2018).
Follicular lymphoma has historically been viewed as having its cell of origin from the germinal center B cell. However, there is increasing evidence that the cells now being identified as the potential follicular lymphoma cell of origin are actually CD27 +ve memory B cells (Sungalee et al., 2014; Tellier et al., 2014). These are long lived cells and it is postulated that they gain the malignant phenotype after repeated transits through the germinal center (Sungalee et al., 2014).
Follicular lymphoma and diffuse large B cell lymphoma (DLBCL) share important similarities with both having a close association with somatic hypermutation and with evidence of on-going somatic hypermutation in the malignant cells (Adam et al., 2007). In contrast the differing timescale and route to malignancy combined with the impact of the presence of BCL-2 activation make the overall biology of these two types of lymphoma very different. Conceptionally the route to malignancy and impact of somatic hypermutation appears to be distinctly different. In DLBCL it is likely that the malignant cells arising during the normal progress through the germinal center with the cells being involved with the full somatic hypermutation process for the first time. In contrast follicular lymphoma is likely to arise from aberrant memory B cells, a cell type that normally has completion somatic hypermutation and no longer expresses AID the key component of this system (Pasqualucci et al., 2004). Memory cells are able to retransit the germinal center and undergo a degree of reactivation of the somatic hypermutation activity (McHeyzer-Williams et al., 2015). However, in the resultant malignant cells the intensity of ongoing somatic hypermutation appears significantly lower in follicular lymphoma than in DLBCL and primary central nervous system lymphoma (Zuckerman et al., 2010).
In addition to the impact of mutation, epigenetic dysregulation plays a key role in the development of follicular lymphoma with mutations occurring in a larger number of key genes controlling changes affecting B cell differentiation and somatic mutation (Kretzmer et al., 2015). The patterns of methylation and hence gene activity differ substantially between follicular lymphoma and high-grade chemotherapy curable germinal center derived lymphomas (Kretzmer et al., 2015).
Whilst it is beyond the scope of this review to examine the details of these changes it is probable that these follicular lymphoma linked changes combined with the high BCL-2 activity may provide a strong counter to the residual impact of the low level activity of the somatic hypermutation associated apoptotic pathways (Zan and Casali, 2015; Huet et al., 2018).
Physiological Changes in Apoptotic Sensitivity During Somatic Hypermutation
The normal physiology of the germinal center B cell is intricately linked with apoptosis, as a result it is unsurprising that the non-malignant germinal cell B cells are extremely sensitive to DNA damage mediated apoptosis (Lindhout et al., 1995).
The extreme sensitivity of the germinal center to radiation exposure has been documented historically (De Bruyn, 1948; Congdon, 1966; Burge, 1975) and the B cells in the germinal center have been noted to be very sensitive to the induction of DNA damage mediated apoptosis by both cytotoxic drugs and radiation (Thielen and Heinen, 2010).
Post Somatic Hypermutation B Cell Malignancies
B cells that have successfully completed somatic hypermutation exit from the germinal center and complete the latter stages of maturation into antibody producing plasma cells. In normal healthy B cells at this point the AID activity and SHM phenotype is rapidly lost (Muramatsu et al., 2000; Lenz et al., 2007).
The B cell malignancies that arise from these later stages in development after the completion of both VDJ rearrangement and somatic hypermutation are generally less sensitive to DNA damaging agents and demonstrate no evidence of ongoing SHM activity (Bakkus et al., 1992; Rollett et al., 2006). The diagnoses arising at these points include plasmocytic lymphoma, CLL (mutated variable region) and myeloma which are usually responsive to but are not cured with cytotoxic chemotherapy.
Overview of the B Cell Malignancies and Their Variation in Chemotherapy Curability
Taken overall, the pattern of chemotherapy curability of malignancies moving along the B cell developmental pathway presents an intriguing picture. The initial malignancies arising closest to the hematopoietic stem cell, have the lowest number of mutations, but only relatively low cure rates with chemotherapy. In contrast the malignancies arising from the next stage in development, that is associated with the VDJ process, generally have more mutations but are highly curable, with over 90% of children with B-ALL being cured with modern therapy. The cells arising from the next stage in B cell development, occurring after VDJ is completed but before somatic hypermutation including Mantle cell lymphoma and chronic lymphocytic leukemia (CLL) are generally sensitive to chemotherapy but non-curable.
The malignancies arising from the next stage in B cell development, during the germinal center phase, include diffuse large B cell lymphoma and Hodgkin’s lymphoma are routinely curable. Finally, the malignancies arising from B cells that have completed VDJ recombination and somatic hypermutation include CLL, plasmocytic lymphoma and myeloma and are non-curable with DNA damaging chemotherapy.
Whilst there are other potential explanations for this unusual biphasic curability curve for B cell malignancies, we would argue that these two peaks of B cell malignancy chemotherapy curability coincide with and are linked to the two apoptosis associated cellular genetic events of immunoglobulin gene VDJ rearrangement and somatic hypermutation/class switching.
As discussed above, the physiology of the B cells during these two processes is dramatically different from any other cells, with extremely rapid turnover, dramatic upregulation of pro-apoptotic pathways and high levels of physiological cell death.
It is apparent that the resultant malignant cells carry some components of the phenotype of the cells that they arise from. We would argue that the presence of these components of the VDJ and somatic hypermutation phenotypes is linked to the extremely high sensitivity of these specific malignancies to DNA damaging chemotherapy.
T Cell Malignancies
T cell malignancies have a much lower incidence than B cell malignancies despite the numbers of B cells and T cells being similar (Anderson Marjault et al., 1998). T cells have a simpler pathway of genetic recombination to achieve variation in the antigenic recognition of the T cell receptor. The T cell receptor (TCR) alpha chain has only rearrangement of the V and J regions whilst the TCR beta chain has the complete rearrangement of the VDJ components similarly to a B cell (Bassing et al., 2002).
Of the T cell malignancies acute T cell ALL has the highest cure rates with a 75% cure rate in children (Goldberg et al., 2003) but with only 10–15% in adults (Rowe, 2010). Molecular analysis indicates that these cells, similar to B ALL have recombined VDJ genes (van Dongen et al., 2003). Additionally, in a similar fashion to that of B -ALL, it is apparent that the VDJ process can be on going in T-ALL with higher rates of clonal evolution seen in childhood cases compared to adult (Szczepański et al., 2003; Smirnova et al., 2016).
Amongst the malignancies arising later in the T cell developmental pathway ALK +ve anaplastic large cell lymphoma also has a high chemotherapy cure rate with long term survival rates of up to 80% are reported (Gascoyne et al., 1999). ALK +ve large cell lymphoma appears similar to Hodgkin’s lymphoma in that it has undergone V[D]J recombination but failed to produce a functional T cell receptor (Bonzheim et al., 2004). The ALK mutation appears to prevent the natural pattern of apoptosis and holds the cell in a suspended pro-apoptotic state.
The T cell malignancies arising later in the development pathway include peripheral T cell lymphoma, angioimmunoblastic lymphoma and adult T cell leukemia/lymphoma each have a poor prognosis with no significant chemotherapy mediated cure rates (Vose and Armitage, 2008).
Acute Myeloid Leukemia (AML)
Acute myeloid leukemia has a significant chemotherapy cure rate but in keeping with the experience in B ALL and T ALL the cure rates are significantly higher in children than adults. Overall pediatric AML has a cure rate approaching 70% whilst in adults the cure rate drops from approximately 50% for young adults to only 13% for those aged 60–69 (Shah et al., 2013; de Rooij et al., 2015).
Despite their later development pathways, myeloid precursor cells initially have a high level of activity of the VDJ recombination system. Genetic studies performed in AML indicate that the immunoglobulin heavy chains genes have undergone VDJ rearrangement in 40–50% of cases and that the ongoing expression of the VDJ recombinase associated proteins RAG1 and RAG2 is frequent (Kyoda et al., 1997; Stavroyianni et al., 2003).
In contrast the malignancies arising from mature myeloid cells, have no evidence of VDJ activity and characteristically have modest responses to chemotherapy (Vose and Armitage, 2008; Borcherding et al., 2019).
Testicular Cancer and Meiosis
Testicular cancer has been curable with chemotherapy for over 60 years (Li et al., 1960) and today patients with metastatic disease have overall cure rates approaching 90% (Feldman et al., 2008).
The malignant cells in testicular cancer usually arise from the pre-malignant precursor carcinoma in situ (CIS). This in turn evolves from an abnormal gonocyte, a developmental cell that has failed to mature normally to become a healthy pre-spermatogonia (Sonne et al., 2008, 2009). Recent data indicates that at the onset of testosterone exposure with puberty, the CIS cell, despite not having effectively matured to the a spermatogonium, has some of the key physiological machinery associated with meiosis activated (Jørgensen et al., 2012; Feichtinger and McFarlane, 2019).
In keeping with the thesis of the persistence of unique phenotype within the malignant cells recent data indicates that significant aspects of expression of genes associated with the meiosis phenotype are found in testicular germ cell tumors (Heaney et al., 2012; Bruggeman et al., 2018).
Physiological Changes in Apoptotic Sensitivity During Meiosis
Meiosis is a complex genetic process that involves genetic recombination and natural apoptosis is an integral component within the process of spermatogenesis. It is estimated that 75% of spermatogenesis is lost via apoptosis (Dunkel et al., 1997).
In the normal process of spermatogenesis, the onset of the process of meiosis has a dramatic effect on the sensitivity to DNA damage induced apoptosis. This has been demonstrated in studies examining the impact of low dose radiation on the survival of cells in the differing stages of spermatogenesis (Rowley et al., 1974; Marjault and Allemand, 2016). The data as shown in Table 3 indicates that the cells that are outside the meiotic process, the quiescent spermatogonial stem cells and the maturing sperm are relatively resistant to induction of apoptosis by low dose radiation despite developing similar levels of DNA damage (Grewenig et al., 2015). In contrast the cells undergoing meiosis, the differentiating spermatogonia and spermatocytes are significantly more sensitive with cell killing rates of up to 99%.
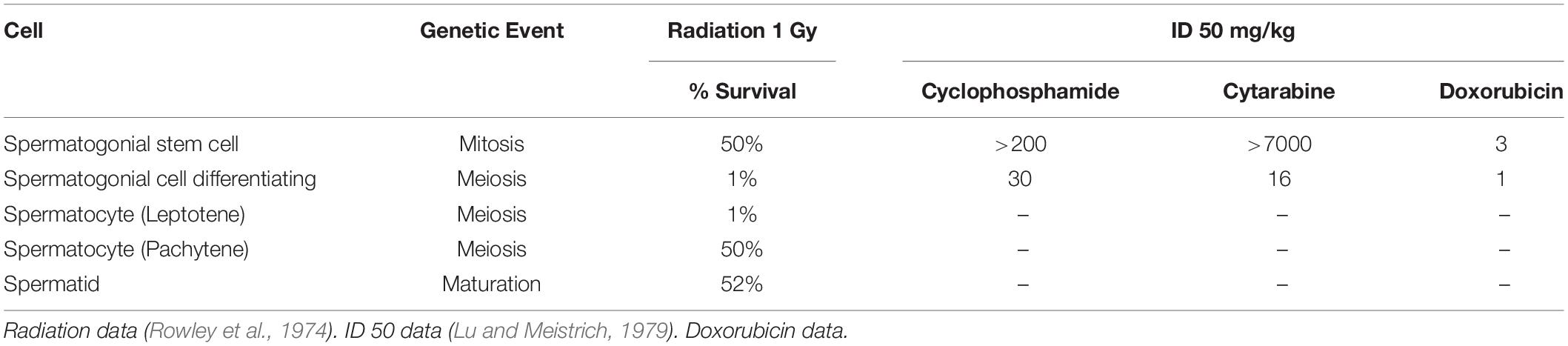
Table 3. Variation in the sensitivity to the induction of apoptosis with DNA damaging radiation and cytotoxic chemotherapy during spermatogenesis.
Experimental data in murine models examining the impact of a number of cytotoxic chemotherapy drugs on apoptosis in spermatogenesis also indicates dramatic changes in sensitivity to chemotherapy between spermatogonial stem cells and differentiating spermatogonial cells (Lu and Meistrich, 1979). As shown in Table 3 the difference in ID50 concentration can vary up to more than a 100-fold difference which is far greater than the ratios seen in conventional malignancies (Izumi et al., 2017).
It is apparent that the processes involved in meiosis are closely linked to naturally occurring apoptosis in health. We would argue that in testicular cancer the malignant cells that arise from these abnormal parent cells that have partially activated components of the meiotic phenotype. As a result, they maintain some of the unique physiology and heightened apoptotic sensitivity that naturally occurs at this unique biological point.
Gestational Malignancies and Nuclear Fusion
The gestational malignancies are rare but have been curable with chemotherapy since the 1950s (Hertz et al., 1956). The majority of cases happen after a complete molar pregnancy and in this situation a cure rate of 100%, with the use of often low dose single agent chemotherapy, can be expected (Sita-Lumsden et al., 2012). The clinically more challenging forms of gestational malignancies, choriocarcinoma and placental site trophoblast tumor generally arise from otherwise genetically normal pregnancies and have high cure rates with combination chemotherapy (Schmid et al., 2009; Alifrangis et al., 2013).
Whilst there is no normal counterpart to the cells of a molar pregnancy, it is apparent that it shares considerable phenotypic characteristic with an early healthy trophoblast cell. In contrast the cells of choriocarcinoma and PSST have phenotypic and epigenetic similarities with more slightly more developmentally mature cells (Kurman et al., 1984; Savage et al., 2019). Similar to the situation in the B cell malignancies it appears that the malignant trophoblast cells remain frozen with their developmental phenotype and do not follow the standard maturation and developmental pathways of trophoblast/placental cells (Savage et al., 2019).
The timing of the developmental origin of the gestational trophoblast tumors appears to have a close relationship with the chemotherapy sensitivity of the malignancies arising along this route. Molar pregnancies that arise at the time of fertilization have a cure rate approaching 100% and patients with this diagnosis generally only require low dose single agent chemotherapy (Sita-Lumsden et al., 2012). Gestational choriocarcinoma which arises slightly later in developmental has chemotherapy cure rates approaching 95% but with most patients requiring more intensive combination chemotherapy (Alifrangis et al., 2013). The rarer diagnosis PSST which is believed to arise later in trophoblast cell development has a lower overall cure rate for patients with metastatic disease of approximately 50% (Schmid et al., 2009).
Physiological Changes in Apoptotic Sensitivity Following Nuclear Fusion
In health gametes and the cells of conception demonstrate a change in their sensitivity to the induction of DNA damage mediated apoptosis in a relatively short period after fertilization. Clinical data indicate that sperm are relatively resistant to DNA damage, with sperm counts in cancer patients only subsiding significantly 1–2 months after the commencement of cytotoxic chemotherapy (Meistrich et al., 1997). This resistance to the induction of DNA damage mediated apoptosis is also supported by data shown in Table 3 that indicates that radiotherapy (at 1Gy) only causes a 50% reduction in spermatid counts compared to a 99% reduction in the number of early spermatocytes (Rowley et al., 1974).
There is little data on the sensitivity of human ova and the early cells of conception to DNA damaging cytotoxic drugs. However, in vitro animal data suggests that there is an appreciable difference in sensitivity to methotrexate mediated apoptosis between the newly fertilized ova and the early stages of the developing blastocyst. Murine data looking at the impact of methotrexate exposure on maturing ova indicates that concentrations of methotrexate of 20uM and above are clearly cytotoxic (Takai et al., 2007). However, the cells are able to tolerate exposure to methotrexate at 10 μM with only modest reductions in the speed of germinal vesicle breakdown and polar body extrusion but without an impact on cell viability (Tian et al., 2018).
In fertilized zygotes, in both murine and bovine in vitro models, a biphasic impact of methotrexate exposure is seen. Fertilized ova and zygotes up to 8 cell stage were largely resistant to exposure to methotrexate at 10 μM. In contrast, methotrexate at 10 μM resulted in the total loss of viability for 8 cells zygotes in the bovine system (Kwong et al., 2010) and a major reduction in viability in the mouse model (O’Neill, 1998). The reason for this increasing sensitivity to methotrexate induced apoptosis is unclear. It is apparent that the cells are increasing their thymidine requirements (O’Neill, 1998) at this stage but also making profound and rapid changes to their patterns of gene expression (Fraser and Lin, 2016).
The in vitro documentation of extreme sensitivity of the early cells of conception to cytotoxic drugs is also reflected clinically in the management of ectopic pregnancies where a single dose of 50 mg of methotrexate is employed (Creinin et al., 1996).
The very high degree of sensitivity to cytotoxic drugs of the cells after conception occurs for only a relatively short time and as the placenta matures it undergoes a rapid change in sensitivity to DNA damage induced apoptosis. This process that is complete by the end of the first trimester by when the trophoblast cells (Sand et al., 1986) and the placenta are resistant to cytotoxic drug induced apoptosis (Abellar et al., 2009). Of the note the very rare proliferative conditions that arise from the mature cells of the placental exhibit a high degree of resistance to chemotherapy drugs (Farasatinasab et al., 2016).
At present there is little data on the underlying mechanisms for the high degree of chemotherapy sensitivity for both the native and malignant trophoblast cells. Recent whole genome sequencing and epigenetic analysis suggests that gestational trophoblast tumors do not have any significant mutational burden and are likely to arise during aberrations of methylation during the early stages of placental development (Rowley et al., 1974; Xing et al., 2019). Following fertilization there are dramatic changes in the methylation patterns of the trophoblast cell (Fraser and Lin, 2016) and it is likely that these changes in gene expression and DNA conformation are the key drivers in the rapidly changing sensitivity to induction of apoptosis from cytotoxic chemotherapy induced DNA damage.
Childhood Malignancies and Gastrulation
At present there is considerable debate regarding the origin of the rare childhood malignancies. Previously we and others have suggested that these rare malignancies with their very primitive pathology may be linked to defects in gastrulation (Ewing, 1921; von Levetzow et al., 2011; Savage, 2015). We hypothesize that these cells remain trapped in a normally transient phenotype in similar way the B cell malignancies are trapped at the point of origin cell type.
The curability of these malignancies with cytotoxic chemotherapy was originally seen nearly 50 years ago and current cure rates approach 70% for neuroblastoma, 75% for Ewing sarcoma 74 and 90% for Wilms tumor (Smith et al., 2010).
Physiological Changes in Apoptotic Sensitivity During Gastrulation
Whilst gastrulation does not include DNA mutation or recombination the process has a major impact on apoptotic sensitivity. In a murine model, the dramatic changes in the sensitivity of cells to DNA damage associated with gastrulation are demonstrated in Figure 3. An exposure of 0.5Gy resulted in <5% apoptotic embryonic cell death at day 5 just prior to gastrulation. In contrast at day 6.5 the apoptotic cell death response to the same low dose of radiation rose to 60%. By day 8.5 as the gastrulation process was completing the apoptotic response fell back to 10% (Heyer et al., 2000).
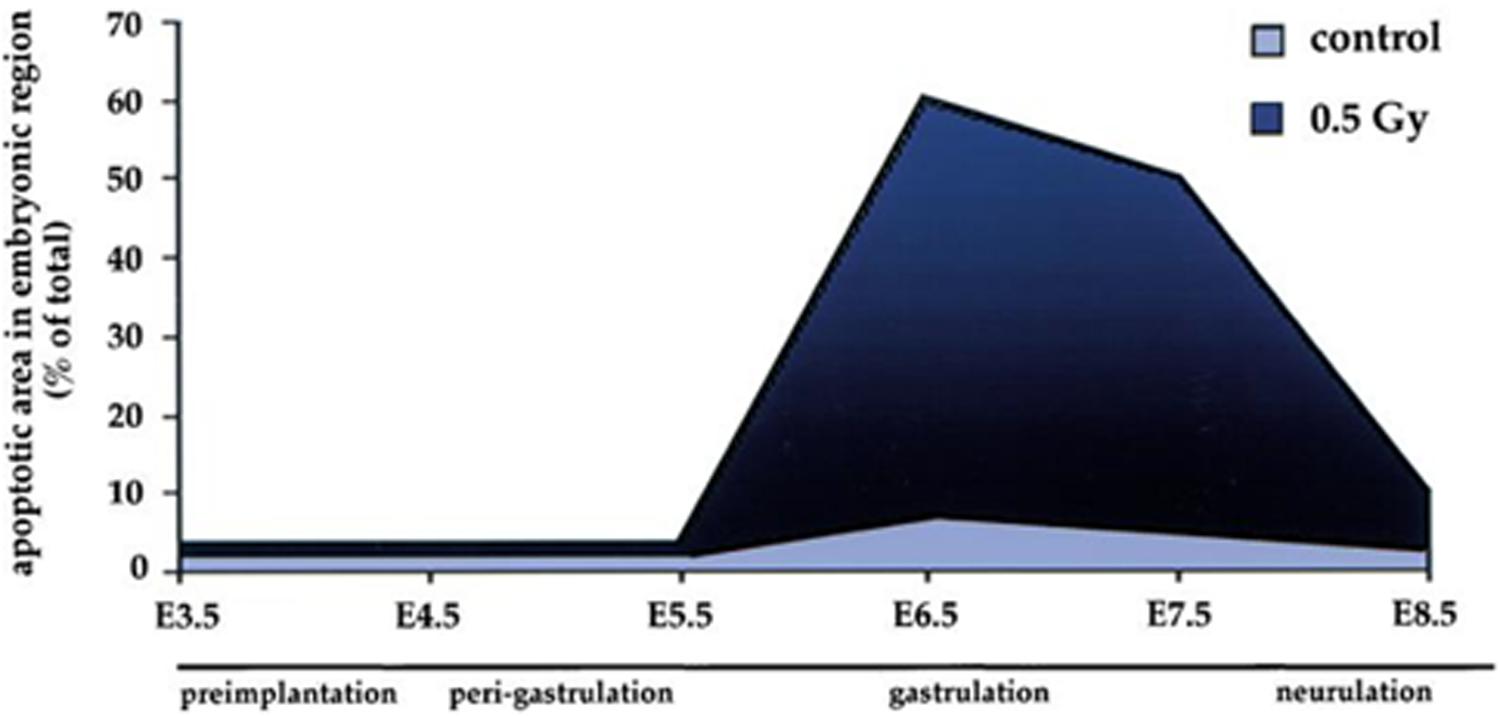
Figure 3. Sensitivity of embryonic cells to low dose radiation (0.5 Gy) before, during and after gastrulation. Data and graph taken from Heyer et al. (2000).
The relationship to defects in gastrulation and the etiology of the rare childhood malignancies remains an area of debate. However, it is apparent that in a similar way to the impact of VDJ recombination, meiosis and nuclear fusion the process of gastrulation is closely related to dramatic changes in apoptotic sensitivity.
Differential Chemotherapy Sensitivity in Non-Mutational and Single Mutation Malignancies
Classically the paradigm for oncogenesis is centered on the sequential development of mutation leading to the development of the malignant phenotype. This appears to be the case for the majority of solid cancers (Fearon and Vogelstein, 1990) and also for many lymphoid malignancies (Greaves et al., 2003).
A small number of relatively rare malignancies, each of which has a short developmental time for oncogenesis, appear to arise via alternate routes. Recent whole genome sequencing and epigenetic analyses have provided an insight into these differing routes to oncogenesis and also potential factors affecting chemotherapy sensitivity and chemotherapy curability.
Non-mutational Malignancies
Two rare malignancies appear to have their route to oncogenesis occurring from epigenetic changes alone without any documented mutational drivers.
In the childhood CNS malignancy CIMP ependymoma, a series of 47 cases were analyzed by whole genome analysis, with the results indicating a very low mutational burden and an absence of documented oncogene or driver mutations (Mack et al., 2014). In contrast to the absence of mutation, epigenetic analysis indicated consistent abnormalities of methylation impacting on the action of the Polycomb repressive complex (Mack et al., 2014).
More recently we have reported the first case of gestational choriocarcinoma to be analyzed with whole genome sequencing and full methylation analysis (Savage et al., 2019). Similarly, to the findings in CIMP ependymoma this cancer also has no appreciable mutational burden. However, the cells appear to have a defect in the natural progression of methylation that leaves the malignant cell fixed with a persisting phenotype of an early trophoblast cell.
Whilst the exact timing of the onset of oncogenesis in gestational choriocarcinoma is yet to be determined it is likely that the malignant cells arise from cells within the first 2 weeks after conception and nuclear fusion. As discussed previously normal healthy cells at this point are immensely sensitive to cytotoxic chemotherapy in vitro (Kwong et al., 2010) and in vivo as demonstrated by the efficacy of low dose methotrexate in the treatment of ectopic pregnancies (Creinin et al., 1996).
Whilst these two malignancies have similar etiology, they have dramatically different responses to chemotherapy treatment as shown in Table 4. CIMP ependymoma is characteristically highly resistant to cytotoxic chemotherapy treatment and carries an extremely poor prognosis (Bouffet and Foreman, 1999). In contrast gestational choriocarcinoma is immensely sensitive to cytotoxic chemotherapy treatment and has a 95% cure rate (Alifrangis et al., 2013).

Table 4. Comparison of the route to oncogenesis, relationship to unique genetic events and chemotherapy curability for non-mutational and single mutation malignancies.
As discussed above we would argue that the key difference that affects the response to chemotherapy, for these two non-mutational malignancies, is the persistence of the ongoing phenotype of the early post nuclear fusion trophoblast cell in choriocarcinoma.
Single Mutational Malignancies
Highly divergent sensitivity to DNA damaging chemotherapy is also seen in two other rare malignancies which are each characterized by a single mutation.
Pediatric rhabdoid malignancy has a single driver mutation which is of SMARCB1 tumor suppressor gene (Kieran et al., 2012). Similarly, infantile ALL also has a single driver mutation in the MLL gene (Dobbins et al., 2013).
Aside from these mutations both infantile ALL and the pediatric rhabdoid malignancies are otherwise mutationally bland with a negligible level of mutation (Kieran et al., 2012; Dobbins et al., 2013).
Despite this apparent similarity of genetic structure and route to oncogenesis these two malignancies also differ dramatically in their sensitivity to DNA damaging chemotherapy treatment. Pediatric rhabdoid malignancies are characterized by a very high level of resistance to DNA damaging cytotoxic chemotherapy. The prognosis for this rare cancer is very poor with the median survival of patients with metastatic disease of less than 1 year (Reinhard et al., 2008; Brennan et al., 2016). In contrast infantile ALL has a high response rate to chemotherapy and approximately 40% of patients are cured (Pieters et al., 2007).
However, whilst the two malignancies are both mutationally bland aside from their single driver mutation there is key difference between them. Infantile ALL arises in cells that are in the earliest stages of VDJ rearrangement that have either germline or an incompletely rearranged VDJ regions (Jansen et al., 2007). In keeping with cases of ALL arising slightly later in the development pathway infantile ALL cells have on going significant expression of the RAG1/2 enzymes indicating that significant components of the early VDJ phenotype are retained in the malignant cells (Jansen et al., 2007).
From these four rare malignancies we can see the extremes of sensitivity to chemotherapy treatment that does not appear to be influenced by the absence of mutation, or by the route to oncogenesis being methylation changes. However, for the two chemotherapy curable malignancies it is apparent that they are closely linked, via their parent cell, to the genetic events of either VDJ recombination or nuclear fusion. We would argue that it is the partial persistence of the unique phenotype associated with these events that leads the cells to retain extremely high levels of sensitivity to DNA damaging therapies.
Discussion
A number of malignancies have been routinely curable with chemotherapy treatment for over 60 years. Review of the natural history of these cells indicates that they each arises from a special transient cell type that is intricately involved with one of the key physiological processes of immunoglobulin gene rearrangements, meiosis, nuclear fusion or gastrulation.
The chemotherapy sensitivity of these malignancies appears to mirror the extreme natural sensitivity to the induction of apoptosis that these parent cells naturally pass through as part of these transient processes in health.
This interpretation of the biological and clinical data offers an explanation as to why other malignancies have not become curable despite immense research endeavors.
At present there is little data on the detailed mechanisms as to how these dramatic changes in apoptotic sensitivity occur. With more information it may be possible to devise therapeutic approaches that could therapeutically exploit these dramatic biological effects.
Data Availability Statement
All datasets generated for this study are included in the article/supplementary material/reference list.
Author Contributions
PS wholly designed, researched the study and wrote the manuscript.
Conflict of Interest
The author declares that the research was conducted in the absence of any commercial or financial relationships that could be construed as a potential conflict of interest.
References
Abellar, R. G., Pepperell, J. R., Greco, D., Gundogan, F., Kostadinov, S., Schwartz, J., et al. (2009). Effects of chemotherapy during pregnancy on the placenta. Pediatr. Dev. Pathol. 12, 35–41. doi: 10.2350/08-03-0435.1
Adam, P., Schoof, J., Hartmann, M., Schwarz, S., Puppe, B., Ott, M., et al. (2007). Cell migration patterns and ongoing somatic mutations in the progression of follicular lymphoma. Cytogenet. Genome Res. 118, 328–336.
Agraz-Doblas, A., Bueno, C., Bashford-Rogers, R., Roy, A., Schneider, P., Bardini, M., et al. (2019). Unraveling the cellular origin and clinical prognostic markers of infant B-cell acute lymphoblastic leukemia using genome-wide analysis. Haematologica 104, 1176–1188. doi: 10.3324/haematol.2018.206375
Alifrangis, C., Agarwal, R., Short, D., Fisher, R. A., Sebire, N. J., Harvey, R., et al. (2013). EMA/CO for high-risk gestational trophoblastic neoplasia: good outcomes with induction low-dose etoposide-cisplatin and genetic analysis. J. Clin. Oncol. 31, 280–286. doi: 10.1200/JCO.2012.43.1817
Alizadeh, A. A., Aranda, V., Bardelli, A., Blanpain, C., Bock, C., Borowski, C., et al. (2015). Toward understanding and exploiting tumor heterogeneity. Nat. Med. 21, 846–853. doi: 10.1038/nm.3915
Anderson, M. I., Armitage, J. O. Jr., and Weisenburger, D. D. (1998). Epidemiology of the non-Hodgkin’s lymphomas: distributions of the major subtypes differ by geographic locations. Non-Hodgkin’s Lymphoma Classification Project. Ann. Oncol. 9, 717–720.
Andersson, A. K., Ma, J., Wang, J., Chen, X., Gedman, A. L., Dang, J., et al. (2015). The landscape of somatic mutations in infant MLL-rearranged acute lymphoblastic leukemias. Nat. Genet. 47, 330–337. doi: 10.1038/ng.3230
Andersson, A., Edén, P., Olofsson, T., and Fioretos, T. (2010). Gene expression signatures in childhood acute leukemias are largely unique and distinct from those of normal tissues and other malignancies. BMC Med. Genom. 3:6. doi: 10.1186/1755-8794-3-6
Andersson, A., Olofsson, T., Lindgren, D., Nilsson, B., Ritz, C., Edén, P., et al. (2005). Molecular signatures in childhood acute leukemia and their correlations to expression patterns in normal hematopoietic subpopulations. Proc. Natl. Acad. Sci. U.S.A. 102, 19069–19074.
Bakkus, M. H., Heirman, C., Van Riet, I., Van Camp, B., and Thielemans, K. (1992). Evidence that multiple myeloma Ig heavy chain VDJ genes contain somatic mutations but show no intraclonal variation. Blood 80, 2326–2335.
Bassing, C. H., Swat, W., and Alt, F. W. (2002). The mechanism and regulation of chromosomal V(D)J recombination. Cell 109, S45–S45.
Beishuizen, A., Verhoeven, M. A., van Wering, E. R., Hählen, K., Hooijkaas, H., and van Dongen, J. J. (1994). Analysis of Ig and T-cell receptor genes in 40 childhood acute lymphoblastic leukemias at diagnosis and subsequent relapse: implications for the detection of minimal residual disease by polymerase chain reaction analysis. Blood 83, 2238–2247.
Biechonski, S., Olender, L., Zipin-Roitman, A., Yassin, M., Aqaqe, N., Marcu-Malina, V., et al. (2018). Attenuated DNA damage responses and increased apoptosis characterize human hematopoietic stem cells exposed to irradiation. Sci. Rep. 8:6071. doi: 10.1038/s41598-018-24440-w
Biondi, A., Cimino, G., Pieters, R., and Pui, C. H. (2000). Biological and therapeutic aspects of infant leukemia. Blood 96, 24–33.
Bird, J., Galili, N., Link, M., Stites, D., and Sklar, J. (1988). Continuing rearrangement but absence of somatic hypermutation in immunoglobulin genes of human B cell precursor leukemia. J. Exp. Med. 168, 229–245.
Bonzheim, I., Geissinger, E., Roth, S., Zettl, A., Marx, A., Rosenwald, A., et al. (2004). Anaplastic large cell lymphomas lack the expression of T-cell receptor molecules or molecules of proximal T-cell receptor signaling. Blood 104, 3358–3356.
Borcherding, N., Voigt, A. P., Liu, V., Link, B. K., Zhang, W., and Jabbari, A. (2019). Single-Cell Profiling of Cutaneous T-Cell Lymphoma reveals underlying heterogeneity associated with disease progression. Clin. Cancer Res. 25, 2996–3005. doi: 10.1158/1078-0432.CCR-18-3309
Bouffet, E., and Foreman, N. (1999). Chemotherapy for intracranial ependymomas. Childs Nerv. Syst. 15, 563–570.
Brennan, B., De Salvo, G. L., Orbach, D., De Paoli, A., Kelsey, A., Mudry, P., et al. (2016). Outcome of extracranial malignant rhabdoid tumours in children registered in the European Paediatric Soft Tissue Sarcoma Study Group Non-Rhabdomyosarcoma soft tissue sarcoma 2005 study-EpSSG NRSTS 2005. Eur. J. Cancer 60, 69–82. doi: 10.1016/j.ejca.2016.02.027
Bruggeman, J. W., Koster, J., Lodder, P., Repping, S., and Hamer, G. (2018). Massive expression of germ cell-specific genes is a hallmark of cancer and a potential target for novel treatment development. Oncogene 37, 5694–5700. doi: 10.1038/s41388-018-0357-2
Burge, J. S. (1975). Histological changes in cervical lymph nodes following clinical irradiation. Proc. R. Soc. Med. 68, 77–79.
Campbell, J. J., Clark, R. A., Watanabe, R., and Kupper, T. S. (2010). Sezary syndrome and mycosis fungoides arise from distinct T-cell subsets: a biologic rationale for their distinct clinical behaviors. Blood 116, 767–771. doi: 10.1182/blood-2009-11-251926
Canellos, G. P., Anderson, J. R., Propert, K. J., Nissen, N., Cooper, M. R., Henderson, E. S., et al. (1992). Chemotherapy of advanced Hodgkin’s disease with MOPP, ABVD or MOPP alternating with ABVD. N. Eng. J Med. 327, 1478–1484.
Chabner, B. A., and Roberts, T. G. Jr. (2005). Timeline: chemotherapy and the war on cancer. Nat. Rev. Cancer. 5, 65–72. doi: 10.1038/nrc1529
Chalmers, Z. R., Connelly, C. F., Fabrizio, D., Gay, L., Ali, S. M., Ennis, R., et al. (2017). Analysis of 100,000 human cancer genomes reveals the landscape of tumor mutational burden. Genome Med. 9:34. doi: 10.1186/s13073-017-0424-2
Congdon, C. C. (1966). The destructive effect of radiation on lymphatic tissue. Cancer Res. 26, 1211–1220.
Creinin, M. D., Vittinghoff, E., Keder, L., Darney, P. D., and Tiller, G. (1996). Methotrexate and misoprostol for early abortion: a multicenter trial. I. Safety and efficacy. Contraception 53, 321–327. doi: 10.1016/0010-7824(96)00080-7
Crespo, M., Bosch, F., Villamor, N., Bellosillo, B., Colomer, D., Rozman, M., et al. (2003). ZAP-70 expression as a surrogate for immunoglobulin-variable-region mutations in chronic lymphocytic leukemia. N. Engl. J. Med. 348, 1764–1775. doi: 10.1056/nejmoa023143
De Bie, J., Demeyer, S., Alberti-Servera, L., Geerdens, E., Segers, H., Broux, M., et al. (2018). Single-cell sequencing reveals the origin and the order of mutation acquisition in T-cell acute lymphoblastic leukemia. Leukemia 32, 1358–1369. doi: 10.1038/s41375-018-0127-8
De Bruyn, P. P. (1948). The effect of X-rays on the lymphatic nodule, with reference to the dose and relative sensitivities of different species. Anat. Rec. 101, 373–340.
de Rooij, J. D., Zwaan, C. M., and van den Heuvel-Eibrink, M. (2015). Pediatric AML: from biology to clinical management. J. Clin. Med. 4, 127–149. doi: 10.3390/jcm4010127
Dean, M., Fojo, T., and Bates, S. (2005). Tumour stem cells and drug resistance. Nat. Rev. Cancer 5, 275–284. doi: 10.1038/nrc1590
DeVita, V. T. Jr., and Chu, E. (2008). A history of cancer chemotherapy. Cancer Res. 68, 8643–8653. doi: 10.1158/0008-5472.can-07-6611
Dimopoulos, M. A., Kastritis, E., Owen, R. G., Kyle, R. A., Landgren, O., Morra, E., et al. (2014). Treatment recommendations for patients with Waldenström macroglobulinemia (WM) and related disorders: IWWM-7 consensus. Blood 124, 1404–1411. doi: 10.1182/blood-2014-03-565135
Dobbins, S. E., Sherborne, A. L., Ma, Y. P., Bardini, M., Biondi, A., Cazzaniga, G., et al. (2013). The silent mutational landscape of infant MLL-AF4 pro-B acute lymphoblastic leukemia. Genes Chromosomes Cancer 52, 954–960. doi: 10.1002/gcc.22090
Dunkel, L., Hirvonen, V., and Erkkilä, K. (1997). Clinical aspects of male germ cell apoptosis during testis development and spermatogenesis. Cell Death Differ. 4, 171–179. doi: 10.1038/sj.cdd.4400234
Durdik, M., Kosik, P., Kruzliakova, J., Jakl, L., Markova, E., and Belyaev, I. (2017). Hematopoietic stem/progenitor cells are less prone to undergo apoptosis than lymphocytes despite similar DNA damage response. Oncotarget 8, 48846–48853. doi: 10.18632/oncotarget.16455
Farasatinasab, M., Moghaddas, A., Dashti-Khadivaki, S., Raoofi, Z., and Nasiripour, S. (2016). Management of abnormal placenta implantation with methotrexate: a review of published data. Gynecol. Obstet. Invest. 81, 481–496. doi: 10.1159/000447556
Farber, S., and Diamond, L. K. (1948). Temporary remissions in acute leukemia in children produced by folic acid antagonist, 4-aminopteroyl-glutamic acid. N. Engl. J. Med. 238, 787–793. doi: 10.1056/nejm194806032382301
Fearon, E. R., and Vogelstein, B. (1990). A genetic model for colorectal tumorigenesis. Cell 61, 759–767. doi: 10.1016/0092-8674(90)90186-i
Federico, M., Bellei, M., Marcheselli, L., Luminari, S., Lopez-Guillermo, A., Vitolo, U., et al. (2009). Follicular lymphoma international prognostic index 2: a new prognostic index for follicular lymphoma developed by the international follicular lymphoma prognostic factor project. J. Clin. Oncol. 27, 4555–4562. doi: 10.1200/JCO.2008.21.3991
Feichtinger, J., and McFarlane, R. J. (2019). Meiotic gene activation in somatic and germ cell tumours. Andrology 7, 415–427. doi: 10.1111/andr.12628
Feldman, D. R., Bosl, G. J., Sheinfeld, J., and Motzer, R. J. (2008). Medical treatment of advanced testicular cancer. JAMA 299, 672–684. doi: 10.1001/jama.299.6.672
Fernàndez, V., Salamero, O., Espinet, B., Solé, F., Royo, C., Navarro, A., et al. (2010). Genomic and gene expression profiling defines indolent forms of mantle cell lymphoma. Cancer Res. 70, 1408–1418. doi: 10.1158/0008-5472.CAN-09-3419
Fraser, R., and Lin, C. J. (2016). Epigenetic reprogramming of the zygote in mice and men: on your marks, get set, go! Reproduction 152, R211–R212.
Fu, K., Weisenburger, D. D., Choi, W. W., Perry, K. D., Smith, L. M., Shi, X., et al. (2008). Addition of rituximab to standard chemotherapy improves the survival of both the germinal center B-cell-like and non-germinal center B-cell-like subtypes of diffuse large B-cell lymphoma. J. Clin. Oncol. 26, 4587–4594. doi: 10.1200/JCO.2007.15.9277
Gascoyne, R. D., Aoun, P., Wu, D., Chhanabhai, M., Skinnider, B. F., Greiner, T. C., et al. (1999). Prognostic significance of anaplastic lymphoma kinase (ALK) protein expression in adults with anaplastic large cell lymphoma. Blood 93, 3913–3921. doi: 10.1182/blood.v93.11.3913.411k22_3913_3921
Gawad, C., Pepin, F., Carlton, V. E., Klinger, M., Logan, A. C., Miklos, D. B., et al. (2012). Massive evolution of the immunoglobulin heavy chain locus in children with B precursor acute lymphoblastic leukemia. Blood 120, 4407–4417. doi: 10.1182/blood-2012-05-429811
Gerlinger, M., Rowan, A. J., Horswell, S., Math, M., Larkin, J., Endesfelder, D., et al. (2012). Intratumor heterogeneity and branched evolution revealed by multiregion sequencing. N. Engl. J. Med. 366, 883–892. doi: 10.1056/NEJMoa1113205
Goldberg, J. M., Silverman, L. B., Levy, D. E., Dalton, V. K., Gelber, R. D., Lehmann, L., et al. (2003). Childhood T-cell acute lymphoblastic leukemia: the Dana-Farber Cancer Institute acute lymphoblastic leukemia consortium experience. J. Clin. Oncol. 21, 3616–3622. doi: 10.1200/jco.2003.10.116
Greaves, M. F., Maia, A. T., Wiemels, J. L., and Ford, A. M. (2003). Leukemia in twins: lessons in natural history. Blood 102, 2321–2333. doi: 10.1182/blood-2002-12-3817
Greipp, P. R., San Miguel, J., Durie, B. G., Crowley, J. J., Barlogie, B., Bladé, J., et al. (2005). International staging system for multiple myeloma. J Clin. Oncol. 23, 3412–3420.
Grewenig, A., Schuler, N., and Rübe, C. E. (2015). Persistent DNA damage in spermatogonial stem cells after fractionated low-dose irradiation of testicular tissue. Int. J. Radiat. Oncol. Biol. Phys. 92, 1123–1131. doi: 10.1016/j.ijrobp.2015.04.033
Griffiths, S. D., Goodhead, D. T., Marsden, S. J., Wright, E. G., Krajewski, S., Reed, J. C., et al. (1994a). Interleukin 7-dependent B lymphocyte precursor cells are ultrasensitive to apoptosis. J. Exp. Med. 179, 1789–1797. doi: 10.1084/jem.179.6.1789
Griffiths, S. D., Marsden, S. J., Wright, E. G., Greaves, M. F., and Goodhead, D. T. (1994b). Lethality and mutagenesis of B lymphocyte progenitor cells following exposure to alpha-particles and X-rays. Int. J. Radiat. Biol. 66, 197–205. doi: 10.1080/09553009414551101
Hanna, N., and Einhorn, L. H. (2014). Testicular cancer: a reflection on 50 years of discovery. J. Clin. Oncol. 32, 3085–3092. doi: 10.1200/jco.2014.56.0896
Heaney, J. D., Anderson, E. L., Michelson, M. V., Zechel, J. L., Conrad, P. A., Page, D. C., et al. (2012). Germ cell pluripotency, premature differentiation and susceptibility to testicular teratomas in mice. Development 139, 1577–1586. doi: 10.1242/dev.076851
Heesch, S., Neumann, M., Schwartz, S., Bartram, I., Schlee, C., Burmeister, T., et al. (2013). Acute leukemias of ambiguous lineage in adults: molecular and clinical characterization. Ann. Hematol. 92, 747–758. doi: 10.1007/s00277-013-1694-4
Hertz, R., Li, M. C., and Spencer, D. B. (1956). Effect of methotrexate therapy upon choriocarcinoma and chorioadenoma. Proc. Soc. Exp. Biol. Med. 93, 361–366. doi: 10.3181/00379727-93-22757
Heyer, B. S., MacAuley, A., Behrendtsen, O., and Werb, Z. (2000). Hypersensitivity to DNA damage leads to increased apoptosis during early mouse development. Genes Dev. 14, 2072–2084.
Holohan, C., Van Schaeybroeck, S., Longley, D. B., and Johnston, P. G. (2013). Cancer drug resistance: an evolving paradigm. Nat. Rev. Cancer 13, 714–726. doi: 10.1038/nrc3599
Housman, G., Byler, S., Heerboth, S., Lapinska, K., Longacre, M., Snyder, N., et al. (2014). Drug resistance in cancer: an overview. Cancers 6, 1769–1792. doi: 10.3390/cancers6031769
Huet, S., Sujobert, P., and Salles, G. (2018). From genetics to the clinic: a translational perspective on follicular lymphoma. Nat. Rev. Cancer 18, 224–239. doi: 10.1038/nrc.2017.127
Hystad, M. E., Myklebust, J. H., Bø, T. H., Sivertsen, E. A., Rian, E., Forfang, L., et al. (2007). Characterization of early stages of human B cell development by gene expression profiling. J. Immunol. 179, 3662–3671. doi: 10.4049/jimmunol.179.6.3662
Inaba, H., Greaves, M., and Mullighan, C. G. (2013). Acute lymphoblastic leukaemia. Lancet 381, 1943–1955. doi: 10.1016/S0140-6736(12)62187-4
Inman, G. J., and Allday, M. J. (2000). Apoptosis induced by TGF-beta 1 in Burkitt’s lymphoma cells is caspase 8 dependent but is death receptor independent. J. Immunol. 165, 2500–2510. doi: 10.4049/jimmunol.165.5.2500
Izumi, D., Ishimoto, T., Miyake, K., Eto, T., Arima, K., Kiyozumi, Y., et al. (2017). Colorectal Cancer Stem Cells Acquire Chemoresistance Through the Upregulation of F-Box/WD repeat-containing protein 7 and the consequent degradation of c-Myc. Stem Cells. 35, 2027–2036. doi: 10.1002/stem.2668
Jacob, J., Kelsoe, G., Rajewsky, K., and Weiss, U. (1991). Intraclonal generation of antibody mutants in germinal centres. Nature 354, 389–392. doi: 10.1038/354389a0
Jansen, M. W., Corral, L., van der Velden, V. H., Panzer-Grümayer, R., Schrappe, M., Schrauder, A., et al. (2007). Immunobiological diversity in infant acute lymphoblastic leukemia is related to the occurrence and type of MLL gene rearrangement. Leukemia 21, 633–634.
Jensen, K., Brusletto, B. S., Aass, H. C., Olstad, O. K., Kierulf, P., and Gautvik, K. M. (2013a). Transcriptional profiling of mRNAs and microRNAs in human bone marrow precursor B cells identifies subset- and age-specific variations. PLoS One 8:e70721. doi: 10.1371/journal.pone.0070721
Jensen, K., Rother, M. B., Brusletto, B. S., Olstad, O. K., Dalsbotten Aass, H. C., van Zelm, M. C., et al. (2013b). Increased ID2 levels in adult precursor B cells as compared with children is associated with impaired Ig locus contraction and decreased bone marrow output. J. Immunol. 191, 1210–1219. doi: 10.4049/jimmunol.1203462
Jørgensen, A., Nielsen, J. E., Jensen, M. B., Graem, N., and Rajpert-De Meyts, E. (2012). Analysis of meiosis regulators in human gonads: a sexually dimorphic spatiotemporal expression pattern suggests involvement of DMRT1 in meiotic entry. Mol. Hum. Reprod. 18, 523–534. doi: 10.1093/molehr/gas030
Kahl, B. S., and Yang, D. T. (2016). Follicular lymphoma: evolving therapeutic strategies. Blood. 127, 2055–2063. doi: 10.1182/blood-2015-11-624288
Kieran, M. W., Roberts, C. W., Chi, S. N., Ligon, K. L., Rich, B. E., Macconaill, L. E., et al. (2012). Absence of oncogenic canonical pathway mutations in aggressive pediatric rhabdoid tumors. Pediatr. Blood Cancer. 59, 1155–1157. doi: 10.1002/pbc.24315
Koncz, G., and Hueber, A. O. (2012). The Fas/CD95 receptor regulates the death of autoreactive B cells and the selection of antigen-specific B Cells. Front. Immunol. 3:207. doi: 10.3389/fimmu.2012.00207
Kretzmer, H., Bernhart, S. H., Wang, W., Haake, A., Weniger, M. A., Bergmann, A. K., et al. (2015). DNA methylome analysis in Burkitt and follicular lymphomas identifies differentially methylated regions linked to somatic mutation and transcriptional control. Nat. Genet. 47, 1316–1325. doi: 10.1038/ng.3413
Kuo, T. C., and Schlissel, M. S. (2009). Mechanisms controlling expression of the RAG locus during lymphocyte development. Curr. Opin. Immunol. 21, 173–178. doi: 10.1016/j.coi.2009.03.008
Küppers, R., Klein, U., Hansmann, M. L., and Rajewsky, K. (1999). Cellular origin of human B-cell lymphomas. N. Engl. J. Med. 341, 1520–1529. doi: 10.1056/nejm199911113412007
Kurman, R. J., Young, R. H., Norris, H. J., Main, C. S., Lawrence, W. D., and Scully, R. E. (1984). Immunocytochemical localization of placental lactogen and chorionic gonadotropin in the normal placenta and trophoblastic tumors, with emphasis on intermediate trophoblast and the placental site trophoblastic tumor. Int. J. Gynecol. Pathol. 3, 101–102.
Kwong, W. Y., Adamiak, S. J., Gwynn, A., Singh, R., and Sinclair, K. D. (2010). Endogenous folates and single-carbon metabolism in the ovarian follicle, oocyte and pre-implantation embryo. Reproduction 139, 705–715. doi: 10.1530/REP-09-0517
Kyoda, K., Nakamura, S., Matano, S., Ohtake, S., and Matsuda, T. (1997). Prognostic significance of immunoglobulin heavy chain gene rearrangement in patients with acute myelogenous leukemia. Leukemia 11, 803–806. doi: 10.1038/sj.leu.2400662
Lao, Z. T., Ding, L. W., An, O., Hattori, N., Sun, Q. Y., Tan, K. T., et al. (2019). Mutational and transcriptomic profiling of acute leukemia of ambiguous lineage reveals obscure but clinically important lineage bias. Haematologica 104, e200–e203. doi: 10.3324/haematol.2018.202911
Lenz, G., Nagel, I., Siebert, R., Roschke, A. V., Sanger, W., Wright, G. W., et al. (2007). Aberrant immunoglobulin class switch recombination and switch translocations in activated B cell-like diffuse large B cell lymphoma. J. Exp. Med. 204, 633–643. doi: 10.1084/jem.20062041
Li, A., Rue, M., Zhou, J., Wang, H., Goldwasser, M. A., Neuberg, D., et al. (2004). Utilization of Ig heavy chain variable, diversity, and joining gene segments in children with B-lineage acute lymphoblastic leukemia: implications for the mechanisms of VDJ recombination and for pathogenesis. Blood 103, 4602–4609. doi: 10.1182/blood-2003-11-3857
Li, M. C., Whitmore, W. F., Golbey, R., and Grabstald, H. (1960). Effects of combined drug therapy on metastatic cancer of the testis. JAMA 174, 1291–1299.
Lindhout, E., Lakeman, A., and de Groot, C. (1995). Follicular dendritic cells inhibit apoptosis in human B lymphocytes by a rapid and irreversible blockade of preexisting endonuclease. J. Exp. Med. 181, 1985–1995. doi: 10.1084/jem.181.6.1985
Liu, G. J., Cimmino, L., Jude, J. G., Hu, Y., Witkowski, M. T., McKenzie, M. D., et al. (2014). Pax5 loss imposes a reversible differentiation block in B-progenitor acute lymphoblastic leukemia. Genes Dev. 28, 1337–1350. doi: 10.1101/gad.240416.114
Liu, L., McGavran, L., Lovell, M. A., Wei, Q., Jamieson, B. A., Williams, S. A., et al. (2004). Nonpositive terminal deoxynucleotidyl transferase in pediatric precursor B-lymphoblastic leukemia. Am. J. Clin. Pathol. 121, 810–815. doi: 10.1309/qd18ppv1nh3teutf
Liu, Y. J., Joshua, D. E., Williams, G. T., Smith, C. A., Gordon, J., and MacLennan, I. C. (1989). Mechanism of antigen-driven selection in germinal centres. Nature 342, 929–931. doi: 10.1038/342929a0
Llorian, M., Stamataki, Z., Hill, S., Turner, M., and Mårtensson, I. L. (2007). The PI3K p110delta is required for down-regulation of RAG expression in immature B cells. J. Immunol. 178, 1981–1985. doi: 10.4049/jimmunol.178.4.1981
Lossos, I. S., Alizadeh, A. A., Eisen, M. B., Chan, W. C., Brown, P. O., Botstein, D., et al. (2000). Ongoing immunoglobulin somatic mutation in germinal center B cell-like but not in activated B cell-like diffuse large cell lymphomas. Proc. Natl. Acad. Sci. U.S.A. 97, 10209–10213. doi: 10.1073/pnas.180316097
Lu, C. C., and Meistrich, M. L. (1979). Cytotoxic effects of chemotherapeutic drugs on mouse testis cells. Cancer Res. 39, 3575–3582.
Mack, S. C., Witt, H., Piro, R. M., Gu, L., Zuyderduyn, S., Stütz, A. M., et al. (2014). Epigenomic alterations define lethal CIMP-positive ependymomas of infancy. Nature 506, 445–450. doi: 10.1038/nature13108
Mao, T. L., Kurman, R. J., Huang, C. C., Lin, M. C., and Shih, IeM (2007). Immunohistochemistry of choriocarcinoma: an aid in differential diagnosis and in elucidating pathogenesis. Am. J. Surg. Pathol. 31, 1726–1732. doi: 10.1097/pas.0b013e318058a529
Marjault, H. B., and Allemand, I. (2016). Consequences of irradiation on adult spermatogenesis: Between infertility and hereditary risk. Mutat. Res. 770, 340–348. doi: 10.1016/j.mrrev.2016.07.004
Maul, R. W., and Gearhart, P. J. (2010). Controlling somatic hypermutation in immunoglobulin variable and switch regions. Immunol. Res. 47, 113–122. doi: 10.1007/s12026-009-8142-5
Mayer, C. T., Gazumyan, A., Kara, E. E., Gitlin, A. D., Golijanin, J., Viant, C., et al. (2017). The microanatomic segregation of selection by apoptosis in the germinal center. Science 358, eaao2602. doi: 10.1126/science.aao2602
McHeyzer-Williams, L. J., Milpied, P. J., Okitsu, S. L., and McHeyzer-Williams, M. G. (2015). Class-switched memory B cells remodel BCRs within secondary germinal centers. Nat. Immunol. 16, 296–305. doi: 10.1038/ni.3095
Meistrich, M. L., Wilson, G., Mathur, K., Fuller, L. M., Rodriguez, M. A., McLaughlin, P., et al. (1997). Rapid recovery of spermatogenesis after mitoxantrone, vincristine, vinblastine, and prednisone chemotherapy for Hodgkin’s disease. J. Clin. Oncol. 15, 3488–3495. doi: 10.1200/jco.1997.15.12.3488
Mitchison, T. J. (2012). The proliferation rate paradox in antimitotic chemotherapy. Mol. Biol. Cell. 23, 1–6. doi: 10.1091/mbc.E10-04-0335
Mohrin, M., Bourke, E., Alexander, D., Warr, M. R., Barry-Holson, K., Le Beau, M. M., et al. (2010). Hematopoietic stem cell quiescence promotes error-prone DNA repair and mutagenesis. Cell Stem Cell 7, 174–185. doi: 10.1016/j.stem.2010.06.014
Muramatsu, M., Kinoshita, K., Fagarasan, S., Yamada, S., Shinkai, Y., and Honjo, T. (2000). Class switch recombination and hypermutation require activation-induced cytidine deaminase (AID), a potential RNA editing enzyme. Cell 102, 553–563. doi: 10.1016/s0092-8674(00)00078-7
Murugaesu, N., Schmid, P., Dancey, G., Agarwal, R., Holden, L., McNeish, I., et al. (2006). Malignant ovarian germ cell tumors: identification of novel prognostic markers and long-term outcome after multimodality treatment. J. Clin. Oncol. 24, 4862–4866. doi: 10.1200/jco.2006.06.2489
Oettinger, M. A., Schatz, D. G., Gorka, C., and Baltimore, D. (1990). RAG-1 and RAG-2, adjacent genes that synergistically activate V(D)J recombination. Science 248, 1517–1523. doi: 10.1126/science.2360047
O’Neill, C. (1998). Endogenous folic acid is essential for normal development of preimplantation embryos. Hum. Reprod. 13, 1312–1316. doi: 10.1093/humrep/13.5.1312
Opstelten, D., and Osmond, D. G. (1983). Pre-B cells in mouse bone marrow: immunofluorescence stathmokinetic studies of the proliferation of cytoplasmic mu-chain-bearing cells in normal mice. J. Immunol. 131, 2635– 2640.
Osmond, D. G. (1991). Proliferation kinetics and the lifespan of B cells in central and peripheral lymphoid organs. Curr. Opin. Immunol. 3, 179–185. doi: 10.1016/0952-7915(91)90047-5
Pasqualucci, L., Guglielmino, R., Houldsworth, J., Mohr, J., Aoufouchi, S., Polakiewicz, R., et al. (2004). Expression of the AID protein in normal and neoplastic B cells. Blood 104, 3318–3325. doi: 10.1182/blood-2004-04-1558
Peham, M., Panzer, S., Fasching, K., Haas, O. A., Fischer, S., Marschalek, R., et al. (2002). Low frequency of clonotypic Ig and T-cell receptor gene rearrangements in t(4;11) infant acute lymphoblastic leukaemia and its implication for the detection of minimal residual disease. Br. J. Haematol. 117, 315–321. doi: 10.1046/j.1365-2141.2002.03428.x
Pflug, N., Bahlo, J., Shanafelt, T. D., Eichhorst, B. F., Bergmann, M. A., Elter, T., et al. (2014). Development of a comprehensive prognostic index for patients with chronic lymphocytic leukemia. Blood 124, 49–62. doi: 10.1182/blood-2014-02-556399
Pieters, R., Schrappe, M., De Lorenzo, P., Hann, I., De Rossi, G., Felice, M., et al. (2007). A treatment protocol for infants younger than 1 year with acute lymphoblastic leukaemia (Interfant99): an observational study and a multicentre randomised trial. Lancet 370, 240–250. doi: 10.1016/S0140-6736(07)61126-X
Pinkel, D. (1971). Five-year follow-up of “total therapy” of childhood lymphocytic leukemia. JAMA 216, 648–652. doi: 10.1001/jama.216.4.648
Pritchard, J. R., Bruno, P. M., Gilbert, L. A., Capron, K. L., Lauffenburger, D. A., and Hemann, M. T. (2013). Defining principles of combination drug mechanisms of action. Proc. Natl. Acad. Sci. U.S.A. 110, E170–E179. doi: 10.1073/pnas.1210419110
Pui, C. H., Pei, D., Campana, D., Cheng, C., Sandlund, J. T., Bowman, W. P., et al. (2014). A revised definition for cure of childhood acute lymphoblastic leukemia. Leukemia 28, 2336–2343. doi: 10.1038/leu.2014.142
Pulivarthy, S. R., Lion, M., Kuzu, G., Matthews, A. G., Borowsky, M. L., Morris, J., et al. (2016). Regulated large-scale nucleosome density patterns and precise nucleosome positioning correlate with V(D)J recombination. Proc. Natl. Acad. Sci. U.S.A. 113, E6427–E6436.
Reinhard, H., Reinert, J., Beier, R., Furtwängler, R., Alkasser, M., Rutkowski, S., et al. (2008). Rhabdoid tumors in children: prognostic factors in 70 patients diagnosed in Germany. Oncol. Rep. 19, 819–823.
Rollett, R. A., Wilkinson, E. J., Gonzalez, D., Fenton, J. A., Short, M. A., Evans, P. A., et al. (2006). Immunoglobulin heavy chain sequence analysis in Waldenstrom’s macroglobulinemia and immunoglobulin M monoclonal gammopathy of undetermined significance. Clin. Lymphoma Myeloma 7, 70–72. doi: 10.3816/clm.2006.n.042
Romano, F. J., Rossetti, S., Conteduca, V., Schepisi, G., Cavaliere, C., Di Franco, R., et al. (2016). Role of DNA repair machinery and p53 in the testicular germ cell cancer: a review. Oncotarget 7, 85641–85649. doi: 10.18632/oncotarget.13063
Rowe, J. M. (2010). Prognostic factors in adult acute lymphoblastic leukaemia. Br. J. Haematol. 150, 389–405. doi: 10.1111/j.1365-2141.2010.08246.x
Rowley, M. J., Leach, D. R., Warner, G. A., and Heller, C. G. (1974). Effect of graded doses of ionizing radiation on the human testis. Radiat. Res. 59, 665–678.
Sand, P. K., Stubblefield, P. A., and Ory, S. J. (1986). Methotrexate inhibition of normal trophoblasts in vitro. Am. J. Obstet. Gynecol. 155, 324–329.
Savage, P. (2015). Clinical observations on chemotherapy curable malignancies: unique genetic events. frozen development and enduring apoptotic potential. BMC Cancer 15:11. doi: 10.1186/s12885-015-1006-6
Savage, P., Monk, D., Hernandez Mora, J. R., van der Westhuizen, N., Rauw, J., Tinker, A., et al. (2019). A case of intraplacental gestational choriocarcinoma; characterised by the methylation pattern of the early placenta and an absence of driver mutations. BMC Cancer 19:744. doi: 10.1186/s12885-019-5906-8
Savage, P., Stebbing, J., Bower, M., and Crook, T. (2009). Why does cytotoxic chemotherapy cure only some cancers? Nat. Clin. Pract. Oncol. 6, 43–52. doi: 10.1038/ncponc1260
Schaaf, M., Reiser, M., Borchmann, P., Engert, A., and Skoetz, N. (2012). High-dose therapy with autologous stem cell transplantation versus chemotherapy or immuno-chemotherapy for follicular lymphoma in adults. Cochrane Database Syst. Rev. 1:CD007678.
Schlissel, M. S., Kaffer, C. R., and Curry, J. D. (2006). Leukemia and lymphoma: a cost of doing business for adaptive immunity. Genes Dev. 20, 1539–1544.
Schmid, P., Nagai, Y., Agarwal, R., Hancock, B., Savage, P. M., Sebire, N. J., et al. (2009). Prognostic markers and long-term outcome of placental-site trophoblastic tumours: a retrospective observational study. Lancet 374, 48–55. doi: 10.1016/S0140-6736(09)60618-8
Schulz, H., Bohlius, J. F., Trelle, S., Skoetz, N., Reiser, M., Kober, T., et al. (2007). Immunochemotherapy with rituximab and overall survival in patients with indolent or mantle cell lymphoma: a systematic review and meta-analysis. J. Natl. Cancer Inst. 99, 706–714.
Sehn, L. H., Berry, B., Chhanabhai, M., Fitzgerald, C., Gill, K., Hoskins, P., et al. (2007). The revised International Prognostic Index (R-IPI) is a better predictor of outcome than the standard IPI for patients with diffuse large B-cell lymphoma treated with R-CHOP. Blood 109, 1857–1861.
Shaffer, A. L., Rosenwald, A., and Staudt, L. M. (2002). Lymphoid malignancies: the dark side of B-cell differentiation. Nat. Rev. Immunol. 2, 920–932.
Shah, A., Andersson, T. M., Rachet, B., Björkholm, M., and Lambert, P. C. (2013). Survival and cure of acute myeloid leukaemia in England, 1971-2006: a population-based study. Br. J. Haematol. 162, 509–516. doi: 10.1111/bjh.12425
Sita-Lumsden, A., Short, D., Lindsay, I., Sebire, N. J., Adjogatse, D., Seckl, M. J., et al. (2012). Treatment outcomes for 618 women with gestational trophoblastic tumours following a molar pregnancy at the Charing Cross Hospital, 2000-2009. Br. J. Cancer 107, 1810–1814. doi: 10.1038/bjc.2012.462
Sive, J. I., Buck, G., Fielding, A., Lazarus, H. M., Litzow, M. R., Luger, S., et al. (2012). Outcomes in older adults with acute lymphoblastic leukaemia (ALL): results from the international MRC UKALL XII/ECOG 2993 trial. Br. J. Haematol. 157, 463–471.
Smirnova, S. Y., Sidorova, Y. V., Ryzhikova, N. V., Sychevskaya, K. A., Parovichnikova, E. N., and Sudarikov, A. B. (2016). Evolution of Tumor Clones in Adult Acute Lymphoblastic Leukemia. Acta Nat. 8, 100–109.
Smith, M. A., Seibel, N. L., Altekruse, S. F., Ries, L. A., Melbert, D. L., O’Leary, M., et al. (2010). Outcomes for children and adolescents with cancer: challenges for the twenty-first century. J. Clin. Oncol. 28, 2625–2634. doi: 10.1200/JCO.2009.27.0421
Sonne, S. B., Almstrup, K., Dalgaard, M., Juncker, A. S., Edsgard, D., Ruban, L., et al. (2009). Analysis of gene expression profiles of microdissected cell populations indicates that testicular carcinoma in situ is an arrested gonocyte. Cancer Res. 69, 5241–5250. doi: 10.1158/0008-5472.CAN-08-4554
Sonne, S. B., Kristensen, D. M., Novotny, G. W., Olesen, I. A., Nielsen, J. E., Skakkebaek, N. E., et al. (2008). Testicular dysgenesis syndrome and the origin of carcinoma in situ testis. Int. J. Androl. 31, 275–287. doi: 10.1111/j.1365-2605.2007.00855.x
Spender, L. C., and Inman, G. J. (2011). Inhibition of germinal centre apoptotic programmes by epstein-barr virus. Adv. Hematol. 2011:829525. doi: 10.1155/2011/829525
Stavroyianni, N., Belessi, C., Stamatopoulos, K., Kosmas, C., Paterakis, G., Abazis, D., et al. (2003). Expression of recombination activating genes-1 and-2 immunoglobulin heavy chain gene rearrangements in acute myeloid leukemia: evaluation of biological and clinical significance in a series of 76 uniformly treated patients and review of the literature. Haematologica 88, 268–274.
Sungalee, S., Mamessier, E., Morgado, E., Grégoire, E., Brohawn, P. Z., Morehouse, C. A., et al. (2014). Germinal center reentries of BCL2-overexpressing B cells drive follicular lymphoma progression. J. Clin. Invest. 124, 5337–5351. doi: 10.1172/JCI72415
Szczepański, T., van der Velden, V. H., Raff, T., Jacobs, D. C., van Wering, E. R., Brüggemann, M., et al. (2003). Comparative analysis of T-cell receptor gene rearrangements at diagnosis and relapse of T-cell acute lymphoblastic leukemia (T-ALL) shows high stability of clonal markers for monitoring of minimal residual disease and reveals the occurrence of second T-ALL. Leukemia 17, 2149–2156.
Takai, Y., Matikainen, T., Jurisicova, A., Kim, M. R., Trbovich, A. M., Fujita, E., et al. (2007). Caspase-12 compensates for lack of caspase-2 and caspase-3 in female germ cells. Apoptosis 12, 791–800.
Tellier, J., Menard, C., Roulland, S., Martin, N., Monvoisin, C., Chasson, L., et al. (2014). Human t(14;18)positive germinal center B cells: a new step in follicular lymphoma pathogenesis? Blood 123, 3462–3465. doi: 10.1182/blood-2013-12-545954
Thielen, C., and Heinen, E. (2010). “Germinal Centres,” in eLS, (Hoboken, NJ: John Wiley & Sons, Ltd), doi: 10.1002/9780470015902.a0000517.pub3
Tian, N., Yu, J., Zhang, S., Ma, W. Y., Wang, T., and Wang, Y. M. (2018). Effects of methotrexate on the quality of oocyte maturation in vitro. Eur. Biophys. J. 47, 249–260. doi: 10.1007/s00249-017-1254-2
Todeschini, G., Bonifacio, M., Tecchio, C., Balter, R., Carli, G., Stefani, P. M., et al. (2012). Intensive short-term chemotherapy regimen induces high remission rate (over 90%) and event-free survival both in children and adult patients with advanced sporadic Burkitt lymphoma/leukemia. Am. J. Hematol. 87, 22–25. doi: 10.1002/ajh.22189
Tsujimoto, Y., Gorham, J., Cossman, J., Jaffe, E., and Croce, C. M. (1985). The t(14;18) chromosome translocations involved in B-cell neoplasms result from mistakes in VDJ joining. Science 229, 1390–1393.
Uckun, F. M., Mitchell, J. B., Obuz, V., Park, C. H., Waddick, K., Friedman, N., et al. (1991). Radiation sensitivity of human B-lineage lymphoid precursor cells. Int. J. Radiat. Oncol. Biol. Phys. 21, 1553–1560.
van Dongen, J. J., Langerak, A. W., Brüggemann, M., Evans, P. A., Hummel, M., Lavender, F. L., et al. (2003). Design and standardization of PCR primers and protocols for detection of clonal immunoglobulin and T-cell receptor gene recombinations in suspect lymphoproliferations: report of the BIOMED-2 Concerted Action BMH4-CT98-3936. Leukemia 17, 2257– 2317.
Victora, G. D., Schwickert, T. A., Fooksman, D. R., Kamphorst, A. O., Meyer-Hermann, M., Dustin, M. L., et al. (2010). Germinal center dynamics revealed by multiphoton microscopy with a photoactivatable fluorescent reporter. Cell 143, 592–605. doi: 10.1016/j.cell.2010.10.032
Viviani, S., Zinzani, P. L., Rambaldi, A., Brusamolino, E., Levis, A., Bonfante, V., et al. (2011). ABVD versus BEACOPP for Hodgkin’s lymphoma when high-dose salvage is planned. N. Engl. J. Med. 365, 203–212. doi: 10.1056/NEJMoa1100340
von Levetzow, C., Jiang, X., Gwye, Y., von Levetzow, G., Hung, L., Cooper, A., et al. (2011). Modeling initiation of Ewing sarcoma in human neural crest cells. PLoS One 29:e19305. doi: 10.1371/journal.pone.0019305
Vose, J., and Armitage, J. (2008). International T-Cell Lymphoma Project, Weisenburger D. International peripheral T-cell and natural killer/T-cell lymphoma study:pathology findings and clinical outcomes. J. Clin. Oncol. 26, 4124–4130.
Weinberg, O. K., Hasserjian, R. P., Baraban, E., Ok, C. Y., Geyer, J. T., Philip, J. K., et al. (2019). Clinical, immunophenotypic, and genomic findings of acute undifferentiated leukemia and comparison to acute myeloid leukemia with minimal differentiation: a study from the bone marrow pathology group. Mod. Pathol. 32, 1373–1385. doi: 10.1038/s41379-019-0263-3
Xing, D., Zheng, G., Pallavajjala, A., Schoolmeester, J. K., Liu, Y., Haley, L., et al. (2019). Lineage-Specific alterations in gynecologic neoplasms with Choriocarcinomatous differentiation: implications for origin and therapeutics. Clin. Cancer Res. 25, 4516–4529. doi: 10.1158/1078-0432.CCR-18-4278
Xu-Monette, Z. Y., Li, J., Xia, Y., Crossley, B., Bremel, R. D., Miao, Y., et al. (2019). Immunoglobulin somatic hypermutation has clinical impact in DLBCL and potential implications for immune checkpoint blockade and neoantigen-based immunotherapies. J. Immunother. Cancer 7:272. doi: 10.1186/s40425-019-0730-x
Yoneda, N., Tatsumi, E., Kawano, S., Matsuo, Y., Minowada, J., and Yamaguchi, N. (1993). Human recombination activating gene-1 in leukemia/lymphoma cells: expression depends on stage of lymphoid differentiation defined by phenotype and genotype. Blood 82, 207–216.
Zaheen, A., Boulianne, B., Parsa, J. Y., Ramachandran, S., Gommerman, J. L., and Martin, A. (2009). AID constrains germinal center size by rendering B cells susceptible to apoptosis. Blood 114, 547–554. doi: 10.1182/blood-2009-03-211763
Zan, H., and Casali, P. (2015). Epigenetics of peripheral B-cell differentiation and the antibody response. Front. Immunol. 6:63. doi: 10.3389/fimmu.2015.00631
Zuckerman, N. S., McCann, K. J., Ottensmeier, C. H., Barak, M., Shahaf, G., Edelman, H., et al. (2010). Ig gene diversification and selection in follicular lymphoma, diffuse large B cell lymphoma and primary central nervous system lymphoma revealed by lineage tree and mutation analyses. Int. Immunol. 22, 875–887. doi: 10.1093/intimm/dxq441
Keywords: cancer, chemotherapy, cure, phenotype, apoptosis
Citation: Savage P (2020) Chemotherapy Curability in Leukemia, Lymphoma, Germ Cell Tumors and Gestational Malignancies: A Reflection of the Unique Physiology of Their Cells of Origin. Front. Genet. 11:426. doi: 10.3389/fgene.2020.00426
Received: 12 February 2020; Accepted: 06 April 2020;
Published: 09 June 2020.
Edited by:
Shicheng Guo, University of Wisconsin–Madison, United StatesReviewed by:
Alexander E. Berezin, Zaporizhzhia State Medical University, UkraineParvin Mehdipour, Tehran University of Medical Sciences, Iran
Copyright © 2020 Savage. This is an open-access article distributed under the terms of the Creative Commons Attribution License (CC BY). The use, distribution or reproduction in other forums is permitted, provided the original author(s) and the copyright owner(s) are credited and that the original publication in this journal is cited, in accordance with accepted academic practice. No use, distribution or reproduction is permitted which does not comply with these terms.
*Correspondence: Philip Savage, savage13561@msn.com