- 1Key Laboratory of Birth Defects and Related Diseases of Women and Children of MOE, Department of Pediatrics, West China Second University Hospital, Sichuan University, Chengdu, China
- 2Department of Pediatrics, The First People’s Hospital of Shuangliu District, West China Airport Hospital of Sichuan University, Chengdu, China
- 3Department of Pediatrics, The Second People’s Hospital of Liangshan Yi Autonomous Prefecture, Xichang, China
- 4Key Laboratory of Birth Defects and Related Diseases of Women and Children of MOE, Department of Obstetrics and Gynecology, West China Second University Hospital, Sichuan University, Chengdu, China
Background: Fetal bradycardia is a common but severe condition. In addition to autoimmune-mediated fetal heart block, several types of channelopathies induce high-degree atrioventricular block (AVB). Long QT syndrome (LQTS) is a major cause of non-autoimmune-mediated fetal heart block. Due to the limitations of prenatal diagnostic technologies, LQTS is seldom identified unless fetal genetic screening is performed. Thus, long-term prenatal dexamethasone (DEX) exposure can become a challenge for these patients. We report on a rare case of a novel KCNH2 variant related to LQTS and associated with high-degree fetal AVB with long-term DEX exposure. This case led us to review our prenatal administration strategy for such patients.
Case Presentation: A fetus was identified with high-degree AVB (2:1 transduction at 28 + 2 gestational weeks). Typical tests of immune function in the pregnant woman were conducted including tests for thyroid function, rheumatic screening, autoimmune antibodies (such as anti-Ro/SSA and anti-La/SSB), and anti-nuclear antibodies (anti-ANA). Following the recommended protocol, the pregnant patient received DEX (0.75 mg/day) during pregnancy. Subsequently, the fetal AVB changed from 2:1 to prolonged AV intervals with ventricular tachycardia, which suggested a therapeutic benefit of DEX in some respects. However, a high-degree AVB with a significantly prolonged QTc interval was identified in the neonate following birth. Genetic testing revealed that a KCNH2 c.1868C>A variant induced LQTS. The body length remained approximately -3.2 SD from the reference value after prenatal long-term DEX exposure, which indicated a developmental restriction. Additionally, the functional validation experiments were performed to demonstrate the prolonged duration of calcium transit both in depolarization and repolarization with the KCNH2 c.1868C>A variant.
Conclusion: Genetic screening should be recommended in fetuses with autoimmune antibody negative high-degree AVB, especially for 2:1 transduction AVB and in fetuses with changes in fetal heart rhythm following initial DEX treatment. Genetic screening may help identify genetic variant–related channelopathies and avoid unexpected prenatal exposure of DEX and its possible long-term adverse postnatal complications.
Introduction
Fetal heart block is a rare and threatening disease and is the most commonly observed type of fetal bradycardia. Among all fetal heart block patients, > 50% of cases are associated with maternal autoimmune diseases, and the incidence of fetal heart block in pregnancies with positive anti–SSA(Ro) and anti–SSB(La) test results is 2%–5% (Manolis et al., 2020). Fetuses with positive antibody screens represent an estimated 25% of neonatal lupus patients. Studies suggest that transplacental anti-nuclear antibodies (ANAs) may attack the cardiac conduction system of the fetus. Suryawanshi et al. (2020) used single-cell RNA-sequencing to determine the immunological activity of immune cells in human heart samples that were positive for anti-SSA/Ro and associated with congenital heart block (CHB). Researchers found increased and heterogeneous interferon responses in various cell types of the CHB heart compared with healthy controls, which contributed to subsequent fibrosis in the heart. Thus, in autoimmune antibody–positive pregnancies, dexamethasone (DEX) is typically administered to prevent the progression of or to cause the reversal of fetal heart block. However, there are also a large number of patients with fetal heart block without positive antibodies. Thus, the administration strategy for DEX treatment has always been challenging when weighing the benefits of DEX treatment on antibody-negative fetuses (Liao et al., 2021; Tang et al., 2021). According to the current therapeutic protocol, DEX is most recommended for such patients; yet the risks of long-term DEX administration is an ongoing debate.
Attempts have been made to identify potential causes of fetal heart block. Studies have reported that long QT syndrome (LQTS) may present as severe fetal bradycardia or as fetal heart block with a 2:1 transduction (Adler et al., 2020). LQTS does not require DEX treatment, and it is difficult to distinguish between LQTS with heart block and autoimmune-associated fetal heart block that require DEX treatment during pregnancy unless fetal genetic testing is performed to identify genetic variant–related arrhythmias (Martínez-Barrios et al., 2022). Moreover, cases with negative antibody screens commonly present postnatally with adverse arrhythmias including LQTS and high-degree AVB. LQTS is considered a clinical disorder with a genetic origin and is characterized by delayed repolarization of cardiomyocytes, electrocardiographic (ECG) QT prolongation, an elevated risk of syncope, and sudden cardiac death caused by polymorphic ventricular tachycardia that is known as torsades des pointes (TdP) (Tang et al., 2021). The loss of function variants in KCNH2 are the leading cause of LQTS (Liao et al., 2021). Three encoding ion channel genes have been identified as responsible for most of the LQTS cases: KCNQ1 (Kv7.1 channel) causing LQT1 (Adler et al., 2020), KCNH2 (Kv11.1 channel) causing LQT2 (Martínez-Barrios et al., 2022), and SCN5A (Nav1.5 channel) causing LQTS (Dai et al., 2021). Thus, the early and timely ability to distinguish congenital LQTS and autoimmune-associated fetal heart block is critical to avoid unnecessary long-term prenatal DEX exposure.
Herein, we report on a rare case of a novel KCNH2 variant-related LQTS with an associated high-degree fetal AVB. After prenatal DEX administration, only a prolonged AV interval was identified in utero. Thus, the fetus received long-term DEX exposure. Postnatal ECG and genetic testing revealed that the neonate had LQTS and a de novo KCNH2 variant, respectively. This case led us to review our prenatal DEX administration strategy for patients and suggests that genetic testing should be conducted in autoimmune antibody-negative fetuses to avoid long-term DEX exposure given that DEX treatment leads to improved outcomes.
Case presentation
Ethical compliance
This report was approved by the ethics committee of the West China Second Hospital of Sichuan University (approval number 2014-034). Informed consent was obtained from the patient’s parents prior to performing whole-exon sequencing and for the inclusion of the patient’s clinical and imaging details in subsequent publications.
History of illness
A 30-year-old pregnant woman was admitted to our center at 28 + 0 gestational weeks for a general fetal screening, and a fetal ultrasound demonstrated fetal bradycardia. Fetal echocardiography was then performed at 28 + 2 gestational weeks to determine if the fetus suffered from a high-degree AVB (2:1 transduction). The fetal echocardiography demonstrated a normal intracardiac structure and normal cardiac function with a 10 out of 10 cardiovascular profile score. Tests for immune activity in pregnant women were performed including thyroid function, rheumatic screening, autoimmune antibodies (such as anti-Ro/SSA and anti-La/SSB), and ANA. All aforementioned tests were negative. In addition, a test was performed for potential viral infections, including tests for adenovirus, coxsackie virus, and B-19 virus, and all were negative. Following the recommended protocol, the pregnant patient received DEX (0.75 mg/day) during pregnancy. Subsequently, the fetal AVB changed from 2:1 to a prolonged AV interval with ventricular tachycardia, which suggests a therapeutic benefit of DEX in some aspects in the pregnant woman administrated DEX (beginning at 4.5 mg/day with a dose reduction at 34 + 3 gestational weeks when the 2:1 transduction AVB changed to prolonged AV with an interval of 147 ms) until delivery at 37 + 0 gestational weeks. Following birth, the neonate was confirmed to have a significantly prolonged QTc interval with high-degree AVB (2:1 transduction). Transient ventricular tachycardia was identified using a Holter monitor. The neonate’s parents had no positive or related family history of arrhythmia, cardiomyopathy, congenital heart disease, or coronary artery disease. The ECGs assessments of the parents presented normal performance (Supplementary Figure S1).
The first intrapartum fetal echocardiography exam was performed at 28 + 2 gestational weeks, and the development of the four cardiac chambers and valvar movements were normal. The atrial rate was 135 beats/min, while the ventricular rate was 68 beats/min (Figures 1A and B), and the cardiovascular system profile was normal (10/10 points). Thus, a diagnosis of fetal AVB (second degree, type II) was identified. The maternal autoimmune antibody test results were negative for anti–Ro/SSA (0.8 RU/mL, n.v. < 20 RU/mL), anti–La/SSB (<0.5 RU/mL, n.v. < 20 RU/mL), and ANAs (negative, n.v. < 1:100). The IgM result for adenovirus, coxsackie virus, and B-19 were all negative. Next, DEX (4.5 mg/day) was provided to the mother. During fetal follow-up at 34 + 3 gestational weeks, a fetal AVB of 2:1 transduction changed into a first-degree AVB with a prolonged AV interval (147 ms) as the ventricular rate was 130–142 beats/min and the cardiovascular system profile remained normal. Thus, the DEX treatment seemed to confer some benefits in this case. At this point, the dosage of DEX was reduced to 1.5 mg/day until delivery.
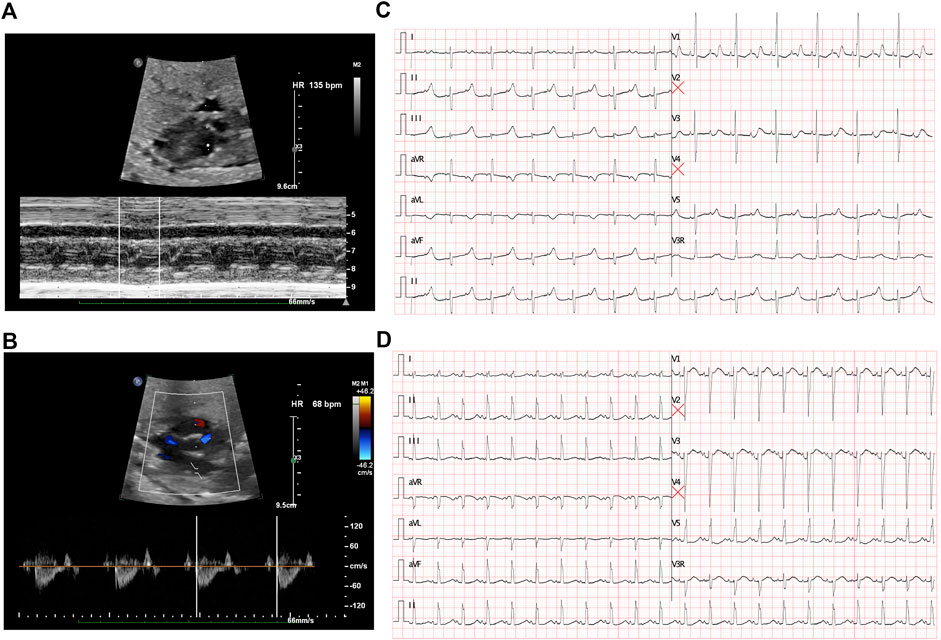
FIGURE 1. Fetal echocardiography to determine bradycardia. (A) M-mode echocardiography demonstrated an atrial rate of 135 beats per minute before treatment. (B) Doppler demonstrated a ventricular rate of 68 beats per minute before treatment and indicated a 2:1 AV block. (C) ECG presented a 2:1 transduction atrioventricular block postnatally. (D) ECG revealed a significant prolonged QTc interval after birth.
A cesarean section was performed at 37 + 0 gestational weeks. After birth, bradycardia was also documented, and the ventricular rate was approximately 65 beats/min with an acceptable SpO2 of approximately 98%. An ECG of the neonate demonstrated a 2:1 transduction AVB (Figure 1C). One week later, the ECG revealed a significantly prolonged QTc interval (498–534 ms, Figure 1D). Moreover, Holter monitoring demonstrated transit ventricular tachycardia (Figure 2A) and doublet premature ventricular contractions (Figure 2B). A slight elevation of cTnI was also observed (0.162 ug/L, n.v. < 0.06 ug/L). The autoimmune antibody test results of the neonate were negative. ECG confirmed the presence of a patent foramen ovale, tricuspid regurgitation (mild) with an ejection fraction of 71%, and fractional shortening of 38%. Thus, a diagnosis of LQTS was suspected for this patient, and whole-exon sequencing was performed to identify any potential genetic variants. This was the first child between the couple.
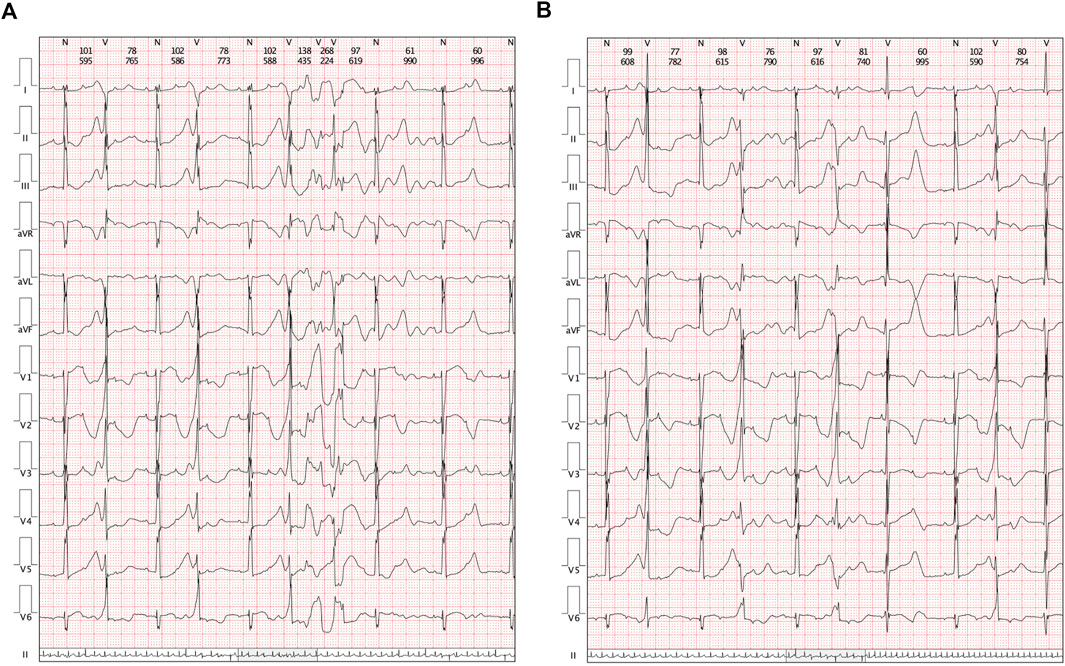
FIGURE 2. Clinical manifestations in Holters. (A) Transit ventricular tachycardia was identified. (B) Holter demonstrated a paired premature ventricular contraction.
Molecular results
To evaluate any potential genetic causes of the neonate’s condition, a peripheral blood sample was obtained from the patient in an ethylenediaminetetraacetic acid anticoagulant blood sample tube and stored at 4 °C for <6 h. DNA was extracted using the Blood Genome Column Medium Extraction Kit (Tiangen Biotech, Beijing, China) in accordance with the manufacturer’s instructions. Protein-coding exome enrichment was performed using the xGen Exome Research Panel v.1.0, which is composed of 429,826 individually synthesized and quality-controlled probes targeting 49.11 Mb of protein-coding regions (>23,000 genes) of the human genome. Whole-exon sequencing was performed using the NovaSeq 6000 platform (Illumina, San Diego, CA, USA), and the raw data were processed using FastP to remove adapters and filter out low-quality reads. Paired-end reads were aligned with the Ensembl GRCh38/hg38 reference genome using the Burrows–Wheeler Aligner tool. Variant annotation was performed in accordance with database-sourced minor allele frequencies (MAFs) and practical guidelines on pathogenicity that were issued by the American College of Medical Genetics. The annotation of MAFs was performed based on the 1000 Genomes, dbSNP, ESP, ExAC, Provean, Sift, Polypen2_hdiv, Polypen2_hvar, and Chigene in-house MAF databases using the R software program (R Foundation for Statistical Computing, Vienna, Austria). A novel mutation in KCNH2 was finally identified. While the parents also received WES analysis, and both of them were absent of KCNH2 c.1868C>A variant. The family pedigree tree of this proband had been presented in (Figure 3A). To our knowledge, KCNH2 c.21868C>A has never before been reported in the 1000G and ExAC databases (Figure 3B). There was no other cardiomyopathy or arrhythmia-related genetic mutation identified by our whole-exon sequencing analysis. Besides, all the genetic variants related with sudden cardiac death (SCD) had been ruled out. As the parents of this neonate did not present with any symptoms of cardiovascular disease, the neonate was considered to be the proband.
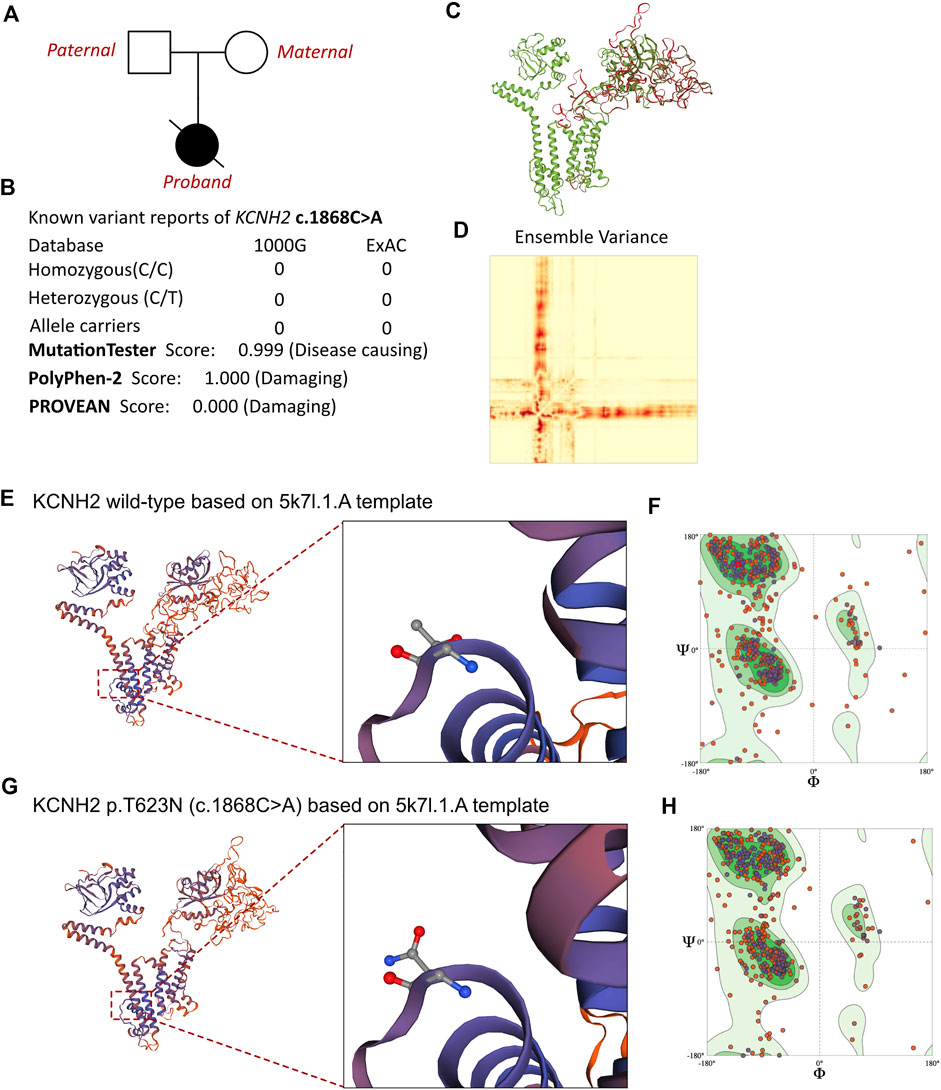
FIGURE 3. Effects of KCNH2 c.1868C>A mutation on molecular protein structure. (A) Family pedigree tree of this proband. (B) Summary of current reports on the individuals of KCNH2 and its predicted damages on the molecular function of the c.1868C>A variant. (C) SWISS-MODEL to predict the variant’s wild type and mutated protein crystal structure, and the variant impaired a large proportion of molecular structure. (D) Ensemble variance between the wild type and mutant protein structure. (E,G) SWISS-MODEL to predict the variant’s wild-type and mutated protein crystal structures using 5k7l.1.A template, and structural changes were identified. (F) Ramachandran plots of wild-type KCNH2. (H) Ramachandran plots of KCNH2 with the p.T623N variant.
MutationTaster and Polyphen-2 were used with the R software to predict the pathogenicity of KCNH2 c.1868C>A and assess the effects of this mutation on the protein structure (Figure 3B). As there were KCNH2 protein crystal structure data available, analysis was performed using SWISS-MODEL (https://swissmodel.expasy.org/) with the 5k7l.1.A template (Figures 3E,G). The capability of the protein structure was estimated using Ramachandran plots (Figures 3F,H). The differences between wild-type and KCNH2 p.T623N mutant crystal structures were estimated by ensemble variance (Figures 3C,D). Changes in the free energy of the model were estimated using the mutation cutoff scanning matrix (mCSM) method (http://biosig.unimelb.edu.au/mcsm/). The signature vector that was ultimately generated was used to train the predictive classification and regression model for calculating the change in the Gibbs folding free energy induced by the mutations.
Based on laboratory analyses and the neonate’s clinical manifestations, a genetic disorder was strongly suspected. Whole-exon sequencing was performed using the Illumina NovaSeq 6000 platform, and a de novo c.1868C>A (p.T623N) heterozygous mutation was identified in the KCNH2 gene. Neither of the neonate’s parents carried this variant. According to the American College of Medical Genetics, these variants have an uncertain pathogenicity (PM1+PM2_Supporting + PP3). The variant we identified, KCNH2 c.1868C>A, had not been reported in any population. This is the first report of this variant. An analysis performed with MutationTester revealed that this mutation is considered pathogenic due to amino acid sequence changes, the protein features affected, and a loss of helix superstructure (probability = 0.999 for c.1868C>A). PolyPhen 2.0 predicted this mutation of p.T623N to be “probably damaging” (score = 1.0, sensitivity = 0.00, and specificity = 1.00). The SWISS-MODEL tool was used to analyze stability after amino acid changes. Ramachandran plots indicated that amino acid positions were altered (Figures 3F,H). Rebuilding the molecular structure based on a 5k7l.1.A template resulted in the identification of residue changes at amino acid position 623 (Figures 3E,G). Using SWISS-MODEL protein stability prediction tools, ensemble changes among the encoded amino acids exhibited significant variance. Three types of calculation methods all demonstrated significant destabilizing changes (mCSM = −0.338 kcal/mol).
To validate the functional impairment of KCNH2 c.1868C>A, two kinds of overexpression plasmids were established as wild type human KCNH2 (KCNH2-wt) and c.1868C>A allele KCNH2 (KCNH2-mut) based on the pgl3 basic vector, and both KCNH2 molecules (wt and mut) were liganded with mScarlet by P2A (pgl3-KCNH2-wt-P2A-mScarlet and pgl3-KCNH2-mut-P2A-mScarlet). Then neonatal mice ventricular myocytes (NMVMs) were isolated using the Neomyt Kit (Cellutron, NC-6031) and plated on 1% Matrigel (Corning, 354234)-coated plates in cardiomyocyte culture media (low glucose DMEM (Gibco), 5% horse serum (American Type Culture Collection, 30–2040), and 2% chicken embryo extract (VWR, 100356-958)). The following day, two plasmids were transfected into NMVMs. Protein was extracted by the RIPA lysis buffer system (Santa Cruz Biotechnology, sc-24948) with Mini Protease Inhibitor Cocktail Tablets (cOmplete, 4693124001). The expression of KNCH2 was assessed by Western Blotting of mScarlet (Figure 4A). NMVMs were harvested on the 7th culture day and fixed using paraformaldehyde. Then, immunofluorescence was performed to demonstrate the expression of transfected KCNH2 (mScarlet), while cardiomyocytes were stained with SAA (Figure 4B). Intracellular Ca2+ recordings were performed after loading with Fluo-4 AM (10 μM, Invitrogen, F14217) for 30 min. After loading, NMVMs were subsequently washed with a normal Tyrode solution (140 mM NaCl; 4 mM KCl; 1 mM MgCl2; 1.8 mM CaCl2; 10 mM Glucose; and 5 mM HEPES, pH 7.4, adjusted with NaOH) to remove the excess dye for 20 min. All image data were acquired using an FV3000 confocal microscope (Figure 4C), which demonstrated the prolonged duration of pgl3-KCNH2-mut-P2A-mScarlet infected NMVMs both in time to peak and decay50 (Figure 4D). Thus, this experiment validated the impaired KCNH2 function-carrying c.1868C>A allele.
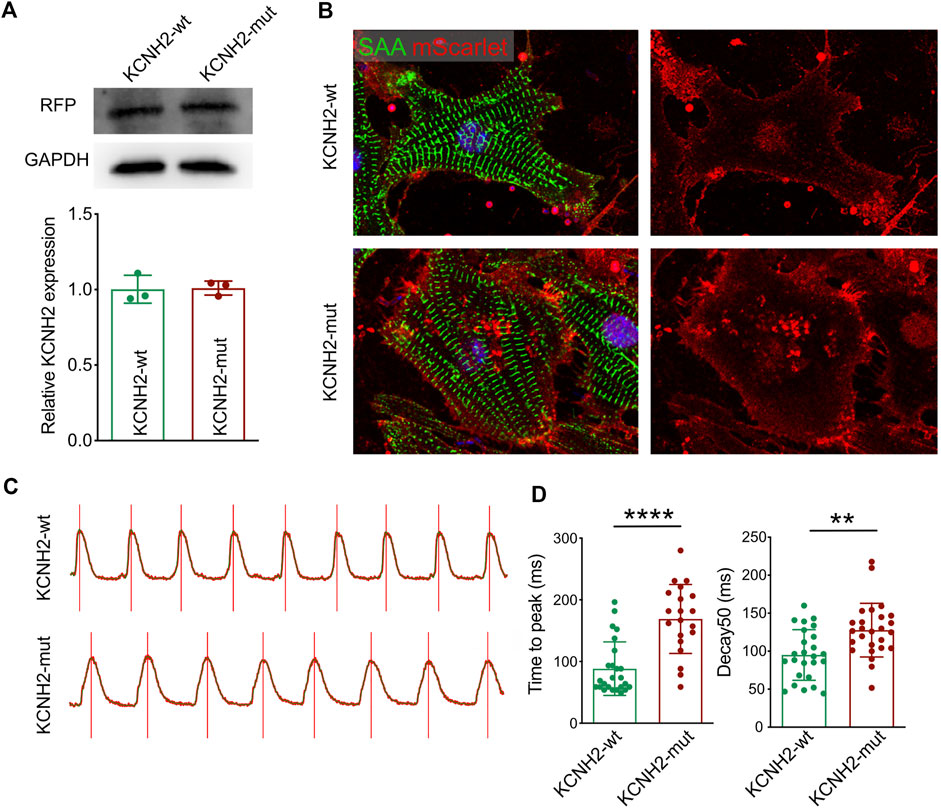
FIGURE 4. Functional validation of KCNH2 c.1868C>A mutation on cardiomyocyte. (A) Western blotting demonstrated the similar expression of KCNH2 protein between wild type and mutation. (B) Immunofluorescence staining revealed the successful infection of KCNH2 overexpression plasmids in cardiomyocytes. (C) Calcium transit waves between KCNH2-wt and KCNH2-mut plasmids transfected cardiomyocytes. (D) The increased duration of time to peak and decay50 was identified in KCNH2-mut cardiomyocytes. RFP, red fluorescence protein; SAA, sarcomeric α-actinin. **, p < 0.01; ****, p < 0.0001.
Outcome and follow-up
After the neonate was transferred to the cardiac intensive care unit, a temporary pacemaker was implanted for the positive treatment. Once the genetic result of KCNH2 c.1868C>A was identified, the neonate remained at an extremely high risk of SCD. Thus, a permanent pacemaker was a therapeutic alternative. The neonate’s parents agreed to the implantation of a permanent pacemaker, and thus, the patient planned to receive an epicardial pacemaker implantation between 3 and 4 months. Additionally, her parents declined to have any medication administration. During two months of postnatal follow-up, the patient did not suffer from syncope or heart dysfunction. However, the body length remained approximately -3.2 SD from the reference value following prenatal long-term DEX exposure, which revealed a developmental restriction. Unfortunately, the patient died from SCD around 2.5 months of age before the scheduled date of pacemaker implantation. Suspected fatal arrhythmia, such as torsades de pointes due to LQTS, was likely the main reason for the induction of death.
Discussion
Inherit arrhythmias lead to higher risks of heart dysfunction, even SCD, in early childhood (Dai et al., 2021). However, most types of inherit arrhythmias have not been identified in fetuses, which indicates a low intrauterine penetrance. Generally, maternal autoimmune diseases impair the conduction system by transplacental transfusion of immune antibodies that attack the heart conduction system and result in localized fibrosis (Suryawanshi et al., 2020). Maternal autoimmune diseases and autoimmune antibodies, especially high-titer anti–SSB/La antibodies and particularly when accompanied by high-titer anti–SSA/Ro antibodies, may cause congenital AVB, sinoatrial node dysfunction, ventricular and junctional tachycardias, and long QT intervals (Wainwright et al., 2020). Indeed, autoimmune factors contribute to more than half of fetal bradycardia cases. However, a large proportion of fetal bradycardia cases lack any evidence of immune involvement. The DEX administration strategy for such cases is critical and challenging.
Congenital LQTS, which is an autoimmune antibody-negative fetal bradycardia, is a cause of fetal AVB (Otsuki et al., 2020). The occurrence of AVB in concert with LQTS is not due to disease of the conduction system itself but rather due to the prolonged ventricular repolarization time that leads to atrial activation before ventricular repolarization is complete (Batra and Balaji, 2019). Generally, QT interval prolongation is responsible for bradycardia (Oka et al., 2010). In a report from Garson et al. that included a series of 287 patients with LQTS, there were 15 patients (5%) with AVB, 13 patients with 2:1 AVB, and only 2 (0.7%) patients with congenital AVB (Mendoza et al., 2010). Early awareness of LQTS is critical in preventing adverse clinical outcomes. Once AVB induced by QT prolongation occurs, it carries a high risk of TDP, which is closely associated with SCD (Rosso et al., 2014). Intrauterine LQTS-induced 2:1 transduction AVB is associated with higher mortality due to fetal hydrops and cardiac dysfunction. In such cases, postnatal genetic screening is recommended by the American Heart Association (AHA) to reach a definitive diagnosis as most conditions of congenital LQTS do not require any treatment during infancy; treatment is only recommended at the onset of ventricular tachycardia or TDP.
According to most protocols or guidelines of fetal arrhythmia management, LQTS should be highly suspected in fetal bradycardia cases, especially for the 2:1 transduction AVB (Kasar et al., 2019), and postnatal genetic screening is recommended to reach a definitive diagnosis. However, if there is no positive family history, it may be difficult to distinguish LQTS from other types of fetal bradycardia using fetal ECG. AHA guidelines recommend fetal magnetocardiography or ECG to monitor the QTc interval; however, there is still a gap in illustrating the actual QTc interval. Fetal therapy with DEX is strongly recommended for autoimmune-mediated fetal bradycardia. DEX treatment of fetuses with established CHB and without heart failure might also be considered with the goal of improving survival or reducing the incidence of dilated cardiomyopathy. The usefulness of DEX therapy in this population has not been established given that current studies have been retrospective and nonrandomized and have had an incomplete follow-up. Generally, DEX is also provided to autoimmune antibody-negative fetuses with DEX dosage reduction based on whether the fetal CHB may be terminated or unchanged after four weeks of treatment. Moreover, if the high-degree fetal AVB changed to first-degree AVB or non-threatening fetal bradycardia, a small dosage of DEX is always continuously administrated to birth. Recently, the long-term adverse effects of prenatal DEX exposure have been noted by researchers (Pal et al., 2022; Shinwell et al., 2022; Wang et al., 2022). In experimental animals, pulmonary fibrosis, osteoporosis, long bone growth impairment, and neonatal hypoglycemia were identified following prenatal DEX exposure (Rivet et al., 2022; Wu et al., 2022; Xie et al., 2022; Zhang et al., 2022). Mechanistically, circadian rhythms, insulin-like growth factor 1 signaling, angiotensin II signaling, histone modifications, and dysregulation of miRNAs were responsible for long-term adverse presentations following DEX exposure (Han et al., 2022; Liu et al., 2022; Madhavpeddi et al., 2022; Murray et al., 2022; Qiu et al., 2022). Thus, the benefits from prenatal DEX administration for autoimmune antibody-negative patients are still being debated.
LQTS is a type of inherit cardiovascular disease that induces the prolonged duration of QT interval. Besides, some cases also demonstrated that LQTS presents as AVB (Aziz et al., 2010; Oka et al., 2010; Sun et al., 2022). It has been considered that AVB could be due to LQTS as a kind of pseudo AVB. The variant of KCNH2 would lead to the loss-of-function of this molecule. The delay repolarization would cause the next beating signaling from atrioventricular node to be irresponsive, which would induce the 2:1 AVB. In this case, the fetus presented with 2:1 transduction AVB at the first fetal ECG, and no positive results had been identified for autoimmune antibodies or potential maternal-related disease screenings. However, the patient initially received DEX therapy, and the high-degree AVB regressed into a mild prolonged AV interval, indicating some positive effects of the DEX treatment. Thus, continuous small dose DEX administration was provided until delivery. After birth, LQTS was confirmed by ECG and genetic screening. Unfortunately, the neonate developed growth restriction postnatally. Subsequently, the case was brought to us to review the treatment strategy for prenatal CHB without positive evidence of autoimmune antibodies and for a critical discussion of the benefits of DEX exposure. Importantly, prenatal genetic screening should be highly recommended for autoimmune antibody-negative fetuses to exclude any genetic syndromes and channelopathies. With the rapid development of next generation sequencing technologies, the expense and duration of such methods have been significantly reduced and allow timely and convincing molecular results. Additionally, the shifted diagnostic timepoint of LQTS or other types of channelopathies may help to avoid unnecessary DEX prenatal exposure.
Conclusion
Prenatal management of fetal CHB with DEX benefits neonates with high-degree AVB and positive autoimmune antibody tests. However, genetic screening should be recommended for fetuses with autoimmune antibody-negative high-degree AVB, especially those with 2:1 AVB and in those cases in which changes in fetal heart rhythm occur with initial DEX treatment. Genetic screening may help identify genetic variant–related channelopathies and is critical for avoiding unexpected prenatal DEX exposure and its long-term adverse postnatal complications.
Data availability statement
The original contributions presented in the study are included in the article/Supplementary Material; further inquiries can be directed to the corresponding authors.
Ethics statement
The studies involving human participants were reviewed and approved by the Ethics Committee of West China Second Hospital of Sichuan University. Written informed consent to participate in this study was provided by the participants’ legal guardian/next of kin. Written informed consent was obtained from the individual(s), and minor(s)’ legal guardian/next of kin, for the publication of any potentially identifiable images or data included in this article.
Author contributions
YL, LW, HY, HY, and KZ were the patient’s physicians. HH and QZ reviewed the literature and contributed to manuscript drafting. YL performed the mutation analysis. SJ performed the functional validation experiments. YL, KZ, and YH conceptualized and designed the study, coordinated and supervised data collection, and critically reviewed the manuscript for important intellectual content. KZ, YL, and HY were responsible for the revision of the manuscript for important intellectual content. All authors issued final approval for the version submitted.
Funding
This work was supported by grants from the Technology Project of Sichuan Province of China (2021YFQ0061 and 2021YJ0211) and Central Government Funds of Guiding Local Scientific and Technological Development for Sichuan Province (2021ZYD0105).
Conflict of interest
The authors declare that the research was conducted in the absence of any commercial or financial relationships that could be construed as a potential conflict of interest.
Publisher’s note
All claims expressed in this article are solely those of the authors and do not necessarily represent those of their affiliated organizations, or those of the publisher, the editors, and reviewers. Any product that may be evaluated in this article, or claim that may be made by its manufacturer, is not guaranteed or endorsed by the publisher.
Supplementary material
The Supplementary Material for this article can be found online at: https://www.frontiersin.org/articles/10.3389/fgene.2022.1010078/full#supplementary-material
Abbreviations
ANAs, anti-nuclear antibodies; AVB, atrioventricular block; CAVB, complete atrioventricular block; DEX, dexamethasone; ECG, electrocardiogram; LQTS, long QT syndrome; MAFs, minor allele frequencies; SCD, sudden cardiac death; SDM, site-directed mutator; TDP, torsades de pointes.
References
Adler, A., Novelli, V., Amin, A. S., Abiusi, E., Care, M., Nannenberg, E. A., et al. (2020). An international, multicentered, evidence-based reappraisal of genes reported to cause congenital long QT syndrome. Circulation 141 (6), 418–428. doi:10.1161/circulationaha.119.043132
Aziz, P. F., Tanel, R. E., Zelster, I. J., Pass, R. H., Wieand, T. S., Vetter, V. L., et al. (2010). Congenital long QT syndrome and 2:1 atrioventricular block: An optimistic outcome in the current era. Heart rhythm. 7 (6), 781–785. doi:10.1016/j.hrthm.2010.02.035
Batra, A. S., and Balaji, S. (2019). Fetal arrhythmias: Diagnosis and management. Indian Pacing Electrophysiol. J. 19 (3), 104–109. doi:10.1016/j.ipej.2019.02.007
Dai, Y., Yin, R., Yang, L., and Li, Z. H. (2021). Clinical and genetic spectrum of neonatal arrhythmia in a NICU. Transl. Pediatr. 10 (10), 2432–2438. doi:10.21037/tp-21-233
Han, H., Xiao, H., Wu, Z., Liu, L., Chen, M., Gu, H., et al. (2022). The miR-98-3p/JAG1/Notch1 axis mediates the multigenerational inheritance of osteopenia caused by maternal dexamethasone exposure in female rat offspring. Exp. Mol. Med. 54 (3), 298–308. doi:10.1038/s12276-022-00743-x
Kasar, T., Kafali, H. C., and Ergul, Y. (2019). A rare association: First degree AV block and long QT syndrome. Cardiol. Young 29 (5), 697–698. doi:10.1017/s1047951119000775
Liao, H., Tang, C., Qiao, L., Zhou, K., Hua, Y., Wang, C., et al. (2021). Prenatal management strategy for immune-associated congenital heart block in fetuses. Front. Cardiovasc. Med. 8, 644122. doi:10.3389/fcvm.2021.644122
Liu, Y., Liu, M., Liu, Y., and Wang, H. (2022). MiR-466b-3p/HDAC7 meditates transgenerational inheritance of testicular testosterone synthesis inhibition induced by prenatal dexamethasone exposure. Biochem. Pharmacol. 199, 115018. doi:10.1016/j.bcp.2022.115018
Madhavpeddi, L., Hammond, B., Carbone, D. L., Kang, P., Handa, R. J., and Hale, T. M. (2022). Impact of angiotensin II receptor antagonism on the sex-selective dysregulation of cardiovascular function induced by in utero dexamethasone exposure. Am. J. Physiol. Heart Circ. Physiol. 322 (4), H597–h606. doi:10.1152/ajpheart.00587.2021
Manolis, A. A., Manolis, T. A., Melita, H., and Manolis, A. S. (2020). Congenital heart block: Pace earlier (Childhood) than later (Adulthood). Trends cardiovasc. Med. 30 (5), 275–286. doi:10.1016/j.tcm.2019.06.006
Martínez-Barrios, E., Cesar, S., Cruzalegui, J., Hernandez, C., Arbelo, E., Fiol, V., et al. (2022). Clinical genetics of inherited arrhythmogenic disease in the pediatric population. Biomedicines 10 (1), 106. doi:10.3390/biomedicines10010106
Mendoza, A., Belda, S., Salguero, R., and Granados, M. A. (2010). Congenital complete atrioventricular block associated with QT prolongation: Description of a patient with an unusual outcome. Pediatr. Cardiol. 31 (6), 887–890. doi:10.1007/s00246-010-9725-6
Murray, A., Tharmalingam, S., Khurana, S., Lalonde, C., Nguyen, P., and Tai, T. C. (2022). Effect of prenatal glucocorticoid exposure on circadian rhythm gene expression in the brains of adult rat offspring. Cells 11 (10), 1613. doi:10.3390/cells11101613).
Oka, Y., Itoh, H., Ding, W. G., Shimizu, W., Makiyama, T., Ohno, S., et al. (2010). Atrioventricular block-induced Torsades de Pointes with clinical and molecular backgrounds similar to congenital long QT syndrome. Circ. J. 74 (12), 2562–2571. doi:10.1253/circj.cj-10-0498
Otsuki, S., Izumi, D., Hasegawa, Y., Yagihara, N., Iijima, K., and Minamino, T. (2020). Suppression of cardiac memory-related severe form of torsades de pointes by landiolol in a patient with congenital long QT syndrome type 2. Hear. Case Rep. 6 (7), 407–410. doi:10.1016/j.hrcr.2020.03.013
Pal, S., Sardar, S., Sarkar, N., Ghosh, M., and Chatterjee, S. (2022). Effect of antenatal dexamethasone in late preterm period on neonatal hypoglycemia: A prospective cohort study from a developing country. J. Trop. Pediatr. 68 (2), fmac021. doi:10.1093/tropej/fmac021
Qiu, J., Fan, X., Ding, H., Zhao, M., Xu, T., Lei, J., et al. (2022). Antenatal dexamethasone retarded fetal long bones growth and development by down-regulating of insulin-like growth factor 1 signaling in fetal rats. Hum. Exp. Toxicol. 41, 9603271211072870. doi:10.1177/09603271211072870
Rivet, T. R., Lalonde, C., and Tai, T. C. (2022). Gene dysregulation in the adult rat paraventricular nucleus and amygdala by prenatal exposure to dexamethasone. Life (Basel) 12 (7), 1077. doi:10.3390/life12071077
Rosso, R., Adler, A., Strasberg, B., Guevara-Valdivia, M. E., Somani, R., Baranchuk, A., et al. (2014). Long QT syndrome complicating atrioventricular block: Arrhythmogenic effects of cardiac memory. Circ. Arrhythm. Electrophysiol. 7 (6), 1129–1135. doi:10.1161/circep.114.002085
Shinwell, E. S., Gurevitz, P., and Portnov, I. (2022). Current evidence for prenatal and postnatal corticosteroids in preterm infants. Arch. Dis. Child. Fetal Neonatal Ed. 107 (2), 121–125. doi:10.1136/archdischild-2020-319706
Sun, H., Liu, X., Hao, X., Zhou, X., Wang, J., Han, J., et al. (2022). Case report: Characterization of a novel NONO intronic mutation in a fetus with X-linked syndromic mental retardation-34. Front. Genet. 13, 593688. doi:10.3389/fgene.2020.593688
Suryawanshi, H., Clancy, R., Morozov, P., Halushka, M. K., Buyon, J. P., and Tuschl, T. (2020). Cell atlas of the foetal human heart and implications for autoimmune-mediated congenital heart block. Cardiovasc. Res. 116 (8), 1446–1457. doi:10.1093/cvr/cvz257
Tang, C., Yu, H., Shao, S., Hua, Y., Chen, M., Zhu, Q., et al. (2021). Case report: Prenatal diagnosis and treatment of fetal autoimmune-associated first-degree atrioventricular block: First report from China. Front. Cardiovasc. Med. 8, 683486. doi:10.3389/fcvm.2021.683486
Wainwright, B., Bhan, R., Trad, C., Cohen, R., Saxena, A., Buyon, J., et al. (2020). Autoimmune-mediated congenital heart block. Best. Pract. Res. Clin. Obstet. Gynaecol. 64, 41–51. doi:10.1016/j.bpobgyn.2019.09.001
Wang, J., Chen, F., Zhu, S., Li, X., Shi, W., Dai, Z., et al. (2022). Adverse effects of prenatal dexamethasone exposure on fetal development. J. Reprod. Immunol. 151, 103619. doi:10.1016/j.jri.2022.103619
Wu, Z., Wen, Y., Xiao, H., Zhu, J., Li, B., Shangguan, Y., et al. (2022). 11β-Hydroxysteroid dehydrogenase 2: A key mediator of high susceptibility to osteoporosis in offspring after prenatal dexamethasone exposure. Pharmacol. Res. 175, 105990. doi:10.1016/j.phrs.2021.105990
Xie, L., Jiao, Z., Zhang, H., Wang, T., Qin, J., Zhang, S., et al. (2022). Altered hippocampal GR/KCC2 signaling mediates susceptibility to convulsion in male offspring following dexamethasone exposure during pregnancy in rats. Toxicol. Lett. 364, 12–23. doi:10.1016/j.toxlet.2022.05.004
Keywords: fetal bradycardia, long QT syndrome, kcnh2, prenatal management, dexamethasone exposure, case report
Citation: Huang H, Jing S, Wu S, Wei L, Zhang Q, Hua Y, Li Y, Yu H and Zhou K (2022) Case Report: A novel KNCH2 variant-induced fetal heart block and the advantages of fetal genomic sequencing in prenatal long-term dexamethasone exposure. Front. Genet. 13:1010078. doi: 10.3389/fgene.2022.1010078
Received: 02 August 2022; Accepted: 03 November 2022;
Published: 29 November 2022.
Edited by:
Przemysław Kosinski, Medical University of Warsaw, PolandReviewed by:
Jiancheng Han, Capital Medical University, ChinaTakeshi Aiba, National Cerebral and Cardiovascular Center, Japan
Copyright © 2022 Huang, Jing, Wu, Wei, Zhang, Hua, Li, Yu and Zhou. This is an open-access article distributed under the terms of the Creative Commons Attribution License (CC BY). The use, distribution or reproduction in other forums is permitted, provided the original author(s) and the copyright owner(s) are credited and that the original publication in this journal is cited, in accordance with accepted academic practice. No use, distribution or reproduction is permitted which does not comply with these terms.
*Correspondence: Kaiyu Zhou, kaiyuzhou313@163.com; Haiyan Yu, fanjy422@163.com; Yifei Li, liyfwcsh@scu.edu.cn
†These authors have contributed equally to this work