- 1Center for Neuroscience Research, Children’s National Health System, Washington, DC, United States
- 2Department of Life Sciences and Systems Biology, Neuroscience Institute Cavalieri Ottolenghi, University of Torino, Orbassano, Italy
- 3Department of Neurosciences “Rita Levi Montalcini”, University of Turin, Turin, Italy
- 4Department of Biological Sciences, University of Maryland, Baltimore County, Baltimore, MD, United States
- 5Research Center for Genetic Medicine, Children’s National Health System, Washington, DC, United States
The vomeronasal system (VNS) is specialized in the detection of salient chemical cues triggering social and neuroendocrine responses. Such responses are not always stereotyped, instead, they vary depending on age, sex, and reproductive state, yet the mechanisms underlying this variability are unclear. Here, by analyzing neuronal survival in the first processing nucleus of the VNS, namely the accessory olfactory bulb (AOB), through multiple bromodeoxyuridine birthdating protocols, we show that exposure of female mice to male soiled bedding material affects the integration of newborn granule interneurons mainly after puberty. This effect is induced by urine compounds produced by mature males, as bedding soiled by younger males was ineffective. The granule cell increase induced by mature male odor exposure is not prevented by pre-pubertal ovariectomy, indicating a lesser role of circulating estrogens in this plasticity. Interestingly, the intake of adult male urine-derived cues by the female vomeronasal organ increases during puberty, suggesting a direct correlation between sensory activity and AOB neuronal plasticity. Thus, as odor exposure increases the responses of newly born cells to the experienced stimuli, the addition of new GABAergic inhibitory cells to the AOB might contribute to the shaping of vomeronasal processing of male cues after puberty. Consistently, only after puberty, female mice are capable to discriminate individual male odors through the VNS.
Introduction
Postnatal development is a critical period for the maturation of brain circuits and implies extensive interactions between external and internal factors affecting both their wiring and tuning. This is particularly important for the brain systems implied in goal oriented behaviors (such as mating or foraging) as they need to adaptively match external stimuli to internal states and drives, in order to limit non-adaptive decisions that could be detrimental for survival (Nowicki et al., 2002; Ramm et al., 2008; Bouret, 2012; Saleem et al., 2014). Because mating and feeding circuits have been mainly considered hard-wired or innate (Anderson, 2016; Kohl et al., 2017), the neural mechanisms involved in this tuning remain poorly investigated. Here, we have analyzed the role of sensory activity in the peripubertal activity-dependent maturation of inhibitory interneurons in the accessory olfactory bulb (AOB), a bulbar circuit specialized in the elaboration of salient signals for reproductive and mating behaviors (Mucignat-Caretta et al., 1995; Keller et al., 2006; Hendrickson et al., 2008; Brennan, 2009; Haga et al., 2010; Flanagan et al., 2011; Ferrero et al., 2013). The onset of female puberty represents a critical period for the development of both reproductive organs and brain circuits regulating the neuroendocrine aspects of sexual behavior (Romeo, 2003). Also, puberty represents a stage for important behavioral changes (Sisk and Zehr, 2005; Blakemore et al., 2010) and a critical period for the activity dependent remodeling of cortical circuits (Piekarski et al., 2017). In mice, at puberty chemical signaling begins to be used to advertise sexual receptivity and to assess both the quality and readiness of mating partners (Moncho-Bogani et al., 2002; Ramm et al., 2008). These two aspects of sexual maturation – the internal physiology and the sensitivity to environmental cues – obviously interact, and, through the vomeronasal system (VNS), chemical stimuli can affect both the development (Vandenbergh, 1967, 1974; Colby and Vandenbergh, 1974; Flanagan et al., 2011; Oboti et al., 2014) and the adult display (Haga et al., 2010; Roberts et al., 2010; Ferrero et al., 2013; Oboti et al., 2014) of female reproductive behaviors.
A tight interaction between neuroendocrine responses and the molecular nature of specific triggering signals has long been considered the main mechanism by which both aspects of sexual maturation are matched. However, chemical signaling is flexible and subjected to changes depending on both external and internal factors (Drickamer, 1992; Lemmen and Evenden, 2009; Garratt et al., 2011; Ferrero et al., 2013; Villar et al., 2015). In fact, the same compounds might acquire different signaling values depending on behavioral and physiological needs (Mucignat-Caretta et al., 1998; Brennan, 2009; Martín-Sánchez et al., 2015; Stowers and Liberles, 2016). Therefore, the question of how the VNS determines the onset of social behavioral responses by detecting such variable signals is unclear. Clarifying this issue is further complicated by the presence of ongoing neurogenesis in both the vomeronasal epithelia (Weiler, 2005) and the AOB (Oboti et al., 2009; see for a review Oboti and Peretto, 2014). Specifically, how this plasticity relates to the onset of puberty and to more mature behavioral displays is still unclear.
In our previous works we have extensively characterized the process of adult neurogenesis in the AOB, showing that, as in the main olfactory bulb (MOB), adult neurogenesis in this region involves a process of continuous addition of GABAergic interneurons originating in the subventricular zone (SVZ; Bonfanti et al., 1997; Peretto et al., 2001; Oboti et al., 2009, 2011). In addition, we have also shown that the integration and survival of AOB immature neurons is modulated by sensory activity (Oboti et al., 2009, 2011). In particular, exposure to vomeronasal organ (VNO)-detected stimuli contained in male urine, during a critical stage of newborn cell maturation, increases neuronal survival in the AOB of female mice (Oboti et al., 2011).
Here, by incorporating bromodeoxyuridine (BrdU) in immature SVZ-derived neurons reaching the AOB, we show that granule cell (GC) survival in the female AOB begins to be particularly sensitive to opposite-sex olfactory stimuli at the onset of puberty. We further show that the number of GCs integrating in the AOB is modulated by olfactory stimuli derived from adult but not juvenile male urine. Moreover, short term post-pubertal exposure of females to adult male derived cues promotes the integration of immature GCs in the bulbar circuits implied in male odor processing. This further correlates with the onset of individual specific responses to male odors detected through the VNS, normally displayed by mature females (Moncho-Bogani et al., 2002; Ramm et al., 2008). By combining ovariectomy with male odor exposure in BrdU-treated animals, we next provide evidence supporting that the effect of male derived cues on AOB GCs does not depend on ovarian hormones. Rather, increased VNO activity levels shown by post-pubertal females in response to these stimulations suggest a major role of sensory activity in this process. Overall, our results indicate that through activity dependent regulation of AOB GC development, VNO- detected stimuli rearrange the AOB inhibitory network involved in their processing, when these cues acquire the meaning of mating signals after puberty.
Materials and Methods
Animals
All experiments were done using CD-1 mice (Charles River, Italy): females and males, pre-pubertal (postnatal day P21–P28), peri-pubertal (P35–P42), and post-pubertal (P52–P90). Animals were housed under a 12-h light-dark cycle in an environmentally controlled room. Female subjects of the same group were maintained 4–6 per cage, whereas male subjects were kept or in isolation for the duration of all experiments or in cages with other male subjects of the same group. Experimental procedures were in accordance with the European Communities Council Directive of 24 November 1986 (86/609/EEC), Recommendation 18/06/2007, Dir. 2010/63/UE, and the Italian law for care and use of experimental animals (DL116/92) and were approved by the Italian Ministry of Health and the Bioethical Committee of the University of Turin (Protocol Number DGSAF0007085-A05/04/2013). All animal care and procedures conducted at the University of Maryland, Baltimore County were in accordance with the National Institutes of Health guide for the Care and Use of Laboratory Animals (2006) and approved by the institutional Animal Care and Use Committee. All experiments were designed to minimize the number of animals used.
Assessment of Puberty Onset
CD1 female mice were weaned at P21 and checked daily for vaginal opening. After vaginal opening, vaginal smears were performed daily and analyzed under an inverted microscope to identify the specific day of first estrus.
BrdU Treatment and Bedding Exposure
To identify newly generated cells in the AOB, female mice were intraperitoneally injected with BrdU in 0.1 M Tris (pH 7.4) twice in 1 day (delay = 8 h, 100 mg/kg body weight), and sacrificed 28 days later for the evaluation of neuronal survival. For all experimental groups, bedding stimulations (consisting of daily renewed male bedding material added with male urine) were timed during the 2nd week after BrdU injection. Males were caged individually or in groups of 3–4 animals and the bedding was left untouched for at least 1 week before being transferred to female cages. Exposures were performed at different stages of peripubertal maturation: from P28 to P35 (pubertal); from P35 to P42 (post-pubertal) and from P52 to P59 (mature). Different sources of male soiled-bedding material were used for each experimental group. Females exposed to sexually mature males, were given a mixture of bedding from three different adult reproductive males not kin-related with females (MB); females exposed to coetaneous males bedding, were given a mixture of bedding from 3/4 kin-unrelated males of the same age of female subjects (cMB; P28–P35 and P35–P42 for the peripubertal exposure; P52–P59 for the adult exposure); females exposed to kin or foster males (of the same age as females) bedding, were given a mixture from 3/4 kin (kMB) or foster (foMB) (cross-foster with females at the age of P2 until P21) males, respectively. The “control” groups were treated similarly but with clean bedding.
Ovariectomy
Juvenile (P21, n = 7) CD1 female mice were deeply anesthetized with a 3:1 solution of ketamine (Ketavet; Gellini, Italy) and xylazine (Rompun; Bayer, Germany) and using aseptic procedures both ovaries were removed by two small incisions on each side in the abdominal area, one through the skin and then another through the muscle wall. Each ovary was tied off with absorbable surgical thread and removed; after that the muscle incision and skin incision were closed using sutures. Sham-operated juvenile (P21, n = 6) CD1 female mice have been subjected to same surgical manipulations without removal of the ovaries. Mice were then allowed to recover for 1 week before further treatments.
VNO Dye-Access Assay
Juvenile (3 weeks old) sexually naïve CD1 female mice were purchased from the Jackson Laboratory and grown to designed ages of either 28, 35, or 52 days old, respectively (n = 5–7 per group).
Urine Collection and Urine-Dye Mixture
Male urine was freshly collected on the same day of the experiment from the same males that were sexually experienced. To induce urination from the males, we placed two female CD1 mice into two clean separate cages without bedding for 2 min. We then removed the female mice and transferred the male mice into the scented cages. Care was taken to remove female urine or feces, if any, before introducing the males into the cages. If no urine appeared after 2 min, the procedure was repeated or the animal was held by hand and the bladder region gently pressed to induce urination. Male urine was immediately collected after urination using a pipette. Urine was pooled into an Eppendorf tube, and placed on ice before use. For the stimulus-dye VNO access assay, a 100 μl of urine was mixed with Rhodamine 6G fluorescent dye (Sigma) to yield a final concentration of urine in 1:10 dilution and 0.002% dye.
Delivery of Urine-Dye Mixture
The VNO dye access assay was performed following a previously developed method from Ogura et al. (2010). Briefly, an individual mouse was transferred and acclimated in a chamber made from clean empty, plastic, pipette tip boxes with clear lids 12.7 cm × 7.6 cm × 5.1 cm (width × depth × height) with a 1.27 cm square hole on a side wall for 10 min. Upon acclimation, a pipette tip containing 5 μl of urine-dye solution was presented to the nostrils in small drops, when the mouse protruded its nose from the small hole. The process was repeated until all 5 μl was delivered. The process usually lasted 3–10 min. For each post-developmental stage, five to seven mice were examined.
VNO Dissection and Fluorescence Image Acquisition
Immediately after the assay, mice were euthanized by CO2 inhalation followed by cervical dislocation. The head was then removed, split roughly along the midline to expose the VNO. The half head containing the VNO was placed on a premade mold and epi-fluorescence images were taken for both lateral and ventral VNO using a 4× lens and an Olympus BX 41 epi-fluorescence compound microscope equipped with a Retiga 4000R camera and Image-Qcapture Pro 7 (QImaging, British Columbia, Canada). Fluorescence dye intensity of the VNO proper and entrance duct, which is narrow, approximately 0.4 mm in length, tubular structure anterior to the VNO proper (Ogura et al., 2010), were measured using NIH ImageJ. Background intensity was measured from the septal respiratory epithelium dorsal to the VNO proper.
Tissue Preparation for Immunohistochemistry
Mice were deeply anesthetized with an intraperitoneal injection of ketamine (Ketavet; Gellini, Italy) and xylazine (Rompun; Bayer, Germany) 3:1 solution. All the animals were transcardially perfused with 0.9% saline solution followed by cold 4% paraformaldehyde (PFA) in 0.1 M phosphate buffer (PB), pH7.4. Brains were removed from skull and post-fixed for 4 h in 4% PFA at 4°C, followed by a cryopreservation step using a 30% sucrose solution in 0.1 M PB pH 7.4 at 4°C. The two hemispheres were separated and embedded in OCT (Bio-Optica), and then frozen and cryostat-sectioned. Free floating parasagittal sections (25 μm) were collected in multi-well dishes in representative series of the AOB. Sections were stored at -20°C in an antifreeze solution (30% ethylene glycol, 30% glycerol, 10% phosphate buffer: 189 mM NaH2PO4, 192.5 mM NaOH; pH 7.4) until use.
Immunohistochemistry
After rinsing in phosphate-buffered saline (PBS) to remove the antifreeze solution, sections were incubated for 24 h at 4°C in primary antibodies diluted in 0.01 M PBS, pH 7.4, 0.5% TritonX-100, and 1% normal sera, made in the same host species of the secondary antibodies. As primary antibodies we used: anti-BrdU, rat IgG monoclonal, dilution 1:5000 (ABC), AbD serotec, Bio-Rad Laboratories, code number OBT0030CX (Liu et al., 2009); anti-c-Fos, rabbit IgG polyclonal, dilution 1:10000 (IFL), Santa Cruz Biotechnologies, Santa Cruz, CA, United States, code number sc52 (Oboti et al., 2011); anti-NeuN, mouse IgG monoclonal, dilution 1:1000 (IFL), Chemicon International, Temecula, CA, United States, code number MAB377 (Oboti et al., 2009); anti-GAD67, dilution 1:1000 (IFL), mouse IgG monoclonal, Chemicon International, Temecula, CA, United States, code number MAB5406 (Oboti et al., 2009). For BrdU immunostaining, sections were pre-treated with 2 N HCl for 30 min at 37°C, for antigene retrieval, and neutralized with borate buffer, pH 8.5, for 10 min. For the avidin-biotin-peroxidase method, sections were incubated for 1 h at room temperature in biotinylated secondary antibody (anti-rat IgG; Vector Laboratories, Burlingame, CA, United States) diluted 1:250 in 0.01 M PBS, pH 7.4 following by avidin-biotin-peroxidase complex (Vector Laboratories). To reveal immunoreactivity, we used 0.015% 3,3′-diaminobenzidine and 0.0024% H2O2 in 0.05 M Tris-HCl, pH 7.6. After adhesion on gelatin-coated glass slides, sections were mounted in DPX (Merck-Millipore, VWR International PBI, Milan, Italy). For immunofluorescence double-staining, the sections were incubated in a mixture of primary antibodies and appropriate blocking sera for 24 h at 4°C, then incubated with appropriate fluorochrome-conjugated secondary antibodies (Cy3-conjugated secondary Ab, 1:800; 488-conjugated secondary Ab, 1:400; Jackson ImmunoResearch Laboratories, United States) and/or biotinylated secondary antibodies (1:250; Vector Laboratories, Burlingame, CA, United States), and finally incubated with avidin-FITC (1:400, Vector Laboratories, Burlingame, CA, United States). The sections were then coverslipped with the anti-fade mounting medium Dabco (Sigma) and analyzed with a laser scanning LAS AF Lite confocal system (Leica Microsystems).
Cell Counting and Statistical Analysis
The number of BrdU-positive nuclei in the AOB GC layer was established by counting peroxidase/DAB-stained 25 μm thick parasagittal sections in all experimental groups. Three series (n = 7 sections/animal) representing the whole AOB were used for each animal. Cells were counted in the AOB GC layer. The area of each examined section was measured with Neurolucida software (MicroBrightField, Colchester, VT, United States). Cell densities were calculated by summing cell counts made on all sections per animal and referred to the proper cellular layer volume (Σ of sampled areas × 25 μm). The percentage of double-labeled c-Fos/BrdU-positive cells, NeuN/BrdU-positive, GAD67/BrdU-positive and triple-labeled c-Fos/NeuN/BrdU-positive and c-Fos/GAD67/BrdU-positive in the AOB GC layer was established by counting labeled cells in parasagittal sections (25 μm thick) with a 40× objective with a confocal microscope using a UPI an FL 40× (N.A. 1.3) Olympus objective. One series (n = 3–4 sections/ animal) representing the whole AOB was used for each animal. In each section, all of the BrdU-positive cells were analyzed for co-expression with c-Fos, NeuN, GAD67, c-Fos/NeuN, or c-Fos/GAD67, and ratios of double-labeled or triple-labeled cells were determined. All cell counts were performed blind to the treatment. For statistical analysis, unpaired Student’s t-tests were used for simple 1:1 comparison of parametric data; paired Student’s t-test were used for sex and individual preference tests, in which same mice were measured twice, before (P20) and after (p41 or P50) puberty; one-way or two-way ANOVA were used for multiple comparisons for parametric data; chi-squared test was used for comparing the percentage of females showing lordosis.
Sex Preference Tests
The preference of female mice for sex related odors was assessed by placing at opposite sides of the homecage (female subjects were either P20 or P41), urine stimuli were derived from adult male or female subjects (pooled from different individuals, 10 μl) on filter paper. The time spent investigating these stimuli was evaluated during 10 min trials.
Learning of Familiar Male Odors
This assay evaluated the learned preference of a female mouse for male or female individual volatile scents that were previously experienced during a 5-day exposure period (familiarization), either with or without direct physical access to the odor source. This attractive response was based on an olfactory associative learning between volatile and non-volatile urinary components. During the exposure step, two urine stimuli obtained from distinct CD1 males (or females; 50 μl each) were placed on filter paper in the cage housing the female. While one of these filter papers was directly accessible to the female (leading to a conditioning learning of urine volatiles), the second one was placed in a meshed box to prevent direct physical contact with the odor source (thus preventing urine volatiles conditioning). Urine donors were adult CD1 males (or females; 4–5 months old) housed individually, with no kinship relation. Stimuli were delivered randomly two times a day each for 5 min within a total 4 h interval allowing for a 1 h resting phase between stimulations. After this learning period, a recognition step consisting of a two-choice olfactory preference test without physical contact was performed. Filter papers containing 50 μl of both directly (CS) or indirectly (US) previously experienced urine were deposited in meshed plastic boxes to prevent direct physical contact. During a 5 min trial period, stimulus investigation time was scored as the time spent in close contact with the stimulus source (distance of the snout from the box <1 cm) as well as the time spent manipulating, chewing, and biting the meshed box in an attempt to reach the stimulus source. As a critical control to rule out any pre-existing preference prior to the learning phase, we examined whether a given female showed a preference for volatiles in the tested urine sources; only females showing no preference were used for this assay.
Female Sexual Receptivity (Lordosis)
Adult and juvenile female mice (N = 11 per group; both sexually naive) were single housed for 1 week and the estrus cycle was determined in order to use only animals in estrus or proestrus prior to the test. Adult males (sexually experienced) were introduced to the female’s home cage and were recorded for 1 h during the dark cycle. The number of lordosis events (in which females show a receptive still posture or arching of the back, allowing or promoting male mounting) by the females was assessed. The number of mounting behaviors as well as the latency to mount shown by male individuals were scored. The lordosis quotient was calculated as the ratio between lordosis events and male mounts, previously described as an index of female reproductive receptivity (Keller et al., 2006; Leinders-Zufall et al., 2014).
SDS-PAGE
Mouse urine sample aliquots (2.5 μl) were mixed with 4x LDS buffer and 20x Reducing Reagent (Thermo Fisher Scientific, Waltham, MA, United States). Samples were then boiled and loaded on 4–12% SDS precast Bis-Tris NuPage gels (Thermo Fisher Scientific, Waltham, MA, United States). Gels were ran for 1 h at 170 V, fixed (50:10:40/methanol: acetic acid: H2O/ v:v:v), stained with Biosafe Coomassie (Bio-Rad, Hercules, CA, United States), and washed extensively with water. Gels were scanned using ChemiDoc MP System. Differences in protein content among samples was estimated by comparing gel band intensities using ImageJ.
Results
Male Odors Promote Granule Cell Survival in the AOB of Post-pubertal Females
Immature neurons migrating to the AOB typically reach a mature phenotype around 4 weeks after genesis (Oboti et al., 2009) and are particularly sensitive to olfactory stimulations after reaching the olfactory bulb (7 days after BrdU injection), between their 2nd and 3rd week (Oboti et al., 2011). They integrate mainly in the GC layer (Oboti et al., 2009, 2011), which is populated for the most part by GABAergic inhibitory neurons (GCs; Larriva-Sahd, 2008). Here, we further confirmed the fully mature phenotype of newborn GCs in the AOB 28 days after their genesis (91.58 ± 4.44% of BrdU-positive cells co-express NeuN, as in mature neurons; Mullen et al., 1992). In addition, we show that the vast majority of the BrdU-labeled neurons found in the AOB 4 weeks after genesis do have a GABAergic phenotype (84.51 ± 4.3% of BrdU-positive cells co-express GAD67, the GABA synthesis enzyme; Mugnaini et al., 1984; Supplementary Figure 1).
We previously found that exposure to male soiled bedding and male-derived urine compounds increases the survival of immature AOB GCs migrating from the SVZ in P50 female mice (a stage at which both brain circuits and neuroendocrine physiology can be considered mature; Finlay and Darlington, 1995), but not in pre-pubertal P20 females (Oboti et al., 2011). However, whether this effect is due to the different sensitivity of sensory circuits in the female brain or to other behavioral mechanisms (e.g., increase motivation in exploring these cues) affecting the female detection of male stimuli through the VNO, is not known.
To address this issue, we first sought to define more precisely the time at which AOB inhibitory circuits become sensitive to male bedding exposure. We analyzed the effect of 1 week male bedding stimulation on 2-weeks-old GCs (more sensitive to sensory activity; Oboti et al., 2011) birthdated by BrdU injections at different peripubertal stages: P21 and P28 (Figures 1A,B). BrdU systemic injection results in nuclear DNA labeling during cell division (Nowakowski et al., 1989) and therefore it has been used to localize newborn neurons at different times after genesis (Figure 1C). Following BrdU treatment, along with a Ca. 50% reduction in the basal level of AOB newborn neuronal survival (neurons born at P21 vs. P28), we found that exposure to adult male soiled bedding promotes the survival of newborn GCs in the AOB after P35 (Figure 1B), but not earlier (Figure 1A). This corresponds to the age of menarche, when the first estrus cycle occurs, as shown by vaginal patency assessment (opening of the external genitalia) and cytological examination (Figure 1D; Goldman et al., 2007). Around P27 almost 60% of the females showed vaginal opening, the 100% being reached only at about P30 (Figure 1D), similarly to what has been previously observed in both the CD1 (Szymanski and Keller, 2014) and C57 mouse strains (Nelson et al., 1990; Oboti et al., 2014). Signs of first estrus cycle occurrence were found only between 3 and 10 days later (50% of the females reached estrus around P35, while the rest reached estrus around P40–P42, similar to what occurring in C57 mice; Figure 1D; Nelson et al., 1990).
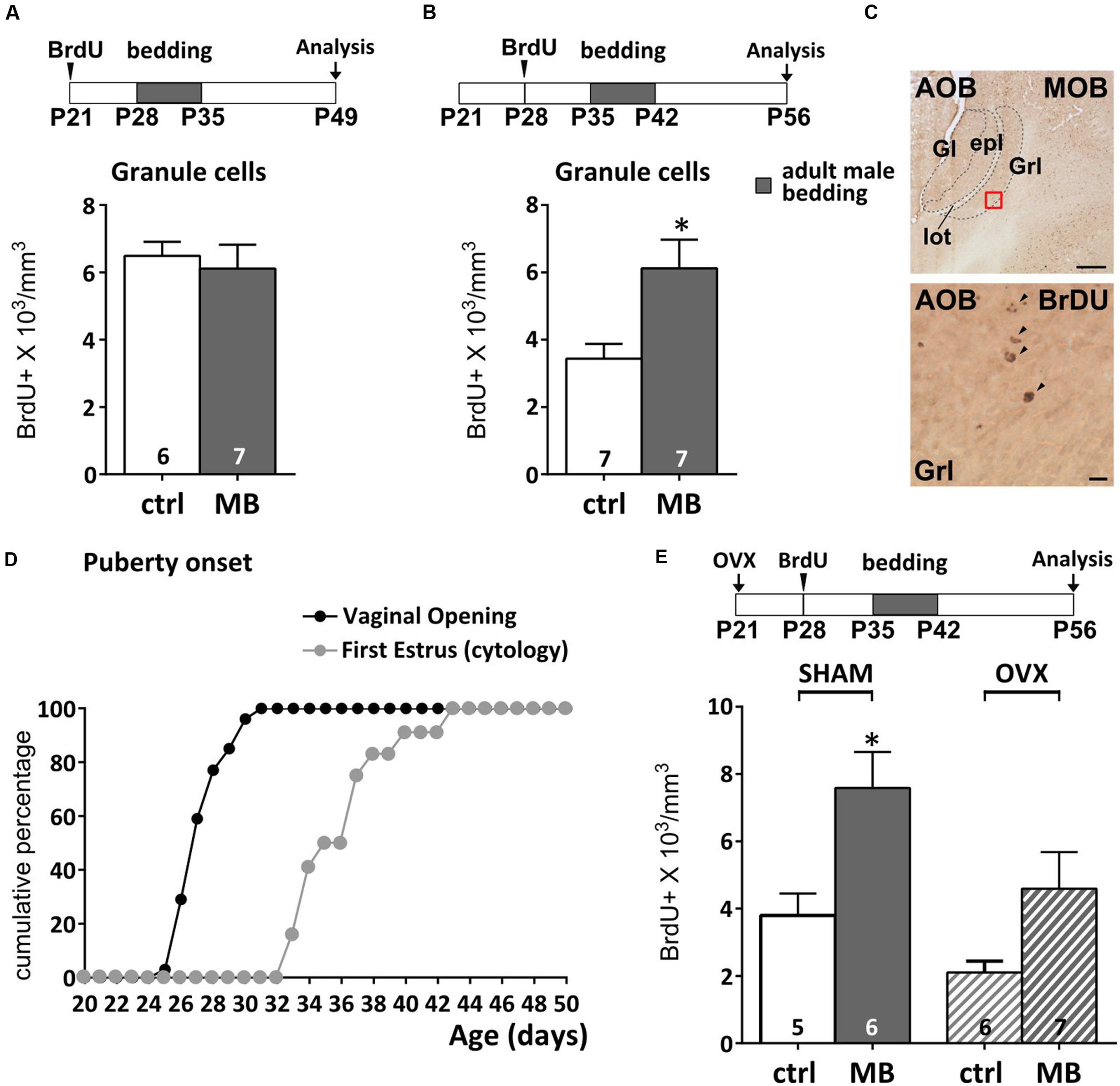
FIGURE 1. Survival of newborn granule cells (GCs) in the accessory olfactory bulb (AOB) is sensitive to vomeronasal inputs after puberty. (A,B) Daily exposure of female mice to male soiled bedding (MB) increases GC survival after the onset of the first estrus cycle (P35–P42). Bedding stimulations were timed during the 2nd week after GC genesis and their survival 28 dpi (unpaired Student’s t-test, P28–P35 ctrl vs. MB, P = 0.669, P35–P42 ctrl vs. MB, ∗P = 0.016). (C) Sagittal section of the AOB showing BrdU labeled nuclei (scale bar 100 μm). The lower panel refers to the red squared area in the GC layer (scale bar 5 μm). (D) Graph showing the onset of female puberty. This can be determined by examination of vaginal opening and the occurrence of the first estrus, assessed through cytological examination (see methods). (E) Bedding exposure in OVX pre-pubertal females (P21) does not modulate GC survival as significantly as in SHAM animals (two-way ANOVA, factors: ovariectomy, F2,10 = 6.766, P = 0.017; stimulus, F2,10 = 12.14, P = 0.002; interaction, F2,10 = 0.520, P = 0.479; Tukey’s post hoc, SHAM ctrl vs. SHAM MB, ∗P = 0.045; OVX ctrl vs. OVX MB, P = 0.206). Abbreviations: ctrl, control; MB, male bedding; MOB, main olfactory bulb; AOB, accessory olfactory bulb; Gl, glomerular layer; epl, external plexiform layer; GrL, granule cell layer; lot, lateral olfactory tract; OVX, ovariectomy; dpi, days post-injection. Sample sizes are indicated on each histogram bar. The values shown are the mean ± SEM.
Puberty onset is accompanied by a surge of luteinizing hormone, follicle stimulating hormone and estrogens (Swerdloff and Odell, 1975) which affects both neuronal proliferation (e.g., prolactin, Menezes et al., 1995; Mak et al., 2007; Larsen et al., 2008; estrogens, Tanapat et al., 1999) and pubertal maturation (Koyama et al., 2013). Therefore, since exposure to male odor stimuli also modulates the production of ovarian hormones (Bronson and Maruniak, 1976), the survival of AOB GCs could depend on indirect hormone-mediated mechanisms, rather than VNO activity per se. Although male odor exposure was delivered when GCs were not further proliferating (7 days after genesis) but began to integrate in the AOB circuits (Luskin, 1993; Lois and Alvarez-Buylla, 1994), we could not rule out hormone-mediated effects on cell division occurring during late stages of migration through the rostral migratory stream (rms; Smith et al., 2001) or cell survival itself, which indeed have been previously reported (Veyrac and Bakker, 2011; Schellino et al., 2016).
We addressed this issue by performing the same sensory stimulations (from P35 to P42) in P28 BrdU-injected animals, after removal of the ovaries at P21. At this stage female gonadal hormones are not yet at peak (Bronson and Maruniak, 1976; Bronson, 1981). Therefore, 7 days after surgery, blood hormone levels remain stable at pre-pubertal levels (Bronson and Maruniak, 1976; Bronson, 1981). A two-way ANOVA indicated a significative effect of ovariectomy (OVX) on BrdU levels (regardless of bedding treatments). However, although OVX showed on average lower levels of BrdU expression in the AOB (Figure 1E), male bedding exposure in ovariectomized mice seemingly affected GC survival as in sham-operated subjects, however, without reaching statistical significance (see Supplementary Table 1 for statistical tests). Overall, these results suggest that gonadal hormones might modulate broad aspects of SVZ-OB neurogenesis (Menezes et al., 1995; Tanapat et al., 1999; Mak et al., 2007; Larsen et al., 2008; Brock et al., 2010; Brus et al., 2016), without critically affecting activity dependent modulation of GC survival (Figure 1E).
Urine Odors from Mature, But Not Juvenile Males, Promote Granule Cell Integration
Male urine has been repeatedly shown to be efficient in promoting survival of AOB newborn cells in female mice (Oboti et al., 2009; Nunez-Parra et al., 2011; Schellino et al., 2016). In CD1 swiss mice, stimuli comprised in the low molecular weight (LMW) fraction of male urine (mass lower than 3 kDa) are more efficient in promoting neuronal integration of immature cells in the AOB of adult females (Oboti et al., 2011). This fraction contains volatile and non-volatile molecules resulting from the metabolism of hormones like testosterone (Knopf et al., 1983). Stimuli in the high molecular weight (HMW) fraction of male urine include instead proteins and large peptidic complexes, which production depends on both age and testosterone levels (Lombardi et al., 1976; Knopf et al., 1983; Chamero et al., 2007; Garratt et al., 2011). Therefore the composition of male chemostimuli varies with age, and urine protein content can be used as a proxy to assess these changes.
To clarify whether or not the maturity of male chemostimuli was differently affecting AOB GC density, we exposed female mice to bedding obtained from cages of coetaneous (cMB; i.e., same age of exposed females) but unrelated males. We found that such stimulation had no effect on BrdU-labeled GCs either at the time of vaginal opening (Figure 2A) or at the age of first estrus (1 week later; Figure 2B), when stimuli from mature males began to be effective (Figure 1B). Conversely, around P50, exposure to bedding material from coetaneous males (P50, cMB) increased the number of newborn GCs in the AOB as more mature stimuli do (Figure 2C). Thus, odor stimuli affected those cells generated right after these treatments (P45), even though females received daily bedding from the same donors (Figure 2C). Since long term exposure to the same odor stimuli has no reported effect on neuronal survival in the OB (Rochefort et al., 2002; Magavi et al., 2005), this suggests the occurrence of changes in the composition of male urine stimuli during peripubertal development. In addition, they also indicate that experience induced remodeling of AOB inhibitory circuits occurs in females at a time when donors (males) and receivers (females) of urine chemosignals are mature for mating. As a confirmation of reached sexual maturity in P50 males, we assessed the presence of urinary proteins, which production and excretion through urine are known to be testosterone dependent (Knopf et al., 1983; Chamero et al., 2007). We used gel electrophoresis to test individual urine samples collected from male CD1 mice at different ages. We found that at P50 male urine was already enriched with a high proportion of HMW compounds (15–25 kDa), while around P30 and younger ages urine protein content was lower, indicating a different composition of the stimuli (Figures 2D,E). Thus, male urine composition is likely to be a key factor affecting AOB GC survival, since female mice did not have previous experience with coetaneous donors in these experiments.
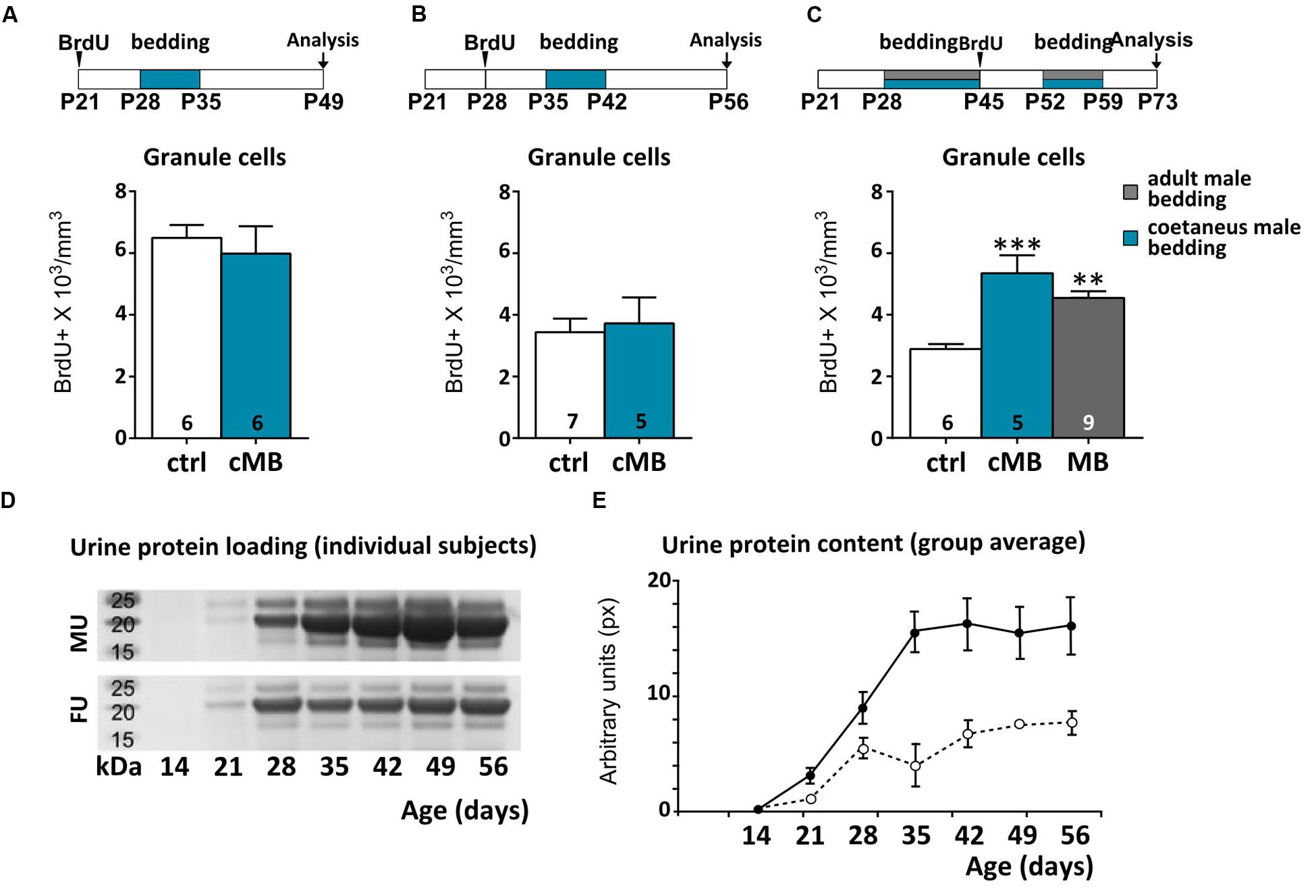
FIGURE 2. Urine stimuli affecting AOB GCs in females are produced by sexually mature males. (A–C) Comparison of the effect of coetaneous male bedding (cMB) stimulations delivered to female mice at different postnatal ages (P28, P35, P52), compared to mature male bedding (MB). Survival of AOB GCs is affected by odor exposures if the donors age is at least P52 (unpaired Student’s t-test, P28–P35 ctrl vs. cMB, P = 0.614, P35-P42 ctrl vs. cMB, P = 0.751; one-way ANOVA, F2,19 = 13.759, P = 0.000; Tukey’s post hoc, ctrl vs. cMB ∗∗∗P = 0.000; ctrl vs. MB, ∗∗P = 0.003). (D) PAGE of urine samples collected from male/female donors at different ages (P14–P56; the bands show the loading of proteins within the 15–27 kDa range, which includes MUPs). (E) Average protein content by age in the two sexes (males, solid line; females, dotted line; repeated measures two-way ANOVA, Supplementary Table 1). Abbreviations: MU, male urine; FU, female urine. Sample sizes are indicated on each histogram bar. The values shown are the mean ± SEM.
Alternatively, early postnatal odor experience (nest odors, littermates) might have biased successive AOB male odor responses simply because the wiring of sensory neurons might have needed to retune (Xia et al., 2006; Jones et al., 2008; Hovis et al., 2012) to a new set of stimuli (e.g., MUPs and MHC-related compounds change with age, Singer et al., 1997; Sherborne et al., 2007). To verify this possibility, we have long-term exposed (P0–P42) female mice to the odors of kin-related coetaneous littermates, in order to evaluate GCs survival after puberty (as above). This was done by protracting bedding exposures a few days before (P42) or after (P50) BrdU injections (P45), in order to control for possible proliferative effects. We found that post-pubertal exposure to coetaneous littermates affected GC survival, similarly to what occurred when mature unrelated male stimuli are used (Figure 3A).
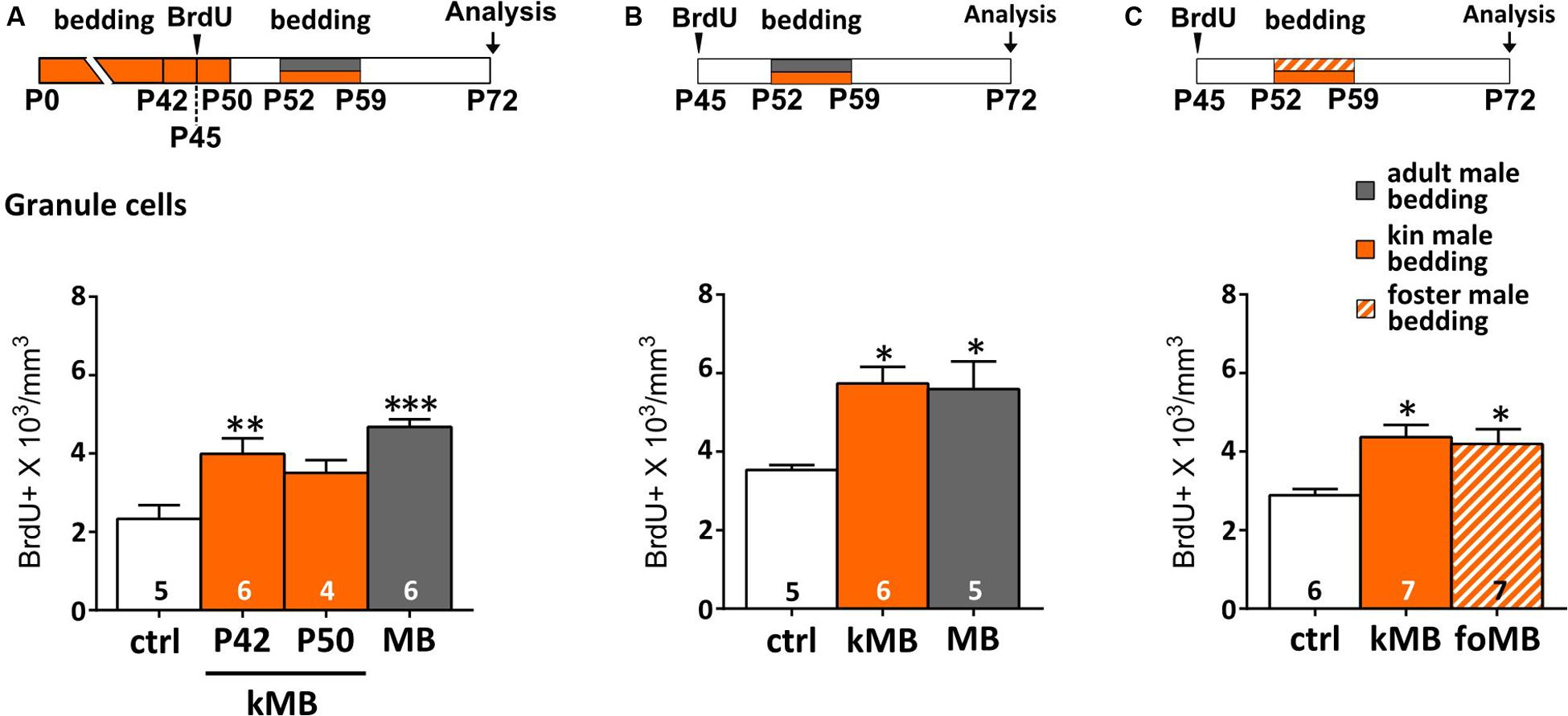
FIGURE 3. Kinship does not prevent male odors to increase AOB GCs. (A) Long-term exposure (until P42) to kin-related male stimuli (kMB) increases AOB GCs, after post-pubertal (P52–P59) exposure to the same stimuli, similarly to mature unrelated male cues (MB). By contrast, exposure to kMB cues until P50 does not increase AOB GCs density (one-way ANOVA, F3,19 = 10.47, P = 0.000; Tukey’s post hoc, ctrl vs. P42 kMB, ∗∗P = 0.008; ctrl vs. P50 kMB, P = 0.123, ctrl vs. MB, ∗∗∗P = 0.000). (B) Post-pubertal exposure to kin-related male stimuli (kMB) affects GC survival as do unrelated male odors (MB; one-way ANOVA, F2,13 = 6.508, P = 0.001; Tukey’s post hoc, ctrl vs. kMB ∗P = 0.02, ctrl vs. MB, ∗P = 0.03). (C) Post-pubertal stimulation (P52–P59) to bedding material soiled by foster littermates (foMB) is as effective as bedding soiled by kMB (one-way ANOVA, F2,17 = 6.493; P = 0.001; Tukey’s post hoc, ctrl vs. foMB ∗P = 0.024, ctrl vs. kMB, ∗P = 0.010). Sample size is indicated on each histogram bar. The values shown are the mean ± SEM.
Specifically, the amount of BrdU-labeled GCs was significantly higher (compared to controls) when stimulations were interrupted 10 days before (P42) the second exposure (thus not covering the proliferative phase of cells labeled with BrdU at P45; Figure 3A). Conversely, lower levels of BrdU-labeling were found in the AOB GC layer when bedding stimulations were interrupted only 2 days before (P50) the second exposure (during the proliferative phase of BrdU-labeled cells; Figure 3A). This is in accordance to previous studies reporting a minor effect of long-term odor exposure on GCs survival (Magavi et al., 2005; Alonso et al., 2006). Kin-related odors affected GC survival in the AOB even when females were separated before puberty (P21; Figure 3B), thus confirming that the maturation of male stimuli is likely to be the most relevant factor affecting AOB plasticity. Finally, to rule out possible effects of donor genetic identity on GC survival in the AOB, we exposed mature females to male soiled bedding material from either kin-related (kMU) or unrelated foster cage mates (foMU), housed together with females until P21. Both stimuli were effective in modulating the survival of newborn GCs (Figure 3C). Overall, these results indicate that the survival of newborn GCs in the AOB of post-pubertal female mice is similarly affected by exposure to kin-related or unrelated males when they reach sexual maturity (P50).
Differential Responses of AOB Circuits and Newborn Granule Cells to Familiar Odor Stimuli
Novel stimuli are shown to be most effective in promoting survival of newborn cells (Rochefort et al., 2002). Once odor stimulations are repeated through familiarization, newborn cells in the OB have been shown to preferentially respond to familiar odors, showing higher levels of c-Fos expression than pre-existing neurons (Magavi et al., 2005). Here, we reported similar effects occurring in the AOB (Figures 4A,B; Oboti et al., 2011). By exposing female mice to different male individual odors, either previously experienced (1 week odor familiarization) or novel, we evaluated odor-induced c-Fos expression in BrdU-positive GC neurons, when they are most responsive (i.e., around 2 weeks of age; Figure 4A; Oboti et al., 2011). Not only did 1 week of bedding exposure increase the amount of newborn GCs (BrdU-positive) in the AOB (Figure 4B), but also increased the percentage of those activated by familiar odors, as shown by a direct comparison between control group and familiarized females (c-Fos/BrdU co-expression; Figure 4C). Notably, c-Fos expression in AOB BrdU-positive cells was always associated to NeuN-positive/GAD67-positive inhibitory interneurons (Supplementary Figure 1). Interestingly, considering the entire AOB population, c-Fos activation in response to novel odors, in presence (unfamiliar) or absence (focal exposure) of previous familiarization, was always higher than the one evoked by either familiar odors or clean bedding (Figure 4D). This indicates that odor experience does not lead to a generalization in AOB responses to male cues but, instead, enhances the differences between different odor patterns, at least at the level of GCs (Figure 4E). Collectively, these results show that AOB immature GCs respond preferentially to familiar odor stimuli sensed during their integration into the local circuits, while the rest of the population shows increased responses to different novel stimuli, regardless of previous experience. Therefore, post-pubertal exposure to male odors increases the number of GCs responding to male odor cues and promote their integration in specific circuits implied in male odor responses.
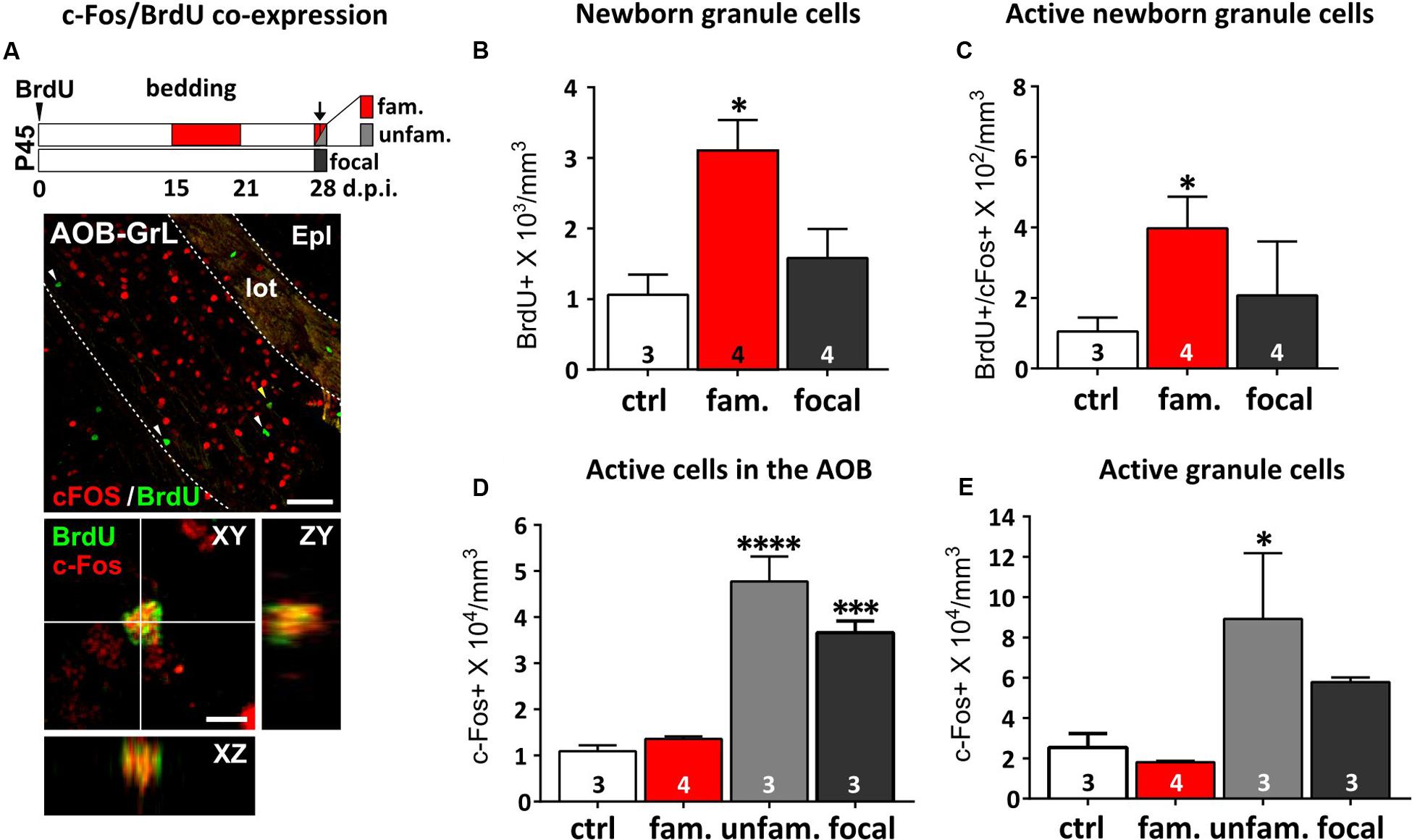
FIGURE 4. Repeated exposure (familiarization) of females to male urine stimuli increases AOB newborn cell odor responses. (A) On the top, protocol used for the analysis of AOB responsiveness to either familiar (fam; exposure to the same male bedding previously experienced from 15 to 21 dpi; red), unfamiliar (unfam; exposure to a different male bedding compared to the previously experienced; light gray) or focal odor exposures (without any previous experience; dark gray). Females were injected with BrdU at P45 and analyzed 28 dpi. On the bottom, co-expression of c-Fos (red) and BrdU (green) immunofluorescence in the AOB granule cell layer (GrL) measured 28 dpi (scale bar 50 μm). Arrowheads indicate c-Fos-negative/BrdU-positive cells. The yellow arrowhead indicates the c-Fos/BrdU double-stained cell magnified in the lower panel (scale bar 5 μm). (B) The BrdU-positive cell density measured in the AOB GC layer increases after male odor familiarization (fam), but not focal odor stimulations (focal; one-way ANOVA, F2,8 = 6.880, P = 0.018; Tukey’s post hoc, ctrl vs. fam, ∗P = 0.021). (C) Odor-evoked activity (c-Fos expression) in the BrdU-positive cells is higher after exposure to familiar stimuli (red), compared to focal stimulations and to novel odors (dark gray; unpaired Student t-test, ctrl vs. fam, ∗P = 0.046). (D) Evaluation of c-Fos expression in the entire cell population of the AOB after exposure of female mice to either familiar, unfamiliar or focal odor exposure (one-way ANOVA, F3,9 = 40.12, P = 0.0001; Tukey’s post hoc, ctrl vs. unfam ∗∗∗∗P = 0.000, ctrl vs. focal, ∗∗∗P = 0.001). (E) This effect is evident even considering the GC layer only (one-way ANOVA, F3,9 = 4.651, P = 0.0315; fam vs. unfam, Tukey’s post hoc, ∗P = 0.032). Sample sizes are indicated on each histogram bar. The values shown are the mean ± SEM.
VNO-Dependent Identity Learning of Male Stimuli Is Displayed by Females after Puberty
Male urine odors sensed through the VNO exert higher activity levels in the female AOB, compared to female odors (Dudley and Moss, 1999; Kumar et al., 1999). In the AOB, neuronal survival was clearly affected by male odor stimuli 3 puberty, when these cues changed in composition (Figures 2D,E). However, the reasons why stimuli from adult males did not induce similar effects in the AOB of younger females were not clear. Since male cues are theoretically able to activate developing vomeronasal receptor neurons (Xia et al., 2006; Hovis et al., 2012; Oboti et al., 2015) and given that pre-pubertal hormone levels might not constrain eventual activity-dependent effects on AOB GC survival (Figure 1E), we hypothesized the presence of behavioral mechanisms limiting the contact of peripubertal female mice to adult male cues.
We tested this idea by first measuring the time spent by mixed housed female mice to investigate odors from both adult males and females, before (P20) and after (P41) puberty onset. While younger females spent more time investigating female odors (paired Student’s t-test, P = 0.029), after the onset of puberty this preference is reversed, with P40 females displaying higher levels of investigation toward male cues (paired Student’s t-test, P = 0.025; Figure 5A). This implies that juvenile females might assign a different meaning to male odors, if compared to reproductively mature subjects. Therefore, it could be that male odor stimuli have an impact on neuronal survival in the AOB not only when they acquire a mature composition but also when they become meaningful mating signals. Since male odors promote female receptivity during mating (Jemiolo et al., 1991; Ramm et al., 2008; Haga et al., 2010; Roberts et al., 2010), we addressed this point by evaluating the impact of peripubertal male odor exposure on female lordosis behavior toward male intruders. Female mice were exposed to male soiled bedding from P35 to P42, prior to behavioral tests. In general, juvenile females (P43–P45) showed low receptivity toward adult male intruders (Figures 5B–D and relative statistics in Supplementary Table 1) as compared to adults, either in absence of any male stimuli, or after 1 week daily exposure to male urine, further supporting that at this age male odors do not represent mating signals promoting female receptivity. Nonetheless, given the role of AOB GCs in shaping mitral cell odor selectivity (Hendrickson et al., 2008), we hypothesized that the addition of new GCs to pre-existing AOB circuits might occur when the vomeronasal sensory neurons begins to tune their discriminative capabilities at puberty. In order to test this possibility, we quantified the female learned preference for individual male volatile odors: after repeated direct exposure to individual male chemostimuli, female mice prefer to investigate the volatile cues derived from the same male, if compared to other subjects. This selective preference is established through short-term experience of urine derived cues sensed through the vomeronasal pathway (Moncho-Bogani et al., 2002; Ramm et al., 2008; Hurst, 2009; Oboti et al., 2014). Accordingly, we found that repeated direct exposure to male individual urine compounds induced in post-pubertal females resulted in a higher interest toward the associated volatile stimuli, as compared to unexperienced odors (Figures 5E,G). Conversely, pre-pubertal exposure to male odors failed to elicit this effect in female mice (Figures 5E,G). However, exposure to mature female odors, had negligible effects in both juvenile and mature females (Figure 5F), indicating sex-specificity in this response. Overall, these results suggest that male stimuli used to establish male individual preference (or simply discrimination of experienced individual odors) may not gain efficient access to the VNO sensory epithelium until more mature stages.
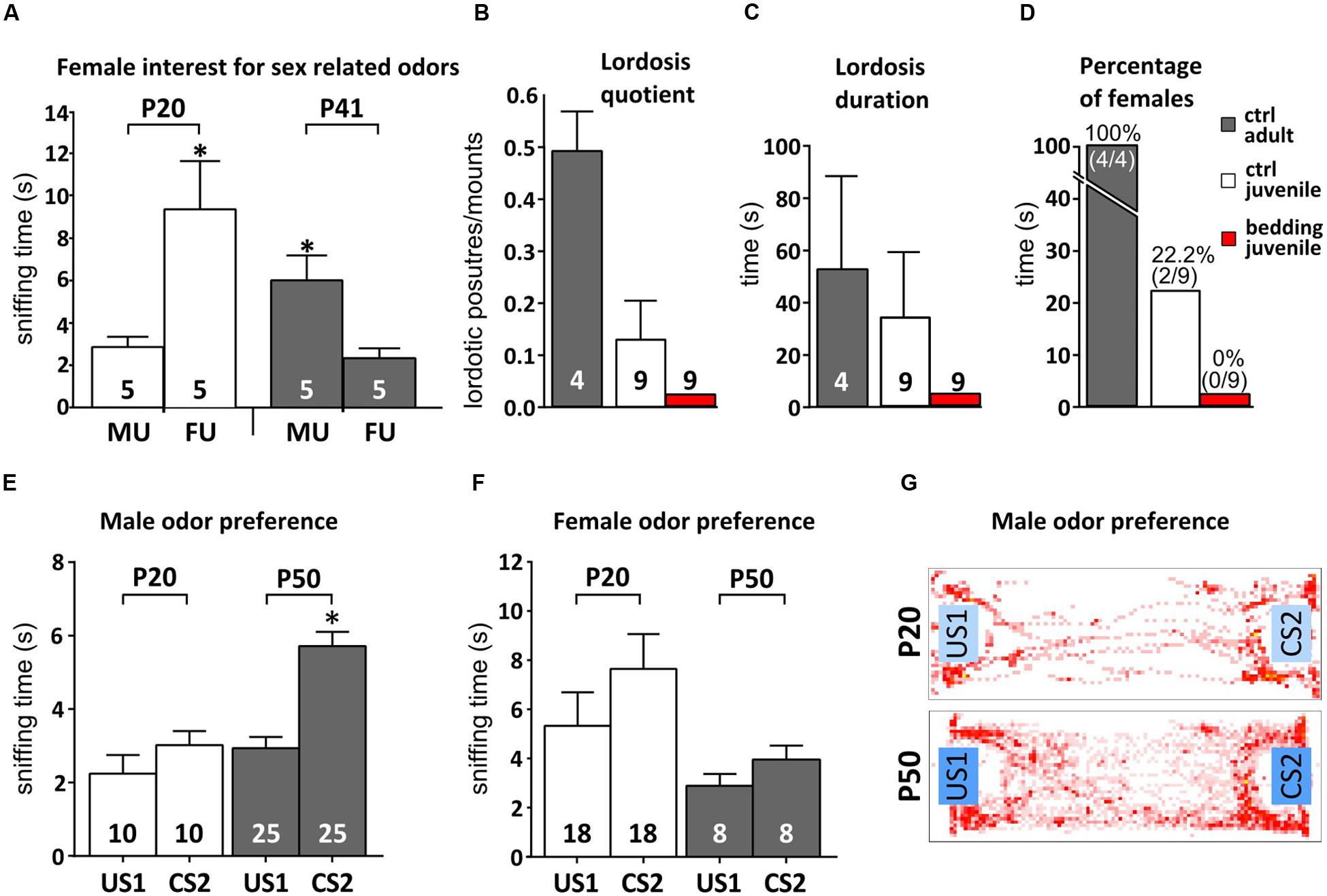
FIGURE 5. Female mice learn to prefer familiar male odors after puberty. (A) Reversal of female preference for sex odors (MU, male urine; FU, female urine) across puberty (P20 and P41; unpaired Student’s t-test, P20 MU < FU, ∗P < 0.05; P41 MU > FU, ∗P < 0.05). (B–D) Evaluation of female receptivity (lordosis quotient, duration and percentage of females displaying lordosis) after peripubertal exposure to male soiled bedding compared to unstimulated controls (ctrl; B- one-way ANOVA, F2,18 = 6.386, P = 0.008; Tukey’s post hoc, juv ctrl vs. juv bed P = 0.402, juv ctrl vs. adult ctrl, P = 0.048, juv bed vs. adult ctrl, P = 0.006; C- one-way ANOVA, F2,18 = 1.672, P = 0.217; Tukey’s post hoc, juv ctrl vs. juv bed P = 0.383, juv ctrl vs. adult ctrl, P = 0.842, juv bed vs. adult ctrl, P = 0.244; D- chi-squared contingency test, χ2(2, N = 22) = 14.16, P = 0.008). Adult levels were calculated in P90 mature female mice. (E) Male odor preference in post-pubertal females after exposure to volatiles male cues. Volatile odors become preferred after repeated direct contact to non-volatile male stimuli (conditioned stimuli, CS) in respect to never experienced volatile odors (unconditioned stimuli, US). The graph shows an increase in preference for volatile male odors (CS2) after familiarization. Female mice develop this preference only after puberty (as P20 females show equal responses to CS and US stimuli; unpaired Student t-test, P50 CS2 > US1, ∗P < 0.05). (F) Exposure to female odors in the same behavioral paradigm does not lead to preference learning. (G) Heatmaps representing the mouse presence in the cage during behavioral tests. Sample sizes are indicated on each histogram bar. The values shown are the mean ± SEM.
To clarify this point, we measured the access of male urine stimuli to the VNO of female mice at different peripubertal ages (P28, P35, and P52). To evaluate the extent up to which male cues reach the VNO epithelia in females, male urine was mixed with a fluorescent dye (rhodamine, 0.002%, Ogura et al., 2010). We found a positive correlation between rhodamine-based fluorescence and age (P28–P52), in both the VNO duct (R2 = 0.726) and lumen (R2 = 0.948), suggesting either an increased access or intake of male derived cues to the VNO sensory epithelia [no significant differences were evident using one-way ANOVA, see Supplementary Table 1; duct F(2,14) = 0.674, P = 0.526; lumen F(2,14) = 0.609, P = 0.558]. At younger stages, rhodamine fluorescence was mainly present in the duct at the entrance of the VNO while at later stages, fluorescence was clearly visible across the whole extent of the inner lumen of the VNO (Figures 6A–D). We evaluated male urine intake bilaterally and analyzed rhodamine fluorescence in both VNOs (Figure 6B). In both the VNO duct and the lumen of the sensory epithelium, there was an increased tendency of rhodamine retention across puberty (by measuring fluorescence in arbitrary units ranged between 0 and 256, the maximum values were observed at older ages P35, P52), according to a delayed patency of the duct giving access to the VNO (Coppola et al., 1993). Overall these results suggest that the detection of male urine compounds through the VNO might increase during female puberty and therefore promote the addition of newborn GCs to AOB circuits involved in individual odor processing.
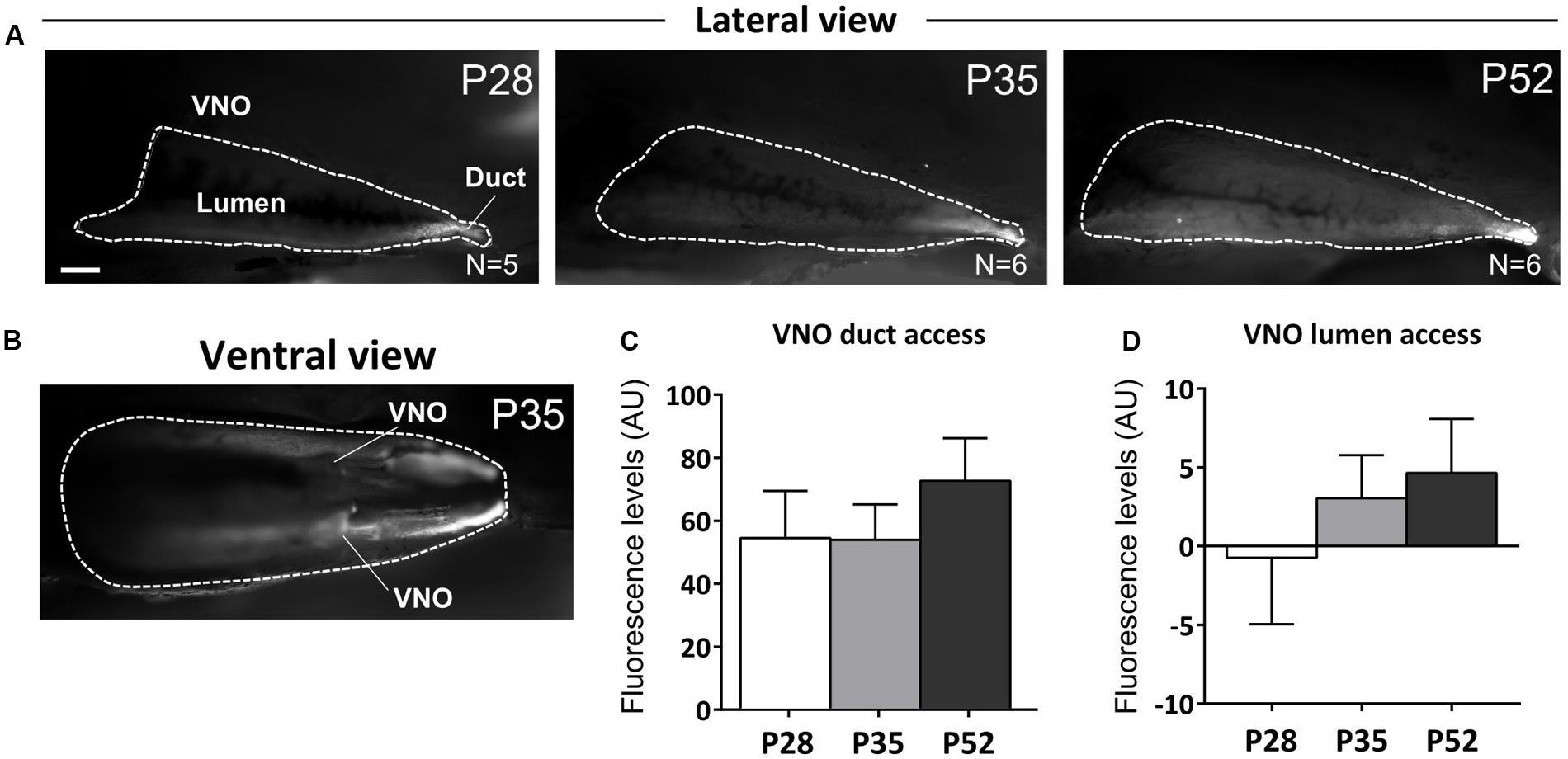
FIGURE 6. Vomeronasal organ intake of male urine derived stimuli increases after puberty. (A,B) Sagittal dissection of the nasal cavity showing rhodamine intake in the VNO upon nostril presentation of dyed-male urine to female mice at different postnatal ages (P28, P35, P52; A-lateral view, B-ventral view; scale bar 0.5 mm). Fluorescent labeling inside the VNO indicates active stimulus intake in the VNO during investigation. (C,D) Fluorescence levels (AU), measured at the level of the VNO duct (C) and internal lumen (D) indicate appropriate stimulus delivery at all three postnatal ages (P28, P35, and P52) and increased levels of stimulus intake (see text for major details). Abbreviations: AU, arbitrary units. The values shown are the mean ± SEM.
Discussion
Previously, we have shown that survival of AOB inhibitory interneurons is modulated by sensory activity only in post-pubertal female mice (Oboti et al., 2009, 2011). Here, we aimed to explore the physiological and behavioral parameters underlying the delayed sensitivity of GC survival to male urine odors. First, we show that male bedding exposure affects GCs at the onset of the first estrus cycle. Male stimuli produced by sexually mature males are more effective than stimuli from younger males. Moreover, the genetic identity of the donors does not affect GC survival, as kin-related and unrelated stimuli induced similar effects. The pubertal onset of GC sensitivity to male odor cues is unlikely mediated by hormonal changes occurring in females at this stage, although gonadal hormones might affect neuronal survival indirectly, by regulating VNO pumping activity and sensory detection (Dey et al., 2015), or by modulating either cell proliferation or migration in the SVZ as previously reported (Menezes et al., 1995; Tanapat et al., 1999; Mak et al., 2007; Larsen et al., 2008). Conversely, this timing correlated with a higher intake of male signals by the female VNO, observed from the onset of puberty onward, therefore directly linking the maturation of AOB inhibitory circuits to olfactory sensory activity. Consistently, after short-term exposure to male odors, newborn GCs showed higher odor specific responses. As a consequence of this experience, male cues become preferred over unfamiliar or novel stimuli (Ramm et al., 2008). Overall, our results indicate that the first brain relay of the VNS, the AOB, is capable of rearranging its circuits by increasing the survival of newborn GCs in response to environmental stimuli once they become meaningful social signals during peripubertal development.
Puberty represents a phase in which the meaning of social signals is subjected to changes, due to the increased complexity of the behavioral contexts in which such signals are used (mating, territoriality, parental care). Because both the identity and the relative composition of social signals might be subject to contingent variation (Sappington and Taylor, 1990; Clark et al., 1997; Moore, 1997; Mucignat-Caretta et al., 1998; Garratt et al., 2011), a reliable way to communicate information about social and reproductive status should imply a certain degree of adaptation in order to optimize the correspondence between chemical signals and behavioral response (Lemmen and Evenden, 2009; Villar et al., 2015). Neuronal turnover in other OB inhibitory networks has long been proposed to meet this demand by optimizing mitral cell odor representations (Alonso et al., 2006; Lepousez et al., 2014). Consistently, odor-evoked activity affects both the maturation and the integration of immature interneurons depending on the extent and value of odor experience (Rochefort et al., 2002; Magavi et al., 2005; Lledo et al., 2006; Schellino et al., 2016). Nonetheless, in most of the functional studies focused on the VNS, this plasticity has been often disregarded (but see for example Imayoshi et al., 2008; Sakamoto et al., 2011, 2014). The main reason for this is the misconception that animal social behaviors must be somehow constrained by the presence of highly conserved molecular signals and highly specialized olfactory receptors to which they bind. In this scenario, increasing behavioral complexity necessarily requires a concurrent increase in the number of signals used to match behavioral needs to behavioral responses (for example adult sexual interactions are promoted by the peptide ESP1 and adult-juvenile interactions inhibited by ESP22; Haga et al., 2010; Ferrero et al., 2013). However, the presence of redundancy in both signal production and detection (Marino et al., 2000) provides evidence that single molecules alone cannot be reliable signals in social communication.
For instance, different molecules are used as puberty accelerating signals (DHT, DHB, MUPs: Novotny et al., 1999a; Mucignat-Caretta et al., 1995), signals for triggering aggression (MUPs, DHT, DHB: Novotny et al., 1985; Chamero et al., 2007) or signals for promoting female attraction (Darcin, ESP1, MUPs: Kimoto et al., 2007; Haga et al., 2010; Roberts et al., 2010; Oboti et al., 2014). Therefore, in the case of MUPs for example, the signaling properties depend probably more on the receiver’s behavioral state, rather than on the specific ligand/receptor interactions.
Given the high affinity of VNO receptors to their ligands, it would be both evolutionarily and metabolically expensive if this olfactory system were to maintain the potential to detect all potential ligands for its receptors. Our results show that the activation of AOB granules depends on the identity of experienced stimuli. Importantly, the inhibitory feedback they provide to AOB mitral cells is responsible for the highly selective responses shown by these output neurons to individual odors (Luo et al., 2003; Hendrickson et al., 2008; Ben-Shaul et al., 2010). Therefore, the activity dependent plasticity of AOB GCs potentially represents a mechanism by which such odor specific responses are established through experience. Eventually, this further implies that social behavioral displays triggered by the VNS might involve a certain degree of adaptation to signal availability occurring through experience. However, future experiments are necessary to define both the contexts and the constraints of such plasticity.
Importantly, we do not want to argue that the VNO is not functional before puberty, as several reports indicated that pre-pubertal animals are indeed sensitive to stimuli detected through vomeronasal sensory neurons (Lomas and Keverne, 1982; Nishimura et al., 1989; Price and Vandenbergh, 1992; Mucignat-Caretta et al., 1995; Novotny et al., 1999a,b; Mucignat et al., 2004; Hovis et al., 2012). Despite juvenile (pre-pubertal) female mice are probably able to sense male odorants through the VNO, they are likely to be less motivated to investigate them since male odors are repulsive for pre-pubertal females (Mucignat-Caretta et al., 1998). However, although these stimuli might affect the advancement of female puberty onset (Lomas and Keverne, 1982; Price and Vandenbergh, 1992; Novotny et al., 1999a,b; Jouhanneau et al., 2014), at this stage they might still be ineffective in promoting sexual receptivity, as shown by our behavioral assays. This is probably because, both behaviorally and physiologically, female mice might be still immature at this stage. In addition, it has been shown that the patency of the vomeronasal duct matures progressively during postnatal development and around puberty (Coppola et al., 1993), suggesting the occurrence of changes in the general physiology of the VNS as well as in the female reproductive physiology, in a phase in which male chemical cues acquire the meaning of sexual signals utilized in the context of mating and reproductive behaviors. All these factors might be equally relevant in explaining the post-pubertal sensitivity of AOB GC survival to sensory activity.
Overall, our results provide evidence in support of the pubertal activity-dependent tuning in sensory circuits conveying salient signals for mating and social behaviors, as proposed for higher order brain circuits as well (see for a review Piekarski et al., 2017). Moreover, they corroborate the idea that the functional maturation of the neural systems implied in goal-oriented behaviors might occur not only through modulatory mechanisms activated by hormones and neurotransmitters (see for example Anderson, 2016), but also through activity-dependent changes in the wiring of the underlying circuits (as shown also by Jones et al., 2008; Hovis et al., 2012).
Author Contributions
LO, ST, and PP designed and planned the experiments. ST, LO, and RS performed the experiments. LO and ST analyzed the data. NH, OA, and WL designed and performed the VNO-dye assay and analyzed the data. ST and MM performed ovariectomies. MS performed the gel electrophoresis experiment. ST prepared the figures of the paper. LO wrote the manuscript.
Funding
This work was supported by Progetti di Ricerca di Interesse Nazionale (PRIN)-Peretto 2010-2011, Ricerca Locale UNITO 2014-2015 (PP) and NIH/NIDCD DC012831 (WL).
Conflict of Interest Statement
The authors declare that the research was conducted in the absence of any commercial or financial relationships that could be construed as a potential conflict of interest.
Acknowledgment
We would like to thank Aminah Sheikh, for proof-reading a revised copy of the manuscript.
Supplementary Material
The Supplementary Material for this article can be found online at: http://journal.frontiersin.org/article/10.3389/fnana.2017.00044/full#supplementary-material
References
Alonso, M., Viollet, C., Gabellec, M. M., Meas-Yedid, V., Olivo-Marin, J. C., and Lledo, P. M. (2006). Olfactory discrimination learning increases the survival of adult-born neurons in the olfactory bulb. J. Neurosci. 26, 10508–10513. doi: 10.1523/JNEUROSCI.2633-06.2006
Anderson, D. J. (2016). Circuit modules linking internal states and social behaviour in flies and mice. Nat. Rev. Neurosci. 17, 692–704. doi: 10.1038/nrn.2016.125
Ben-Shaul, Y., Katz, L. C., Mooney, R., and Dulac, C. (2010). In vivo vomeronasal stimulation reveals sensory encoding of conspecific and allospecific cues by the mouse accessory olfactory bulb. Proc. Natl. Acad. Sci. U.S.A. 107, 5172–5177. doi: 10.1073/pnas.0915147107
Blakemore, S. J., Burnett, S., and Dahl, R. E. (2010). The role of puberty in the developing adolescent brain. Hum. Brain Mapp. 31, 926–933. doi: 10.1002/hbm.21052
Bonfanti, L., Peretto, P., Merighi, A., and Fasolo, A. (1997). Newly-generated cells from the rostral migratory stream in the accessory olfactory bulb of the adult rat. Neuroscience 81, 489–502. doi: 10.1016/S0306-4522(97)00090-0
Bouret, S. G. (2012). Nutritional programming of hypothalamic development: critical periods and windows of opportunity. Int. J. Obes 2, S19–S24. doi: 10.1038/ijosup.2012.17
Brennan, P. A. (2009). Outstanding issues surrounding vomeronasal mechanisms of pregnancy block and individual recognition in mice. Behav. Brain Res. 200, 287–294. doi: 10.1016/j.bbr.2008.10.045
Brock, O., Keller, M., Veyrac, A., Douhard, Q., and Bakker, A. J. (2010). Short term treatment with estradiol decreases the rate of newly generated cells in the subventricular zone and main olfactory bulb of adult female mice. Neuroscience 166, 368–376. doi: 10.1016/j.neuroscience.2009.12.050
Bronson, F. H. (1981). The regulation of luteinizing hormone secretion by estrogen: relationships among negative feedback, surge potential, and male stimulation in juvenile, peripubertal, and adult female mice. Endocrinology 108, 506–516. doi: 10.1210/endo-108-2-506
Bronson, F. H., and Maruniak, J. A. (1976). Differential effects of male stimuli on follicle-stimulating hormone, luteinizing hormone, and prolactin secretion in prepubertal female mice. Endocrinol. 98, 1101–1108. doi: 10.1210/endo-98-5-1101
Brus, M., Trouillet, A. C., Hellier, V., and Bakker, J. (2016). Estradiol-induced neurogenesis in the female accessory olfactory bulb is required for the learning of the male odor. J. Neurochem. 1238, 457–468. doi: 10.1111/jnc.13677
Chamero, P., Marton, T. F., Logan, D. W., Flanagan, K., Cruz, J. R., Saghatelian, A., et al. (2007). Identification of protein pheromones that promote aggressive behaviour. Nature 450, 899–902. doi: 10.1038/nature05997
Clark, D. C., DeBano, S. J., and Moore, A. J. (1997). The influence of environmental quality on sexual selection in Nauphoeta cinerea (Dictyoptera: Blaberidae). Behav. Ecol. 8, 46–53. doi: 10.1093/beheco/8.1.46
Colby, D. R., and Vandenbergh, J. G. (1974). Regulatory effects on puberty of urinary pheromones in the mouse. Biol. Reprod. 11, 268–279. doi: 10.1095/biolreprod11.3.268
Coppola, D. M., Budde, J., and Millar, L. (1993). The vomeronasal duct has a protracted postnatal development in the mouse. J. Morphol. 218, 59–64. doi: 10.1002/jmor.1052180105
Dey, S., Chamero, P., Pru, J. K., Chien, M. S., Ibarra-Soria, X., Spencer, K. R., et al. (2015). Cyclic regulation of sensory perception by a female hormone alters behavior. Cell 161, 1334–1344. doi: 10.1016/j.cell.2015.04.052
Drickamer, L. C. (1992). Pre- and postweaning excretion of puberty-influencing chemosignals in house mice. Dev. Psychobiol. 25, 1–16. doi: 10.1002/dev.420250102
Dudley, C. A., and Moss, R. L. (1999). Activation of an anatomically distinct subpopulation of accessory olfactory bulb neurons by chemosensory stimulation. Neuroscience 91, 1549–1556. doi: 10.1016/S0306-4522(98)00711-8
Ferrero, D. M., Moeller, L. M., Osakada, T., Horio, N., Li, Q., Roy, D. S., et al. (2013). A juvenile mouse pheromone inhibits sexual behaviour through the vomeronasal system. Nature 502, 368–371. doi: 10.1038/nature12579
Finlay, B. L., and Darlington, R. B. (1995). Linked regularities in the development and evolution of mammalian brains. Science 268, 1578–1584. doi: 10.1126/science.7777856
Flanagan, K. A., Webb, W., and Stowers, L. (2011). Analysis of male pheromones that accelerate female reproductive organ development. PLoS ONE 6:e16660. doi: 10.1371/journal.pone.0016660
Garratt, M., Stockley, P., Armstrong, S. D., Beynon, R. J., and Hurst, J. L. (2011). The scent of senescence: sexual signalling and female preference in house mice. J. Evol. Biol. 24, 2398–2409. doi: 10.1111/j.1420-9101.2011.02367.x
Goldman, J. M., Murr,ÃA. S., and Cooper, R. L. (2007). The rodent estrous cycle: characterization of vaginal cytology and its utility in toxicological studies. Birth Defects Res. B Dev. Reprod. Toxicol. 80, 84–97. doi: 10.1002/bdrb.20106
Haga, S., Hattori, T., Sato, T., Sato, K., Matsuda, S., Kobayakawa, R., et al. (2010). The male mouse pheromone ESP1 enhances female sexual receptive behaviour through a specific vomeronasal receptor. Nature 466, 118–122. doi: 10.1038/nature09142
Hendrickson, R. C., Krauthamer, S., Essenberg, J. M., and Holy, T. E. (2008). Inhibition shapes sex selectivity in the mouse accessory olfactory bulb. J. Neurosci. 28, 12523–12534. doi: 10.1523/jneurosci.2715-08.2008
Hovis, K. R., Ramnath, R., Dahlen, J. E., Romanova, A. L., LaRocca, G., Bier, M. E., et al. (2012). Activity regulates functional connectivity from the vomeronasal organ to the accessory olfactory bulb. J. Neurosci. 32, 7907–7916. doi: 10.1523/JNEUROSCI.2399-11.2012
Hurst, J. L. (2009). Female recognition and assessment of males through scent. Behav. Brain. Res. 200, 295–303. doi: 10.1016/j.bbr.2008.12.020
Imayoshi, I., Sakamoto, M., Ohtsuka, T., Takao, K., Miyakawa, T., Yamaguchi, M., et al. (2008). Roles of continuous neurogenesis in the structural and functional integrity of the adult forebrain. Nat. Neurosci. 11, 1153–1161. doi: 10.1038/nn.2185
Jemiolo, B., Xie, T. M., and Novotny, M. (1991). Socio-sexual olfactory preference in female mice: attractiveness of synthetic chemosignals. Physiol. Behav. 50, 1119–1122. doi: 10.1016/0031-9384(91)90570-E
Jones, S. V., Choi, D. C., Davis, M., and Ressler, K. J. (2008). Learning-dependent structural plasticity in the adult olfactory pathway. J. Neurosci 28, 13106–13111. doi: 10.1523/JNEUROSCI.4465
Jouhanneau, M., Cornilleau, F., and Keller, M. (2014). Peripubertal exposure to male odors influences female puberty and adult expression of male-directed odor preference in mice. Horm. Behav. 65, 128–133. doi: 10.1016/j.yhbeh.2013.12.006
Keller, M., Pierman, S., Douhard, Q., Baum, M. J., and Bakker, J. (2006). The vomeronasal organ is required for the expression of lordosis behaviour, but not sex discrimination in female mice. Eur. J. Neurosci. 23, 521–530. doi: 10.1111/j.1460-9568.2005.04589.x
Kimoto, H., Sato, K., Nodari, F., Haga, S., Holy, T. E., and Touhara, K. (2007). Sex- and strain-specific expression and vomeronasal activity of mouse ESP family peptides. Curr. Biol. 17, 1879–1884. doi: 10.1016/j.cub.2007.09.042
Knopf, J. L., Gallagher, J. F., and Held, W. A. (1983). Differential, multihormonal regulation of the mouse major urinary protein gene family in the liver. Mol. Cell. Biol. 3, 2232–2240. doi: 10.1128/MCB.3.12.2232
Kohl, J., Autry, A. E., and Dulac, C. (2017). The neurobiology of parenting: a neural circuit perspective. Bioessays 39, 1–11. doi: 10.1002/bies.201600159
Koyama, S., Soini, H. A., Foley, J., Novotny, M. V., and Lai, C. (2013). Stimulation of cell proliferation in the subventricular zone by synthetic murine pheromones. Front. Behav. Neurosci. 7:101. doi: 10.3389/fnbeh.2013.00101
Kumar, S., Talis, A. L., and Howley, P. M. (1999). Identification of HHR23A as a substrate for E6-associated protein-mediated ubiquitination. J. Biol. Chem. 274, 18785–18792. doi: 10.1074/jbc.274.26.18785
Larriva-Sahd, J. (2008). The accessory olfactory bulb in the adult rats: a cytological study of its cell types, neuropil, neuronal modules, and interactions with the main olfactory system. J. Comp. Neurol. 510, 309–350. doi: 10.1002/cne.21790
Larsen, C. M., Kokay, I. C., and Grattan, D. R. (2008). Male pheromones initiate prolactin-induced neurogenesis and advance maternal behavior in female mice. Horm. Behav. 53, 509–517. doi: 10.1016/j.yhbeh.2007.11.020
Leinders-Zufall, T., Ishii, T., Chamero, P., Hendrix, P., Oboti, L., Schmid, A., et al. (2014). A family of nonclassical class I MHC genes contributes to ultrasensitive chemodetection by mouse vomeronasal sensory neurons. J. Neurosci. 34, 5121–5133. doi: 10.1523/jneurosci.0186-14.2014
Lemmen, J., and Evenden, M. (2009). Peripheral and behavioral plasticity of pheromone response and its hormonal control in a long-lived moth. J. Exp. Biol. 21, 2006–2006. doi: 10.1242/jeb.030858
Lepousez, G., Nissant, A., Bryant, A. K., Gheusi, G., Greer, C. A., and Lledo, P. M. (2014). Olfactory learning promotes input-specific synaptic plasticity in adult-born neurons. Proc. Natl. Acad. Sci. U.S.A. 111, 13984–13989. doi: 10.1073/pnas.1404991111
Liu, X. S., Chopp, M., Zhang, R. L., Hozeska-Solgot, A., Gregg, S. C., Buller, B., et al.. (2009). Angiopoietin 2 mediates the differentiation and migration of neural progenitor cells in the subventricular zone after stroke. J. Biol. Chem. 284, 22680–22689. doi: 10.1074/jbc.M109.006551
Lledo, P.-M., Alonso, M., and Grubb, M. S. (2006). Adult neurogenesis and functional plasticity in neuronal circuits. Nat. Rev. Neurosci. 7, 179–193. doi: 10.1038/nrn1867
Lois, C., and Alvarez-Buylla, A. (1994). Long-distance neuronal migration in the adult mammalian brain. Science 264, 1145–1148. doi: 10.1126/science.8178174
Lomas, D. E., and Keverne, E. B. (1982). Role of the vomeronasal organ and prolactin in the acceleration of puberty in female mice. J. Reprod. Fertil. 66, 101–107. doi: 10.1530/jrf.0.0660101
Lombardi, J. R., Vandenbergh, J. G., and Whitsett, J. M. (1976). Androgen control of the sexual maturation pheromone in house mouse urine. Biol. Reprod. 15, 179–186. doi: 10.1095/biolreprod15.2.179
Luo, M., Fee, M. S., and Katz, L. C. (2003). Encoding pheromonal signals in the accessory olfactory bulb of behaving mice. Science 299, 1196–1201. doi: 10.1126/science.1082133
Luskin, M. B. (1993). Restricted proliferation and migration of postnatally generated neurons derived from the forebrain subventricular zone. Neuron 11, 173–189. doi: 10.1016/0896-6273(93)90281-U
Magavi, S. S. P., Mitchell, B. D., Szentirmai, O., Carter, B. S., and Macklis, J. D. (2005). Adult-born and preexisting olfactory granule neurons undergo distinct experience-dependent modifications of their olfactory responses in vivo. J. Neurosci. 25, 10729–10739. doi: 10.1523/JNEUROSCI.2250
Mak, G. K., Enwere, E. K., Gregg, C., Pakarainen, T., Poutanen, M., Huhtaniemi, I., et al. (2007). Male pheromone-stimulated neurogenesis in the adult female brain: possible role in mating behavior. Nat. Neurosci. 10, 1003–1011. doi: 10.1038/nn1928
Marino, I. P., Rosa, E., and Grebogi, C. (2000). Exploiting the natural redundancy of chaotic signals in communication systems. Phys. Rev. Lett. 85, 2629–2632. doi: 10.1103/physrevlett.85.2629
Martín-Sánchez, A., McLean, L., Beynon, R. J., Hurst, J. L., Ayala, G., Lanuza, E., et al. (2015). From sexual attraction to maternal aggression: when pheromones change their behavioural significance. Horm. Behav. 68, 65–76. doi: 10.1016/j.yhbeh
Menezes, J. R., Smith, C. M., Nelson, K. C., and Luskin, M. B. (1995). The division of neuronal progenitor cells during migration in the neonatal mammalian forebrain. Mol. Cell. Neurosci. 6, 496–508. doi: 10.1006/mcne.1995.0002
Moncho-Bogani, J., Lanuza, E., Hernandez, A., Novejarque, A., and Martinez-Garcia, F. (2002). Attractive properties of sexual pheromones in mice: innate or learned? Physiol. Behav. 77, 167–176. doi: 10.1016/s0031-9384(02)00842-9
Moore, A. J. (1997). The evolution of social signals: morphological, functional, and genetic integration of the sex pheromone in Nauphoeta cinerea. Evolution 51, 1920–1928. doi: 10.2307/2411013
Mucignat, C., Benati, D., Righetti, C., and Zancanaro, C. (2004). High-resolution magnetic resonance spectroscopy of the mouse vomeronasal organ. Chem. Senses 29, 693–696. doi: 10.1093/chemse/bjh073
Mucignat-Caretta, C., Caretta, A., and Baldini, E. (1998). Protein bound male urinary pheromones: differential responses according to age and gender. J. Physiol. 486, 517–522. doi: 10.1113/jphysiol.1995.sp020830
Mucignat-Caretta, C., Caretta, A., and Cavaggioni, A. (1995). Acceleration of puberty onset in female mice by male urinary proteins. Chem. Senses 23, 67–70. doi: 10.1113/jphysiol.1995.sp020830
Mugnaini, E., Oertel, W. H., and Wouterlood, F. F. (1984). Immunocytochemical localization of GABA neurons and dopamine neurons in the rat main and accessory olfactory bulbs. Neurosci. Lett. 47, 221–226. doi: 10.1016/0304-3940(84)90517-2
Mullen, R. J., Buck, C. R., and Smith, A. M. (1992). NeuN, a neuronal specific nuclear protein in vertebrates. Development 116, 201–211.
Nelson, R. J., Badura, L. L., and Goldman, B. D. (1990). Mechanisms of seasonal cycles of behavior. Annu. Rev. Psychol. 41, 81–108. doi: 10.1146/annurev.ps.41.020190.000501
Nishimura, K., Utsumi, K., Yuhara, M., Fujitani, Y., and Iritani, A. (1989). Identification of puberty-accelerating pheromones in male mouse urine. J. Exp. Zool. 251, 300–305. doi: 10.1002/jez.1402510306
Novotny, M., Harvey, S., Jemiolo, B., and Alberts, J. (1985). Synthetic pheromones that promote inter-male aggression in mice (chemical signals/behavior/olfactory message). Proc. Natl. Acad. Sci. U.S.A. 82, 2059–2061. doi: 10.1073/pnas.82.7.2059
Novotny, M. V., Jemiolo, B., Wiesler, D., Ma, W., Harvey, S., Xu, F., et al. (1999a). A unique urinary constituent, 6-hydroxy-6-methyl-3heptanone, is a pheromone that accelerates puberty in female mice. Chem. Biol. 6, 377–383. doi: 10.1016/S1074-5521(99)80049-0
Novotny, M. V., Ma, W., Wiesler, D., and Zidek, L. (1999b). Positive identification of the puberty-accelerating pheromone of the house mouse: the volatile ligands associating with the major urinary protein. Proc. Biol. Sci. 266, 2017–2022. doi: 10.1098/rspb.1999.0880
Nowakowski, R. S., Lewin, S. B., and Miller, M. W. (1989). Bromodeoxyuridine immunohistochemical determination of the lengths of the cell cycle and the DNA-synthetic phase for an anatomically defined population. J. Neurocytol. 18, 311–318. doi: 10.1007/BF01190834
Nowicki, S., Searcy, W. A., and Peters, S. (2002). Quality of song learning affects female response to male bird song. Proc. Biol. Sci. 269, 1949–1954. doi: 10.1098/rspb.2002.2124
Nunez-Parra, A., Pugh, V., and Araneda, R. C. (2011). Regulation of adult neurogenesis by behavior and age in the accessory olfactory bulb. Mol. Cell. Neurosci. 47, 274–285. doi: 10.1016/j.mcn.2011.05.003
Oboti, L., Ibarra-Soria, X., Pérez-Gómez, A., Schmid, A., Pyrski, M., Paschek, N., et al. (2015). Pregnancy and estrogen enhance neural progenitor-cell proliferation in the vomeronasal sensory epithelium. BMC Biol. 13:104. doi: 10.1186/s12915-015-0211-8
Oboti, L., and Peretto, P. (2014). How neurogenesis finds its place in a hardwired sensory system. Front. Neurosci. 8:102. doi: 10.3389/fnins.2014.00102
Oboti, L., Pérez-Gómez, A., Keller, M., Jacobi, E., Birnbaumer, L., Leinders-Zufall, T., et al. (2014). A wide range of pheromone-stimulated sexual and reproductive behaviors in female mice depend on G protein Gαo. BMC Biol. 12:31. doi: 10.1186/1741-7007-12-31
Oboti, L., Savalli, G., Giachino, C., De Marchis, S., Panzica, G. C., Fasolo, A., et al. (2009). Integration and sensory experience-dependent survival of newly-generated neurons in the accessory olfactory bulb of female mice. Eur. J. Neurosci. 29sa, 679–692. doi: 10.1111/j.1460-9568.2009.06614.x
Oboti, L., Schellino, R., Giachino, C., Chamero, P., Pyrski, M., Leinders-Zufall, T., et al. (2011). Newborn interneurons in the accessory olfactory bulb promote mate recognition in female mice. Front. Neurosci. 5:113. doi: 10.3389/fnins.2011.00113
Ogura, T., Krosnowski, K., Zhang, L., Bekkerman, M., and Lin, W. (2010). Chemoreception regulates chemical access to mouse vomeronasal organ: role of solitary chemosensory cells. PLoS ONE 5:e11924. doi: 10.1371/journal.pone.0011924
Peretto, P., Giachino, C., Panzica, G. C., and Fasolo, A. (2001). Sexually dimorphic neurogenesis is topographically matched with the anterior accessory olfactory bulb of the adult rat. Cell Tissue Res. 306, 385–389. doi: 10.1007/s00441-001-0471-1
Piekarski, D. J., Johnson, C. M., Boivin, J. R., Thomas, A. W., Lin, W. C., Delevich, K., et al. (2017). Does puberty mark a transition in sensitive periods for plasticity in the associative neocortex? Brain Res. 1654, 123–144. doi: 10.1016/j.brainres.2016.08.042
Price, M. A., and Vandenbergh, J. G. (1992). Analysis of puberty-accelerating pheromones. J. Exp. Zool. 264, 42–45. doi: 10.1002/jez.1402640107
Ramm, S. A., Cheetham, S. A., and Hurst, J. L. (2008). Encoding choosiness: female attraction requires prior physical contact with individual male scents in mice. Proc. Biol. Sci. 275, 1727–1735. doi: 10.1098/rspb.2008.0302
Roberts, S. A., Simpson, D. M., Armstrong, S. D., Davidson, A. J., Robertson, D. H., McLean, L., et al. (2010). Darcin: a male pheromone that stimulates female memory and sexual attraction to an individual male’s odour. BMC Biol. 8:75. doi: 10.1186/1741-7007-8-75
Rochefort, C., Gheusi, G., Vincent, J.-D., and Lledo, P.-M. (2002). Enriched odor exposure increases the number of newborn neurons in the adult olfactory bulb and improves odor memory. J. Neurosci. 22, 2679–2689.
Romeo, R. D. (2003). Puberty: a period of both organizational and activational effects of steroid hormones on neurobehavioural development. J. Neuroendocrinol. 15, 1185–1192. doi: 10.1111/j.1365-2826.2003.01106.x
Sakamoto, M., Imayoshi, I., Ohtsuka, T., Yamaguchi, M., Mori, K., and Kageyama, R. (2011). Continuous neurogenesis in the adult forebrain is required for innate olfactory responses. Proc. Natl. Acad. Sci. U.S.A. 108, 8479–8484. doi: 10.3389/fnins.2014.00121
Sakamoto, M., Kageyama, R., and Imayoshi, I. (2014). The functional significance of newly born neurons integrated into olfactory bulb circuits. Front. Neurosci. 8:12. doi: 10.3389/fnins.2014.00121
Saleem, S., Ruggles, P. H., Abbott, W. K., and Carney, G. E. (2014). Sexual experience enhances drosophila melanogaster male mating behavior and success. PLoS ONE 9:e96639. doi: 10.1371/journal.pone.0096639
Sappington, T. W., and Taylor, O. R. (1990). Developmental and environmental sources of pheromone variation inColias eurytheme butterflies. J. Chem. Ecol. 16, 2771–2786. doi: 10.1007/BF00988085
Schellino, R., Trova, S., Cimino, I., Farinetti, A., Jongbloets, B. C., Pasterkamp, R. J., et al. (2016). Opposite-sex attraction in male mice requires testosterone-dependent regulation of adult olfactory bulb neurogenesis. Sci. Rep. 6:36063. doi: 10.1038/srep36063
Sherborne, A. L., Thom, M. D., Paterson, S., Jury, F., Ollier, W. E. R., Stockley, P., et al. (2007). The genetic basis of inbreeding avoidance in house mice. Curr. Biol. 17, 2061–2066. doi: 10.1016/j.cub.2007.10.041
Singer, A. G., Beauchamp, G. K., and Yamazaki, K. (1997). Volatile signals of the major histocompatibility complex in male mouse urine. Proc. Natl. Acad. Sci. U.S.A. 94, 2210–2214. doi: 10.1073/pnas.94.6.2210
Sisk, C. L., and Zehr, J. L. (2005). Pubertal hormones organize the adolescent brain and behavior. Front. Neuroendocrinol. 26:163–174. doi: 10.1016/j.yfrne.2005.10.003
Smith, M. T., Pencea, V., Wang, Z., Luskin, M. B., and Insel, T. R. (2001). Increased number of BrdU-labeled neurons in the rostral migratory stream of the estrous prairie vole. Horm. Behav. 39, 11–21. doi: 10.1006/hbeh.2000.1630
Stowers, L., and Liberles, S. D. (2016). State-dependent responses to sex pheromones in mouse. Curr. Opin. Neurobiol. 38, 74–79. doi: 10.1016/j.conb.2016.04.001
Swerdloff, R. S., and Odell, W. D. (1975). Hormonal mechanisms in the onset of puberty. Postgrad. Med. J. 51, 200–208. doi: 10.1136/pgmj.51.594.200
Szymanski, L. A., and Keller, M. (2014). Activation of the olfactory system in response to male odors in female prepubertal mice. Behav. Brain Res. 271, 30–38. doi: 10.1016/j.bbr.2014.05.051
Tanapat, P., Hastings, N. B., Reeves, A. J., and Gould, E. (1999). Estrogen stimulates a transient increase in the number of new neurons in the dentate gyrus of the adult female rat. J. Neurosci. 19, 5792–5801.
Vandenbergh, J. G. (1967). Effect of the presence of a male on the sexual maturation of female mice. Endocrinology 81, 345–349. doi: 10.1210/endo-81-2-345
Vandenbergh, J. G. (1974). Social determinants of the onset of puberty in rodents. J. Sex Res. 10, 181–193. doi: 10.1080/00224497409550849
Veyrac, A., and Bakker, J. (2011). Postnatal and adult exposure to estradiol differentially influences adult neurogenesis in the main and accessory olfactory bulb of female mice. FASEB J. 25, 1048–1057. doi: 10.1096/fj.10-172635
Villar, G., Baker, T. C., Patch, H. M., and Grozinger, C. M. (2015). Neurophysiological mechanisms underlying sex- and maturation-related variation in pheromone responses in honey bees (Apis mellifera). J. Comp. Physiol. A Neuroethol. Sens. Neural. Behav. Physiol. 201, 731–739. doi: 10.1007/s00359-015-1006-7
Weiler, E. (2005). Postnatal development of the rat vomeronasal organ. Chem. Senses 30, i127–i128. doi: 10.1093/chemse/bjh147
Keywords: puberty, vomeronasal system, neurogenesis, sensory adaptation, sexual signals
Citation: Oboti L, Trova S, Schellino R, Marraudino M, Harris NR, Abiona OM, Stampar M, Lin W and Peretto P (2017) Activity Dependent Modulation of Granule Cell Survival in the Accessory Olfactory Bulb at Puberty. Front. Neuroanat. 11:44. doi: 10.3389/fnana.2017.00044
Received: 23 February 2017; Accepted: 01 May 2017;
Published: 23 May 2017.
Edited by:
Yun-Qing Li, Fourth Military Medical University, ChinaReviewed by:
Mark Spritzer, Middlebury College, United StatesUrsula H. Winzer-Serhan, Texas A&M University College of Dentistry, United States
Copyright © 2017 Oboti, Trova, Schellino, Marraudino, Harris, Abiona, Stampar, Lin and Peretto. This is an open-access article distributed under the terms of the Creative Commons Attribution License (CC BY). The use, distribution or reproduction in other forums is permitted, provided the original author(s) or licensor are credited and that the original publication in this journal is cited, in accordance with accepted academic practice. No use, distribution or reproduction is permitted which does not comply with these terms.
*Correspondence: Livio Oboti, bGl2aW8ub2JvdGlAZ21haWwuY29t Paolo Peretto, cGFvbG8ucGVyZXR0b0B1bml0by5pdA==
†These authors have contributed equally to this work.