- 1Forestry College, Henan University of Science and Technology, Luoyang, China
- 2State Key Laboratory of Integrated Management of Pest Insects and Rodents, Institute of Zoology, Chinese Academy of Sciences, Beijing, China
- 3Department of Entomology, College of Plant Protection, Henan Agricultural University, Zhengzhou, China
- 4State Key Laboratory for Biology of Plant Diseases and Insect Pests, Institute of Plant Protection, Chinese Academy of Agricultural Sciences, Beijing, China
Moths develop sophisticated olfactory systems to sense the airborne chemical cues from the environment. Understanding the structural basis in the neuronal center is a fundamental neuroethological step. Little is known about the emerging crop pest Athetis dissimilis with regard to its morphology or its neuronal organizations. Through antibody staining and digital 3D modeling, we re-constructed the primary olfactory center—the antennal lobe of A. dissimilis. In the antennal lobes 68.8 ± 3.1 male glomeruli and 70.8 ± 1.0 female glomeruli were identified with obvious sexual dimorphism. In particular, male adults of A. dissimilis contain a macroglomerular complex (MGC) that consists of three subunits, while the female lobe has four relatively enlarged glomeruli at the entrance of the antennal nerve. Glomeruli were later clustered with deviation and variance, and referring to reported olfactory related receptor family genes in seven different moth species, we found that glomerular counts of these insects are better related to the sum of odorant receptor and ionotropic receptor numbers, suggesting olfactory receptors and ionotropic receptors may both involved in olfaction of Noctuidae moths.
Introduction
The olfactory system is one of the most important sensory features in insect species, which broadly involves behavioral decisions (Dweck et al., 2015; Ebrahim et al., 2015; Joseph and Carlson, 2015; Wan et al., 2019). Detection of the airborne cues in insects starts in olfactory receptor neurons (ORNs) housed in sensilla that are mostly located on the antennae (Keil and Steinbrecht, 1984). The axons of ORNs directly project to the antennal lobe (AL), where synaptic attachments are made with second-order neurons in sophisticated structures called the glomeruli (Stocker et al., 1990; Christensen and Hildebrand, 2002). Postsynaptic projections in glomeruli contain projection neurons and local neurons, where olfactory signals are concentrated or diluted into the protocerebrum (Homberg et al., 1989). Usually, innate behaviors are decided finally in the lateral horn, and learning and memory driving behaviors are processed in the mushroom body (Yang et al., 1995; Gupta and Stopfer, 2012). Thus, anatomic characterization of ALs is important for neuroethological studies to understand insect behavioral decision (Sato and Touhara, 2008; Bisch-Knaden et al., 2018).
Athetis dissimilis (Hampson; Lepidoptera: Noctuidae) is found in Asian countries including Japan, Korea, India, Philippines, and Indonesia (Dong et al., 2016). It has become an emerging corn pest since its discovery in 2012 in Shandong province, China (Li et al., 2014). Larvae of this species live under plant residues, making it difficult to control. Novel management strategies such as olfaction-based ecological trapping are in urgent demand. Recent works have reported fundamental information on sensillar morphology and chemosensory genes within this species (Dong et al., 2016; Song et al., 2018a,b; Liu et al., 2019). In addition, some other works also involved trials in terms of trapping method development (Guo et al., 2016; Kim et al., 2016). However, the initial information of the ALs in this species remains unknown.
In the current study, we first investigated the morphology of A. dissimilis in all life stages. Utilization of synaptic antibody staining provided the first digital atlas of ALs in this species. Gender-based special structures including the macroglomerular complex (MGC) in males and the large female glomeruli (LFGs) are identified and described. Later comparison and clustering analysis revealed differences between the genders in terms of variations of glomeruli. Finally, a correlation between glomeruli numbers and olfaction-related receptors was carried out to show involvement of receptor classes in olfactory reception of Noctuidae species.
Materials and Methods
Insects
This work used a laboratory colony of both genders of A. dissimilis adults that has been described in previous works (Dong et al., 2016). A wild-type strain was collected at the campus of Academy of Agriculture and Forestry (N34°38′5.35″, E112°27′58.15″) during June to July 2015. Rejuvenation was ensured once every 10 generations by backcrossing with freshly collected field strains. Insects were reared under conditions of 27 ± 1°C with 70 ± 5% relative humidity and maintaining a 16 h: 8 h light/dark cycle.
Wholemount Labeling of Brain
Brains of A. dissimilis were prepared according to previous works (Zhao et al., 2016). Insects were decapitated and ALs were dissected in Ringer’s solution (Jiang et al., 2019) before transfer to 4% paraformaldehyde in 0.1 M phosphate-buffered saline (PBS, pH 7.4) to be fixed at 4°C overnight. Brains were then rinsed in PBS (4 × 15 min) and preincubated with 5% normal goat serum (NGS; Sigma, St. Louis, MO, USA) in 0.1 M PBS containing 0.5% Triton X-100 (PBST; 0.1 M, pH 7.4) at 4°C overnight. SYNORF1 (Developmental Studies Hybridoma Bank, University of Iowa; Klagges et al., 1996; Berg et al., 2002) primary antibody was used at a concentration of 1:100 (with 5% NGS in PBST) to stain the brains at 4°C for 5 days. The brains were later rinsed in PBS (6 × 20 min) and subsequently incubated with Cy2-conjugated anti-mouse (Invitrogen, Eugene, OR, USA; dilution 1:300 with 1% NGS in PBST) for 3 days at 4°C. Finally, the brains were washed in PBS (6 × 20 min) and then dehydrated 20 min for each concentration with ascending ethanol series (including 50%, 70%, 90%, 95%, and 100%) before being cleaned and mounted in methyl salicylate in a perforated aluminum slide with two glass coverslips.
Confocal Image Acquisition, Glomeruli Identification, and Three-Dimensional Reconstructions
All image stacks were acquired with a confocal laser scanning microscope (LSM 780, META Zeiss, Jena, Germany) with a 10× objective (Plan-Neofluar 10×/0.3) on the anti-synapsin immunolabeled whole-mount preparations. An argon laser at 488 nm was used to excite the Cy2 dye. The resolution of the confocal images was set to 1,024 × 1,024 voxels and the section interval was set to 3 or 4 μm. Amira software (AMIRA 5.3, Visage Imaging, Fürth, Germany) was used as previously described to conduct segmentation and reconstruction of the digital atlas of the ALs. Parameters for later analysis were acquired with the TissueStatistics tool embedded in Amira.
Statistical Analysis
A parametric test for comparing volumes and counts between genders was performed using two-way t-tests in SPSS (IBM SPSS Statistics 22.0.0, Chicago, IL, USA). Circos 0.69-9 plots were constructed using Circos (Krzywinski et al., 2009). A dendrogram was developed with the Median Method in Statgraphics Centurion XVII (Statpoint Technologies Inc., Warrenton, VA, USA).
Results
Morphology of A. dissimilis in Different Stages
The egg of A. dissimilis is oval to nearly circular with a diameter of ~450 μm. Sides are truncated by a macropylar area at the anterior end and marked by a reticulate pattern of prominent longitudinal ridges joined by lesser cross ridges (Figure 1A). The male spermatophore body is ovoid and attached to a ~12 mm spermatophore neck (Figure 1B). Larva of A. dissimilis can develop to 6th instar before pupation (Figure 1C). A white dotted line exists along the longitude of the middle dorsal side of the larvae, paralleled with four symmetrical dotted stripes (Figure 1C). The pupa is light brown ventrally and dark reddish brown dorsally. Forewings extend to the fifth section of the abdomen (A5). An anterior row of short, stout, dorsal spines is present on segments A6–8. Sexual dimorphism presents ventrally on A9–10. The cremaster consists of a large pair of stout hooks arising dorsally from A10 (Figures 1D,E). Adults of both genders are moderately large, generally dark grayish moths with a small, white subapical spot on the forewing near the apex of the discal cell and a smaller black one at the anterior of the initial spot (Figures 1F,G). A female adult presents a spade-shaped ovipositor at the tip of the abdomen (Figure 1F), while the male has a phallic organ that is surrounded by a remarkable panicle of hairpencils (Figure 1G).
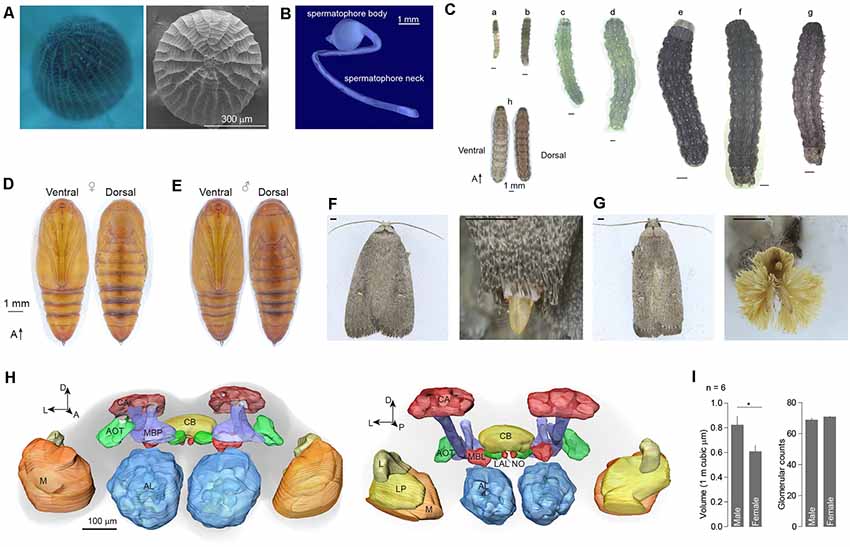
Figure 1. External morphology and brain structure of Athetis dissimilis. (A) Overview of anterior of eggs showing the micropylar area. (B) The spermatophore organ of males. (C) Morphology of different stages of larvae, which included 1st to 6th instars (a–f), matured larva (g), and overview (h). (D) Pupal morphology of females. (E) Pupal morphology of males. (F) Morphology of adults and copulation organs in female. Scale bar indicates 1 mm. (G) Morphology of adults and copulation organs in male. Scale bar indicates 1 mm. (H) Brain reconstruction of A. dissimilis from anterior (left) and posterior (right) views. Arrangement of neuropils: AL (antennal lobe), MBP (mushroom body peduncle), AOT (anterior optic tubercle), CA (calyx), CB (central complex), MBL (mushroom body lobe), LAL (lateral accessory lobe), NO (nodulus), L (lobula), LP (lobula plate), and M (medulla). (I) Comparison of single antennal lobe between male and female A. dissimilis. Asterisk indicates significant larger in volumes of male ALs than females (t-test, t(10) = 2.39, P = 0.0381). No difference was observed in terms of glomerular counts between genders (t-test, t(10) = 1.49, P = 0.166).
Digital Atlas Showed Sexual Dimorphism Between Genders
A total of 12 brains of A. dissimilis were prepared (Supplementary Table S1). ALs within six brains from each gender were analyzed (Supplementary Tables S2, S3). The synaptic specific antibody staining resulted in an intense labeling of the ALs and the key neuropils including mushroom body peduncle, anterior optic tubercle, calyx, central complex, mushroom body lobe, lateral accessory lobe, nodulus, lobula, lobula plate, and medulla (Figure 1H). The total volumes of glomeruli were 822,516.77 ± 180,998.80 μm3 in males and 607,876.42 ± 125,467.75 μm3 in females, respectively (mean ± SD; Supplementary Table S1). Significantly larger ALs were observed in males than in females (Figure 1I). By manual segmentation and cross checking among specimens, we allocated a total of 68.8 ± 3.1 glomeruli in male AL and 70.8 ± 1.0 in female AL, respectively (mean ± SD; Supplementary Table S1). No difference in glomerular counts was observed between genders (Figure 1I).
On the entry of male antenna, there are three enlarged glomeruli forming the MGC: the cumulus (CU), dorsal-anterior (DA), dorsal-posterior (DP; Figure 2A). The CU glomerulus in males has an outstanding volume that was significantly larger than that of any other glomeruli in the ALs. In females, four LFG subunits were located near the antennal nerve (Figure 2B) but did not exhibit remarkable enlargement in volumes. The border of each glomerulus was well captured and individual glomeruli could be identified with ease. Weak staining of the antennal and interglomerular nerve was observed, but no nerve tracts within the glomeruli were visible (Figure 2). All glomeruli were arrayed in a demarcated layer surrounding the hub (Figure 3). As the most anterior and prominent part of the deutocerebrum, ALs were surrounded by the medial cell cluster (MCCl) and the lateral cell cluster (LCCl), which were also strongly labeled yet with no glomerular organization (Figures 3A,B). Both MGCs and LFGs were identical in spatial allocations and relatively larger in size compared to other ordinary glomeruli (Figures 3C,D).
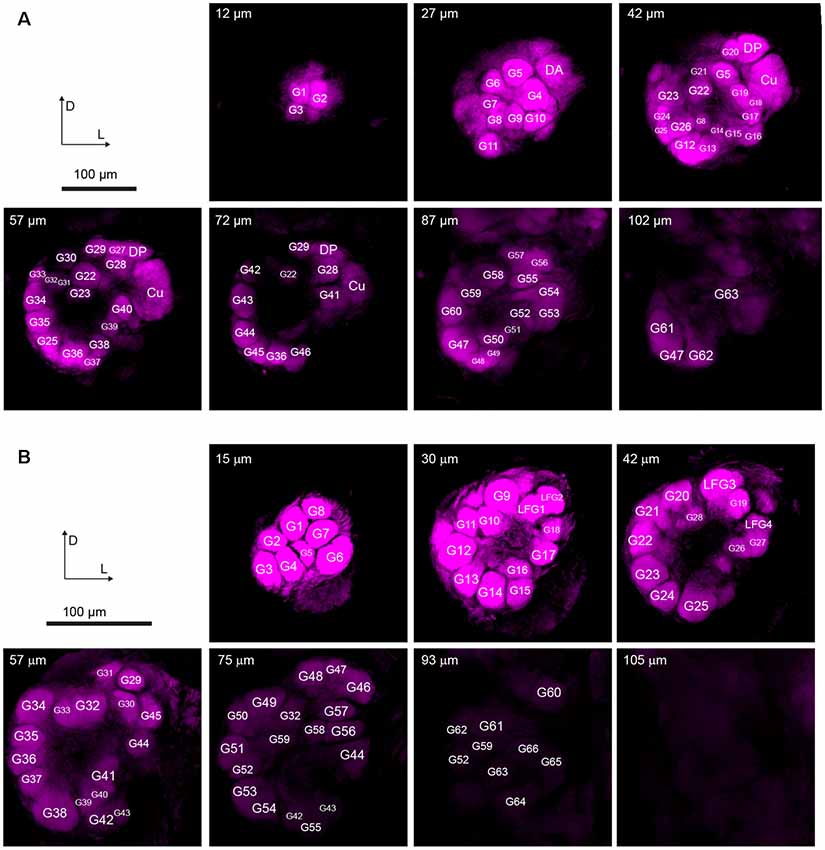
Figure 2. Confocal images showing arrangements of the antennal lobes in both sexes of A. dissimilis. (A) Organization of 66 glomeruli in male A. dissimilis (specimen 3) from the anterior view. Representative depths of 12 μm, 27 μm, 42 μm, 57 μm, 72 μm, 87 μm, and 102 μm are shown, respectively. Macroglomerular complex (MGC) area cluster is indicated by cumulus (CU), dorsal-anterior (DA), and dorsal-posterior (DP). Other ordinary glomeruli (G) are numbered and labeled among replicates. (B) Organization of 70 glomeruli in female A. dissimilis (specimen 1) from the anterior view. Representative depths of 15 μm, 30 μm, 42 μm, 57 μm, 75 μm, 93 μm, and 105 μm are shown, respectively. Large female glomerulus (LFG) area cluster is indicated by LFG 1–4. Other ordinary glomeruli (G) are numbered and labeled among replicates.
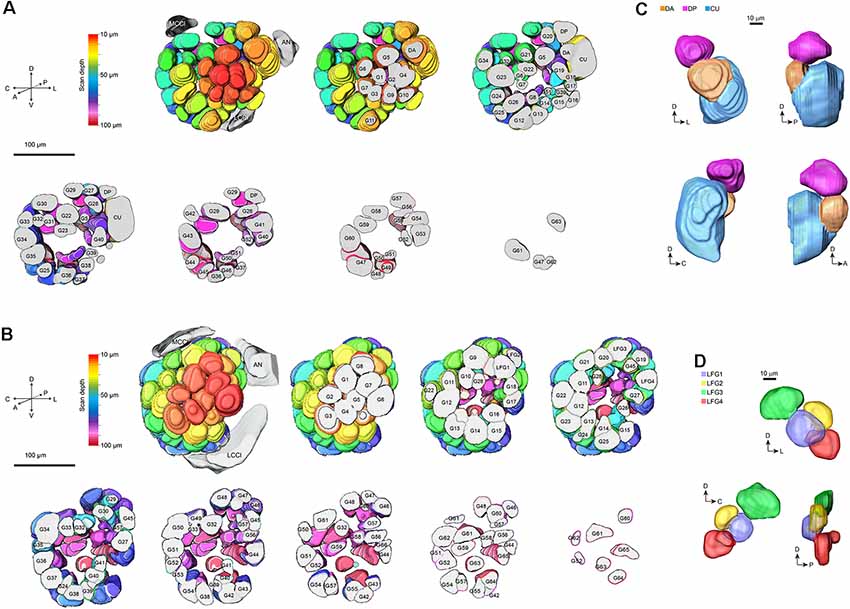
Figure 3. 3-D modeling of antennal lobes in Figure 2. (A) Spatial organizations of glomeruli in male A. dissimilis by the anterior sectional view. Colors indicate scanning depth. Labeling of the glomeruli was the same as that in Figure 2. AN, antennal nerve; LCCl, the lateral cell cluster; MCCl, the medial cell cluster. (B) Spatial organizations of glomeruli in female A. dissimilis by anterior sectional view. Colors indicate scanning depth. Labeling of the glomeruli was the same as that in Figure 2. AN, antennal nerve; LCCl, the lateral cell cluster; MCCl, the medial cell cluster. (C) Anterior, posterior, and anteroposterior views of modeling of the MGC area in males, showing representative spatial organization of CU, DA, and DP, respectively. (D) Anterior, posterior, and anteroposterior views of modeling of the LFG area in females, showing representative spatial organization of LFG 1–4, respectively.
The volume of a single glomerulus ranged from 5,139 ± 1,590 μm3 (G51) to 97,709 ± 19,605 μm3 (CU) in males and 5,156 ± 1,603 μm3 (G26) to 13,338 ± 2,995 μm3 (LFG3) in females, respectively (Supplementary Tables S2, S3). The volume, deviation (meaning the shape of glomeruli), and variation (meaning the consistency of glomeruli among individuals) of each glomerulus were determined using heatmaps of the Circos plot (Figures 4A,B; Krzywinski et al., 2009). Female glomeruli had higher variations in volume, while male glomeruli were relatively more consistent (Figures 4A,B). There were similar proportions of high-deviation glomeruli in ALs of both genders, indicating these glomeruli had various shapes (Figures 4A,B). When all parameters were assembled, several groups of glomeruli were highly correlated, lying in different clusters (Figure 4C). Cumulus formed a cluster distinguishable from other glomeruli, as it was higher in size (Figure 4D). Furthermore, glomerular clusters in A. dissimilis ALs showed different variations in terms of either size or shape; e.g., LFGs were relatively more identical in sizes and shapes while a separated cluster glomerulus G17 showed remarkable variations in sizes and shapes among individuals (Figures 4C,D).
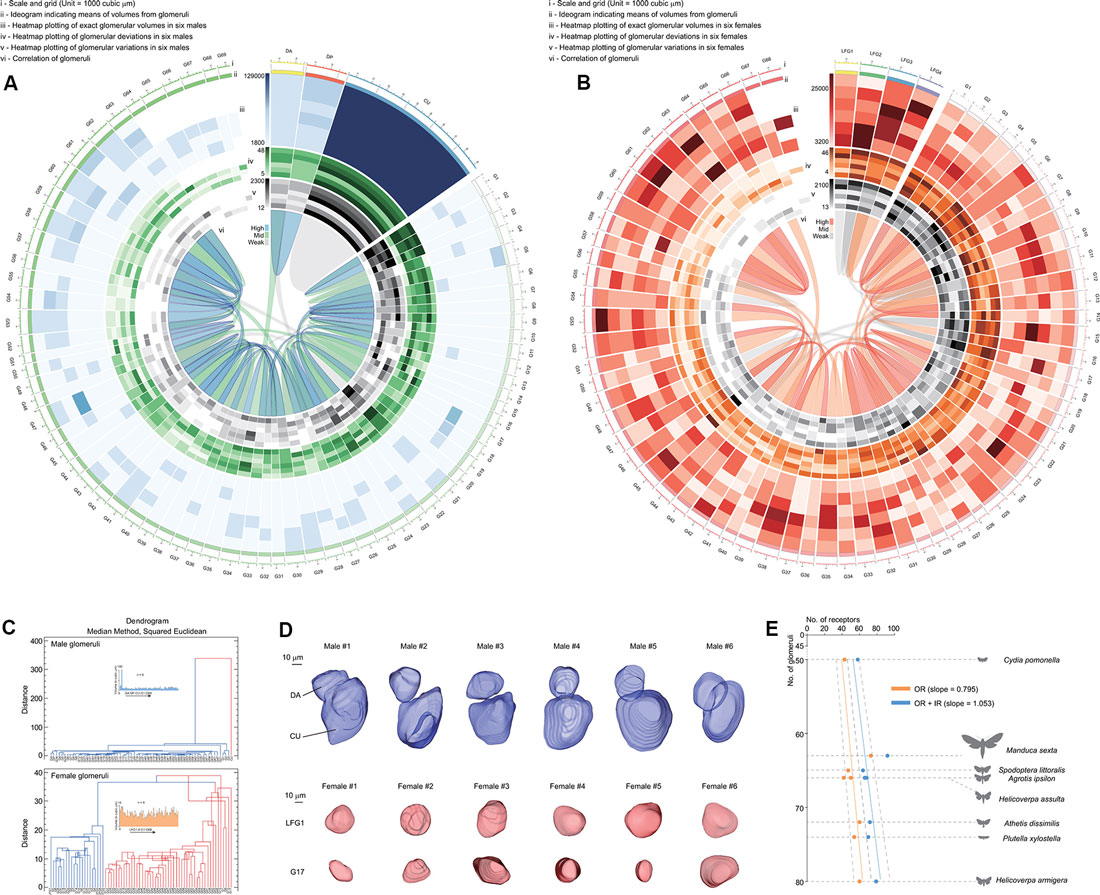
Figure 4. Statistics and analysis of the atlas results in A. dissimilis. (A) Overview of results in males including six replicates by Circos plot using data from Supplementary Table S2. Volume, deviation, and variation of glomeruli in all specimens are respectively shown with heat map circles. Width of the block indicates means of volumes in glomeruli. Blue ribbons indicate strong correlations of data pooled between glomeruli, and green and gray indicate medium and weak correlations. (B) Overview of results in females including six replicates by Circos plot using data from Supplementary Table S3. (C) Dendrograms showing clustering of glomeruli in both sexes pooled from all specimens. The median method using the squared Euclidean distance metric was utilized to analyze volumes, deviations, and variations among glomeruli. (D) Select glomeruli in both sexes and among specimens showing representative characters. DA and LFG1: glomeruli with constant volumes and shapes among replicates. CU and G17: glomeruli that formed distinct clusters with the previous ones, indicating that they were either larger in sizes or more distorted in shape/volume. (E) Exploration of relationships between olfaction-related receptors (odorant receptors, OR; ionotropic receptors, IR) and numbers of glomeruli in ALs in seven moth species. Orange indicates correlation of ORs against glomerular numbers, and blue indicates ORs + IRs against glomerular numbers in each species, respectively. A slope of 1.053 indicates that the sum of both ORs and IRs was better correlated with numbers of glomeruli in each species (linear regression, t(7) = 18.5, P < 0.0001). Dotted lines indicate 95% CI.
Discussion
Antennal Lobe Morphological Conservation in Noctuidae
The moth antennal lobe is well known to be sexually dimorphic. We found that the arrangements of A. dissimilis antennal lobe is conserved within Noctuidae species. Male A. dissimilis moth shows three enlarged subunits in MGC of ALs and this three-part MGC arrangement is also similar to that of other reported species including Helicoverpa armigera, H. assulta, and Mythimna separata (Wu et al., 2015; Jiang et al., 2019). Specific glomeruli of MGC serving as projections are those of pheromone tuning ORNs and the major pheromone component generally projects to the largest subunit, CU (Hansson et al., 1992). To recognize pheromone more sensitively, male MGC show increasing size among olfactory glomeruli under high selection pressure (Hansson and Stensmyr, 2011). In contrast to the MGC, female LFGs were thought to be involved in encoding olfactory information of female-specific ovipositional behavior but more research is needed to confirm this. For A. dissimilis, our research offered a new perspective to understand how this pest senses olfactory cues. The three-part MGC arrangement of the male antennal lobe indicates that the sex pheromone of females may consist of two components. However, sex pheromones of female moths still need to be identified precisely. The ordinary glomeruli (OGs) have been reported to process plant odor information (Christensen and Hildebrand, 2002). In attempts to characterize the OGs in A. dissimilis functionally, further in vivo optical imaging or intracellular recording will be worthwhile to utilize in this pest.
Olfactory Receptor Neurons and Ionotropic Receptor Neurons May Both Project to Antennal Lobes
In insects, ORNs expressing certain odorant receptor (ORs) that project from the antenna to the corresponding glomerulus (Vosshall and Stocker, 2007). We asked what kind of chemosensory receptors are involved by comparison between numbers of receptors and glomeruli as based on the one OR/one ORN rule (Vosshall et al., 2000). When comparing glomeruli with ORs in several reported moth species including A. dissimilis (Supplementary Table S4; Bengtsson et al., 2012; Jacquin-Joly et al., 2012; Liu et al., 2012, 2014; Poivet et al., 2013; Gu et al., 2014; Koenig et al., 2015; Xu et al., 2015; Zhang et al., 2015; Dong et al., 2016; Yang et al., 2017), the slope is 0.795, indicating that more glomeruli were not projected by ORs. The reason may be that other chemosensory receptor neurons may also be involved in projection to the ALs. Recent work has revealed that ionotropic receptors (IRs) are involved in olfaction in moths (Tang et al., 2020). When additional IRs were added to the correlation, we found a better slope of 1.053, meaning each receptor can project to its corresponding glomerulus (Figure 4E). This provided evidence that olfactory processes in moths involve both ORs and IRs at the periphery. The slope of 1.053 from ORs + IRs to glomeruli in A. dissimilis moths actually is not perfectly correlated, indicating that there are more receptor types than the number of glomeruli within one species. In Drosophila, it is reported that more than one IR may project to the same glomerulus in the ALs (Grabe et al., 2016). We thus speculated that a similar mechanism may also occur in moth species, indicating that several different IRs may project to the same glomerulus.
Data Availability Statement
All datasets generated for this study are included in the article/Supplementary Material.
Author Contributions
RT and X-CZ conceived the project. J-FD and X-CZ conducted the experiments. N-JJ and RT analyzed the data. RT drafted the manuscript with inputs from all.
Funding
This work was supported by the Program for Science and Technology Innovation Talents in Universities of Henan Province (19HASTIT011), Scientific and Technological Research Projects in Henan Province (182102110182), Agricultural Transformation of Scientific and Technological Achievements of Tianjin (201901070), and Central Public-Interest Scientific Institution Basal Research Fund (No. Y2020GH21-1) of China.
Conflict of Interest
The authors declare that the research was conducted in the absence of any commercial or financial relationships that could be construed as a potential conflict of interest.
Acknowledgments
We thank B. F. A. Xiao-Qian Bao for technical supports on development of insect graphics.
Supplementary Material
The Supplementary Material for this article can be found online at: https://www.frontiersin.org/articles/10.3389/fnana.2020.00023/full#supplementary-material.
References
Bengtsson, J. M., Trona, F., Montagné, N., Anfora, G., Ignell, R., Witzgall, P., et al. (2012). Putative chemosensory receptors of the codling moth, Cydia pomonella, identified by antennal transcriptome analysis. PLoS One 7:e31620. doi: 10.1371/journal.pone.0031620
Berg, B. G., Galizia, C. G., Brandt, R., and Mustaparta, H. (2002). Digital atlases of the antennal lobe in two species of tobacco budworm moths, the oriental helicoverpa assulta (male) and the american heliothis virescens (male and female). J. Comp. Neurol. 446, 123–134. doi: 10.1002/cne.10180
Bisch-Knaden, S., Dahake, A., Sachse, S., Knaden, M., and Hansson, B. S. (2018). Spatial representation of feeding and oviposition odors in the brain of a hawkmoth. Cell Rep. 22, 2482–2492. doi: 10.1016/j.celrep.2018.01.082
Christensen, T. A., and Hildebrand, J. G. (2002). Pheromonal and host-odor processing in the insect antennal lobe: how different? Curr. Opin. Neurobiol. 12, 393–399. doi: 10.1016/s0959-4388(02)00336-7
Dong, J., Song, Y., Li, W., Shi, J., and Wang, Z. (2016). Identification of putative chemosensory receptor genes from the Athetis dissimilis antennal transcriptome. PLoS One 11:e0147768. doi: 10.1371/journal.pone.0147768
Dweck, H. K., Ebrahim, S. A., Thoma, M., Mohamed, A. A., Keesey, I. W., Trona, F., et al. (2015). Pheromones mediating copulation and attraction in Drosophila. Proc. Natl. Acad. Sci. U S A 112, E2829–E2835. doi: 10.1073/pnas.1504527112
Ebrahim, S. A., Dweck, H. K., Stökl, J., Hofferberth, J. E., Trona, F., Weniger, K., et al. (2015). Drosophila avoids parasitoids by sensing their semiochemicals via a dedicated olfactory circuit. PLoS Biol. 13:e1002318. doi: 10.1371/journal.pbio.1002318
Grabe, V., Baschwitz, A., Dweck, H. K. M., Lavista-Llanos, S., Hansson, B. S., and Sachse, S. (2016). Elucidating the neuronal architecture of olfactory glomeruli in the Drosophila antennal lobe. Cell Rep. 16, 3401–3413. doi: 10.1016/j.celrep.2016.08.063
Gu, S. H., Sun, L., Yang, R. N., Wu, K. M., Guo, Y. Y., Li, X. C., et al. (2014). Molecular characterization and differential expression of olfactory genes in the antennae of the black cutworm moth Agrotis ipsilon. PLoS One 9:e103420. doi: 10.1371/journal.pone.0103420
Guo, T. T., Li, L. L., Men, X. Y., Lu, Z. B., Chen, H., Wang, Z. Y., et al. (2016). Impact of temperature on the growth and development of Athetis dissimilis (Lepidoptera: Noctuidae). J. Econ. Entomol. 110, 274–281. doi: 10.1093/jee/tow229
Gupta, N., and Stopfer, M. (2012). Functional analysis of a higher olfactory center, the lateral horn. J. Neurosci. 32, 8138–8148. doi: 10.1523/JNEUROSCI.1066-12.2012
Hansson, B. S., Ljungberg, H., Hallberg, E., and Löfstedt, C. (1992). Functionalspecialization of olfactory glomeruli in a moth. Science 256, 1313–1315. doi: 10.1126/science.1598574
Hansson, B. S., and Stensmyr, M. C. (2011). Evolution of insect olfaction. Neuron 72, 698–711. doi: 10.1016/j.neuron.2011.11.003
Homberg, U., Christensen, T. A., and Hildebrand, J. G. (1989). Structure and function of the deutocerebrum in insects. Annu. Rev. Entomol. 34, 477–501. doi: 10.1146/annurev.en.34.010189.002401
Jacquin-Joly, E., Legeai, F., Montagné, N., Monsempes, C., François, M.-C., Poulain, J., et al. (2012). Candidate chemosensory genes in female antennae of the noctuid moth Spodoptera littoralis. Int. J. Biol. Sci. 8, 1036–1050. doi: 10.7150/ijbs.4469
Jiang, N. J., Tang, R., Wu, H., Xu, M., Ning, C., Huang, L. Q., et al. (2019). Dissecting sex pheromone communication of Mythimna separata (Walker) in North China from receptor molecules and antennal lobes to behavior. Insect Biochem. Mol. Biol. 111:103176. doi: 10.1016/j.ibmb.2019.103176
Joseph, R. M., and Carlson, J. R. (2015). Drosophila chemoreceptors: a molecular interface between the chemical world and the brain. Trends Genet. 31, 683–695. doi: 10.1016/j.tig.2015.09.005
Keil, T. A., and Steinbrecht, R. A. (1984). “Mechanosensitive and olfactory sensilla of insects,” in Insect Ultrastructure, eds R. C. King and H. Akai (New York, NY: Springer), 477–516.
Kim, J., Byun, B.-K., Oh, H.-W., Jang, S. A., and Park, C. G. (2016). Athetis dissimilis (Lepidoptera: Noctuidae) is attracted to the sex pheromone of Euzophera batangensis (Lepidoptera: Pyralidae). J. Asia Pac. Entomol. 19, 841–845. doi: 10.1016/j.aspen.2016.07.016
Klagges, B. R. E., Heimbeck, G., Godenschwege, T. A., Hofbauer, A., Pflugfelder, G. O., Reifegerste, R., et al. (1996). Invertebrate synapsins: a single gene codes for several isoforms in Drosophila. J. Neurosci. 16, 3154–3165. doi: 10.1523/JNEUROSCI.16-10-03154.1996
Koenig, C., Hirsh, A., Bucks, S., Klinner, C., Vogel, H., Shukla, A., et al. (2015). A reference gene set for chemosensory receptor genes of Manduca sexta. Insect Biochem. Mol. Biol. 66, 51–63. doi: 10.1016/j.ibmb.2015.09.007
Krzywinski, M., Schein, J., Birol, I., Connors, J., Gascoyne, R., Horsman, D., et al. (2009). Circos: an information aesthetic for comparative genomics. Genome Res. 19, 1639–1645. doi: 10.1101/gr.092759.109
Li, J., Yu, Y., Zhang, A., Men, X., Zhou, X., Zhai, Y., et al. (2014). Morphologically alike species of Athetis lepigone (Möschler)—Athetis dissimilis (Hampson) found in Shandong Province of China. Plant Prot. 40, 193–195. doi: 10.3969/j.issn.0529-1542.2014.06.038
Liu, Y., Gu, S., Zhang, Y., Guo, Y., and Wang, G. (2012). Candidate olfaction genes identified within the Helicoverpa armigera antennal transcriptome. PLoS One 7:e48260. doi: 10.1371/journal.pone.0048260
Liu, X. L., Sun, S. J., Khuhro, S. A., Elzaki, M. E. A., Yan, Q., and Dong, S. L. (2019). Functional characterization of pheromone receptors in the moth Athetis dissimilis (Lepidoptera: Noctuidae). Pestic. Biochem. Physiol. 158, 69–76. doi: 10.1016/j.pestbp.2019.04.011
Liu, N. Y., Xu, W., Papanicolaou, A., Dong, S. L., and Anderson, A. (2014). Identification and characterization of three chemosensory receptor families in the cotton bollworm Helicoverpa armigera. BMC Genomics 15:597. doi: 10.1186/1471-2164-15-597
Poivet, E., Gallot, A., Montagné, N., Glaser, N., Legeai, F., and Jacquin-Joly, E. (2013). A comparison of the olfactory gene repertoires of adults and larvae in the noctuid moth Spodoptera littoralis. PLoS One 8:e60263. doi: 10.1371/journal.pone.0060263
Sato, K., and Touhara, K. (2008). “Insect olfaction: receptors, signal transduction, and behavior,” in Chemosensory Systems in Mammals, Fishes, and Insects, eds S. Korsching and W. Meyerhof (Berlin; Heidelberg: Springer), 203–220.
Song, Y. Q., Dong, J., and Sun, H. (2018a). Types and distribution of sensilla on larval antennae and mouthparts of Athetis dissimilis. Plant Prot. 2, 122–128. doi: 10.16688/j.zwbh.2017258
Song, Y. Q., Dong, J. F., and Sun, H. Z. (2018b). Scanning electron microscopic observation on antennal sensilla of Athetis dissimilis. J. Chin. Electron Microsc. Soci. 1, 77–83.
Stocker, R., Lienhard, M., Borst, A., and Fischbach, K. (1990). Neuronal architecture of the antennal lobe in Drosophila melanogaster. Cell Tissue Res. 262, 9–34. doi: 10.1007/bf00327741
Tang, R., Jiang, N. J., Ning, C., Li, G. C., Huang, L. Q., and Wang, C. Z. (2020). The olfactory reception of acetic acid and ionotropic receptors in the Oriental armyworm, Mythimna separata Walker. Insect Biochem. Mol. Biol. 118:103312. doi: 10.1016/j.ibmb.2019.103312
Vosshall, L. B., and Stocker, R. F. (2007). Molecular architecture of smell and taste in Drosophila. Annu. Rev. Neurosci. 30, 505–533. doi: 10.1146/annurev.neuro.30.051606.094306
Vosshall, L. B., Wong, A. M., and Axel, R. (2000). An olfactory sensory map in the fly brain. Cell 102, 147–159. doi: 10.1016/s0092-8674(00)00021-0
Wan, F., Yin, C., Tang, R., Chen, M., Wu, Q., Huang, C., et al. (2019). A chromosome-level genome assembly of Cydia pomonella provides insights into chemical ecology and insecticide resistance. Nat. Commun. 10:4237. doi: 10.1038/s41467-019-12175-9
Wu, H., Xu, M., Hou, C., Huang, L. Q., Dong, J. F., and Wang, C. Z. (2015). Specific olfactory neurons and glomeruli are associated to differences in behavioral responses to pheromone components between two Helicoverpa species. Front. Behav. Neurosci. 9:206. doi: 10.3389/fnbeh.2015.00206
Xu, W., Papanicolaou, A., Liu, N. Y., Dong, S. L., and Anderson, A. (2015). Chemosensory receptor genes in the Oriental tobacco budworm Helicoverpa assulta. Insect Mol. Biol. 24, 253–263. doi: 10.1111/imb.12153
Yang, M. Y., Armstrong, J. D., Vilinsky, I., Strausfeld, N. J., and Kaiser, K. (1995). Subdivision of the Drosophila mushroom bodies by enhancer-trap expression patterns. Neuron 15, 45–54. doi: 10.1016/0896-6273(95)90063-2
Yang, S., Cao, D., Wang, G., and Liu, Y. (2017). Identification of genes involved in chemoreception in Plutella xyllostella by antennal transcriptome analysis. Sci. Rep. 7:11941. doi: 10.1038/s41598-017-11646-7
Zhang, J., Wang, B., Dong, S., Cao, D., Dong, J., Walker, W. B., et al. (2015). Antennal transcriptome analysis and comparison of chemosensory gene families in two closely related noctuidae moths, Helicoverpa armigera and H. assulta. PLoS One 10:e0117054. doi: 10.1371/journal.pone.0117054
Keywords: antennal lobe, Athetis dissimilis, digital atlas, glomerulus, sexual dimorphism
Citation: Dong J-F, Jiang N-J, Zhao X-C and Tang R (2020) Antennal Lobe Atlas of an Emerging Corn Pest, Athetis dissimilis. Front. Neuroanat. 14:23. doi: 10.3389/fnana.2020.00023
Received: 08 February 2020; Accepted: 16 April 2020;
Published: 28 May 2020.
Edited by:
Jackson Cioni Bittencourt, University of São Paulo, BrazilReviewed by:
Yang Liu, Institute of Plant Protection (CAAS), ChinaRosa M. Villalba, Emory University, United States
Copyright © 2020 Dong, Jiang, Zhao and Tang. This is an open-access article distributed under the terms of the Creative Commons Attribution License (CC BY). The use, distribution or reproduction in other forums is permitted, provided the original author(s) and the copyright owner(s) are credited and that the original publication in this journal is cited, in accordance with accepted academic practice. No use, distribution or reproduction is permitted which does not comply with these terms.
*Correspondence: Xin-Cheng Zhao, eGluY2hlbmdAaGVuYXUuZWR1LmNu; Rui Tang, bWluYW1vQDE2My5jb20=
† These authors have contributed equally to this work
‡ORCID: Nan-Ji Jiang orcid.org/0000-0003-4251-2795
Rui Tang orcid.org/0000-0002-9313-0802