- 1Laboratory of Cellular and Molecular Neurobiology of Parkinson’s Disease, Research Center for Molecular Medicine and Chronic Diseases (CIMUS), Deptartment of Morphological Sciences, Health Research Institute of Santiago de Compostela (IDIS), University of Santiago de Compostela, Santiago de Compostela, Spain
- 2Networking Research Center on Neurodegenerative Diseases (CiberNed), Madrid, Spain
- 3Department of Clinical Neurology, Hospital Alvaro Cunqueiro, University Hospital Complex, Vigo, Spain
Parkinson’s disease (PD) is characterized by the progressive loss of dopaminergic neurons in the substantia nigra. However, other non-dopaminergic neuronal systems such as the serotonergic system are also involved. Serotonergic dysfunction is associated with non-motor symptoms and complications, including anxiety, depression, dementia, and sleep disturbances. This pathology reduces patient quality of life. Interaction between the serotonergic and other neurotransmitters systems such as dopamine, noradrenaline, glutamate, and GABA controls the activity of striatal neurons and are particularly interesting for understanding the pathophysiology of PD. Moreover, serotonergic dysfunction also causes motor symptoms. Interestingly, serotonergic neurons play an important role in the effects of L-DOPA in advanced PD stages. Serotonergic terminals can convert L-DOPA to dopamine, which mediates dopamine release as a “false” transmitter. The lack of any autoregulatory feedback control in serotonergic neurons to regulate L-DOPA-derived dopamine release contributes to the appearance of L-DOPA-induced dyskinesia (LID). This mechanism may also be involved in the development of graft-induced dyskinesias (GID), possibly due to the inclusion of serotonin neurons in the grafted tissue. Consistent with this, the administration of serotonergic agonists suppressed LID. In this review article, we summarize the interactions between the serotonergic and other systems. We also discuss the role of the serotonergic system in LID and if therapeutic approaches specifically targeting this system may constitute an effective strategy in PD.
Introduction
Parkinson’s disease (PD) is one of the most common neurodegenerative disorders, which is characterized by the progressive loss of dopaminergic neurons in the substantia nigra compacta (SNc). Dopamine replacement therapy using the precursor L-DOPA is the main treatment for the disease. However, long-term use of L-DOPA leads to the development of dyskinesias and non-motor manifestations (Espay et al., 2018), showing that the pathological process extends beyond the dopaminergic system and that other neurotransmitter systems such as the serotonergic system are involved.
The dorsal raphe nucleus (DRN) contains the largest group of serotonin-producing neurons, and changes in DRN function have been implicated in neuropsychiatric diseases and movement disorders (Hornung, 2010; Huot et al., 2011). Classical studies using tracing techniques and recent works using single-cell RNA sequencing, in situ hybridization and adeno-associated viruses technology showed a dense serotonergic innervation of basal ganglia, including the caudate nucleus and SNc (Dahlström and Fuxe, 1964; Lavoie and Parent, 1990; Muzerelle et al., 2016; Huang et al., 2019).
Nowadays, seven classes of serotonin receptors (5-HT1–7) and at least 15 receptor subtypes have been identified (Hoyer et al., 2002; Hannon and Hoyer, 2008). Some of these receptors (5-HT2C, 5-HT6, 5-HT7) may have a constitutive activity, which may be associated with pathophysiological conditions (De Deurwaerdère et al., 2020). Type 1A/1B and 2A receptors (5-HT1A/1B and 5-HT2A) appear particularly interesting for PD (Huot and Fox, 2013).
Interactions Between Serotonin and Other Neurotransmitters in the Basal Ganglia
Several studies have highlighted a crucial role for the interactions between serotonergic and other neurotransmitter systems in movement control and pathophysiology of the basal ganglia (Di Matteo et al., 2008; Parent et al., 2011), and particularly PD (Ciranna, 2006; Figure 1).
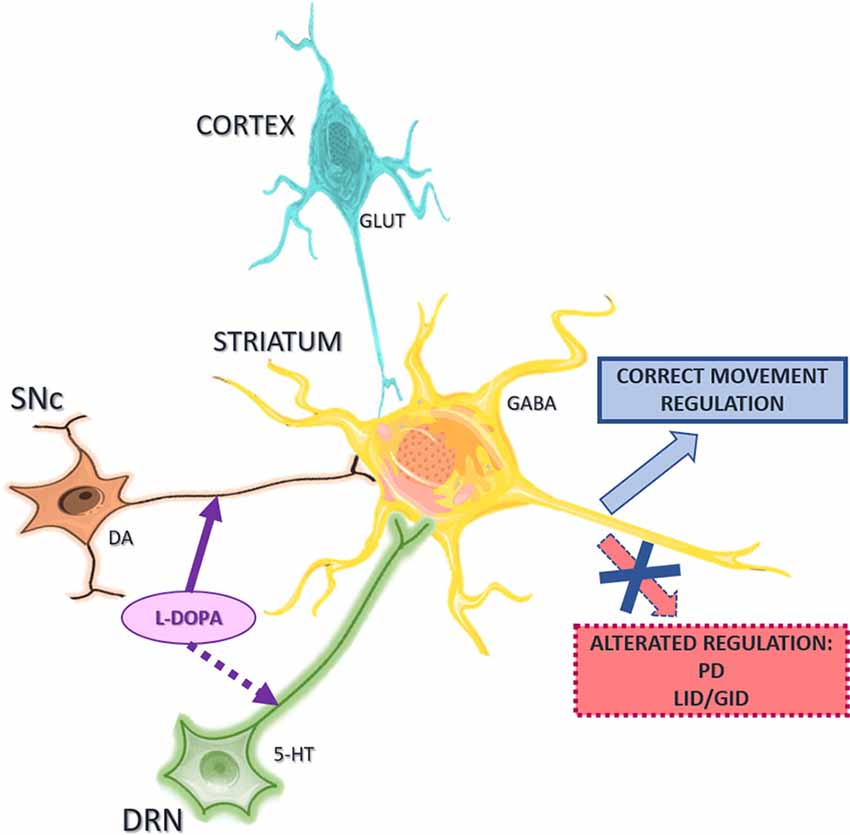
Figure 1. Schematic representation of the major neuronal neurotransmitter systems acting on striatal projection neurons. Interactions between serotonergic, glutamatergic, and dopaminergic systems control the activity of striatal neurons for correct regulation of movement. Abnormal interactions lead to abnormal movement and neurological disorders such as Parkinson’s disease (PD), L-DOPA-induced dyskinesia (LID) or graft-induced dyskinesia (GID). Abbreviations: SNc, substantia nigra pars compacta; DRN, dorsal raphe nucleus; 5-HT, serotonin; DA, dopamine, GLUT, glutamate. The figure was produced using Servier Medical Art (www.servier.com).
Interactions With the Dopaminergic System
Interactions between serotonin and dopamine have been investigated for decades, but the role of the serotonergic transmission in modulating the activity of dopaminergic neurons is still unclear (De Deurwaerdère and Di Giovanni, 2017; Ogawa and Watabe-Uchida, 2018). Several studies have suggested that serotonin input is inhibitory (Sinton and Fallon, 1988; Arborelius et al., 1993), as chronic serotonin transporter (SERT) blockade using serotonin-selective reuptake inhibitors (SSRIs) reduces dopaminergic signaling and elicits basal ganglia dysfunction (Morelli et al., 2011). However, DNR lesions did not affect SNc activity in other experiments (Kelland et al., 1990), and the lack of serotonin in the Tph2 (tryptophan hydroxylase 2, the rate-limiting enzyme for serotonin synthesis) knockout mice did not change the number of dopaminergic neurons (Gutknecht et al., 2012). However, recent optogenetic studies also showed interactions between the dopamine and serotonin systems, involving the mesolimbic system at the level of the ventral tegmental area in controlling motivation (McDevitt et al., 2014; Browne et al., 2019). Moreover, optogenetic stimulation of serotonergic terminals induced dopamine release from serotonin terminals following treatment with L-DOPA, with a loss of serotonin-mediated synaptic transmission (Gantz et al., 2015).
A depletion of striatal serotonin after dopaminergic lesions and in parkinsonian brains has been observed (Karstaedt et al., 1994; Rylander et al., 2010). However, in the neonatal brain, dopaminergic lesions with 6-hydroxydopamine (6-OHDA) led to striatal serotonergic hyperinnervation (Stachowiak et al., 1984; Snyder et al., 1986; Towle et al., 1989; Avale et al., 2004; Brown and Gerfen, 2006). In adult rodents, the sprouting of striatal serotonergic afferents was observed after dopaminergic lesions (Zhou et al., 1991; Guerra et al., 1997; Rozas et al., 1998; Maeda et al., 2003). Interestingly, dopamine-rich intrastriatal grafts did not prevent or revert the 6OHDA-induced serotonergic hyperinnervation (Guerra et al., 1997). Consistent with hyperinnervation, 6-OHDA lesions significantly increased firing discharges of serotonin neurons (Zhang et al., 2007; Kaya et al., 2008; Wang et al., 2009; Prinz et al., 2013). However, other studies did not observe changes (Miguelez et al., 2011, 2016) or even a decrease in the firing activity (Guiard et al., 2008). An increase in serotonin levels only in the first week (Silva et al., 2016) and a decrease of SERT availability after the 6-OHDA lesion (Walker et al., 2019) was also observed. Different experimental protocols, age of the animals, site of injection, and survival time after lesion may explain discrepancies between studies.
Interactions between dopaminergic and serotonergic systems were also observed during development (Lauder, 1990). In rat mesencephalic precursors, the reduction of serotonin levels induced an increase in the differentiation of dopaminergic neurons. Conversely, serotonin decreased the generation of dopaminergic neurons from mesencephalic precursors via serotonin type 7 and type 4 receptors (Rodriguez-Pallares et al., 2003; Parga et al., 2007).
Interactions With the Glutamatergic System
In the basal ganglia, several studies have shown interactions of dopaminergic and serotonergic afferents with corticostriatal glutamatergic terminals. Fenfluramine is a halogenated amphetamine derivative thought to induce serotonin release and to reduce re-uptake. Fenfluramine induced striatal expression of Fos (used as a neuronal activity marker), which was reduced by dopaminergic and serotonergic lesions and suppressed by NMDA glutamate receptor antagonists, suggesting that stimulation of glutamate receptors is essential for the observed neuronal response (Guerra et al., 1998). Furthermore, Fenfluramine induced an increase in striatal levels of preproenkephalin mRNA, and this increase was blocked by dopamine receptor antagonists, NMDA glutamate receptor antagonists, or serotonergic lesions (Liste et al., 2000). These interactions are also supported by other studies showing that intraneuronal signaling pathways may interact to regulate gene transcription in the striatum (Ciranna, 2006). Consistent with this, 5-HT1A activation decreased glutamate release from corticostriatal projections (Dupre et al., 2013; Miguelez et al., 2014), and serotonergic denervation led to the loss of the serotonin inhibitory control on glutamate release (Vermeiren et al., 2018). It was also observed that a glutamatergic projection arising from the DRN-VGluT3 neurons provide excitatory synaptic input to the mesoaccumbens dopamine neurons. The discovery of this pathway opens new avenues to examine its participation in mental disorders related to motivation (Qi et al., 2014). Furthermore, a path-specific input from DRN serotonergic neurons to the ventral tegmental area promotes reward by the release of glutamate and activation of mesoaccumbens dopamine neurons (Wang H. L. et al., 2019). Interestingly, DRN serotonin neurons receive both excitatory and inhibitory inputs from the same brain areas, including the susbstantia nigra and cerebral cortex, to control neuronal activity (Zhou et al., 2017).
Interactions With the Noradrenergic System
Although it is usually considered that the striatum is not significantly innervated by the noradrenergic system, locus coeruleus neurons send direct projections to the main striatal afferent systems, including the serotonergic system (Aston-Jones and Grzanna, 1995). The lack of serotonin innervation in the Tph knockout mice model induces a reduction in the number of noradrenergic neurons and noradrenaline levels in the locus coeruleus (Gutknecht et al., 2012; Pratelli and Pasqualetti, 2019). Analysis of the striatal responses to amphetamine is a useful tool to study the interaction between the noradrenergic and serotonergic systems. Amphetamine acts by increasing dopamine levels, but other neurochemical systems are also involved. The α1-adrenergic receptor antagonist Prazosin or lesions of the serotonergic system reduced locomotor activity and the striatal expression of Fos induced by amphetamine (Muñoz et al., 2003). These results showed that the noradrenergic and serotonergic systems play an important role in modulating the activity of striatal neurons. Other studies were consistent with this as they showed that the release of serotonin is subjected to noradrenergic influence mediated by α1-adrenergic receptors and that administration of Prazosin reduces serotonin levels (Rouquier et al., 1994; Hjorth et al., 1995; Rea et al., 2010). Moreover, studies using the 6-OHDA model revealed that both noradrenaline and serotonin depletion contribute to dysregulation of the basal ganglia in PD (Delaville et al., 2012). Furthermore, both noradrenergic and serotonergic systems modulate neurotransmission in the prefrontal cortex, which is altered in several psychiatric and neurological disorders (Hensler et al., 2013). Indeed, the most widely used antidepressants are SSRIs and noradrenaline reuptake inhibitors. A electrophysiological study showed that L-DOPA did not modify the basal neuronal activity in the locus coeruleus, however, it enhanced the response to noradrenaline reuptake inhibitors and decreased the effect of SSRI antidepressants (Miguelez et al., 2013).
Interactions With the GABAergic System
Serotonin exerts a modulatory action on the effects of gamma-amino-butyric acid (GABA), which is the main brain neurotransmitter mediating inhibitory signals. Deficiency in brain serotonin results in alterations in the GABAergic system (Pratelli and Pasqualetti, 2019). The use of low doses of diazepam is enough to induce effects in Tph2 −/− mice, while they are not effective in wild type mice (Mosienko et al., 2015). At the presynaptic level, serotonin inhibits GABA release via 5-HT1A and 5-HT1B receptors and stimulates GABA release via 5-HT3 and 5HT2 receptors. GABA-mediated effects can also be modulated by serotonin at a post-synaptic level through different receptors and mechanisms, as observed in pyramidal neurons from the prefrontal cortex, hippocampus or thalamus (Ciranna, 2006; Miguelez et al., 2014). In GABAergic neurons, selective 5-HT1A receptor-mediated signaling paradoxically increases c-fos expression and induces excitation in the prefrontal cortex pyramidal neurons (Masana et al., 2012; Hensler et al., 2013). However, the interactions are complex because serotonin also modulates other neurotransmitters. A deeper knowledge of neurotransmitter interactions will provide useful strategies for the therapy of several diseases (see Table 1).
Serotonin and Parkinson’s Disease
PD patients and PD animal models showed serotonergic neuronal loss and Lewy bodies within serotonergic neurons (Paulus and Jellinger, 1991; Huot and Fox, 2013). Moreover, serotonin levels and SERT expression are reduced in several nuclei in PD (Ciranna, 2006; Rylander et al., 2010). However, several findings indicate that the loss of SERT is not correlated with the disease duration and disability (Politis et al., 2010a; Politis and Loane, 2011). In the basal ganglia, changes in receptor expression were also observed, such as the increase in 5-HT2C levels and a decrease in 5-HT1A expression (Fox and Brotchie, 2000; Ballanger et al., 2012). Nevertheless, the differential expression of these receptors between regions and discrepancies between different studies using PD models have also been published (Miguelez et al., 2014). Serotonin signaling modulates the RhoA/Rho kinase pathway (Mair et al., 2008; Tanaka et al., 2014), which is involved in neuroinflammation and neurodegenerative disorders such as PD (Labandeira-Garcia et al., 2015; Koch et al., 2018). In PD, serotonin dysfunction, together with the noradrenergic dysfunction (Vermeiren and De Deyn, 2017), are involved in non-motor symptoms such as depression, weight loss, fatigue, and sleep disturbances. Recent studies have shown that administration of the serotonin precursor 5-hydroxytryptophan improves depressive symptoms in PD patients (Meloni et al., 2020). Furthermore, serotonin dysregulation leads to motor alterations such as tremor, L-DOPA-induced dyskinesia (LID), and graft-induced dyskinesias (GID).
Involvement of Serotonin in L-DOPA-Induced Dyskinesias
Evidence from animal and human studies shows that striatal serotonergic terminals may contribute to the development of LID by promoting a non- physiological release of dopamine (Carta et al., 2007; Rylander et al., 2010; Navailles and De Deurwaerdere, 2012; Politis et al., 2014; Jenner, 2018).
The efficacy of L-DOPA is attributed to its conversion into dopamine by the enzyme aromatic L-amino acid decarboxylase (AADC) in striatal dopaminergic terminals. However, in advanced stages of the disease, the dopaminergic denervation is almost complete and other cell types showing AADC activity convert exogenous L-DOPA into dopamine, including serotonergic terminals (Arai et al., 1994; Maeda et al., 2005), endothelial cells (Melamed et al., 1980), glial cells (Li et al., 1992), and monoaminergic or nonaminergic striatal neurons (Mura et al., 1995; Lopez-Real et al., 2003). It was initially suggested that L-DOPA may produce dopamine-like responses in the absence of dopamine release. Using the AADC inhibitor NSD-1015, we showed that rotation and striatal Fos expression induced by L-DOPA were absent (Lopez et al., 2001), indicating that these effects are not due to a direct action of L-DOPA and are due to its conversion to dopamine. In the same study, we found that the effects of exogenous L-DOPA were blocked by removing serotonergic innervation (Lopez et al., 2001). Interestingly, removal of serotonin afferents or dampening of serotonin activity by 5-HT1A and 5-HT1B agonists blocked LID in rat and primate models (Carta et al., 2007; Muñoz et al., 2008; Fisher et al., 2020). Serotonergic neurons can convert L-DOPA into dopamine, which is stored and released as a “false neurotransmitter.” However, serotonergic terminals are unable to regulate dopamine release due to the lack of regulatory feedback mediated by the dopamine transporter and type-2 dopamine autoreceptors. In this scenario, activation of serotonin autoreceptors by selective agonists reduces dopamine release dampening synaptic dopamine peaks and LID (Carta et al., 2008, 2010). Administration of higher doses of 5-HT1A and 5-HT1B agonists also suppressed apomorphine induced-dyskinesia but by a different mechanism involving the activation of postsynaptic 5HT1-receptors expressed in non-serotonergic neurons in different brain areas (Muñoz et al., 2009). Other studies provided further support about the key role of the serotonin in LID. Recent studies showed a selective regulation of 5-HT1B serotonin receptor mRNA expression by L-DOPA treatment (Padovan-Neto et al., 2020), and dyskinetic monkeys and patients showed sprouting of serotonin terminals and increase in SERT levels (Rylander et al., 2010; Beaudoin-Gobert et al., 2018; Walker et al., 2019). BDNF overexpression increased the susceptibility to LID due to serotonin hyperinnervation (Tronci et al., 2017), and other recent studies further supported the role of BDNF in LID (Sanna et al., 2020). In addition to DA, other metabolic products released by the serotonin neurons such as trace amines, may also be involved in L-DOPA effects acting as “false neurotransmitters” (Chagraoui et al., 2019). The interaction between the serotonin system and L-DOPA is thought to be more relevant at terminal level, rather than at the somatic level, because no changes in serotonin neuron somas or serotonin levels were observed in the DRN of dyskinetic rats (Rylander et al., 2010; Bishop et al., 2012).
Compounds acting through the serotonin system such as anpirtoline, (Bézard et al., 2013) or eltoprazine (Ghiglieri et al., 2016), which are a dual 1A/1B affinity 5HT agonist, or 5-HT2A antagonists (Meco et al., 2003; Frouni et al., 2019; Kwan et al., 2019) showed beneficial effects against LID. LIDs are accompanied by impairment in corticostriatal bidirectional synaptic plasticity (Picconi et al., 2003), and eltoprazine reduces LIDs by the regulation of long-term potentiation and synaptic depotentiation in striatal neurons (Ghiglieri et al., 2016). The role of SERT is also being explored as a possible target against LID, and SERT blockade with SSRIs is also effective. However, data from non- human primates treated with some of these drugs also led to worsening of parkinsonian symptoms. However, opposite results were also observed (Bishop et al., 2012; Conti et al., 2014; Fidalgo et al., 2015; Lanza and Bishop, 2018). Recently, Vilazodone, a selective SSRI, and a partial 5-HT1A agonist have been shown to reduce LID without compromising L-DOPA efficacy (Meadows et al., 2018).
Clinical trials with serotonergic drugs are ongoing, revealing the promising antidyskinetic effects of 5HT1A agonists such as buspirone (Politis et al., 2014), sarizotan (Bara-Jimenez et al., 2005; Goetz et al., 2007), and tandospirone (Kannari et al., 2002). However, these drugs, at high doses, may interfere therapeutic effects of L-DOPA, due to the presence of the autoreceptors in non-serotonergic neurons and possible antagonistic action on dopaminergic receptors. Eltoprazine could provide effective suppression of LID and a wider therapeutic window (Svenningsson et al., 2015; Frouni et al., 2019; Wang Q. et al., 2019). Recently, compounds acting through 5-HT3 receptors also reduced LID (Kwan et al., 2020). However, the pathophysiology of dyskinesia is complex as the glutamatergic system is also involved. An interesting possibility is to combine 5HT1 agonists with drugs that modulate the glutamatergic function (Tison et al., 2013; Carta and Björklund, 2018). Neuroinflammation and angiogenesis are also involved in the development of dyskinesia and are also therapeutic targets (Muñoz et al., 2014; Bishop, 2019; Boi et al., 2019).
Involvement of Serotonin in Graft-Induced Dyskinesias
Clinical trials using transplants of fetal dopamine neuroblasts have shown promising results, although many patients have developed GID (Freed et al., 2001; Olanow et al., 2009; Bjorklund and Kordower, 2013; Li et al., 2016; Barker, 2019). The mechanism underlying GID is still unclear (Freed et al., 2001; Hagell et al., 2002; Barker and Kuan, 2010). Serotonergic neurons usually present in the grafted cell suspension contribute to serotonergic innervation of the ventral mesencephalic grafts and the surrounding striatum (Guerra et al., 1997). It has been suggested that the inclusion of serotonergic neurons in the grafted ventral midbrain tissue may lead to the development of GID (Politis and Loane, 2011; Shin et al., 2012b). Experimental studies using different proportions of dopamine and serotonin neurons in the grafted cell suspension showed that the increase in the number of serotonin neurons within the transplant led to progressive worsening of dyskinesia, and the relative density of dopamine and serotonin innervation in the grafted striatum appears as a critical factor, even more than the absolute number of serotonin neurons within the grafts (Carlsson et al., 2007, 2009). In PD patients with GID, grafted tissue contained a large number of serotonergic neurons and excessive graft-derived serotonergic innervation (Politis et al., 2011; Tronci et al., 2015). Moreover, the serotonin 5-HT1A receptor agonist buspirone produced significant dampening of GID in grafted patients. However, this effect could also be explained by the dopamine D2 receptor partial antagonistic effects of the drug (Politis et al., 2010b, 2011; Shin et al., 2012a), and the long term effect of this compound is uncertain (Beaulieu-Boire and Fasano, 2015). Removal of the endogenous serotonin innervation abolished the anti-GID properties of the 5-HT1A and 5-HT1B agonists, suggesting that the effect of these drugs on GID is mediated by the activation of presynaptic host-derived receptors (Shin et al., 2012a). Nevertheless, dopamine receptor blockade in fetal mesencephalic grafts induces a striking enhancement of the antidyskinetic effect suggesting that both serotonergic and dopaminergic mechanisms may interact in the development of GID (Shin et al., 2014). Controversial data have shown high striatal 5-HT transporter content in the absence of graft-induced dyskinesia (Mendez et al., 2005; Lane, 2019). Studies using a new experimental model, in which the activity of the transplanted dopaminergic neurons can be selectively modulated using a bimodal chemogenetic approach (DREADD), revealed a novel dyskinesia mechanism mediated by the serotonin 5-HT6 receptors (Aldrin-Kirk et al., 2016). The next step should be to evaluate the impact of L-DOPA therapy on grafts from new cell sources, particularly human embryonic and induced pluripotential stem cells that will be used in upcoming clinical trials (Kirkeby et al., 2017; Studer, 2017; Table 1).
Concluding Remarks
Interactions between serotonergic and other neurotransmitter systems reveal that serotonin plays a crucial role in the control of movement by the basal ganglia. These interactions are of great interest for understanding the pathophysiology of PD and to develop novel therapeutic strategies. Manipulation of the serotonergic system represents a valuable target to treat LID and GID in PD patients. However, further investigation is required to clarify mechanisms of neurotransmitter interactions and to determine optimal compounds and doses for effective therapies.
Author Contributions
All authors have contributed to this work and approved its final version for submission. AM developed the idea for this review and wrote the manuscript. AL-L, CL, and JL-G prepared the figure and were involved in the literature review and preparation and revision of the manuscript.
Funding
This work was supported by Spanish Ministry of Economy and Competitiveness (Ministerio de Ciencia y Tecnología; RTI2018-098830-B-I00); Spanish Ministry of Health (RD16/0011/0016 and Consellería de Cultura, Educación e Ordenación Universitaria, Xunta de Galicia; CIBERNED); Galician Government (XUGA, ED431C 2018/10, ED431G/05); and FEDER (Regional European Development Fund).
Conflict of Interest
The authors declare that the research was conducted in the absence of any commercial or financial relationships that could be construed as a potential conflict of interest.
References
Aldrin-Kirk, P., Heuer, A., Wang, G., Mattsson, B., Lundblad, M., Parmar, M., et al. (2016). DREADD modulation of transplanted DA neurons reveals a novel Parkinsonian dyskinesia mechanism mediated by the serotonin 5-HT6 receptor. Neuron 90, 955–968. doi: 10.1016/j.neuron.2016.04.017
Arai, R., Karasawa, N., Geffard, M., Nagatsu, T., and Nagatsu, I. (1994). Immunohistochemical evidence that central serotonin neurons produce dopamine from exogenous L-DOPA in the rat, with reference to the involvement of aromatic L-amino acid decarboxylase. Brain Res. 667, 295–299. doi: 10.1016/0006-8993(94)91511-3
Arborelius, L., Nomikos, G. G., Hacksell, U., and Svensson, T. H. (1993). (R)-8-OH-DPAT preferentially increases dopamine release in rat medial prefrontal cortex. Acta Physiol. Scand. 148, 465–466. doi: 10.1111/j.1748-1716.1993.tb09584.x
Aston-Jones, G. S. M., and Grzanna, R. (1995). The Locus Coeruleus, A5 and A7 Noradrenergic Cell Groups. New York, NY: Academic Press.
Avale, M. E., Nemirovsky, S. I., Raisman-Vozari, R., and Rubinstein, M. (2004). Elevated serotonin is involved in hyperactivity but not in the paradoxical effect of amphetamine in mice neonatally lesioned with 6-hydroxydopamine. J. Neurosci. Res. 78, 289–296. doi: 10.1002/jnr.20245
Ballanger, B., Klinger, H., Eche, J., Lerond, J., Vallet, A. E., Le Bars, D., et al. (2012). Role of serotonergic 1A receptor dysfunction in depression associated with Parkinson’s disease. Mov. Disord. 27, 84–89. doi: 10.1002/mds.23895
Bara-Jimenez, W., Bibbiani, F., Morris, M. J., Dimitrova, T., Sherzai, A., Mouradian, M. M., et al. (2005). Effects of serotonin 5-HT1A agonist in advanced Parkinson’s disease. Mov. Disord. 20, 932–936. doi: 10.1002/mds.20370
Barker, R. A. (2019). Designing stem-cell-based dopamine cell replacement trials for Parkinson’s disease. Nat. Med. 25, 1045–1053. doi: 10.1038/s41591-019-0507-2
Barker, R. A., and Kuan, W. L. (2010). Graft-induced dyskinesias in Parkinson’s disease: what is it all about? Cell Stem Cell 7, 148–149. doi: 10.1016/j.stem.2010.07.003
Beaudoin-Gobert, M., Météreau, E., Duperrier, S., Thobois, S., Tremblay, L., and Sgambato, V. (2018). Pathophysiology of levodopa-induced dyskinesia: insights from multimodal imaging and immunohistochemistry in non-human primates. NeuroImage 183, 132–141. doi: 10.1016/j.neuroimage.2018.08.016
Beaulieu-Boire, I., and Fasano, A. (2015). Graft-induced dyskinesias fail to respond to 5HT1A agonist in the long-term. Mov. Disord. 30, 872–873. doi: 10.1002/mds.26180
Bézard, E., Muñoz, A., Tronci, E., Pioli, E. Y., Li, Q., Porras, G., et al. (2013). Anti-dyskinetic effect of anpirtoline in animal models of L-DOPA-induced dyskinesia. Neurosci. Res. 77, 242–246. doi: 10.1016/j.neures.2013.10.002
Bishop, C. (2019). Neuroinflammation: fanning the fire of L-DOPA-induced dyskinesia. Mov. Disord. 34, 1758–1760. doi: 10.1002/mds.27900
Bishop, C., George, J. A., Buchta, W., Goldenberg, A. A., Mohamed, M., Dickinson, S. O., et al. (2012). Serotonin transporter inhibition attenuates l-DOPA-induced dyskinesia without compromising L-DOPA efficacy in hemi-parkinsonian rats. Eur. J. Neurosci. 36, 2839–2848. doi: 10.1111/j.1460-9568.2012.08202.x
Bjorklund, A., and Kordower, J. H. (2013). Cell therapy for Parkinson’s disease: what next? Mov. Disord. 28, 110–115. doi: 10.1002/mds.25343
Boi, L., Pisanu, A., Greig, N. H., Scerba, M. T., Tweedie, D., Mulas, G., et al. (2019). Immunomodulatory drugs alleviate L-DOPA-induced dyskinesia in a rat model of Parkinson’s disease. Mov. Disord. 34, 1818–1830. doi: 10.1002/mds.27799
Browne, C. J., Abela, A. R., Chu, D., Li, Z., Ji, X., Lambe, E. K., et al. (2019). Dorsal raphe serotonin neurons inhibit operant responding for reward via inputs to the ventral tegmental area but not the nucleus accumbens: evidence from studies combining optogenetic stimulation and serotonin reuptake inhibition. Neuropsychopharmacology 44, 793–804. doi: 10.1038/s41386-018-0271-x
Brown, P., and Gerfen, C. R. (2006). Plasticity within striatal direct pathway neurons after neonatal dopamine depletion is mediated through a novel functional coupling of serotonin 5-HT2 receptors to the ERK 1/2 map kinase pathway. J. Comp. Neurol. 498, 415–430. doi: 10.1002/cne.21034
Carlsson, T., Carta, M., Muñoz, A., Mattsson, B., Winkler, C., Kirik, D., et al. (2009). Impact of grafted serotonin and dopamine neurons on development of L-DOPA-induced dyskinesias in parkinsonian rats is determined by the extent of dopamine neuron degeneration. Brain 132, 319–335. doi: 10.1093/brain/awn305
Carlsson, T., Carta, M., Winkler, C., Björklund, A., and Kirik, D. (2007). Serotonin neuron transplants exacerbate L-DOPA-induced dyskinesias in a rat model of Parkinson’s disease. J. Neurosci. 27, 8011–8022. doi: 10.1523/JNEUROSCI.2079-07.2007
Carta, M., and Björklund, A. (2018). The serotonergic system in L-DOPA-induced dyskinesia: pre-clinical evidence and clinical perspective. J. Neural Transm. 125, 1195–1202. doi: 10.1007/s00702-018-1865-5
Carta, M., Carlsson, T., Kirik, D., and Björklund, A. (2007). Dopamine released from 5-HT terminals is the cause of L-DOPA-induced dyskinesia in parkinsonian rats. Brain 130, 1819–1833. doi: 10.1093/brain/awm082
Carta, M., Carlsson, T., Muñoz, A., Kirik, D., and Björklund, A. (2008). Serotonin-dopamine interaction in the induction and maintenance of L-DOPA-induced dyskinesias. Prog. Brain Res. 172, 465–478. doi: 10.1016/s0079-6123(08)00922-9
Carta, M., Carlsson, T., Muñoz, A., Kirik, D., and Björklund, A. (2010). Role of serotonin neurons in the induction of levodopa- and graft-induced dyskinesias in Parkinson’s disease. Mov. Disord. 25, S174–S179. doi: 10.1002/mds.22792
Chagraoui, A., Boulain, M., Juvin, L., Anouar, Y., Barriere, G., and Deurwaerdere, P. (2019). L-DOPA in Parkinson’s disease: looking at the “false” neurotransmitters and their meaning. Int. J. Mol. Sci. 21:E294. doi: 10.3390/ijms21010294
Ciranna, L. (2006). Serotonin as a modulator of glutamate- and GABA-mediated neurotransmission: implications in physiological functions and in pathology. Curr. Neuropharmacol. 4, 101–114. doi: 10.2174/157015906776359540
Conti, M. M., Ostock, C. Y., Lindenbach, D., Goldenberg, A. A., Kampton, E., Dell’isola, R., et al. (2014). Effects of prolonged selective serotonin reuptake inhibition on the development and expression of L-DOPA-induced dyskinesia in hemi-parkinsonian rats. Neuropharmacology 77, 1–8. doi: 10.1016/j.neuropharm.2013.09.017
Dahlström, A., and Fuxe, K. (1964). Localization of monoamines in the lower brain stem. Experientia 20, 398–399. doi: 10.1007/bf02147990
De Deurwaerdère, P., Bharatiya, R., Chagraoui, A., and Di Giovanni, G. (2020). Constitutive activity of 5-HT receptors: factual analysis. Neuropharmacology 168:107967. doi: 10.1016/j.neuropharm.2020.107967
De Deurwaerdère, P., and Di Giovanni, G. (2017). Serotonergic modulation of the activity of mesencephalic dopaminergic systems: therapeutic implications. Prog. Neurobiol. 151, 175–236. doi: 10.1016/j.pneurobio.2016.03.004
Delaville, C., Navailles, S., and Benazzouz, A. (2012). Effects of noradrenaline and serotonin depletions on the neuronal activity of globus pallidus and substantia nigra pars reticulata in experimental parkinsonism. Neuroscience 202, 424–433. doi: 10.1016/j.neuroscience.2011.11.024
Di Matteo, V., Pierucci, M., Esposito, E., Crescimanno, G., Benigno, A., and Di Giovanni, G. (2008). Serotonin modulation of the basal ganglia circuitry: therapeutic implication for Parkinson’s disease and other motor disorders. Prog. Brain Res. 172, 423–463. doi: 10.1016/s0079-6123(08)00921-7
Dupre, K. B., Ostock, C. Y., George, J. A., Eskow Jaunarajs, K. L., Hueston, C. M., and Bishop, C. (2013). Effects of 5-HT1A receptor stimulation on D1 receptor agonist-induced striatonigral activity and dyskinesia in hemiparkinsonian rats. ACS Chem. Neurosci. 4, 747–760. doi: 10.1021/cn300234z
Espay, A. J., Morgante, F., Merola, A., Fasano, A., Marsili, L., Fox, S. H., et al. (2018). Levodopa-induced dyskinesia in Parkinson disease: current and evolving concepts. Ann. Neurol. 84, 797–811. doi: 10.1002/ana.25364
Fidalgo, C., Ko, W. K., Tronci, E., Li, Q., Stancampiano, R., Chuan, Q., et al. (2015). Effect of serotonin transporter blockade on L-DOPA-induced dyskinesia in animal models of Parkinson’s disease. Neuroscience 298, 389–396. doi: 10.1016/j.neuroscience.2015.04.027
Fisher, R., Hikima, A., Morris, R., Jackson, M. J., Rose, S., Varney, M. A., et al. (2020). The selective 5-HT1A receptor agonist, NLX-112, exerts anti-dyskinetic and anti-parkinsonian-like effects in MPTP-treated marmosets. Neuropharmacology 167:107997. doi: 10.1016/j.neuropharm.2020.107997
Fox, S. H., and Brotchie, J. M. (2000). 5-HT2C receptor binding is increased in the substantia nigra pars reticulata in Parkinson’s disease. Mov. Disord. 15, 1064–1069. doi: 10.1002/1531-8257(200011)15:6<1064::aid-mds1002>3.0.co;2-c
Freed, C. R., Greene, P. E., Breeze, R. E., Tsai, W. Y., Dumouchel, W., Kao, R., et al. (2001). Transplantation of embryonic dopamine neurons for severe Parkinson’s disease. N. Engl. J. Med. 344, 710–719. doi: 10.1097/00001756-200105250-00002
Frouni, I., Kwan, C., Bédard, D., Belliveau, S., Bourgeois-Cayer, E., Gaudette, F., et al. (2019). Effect of the selective 5-HT2A receptor antagonist EMD-281,014 on L-DOPA-induced abnormal involuntary movements in the 6-OHDA-lesioned rat. Exp. Brain Res. 237, 29–36. doi: 10.1007/s00221-018-5390-4
Gantz, S. C., Levitt, E. S., Llamosas, N., Neve, K. A., and Williams, J. T. (2015). Depression of serotonin synaptic transmission by the dopamine precursor L-DOPA. Cell Rep. 12, 944–954. doi: 10.1016/j.celrep.2015.07.005
Ghiglieri, V., Mineo, D., Vannelli, A., Cacace, F., Mancini, M., Pendolino, V., et al. (2016). Modulation of serotonergic transmission by eltoprazine in L-DOPA-induced dyskinesia: behavioral, molecular, and synaptic mechanisms. Neurobiol. Dis. 86, 140–153. doi: 10.1016/j.nbd.2015.11.022
Goetz, C. G., Damier, P., Hicking, C., Laska, E., Müller, T., Olanow, C. W., et al. (2007). Sarizotan as a treatment for dyskinesias in Parkinson’s disease: a double-blind placebo-controlled trial. Mov. Disord. 22, 179–186. doi: 10.1002/mds.21226
Guerra, M. J., Liste, I., and Labandeira-Garcia, J. L. (1997). Effects of lesions of the nigrostriatal pathway and of nigral grafts on striatal serotonergic innervation in adult rats. Neuroreport 8, 3485–3488. doi: 10.1097/00001756-199711100-00014
Guerra, M. J., Liste, I., and Labandeira-Garcia, J. L. (1998). Interaction between the serotonergic, dopaminergic and glutamatergic systems in fenfluramine-induced Fos expression in striatal neurons. Synapse 28, 71–82. doi: 10.1002/(sici)1098-2396(199801)28:1<71::aid-syn9>3.0.co;2-9
Guiard, B. P., El Mansari, M., Merali, Z., and Blier, P. (2008). Functional interactions between dopamine, serotonin and norepinephrine neurons: an in vivo electrophysiological study in rats with monoaminergic lesions. Int. J. Neuropsychopharmacol. 11, 625–639. doi: 10.1017/s1461145707008383
Gutknecht, L., Araragi, N., Merker, S., Waider, J., Sommerlandt, F. M., Mlinar, B., et al. (2012). Impacts of brain serotonin deficiency following Tph2 inactivation on development and raphe neuron serotonergic specification. PLoS One 7:e43157. doi: 10.1371/journal.pone.0043157
Hagell, P., Piccini, P., Björklund, A., Brundin, P., Rehncrona, S., Widner, H., et al. (2002). Dyskinesias following neural transplantation in Parkinson’s disease. Nat. Neurosci. 5, 627–628. doi: 10.1038/nn863
Hannon, J., and Hoyer, D. (2008). Molecular biology of 5-HT receptors. Behav. Brain Res. 195, 198–213. doi: 10.1016/j.bbr.2008.03.020
Hensler, J. G., Artigas, F., Bortolozzi, A., Daws, L. C., De Deurwaerdere, P., Milan, L., et al. (2013). Catecholamine/Serotonin interactions: systems thinking for brain function and disease. Adv. Pharmacol. 68, 167–197. doi: 10.1016/B978-0-12-411512-5.00009-9
Hjorth, S., Bengtsson, H. J., Milano, S., Lundberg, J. F., and Sharp, T. (1995). Studies on the role of 5-HT1A autoreceptors and α1-adrenoceptors in the inhibition of 5-HT release—I. BMY7378 and prazosin. Neuropharmacology 34, 615–620. doi: 10.1016/0028-3908(95)00038-8
Hornung, J.-P. (2010). “Chapter 1.3 - The Neuroanatomy of the serotonergic system,” in Handbook of Behavioral Neuroscience, eds C. P. Müller and B. L. Jacobs (Amsterdam, Netherlands: Elsevier), 51–64.
Hoyer, D., Hannon, J. P., and Martin, G. R. (2002). Molecular, pharmacological and functional diversity of 5-HT receptors. Pharmacol. Biochem. Behav. 71, 533–554. doi: 10.1016/s0091-3057(01)00746-8
Huang, K. W., Ochandarena, N. E., Philson, A. C., Hyun, M., Birnbaum, J. E., Cicconet, M., et al. (2019). Molecular and anatomical organization of the dorsal raphe nucleus. Elife 8:e46464. doi: 10.7554/eLife.46464
Huot, P., and Fox, S. H. (2013). The serotonergic system in motor and non-motor manifestations of Parkinson’s disease. Exp. Brain Res. 230, 463–476. doi: 10.1007/s00221-013-3621-2
Huot, P., Fox, S. H., and Brotchie, J. M. (2011). The serotonergic system in Parkinson’s disease. Prog. Neurobiol. 95, 163–212. doi: 10.1016/j.pneurobio.2011.08.004
Jenner, P. (2018). The treatment of levodopa-induced dyskinesias: surfing the serotoninergic wave. Mov. Disord. 33, 1670–1672. doi: 10.1002/mds.27525
Kannari, K., Kurahashi, K., Tomiyama, M., Maeda, T., Arai, A., Baba, M., et al. (2002). Tandospirone citrate, a selective 5-HT1A agonist, alleviates L-DOPA-induced dyskinesia in patients with Parkinson’s disease. No To Shinkei 54, 133–137.
Karstaedt, P. J., Kerasidis, H., Pincus, J. H., Meloni, R., Graham, J., and Gale, K. (1994). Unilateral destruction of dopamine pathways increases ipsilateral striatal serotonin turnover in rats. Exp. Neurol. 126, 25–30. doi: 10.1006/exnr.1994.1039
Kaya, A. H., Vlamings, R., Tan, S., Lim, L. W., Magill, P. J., Steinbusch, H. W., et al. (2008). Increased electrical and metabolic activity in the dorsal raphe nucleus of Parkinsonian rats. Brain Res. 1221, 93–97. doi: 10.1016/j.brainres.2008.05.019
Kelland, M. D., Freeman, A. S., and Chiodo, L. A. (1990). Serotonergic afferent regulation of the basic physiology and pharmacological responsiveness of nigrostriatal dopamine neurons. J. Pharmacol. Exp. Ther. 253, 803–811.
Kirkeby, A., Parmar, M., and Barker, R. A. (2017). Strategies for bringing stem cell-derived dopamine neurons to the clinic: a European approach (STEM-PD). Prog. Brain Res. 230, 165–190. doi: 10.1016/bs.pbr.2016.11.011
Koch, J. C., Tatenhorst, L., Roser, A. E., Saal, K. A., Tönges, L., and Lingor, P. (2018). ROCK inhibition in models of neurodegeneration and its potential for clinical translation. Pharmacol. Ther. 189, 1–21. doi: 10.1016/j.pharmthera.2018.03.008
Kwan, C., Frouni, I., Bédard, D., Hamadjida, A., and Huot, P. (2020). Ondansetron, a highly selective 5-HT3 receptor antagonist, reduces L-DOPA-induced dyskinesia in the 6-OHDA-lesioned rat model of Parkinson’s disease. Eur. J. Pharmacol. 871:172914. doi: 10.1016/j.ejphar.2020.172914
Kwan, C., Frouni, I., Bedard, D., Nuara, S. G., Gourdon, J. C., Hamadjida, A., et al. (2019). 5-HT2A blockade for dyskinesia and psychosis in Parkinson’s disease: is there a limit to the efficacy of this approach? A study in the MPTP-lesioned marmoset and a literature mini-review. Exp. Brain Res. 237, 435–442. doi: 10.1007/s00221-018-5434-9
Labandeira-Garcia, J. L., Rodríguez-Perez, A. I., Villar-Cheda, B., Borrajo, A., Dominguez-Meijide, A., and Guerra, M. J. (2015). Rho kinase and dopaminergic degeneration: a promising therapeutic target for Parkinson’s disease. Neuroscientist 21, 616–629. doi: 10.1177/1073858414554954
Lane, E. L. (2019). L-DOPA for Parkinson’s disease-a bittersweet pill. Eur. J. Neurosci. 49, 384–398. doi: 10.1111/ejn.14119
Lanza, K., and Bishop, C. (2018). Serotonergic targets for the treatment of L-DOPA-induced dyskinesia. J. Neural Transm. 125, 1203–1216. doi: 10.1007/s00702-017-1837-1
Lauder, J. M. (1990). Ontogeny of the serotonergic system in the rat: serotonin as a developmental signal. Ann. N Y Acad. Sci. 600, 297–313; discussion 314. doi: 10.1111/j.1749-6632.1990.tb16891.x
Lavoie, B., and Parent, A. (1990). Immunohistochemical study of the serotoninergic innervation of the basal ganglia in the squirrel monkey. J. Comp. Neurol. 299, 1–16. doi: 10.1002/cne.902990102
Li, W., Englund, E., Widner, H., Mattsson, B., van Westen, D., Lätt, J., et al. (2016). Extensive graft-derived dopaminergic innervation is maintained 24 years after transplantation in the degenerating parkinsonian brain. Proc. Natl. Acad. Sci. U S A 113, 6544–6549. doi: 10.1073/pnas.1605245113
Li, X. M., Juorio, A. V., Paterson, I. A., Walz, W., Zhu, M. Y., and Boulton, A. A. (1992). Gene expression of aromatic L-amino acid decarboxylase in cultured rat glial cells. J. Neurochem. 59, 1172–1175. doi: 10.1111/j.1471-4159.1992.tb08363.x
Liste, I., Muñoz, A., Guerra, M. J., and Labandeira-Garcia, J. L. (2000). Fenfluramine-induced increase in preproenkephalin mRNA levels in the striatum: interaction between the serotonergic, glutamatergic and dopaminergic systems. Synapse 35, 182–191. doi: 10.1002/(sici)1098-2396(20000301)35:3<182::aid-syn3>3.0.co;2-t
Lopez, A., Muñoz, A., Guerra, M. J., and Labandeira-Garcia, J. L. (2001). Mechanisms of the effects of exogenous levodopa on the dopamine-denervated striatum. Neuroscience 103, 639–651. doi: 10.1016/s0306-4522(00)00588-1
Lopez-Real, A., Rodriguez-Pallares, J., Guerra, M. J., and Labandeira-Garcia, J. L. (2003). Localization and functional significance of striatal neurons immunoreactive to aromatic L-amino acid decarboxylase or tyrosine hydroxylase in rat Parkinsonian models. Brain Res. 969, 135–146. doi: 10.1016/s0006-8993(03)02291-1
Maeda, T., Kannari, K., Shen, H., Arai, A., Tomiyama, M., Matsunaga, M., et al. (2003). Rapid induction of serotonergic hyperinnervation in the adult rat striatum with extensive dopaminergic denervation. Neurosci. Lett. 343, 17–20. doi: 10.1016/s0304-3940(03)00295-7
Maeda, T., Nagata, K., Yoshida, Y., and Kannari, K. (2005). Serotonergic hyperinnervation into the dopaminergic denervated striatum compensates for dopamine conversion from exogenously administered l-DOPA. Brain Res. 1046, 230–233. doi: 10.1016/j.brainres.2005.04.019
Mair, K. M., MacLean, M. R., Morecroft, I., Dempsie, Y., and Palmer, T. M. (2008). Novel interactions between the 5-HT transporter, 5-HT1B receptors and Rho kinase in vivo and in pulmonary fibroblasts. Br. J. Pharmacol. 155, 606–616. doi: 10.1038/bjp.2008.310
Masana, M., Santana, N., Artigas, F., and Bortolozzi, A. (2012). Dopamine neurotransmission and atypical antipsychotics in prefrontal cortex: a critical review. Curr. Top. Med. Chem. 12, 2357–2374. doi: 10.2174/1568026611212210008
McDevitt, R. A., Tiran-Cappello, A., Shen, H., Balderas, I., Britt, J. P., Marino, R. A. M., et al. (2014). Serotonergic versus nonserotonergic dorsal raphe projection neurons: differential participation in reward circuitry. Cell Rep. 8, 1857–1869. doi: 10.1016/j.celrep.2014.08.037
Meadows, S. M., Conti, M. M., Gross, L., Chambers, N. E., Avnor, Y., Ostock, C. Y., et al. (2018). Diverse serotonin actions of vilazodone reduce l-3,4-dihidroxyphenylalanine-induced dyskinesia in hemi-parkinsonian rats. Mov. Disord. 33, 1740–1749. doi: 10.1002/mds.100
Meco, G., Fabrizio, E., Di Rezze, S., Alessandri, A., and Pratesi, L. (2003). Mirtazapine in L-dopa-induced dyskinesias. Clin. Neuropharmacol. 26, 179–181. doi: 10.1097/00002826-200307000-00005
Melamed, E., Hefti, F., and Wurtman, R. J. (1980). Decarboxylation of exogenous L-DOPA in rat striatum after lesions of the dopaminergic nigrostriatal neurons: the role of striatal capillaries. Brain Res. 198, 244–248. doi: 10.1016/0006-8993(80)90363-7
Meloni, M., Puligheddu, M., Carta, M., Cannas, A., Figorilli, M., and Defazio, G. (2020). Efficacy and safety of the 5-Hydroxytryptophan on depression and apathy in Parkinson’s disease: a preliminary finding. Eur. J. Neurol. 27, 779–786. doi: 10.1111/ene.14179
Mendez, I., Sanchez-Pernaute, R., Cooper, O., Viñuela, A., Ferrari, D., Bjorklund, L., et al. (2005). Cell type analysis of functional fetal dopamine cell suspension transplants in the striatum and substantia nigra of patients with Parkinson’s disease. Brain 128, 1498–1510. doi: 10.1093/brain/awh510
Miguelez, C., Berrocoso, E., Mico, J. A., and Ugedo, L. (2013). L-DOPA modifies the antidepressant-like effects of reboxetine and fluoxetine in rats. Neuropharmacology 67, 349–358. doi: 10.1016/j.neuropharm.2012.11.016
Miguelez, C., Grandoso, L., and Ugedo, L. (2011). Locus coeruleus and dorsal raphe neuron activity and response to acute antidepressant administration in a rat model of Parkinson’s disease. Int. J. Neuropsychopharmacol. 14, 187–200. doi: 10.1017/s146114571000043x
Miguelez, C., Morera-Herreras, T., Torrecilla, M., Ruiz-Ortega, J. A., and Ugedo, L. (2014). Interaction between the 5-HT system and the basal ganglia: functional implication and therapeutic perspective in Parkinson’s disease. Front. Neural Circuits 8:21. doi: 10.3389/fncir.2014.00021
Miguelez, C., Navailles, S., De Deurwaerdère, P., and Ugedo, L. (2016). The acute and long-term L-DOPA effects are independent from changes in the activity of dorsal raphe serotonergic neurons in 6-OHDA lesioned rats. Br. J. Pharmacol. 173, 2135–2146. doi: 10.1111/bph.13447
Morelli, E., Moore, H., Rebello, T. J., Gray, N., Steele, K., Esposito, E., et al. (2011). Chronic 5-HT transporter blockade reduces DA signaling to elicit basal ganglia dysfunction. J. Neurosci. 31, 15742–15750. doi: 10.1523/JNEUROSCI.2989-11.2011
Mosienko, V., Beis, D., Pasqualetti, M., Waider, J., Matthes, S., Qadri, F., et al. (2015). Life without brain serotonin: reevaluation of serotonin function with mice deficient in brain serotonin synthesis. Behav. Brain Res. 277, 78–88. doi: 10.1016/j.bbr.2014.06.005
Muñoz, A., Carlsson, T., Tronci, E., Kirik, D., Björklund, A., and Carta, M. (2009). Serotonin neuron-dependent and -independent reduction of dyskinesia by 5-HT1A and 5-HT1B receptor agonists in the rat Parkinson model. Exp. Neurol. 219, 298–307. doi: 10.1016/j.expneurol.2009.05.033
Muñoz, A., Garrido-Gil, P., Dominguez-Meijide, A., and Labandeira-Garcia, J. L. (2014). Angiotensin type 1 receptor blockage reduces l-dopa-induced dyskinesia in the 6-OHDA model of Parkinson’s disease. Involvement of vascular endothelial growth factor and interleukin-1β. Exp. Neurol. 261, 720–732. doi: 10.1016/j.expneurol.2014.08.019
Muñoz, A., Li, Q., Gardoni, F., Marcello, E., Qin, C., Carlsson, T., et al. (2008). Combined 5-HT1A and 5-HT1B receptor agonists for the treatment of L-DOPA-induced dyskinesia. Brain 131, 3380–3394. doi: 10.1093/brain/awn235
Muñoz, A., Lopez-Real, A., Labandeira-Garcia, J. L., and Guerra, M. J. (2003). Interaction between the noradrenergic and serotonergic systems in locomotor hyperactivity and striatal expression of Fos induced by amphetamine in rats. Exp. Brain Res. 153, 92–99. doi: 10.1007/s00221-003-1582-6
Mura, A., Jackson, D., Manley, M. S., Young, S. J., and Groves, P. M. (1995). Aromatic L-amino acid decarboxylase immunoreactive cells in the rat striatum: a possible site for the conversion of exogenous L-DOPA to dopamine. Brain Res. 704, 51–60. doi: 10.1016/0006-8993(95)01104-8
Muzerelle, A., Scotto-Lomassese, S., Bernard, J. F., Soiza-Reilly, M., and Gaspar, P. (2016). Conditional anterograde tracing reveals distinct targeting of individual serotonin cell groups (B5–B9) to the forebrain and brainstem. Brain Struct. Funct. 221, 535–561. doi: 10.1007/s00429-014-0924-4
Navailles, S., and De Deurwaerdere, P. (2012). Contribution of serotonergic transmission to the motor and cognitive effects of high-frequency stimulation of the subthalamic nucleus or levodopa in Parkinson’s disease. Mol. Neurobiol. 45, 173–185. doi: 10.1007/s12035-011-8230-0
Ogawa, S. K., and Watabe-Uchida, M. (2018). Organization of dopamine and serotonin system: anatomical and functional mapping of monosynaptic inputs using rabies virus. Pharmacol. Biochem. Behav. 174, 9–22. doi: 10.1016/j.pbb.2017.05.001
Olanow, C. W., Gracies, J. M., Goetz, C. G., Stoessl, A. J., Freeman, T., Kordower, J. H., et al. (2009). Clinical pattern and risk factors for dyskinesias following fetal nigral transplantation in Parkinson’s disease: a double blind video-based analysis. Mov. Disord. 24, 336–343. doi: 10.1002/mds.22208
Padovan-Neto, F. E., Patterson, S., Nm, F. V., Altwal, F., Beverley, J. A., West, A. R., et al. (2020). Selective regulation of 5-HT1B serotonin receptor expression in the striatum by dopamine depletion and repeated L-DOPA treatment: relationship to L-DOPA-induced dyskinesias. Mol. Neurobiol. 57, 736–751. doi: 10.1007/s12035-019-01739-x
Parent, M., Wallman, M. J., Gagnon, D., and Parent, A. (2011). Serotonin innervation of basal ganglia in monkeys and humans. J. Chem. Neuroanat. 41, 256–265. doi: 10.1016/j.jchemneu.2011.04.005
Parga, J., Rodriguez-Pallares, J., Muñoz, A., Guerra, M. J., and Labandeira-Garcia, J. L. (2007). Serotonin decreases generation of dopaminergic neurons from mesencephalic precursors via serotonin type 7 and type 4 receptors. Dev. Neurobiol. 67, 10–22. doi: 10.1002/neu.20306
Paulus, W., and Jellinger, K. (1991). The neuropathologic basis of different clinical subgroups of Parkinson’s disease. J. Neuropathol. Exp. Neurol. 50, 743–755. doi: 10.1097/00005072-199111000-00006
Picconi, B., Centonze, D., Håkansson, K., Bernardi, G., Greengard, P., Fisone, G., et al. (2003). Loss of bidirectional striatal synaptic plasticity in L-DOPA-induced dyskinesia. Nat. Neurosci. 6, 501–506. doi: 10.1038/nn1040
Politis, M., and Loane, C. (2011). Serotonergic dysfunction in Parkinson’s disease and its relevance to disability. ScientificWorldJournal 11, 1726–1734. doi: 10.1100/2011/172893
Politis, M., Oertel, W. H., Wu, K., Quinn, N. P., Pogarell, O., Brooks, D. J., et al. (2011). Graft-induced dyskinesias in Parkinson’s disease: high striatal serotonin/dopamine transporter ratio. Mov. Disord. 26, 1997–2003. doi: 10.1002/mds.23743
Politis, M., Wu, K., Loane, C., Brooks, D. J., Kiferle, L., Turkheimer, F. E., et al. (2014). Serotonergic mechanisms responsible for levodopa-induced dyskinesias in Parkinson’s disease patients. J. Clin. Invest. 124, 1340–1349. doi: 10.1172/JCI71640
Politis, M., Wu, K., Loane, C., Kiferle, L., Molloy, S., Brooks, D. J., et al. (2010a). Staging of serotonergic dysfunction in Parkinson’s disease: an in vivo 11C-DASB PET study. Neurobiol. Dis. 40, 216–221. doi: 10.1016/j.nbd.2010.05.028
Politis, M., Wu, K., Loane, C., Quinn, N. P., Brooks, D. J., Rehncrona, S., et al. (2010b). Serotonergic neurons mediate dyskinesia side effects in Parkinson’s patients with neural transplants. Sci. Transl. Med. 2:38ra46. doi: 10.1126/scitranslmed.3000976
Pratelli, M., and Pasqualetti, M. (2019). Serotonergic neurotransmission manipulation for the understanding of brain development and function: learning from Tph2 genetic models. Biochimie 161, 3–14. doi: 10.1016/j.biochi.2018.11.016
Prinz, A., Selesnew, L. M., Liss, B., Roeper, J., and Carlsson, T. (2013). Increased excitability in serotonin neurons in the dorsal raphe nucleus in the 6-OHDA mouse model of Parkinson’s disease. Exp. Neurol. 248, 236–245. doi: 10.1016/j.expneurol.2013.06.015
Qi, J., Zhang, S., Wang, H. L., Wang, H., de Jesus Aceves Buendia, J., Hoffman, A. F., et al. (2014). A glutamatergic reward input from the dorsal raphe to ventral tegmental area dopamine neurons. Nat. Commun. 5:5390. doi: 10.1038/ncomms6390
Rea, K., Folgering, J., Westerink, B. H., and Cremers, T. I. (2010). α1-adrenoceptors modulate citalopram-induced serotonin release. Neuropharmacology 58, 962–971. doi: 10.1016/j.neuropharm.2009.12.018
Rodriguez-Pallares, J., Guerra, M. J., and Labandeira-Garcia, J. L. (2003). Elimination of serotonergic cells induces a marked increase in generation of dopaminergic neurons from mesencephalic precursors. Eur. J. Neurosci. 18, 2166–2174. doi: 10.1046/j.1460-9568.2003.02949.x
Rouquier, L., Claustre, Y., and Benavides, J. (1994). α 1-adrenoceptor antagonists differentially control serotonin release in the hippocampus and striatum: a microdialysis study. Eur. J. Pharmacol. 261, 59–64. doi: 10.1016/0014-2999(94)90300-x
Rozas, G., Liste, I., Guerra, M. J., and Labandeira-Garcia, J. L. (1998). Sprouting of the serotonergic afferents into striatum after selective lesion of the dopaminergic system by MPTP in adult mice. Neurosci. Lett. 245, 151–154. doi: 10.1016/s0304-3940(98)00198-0
Rylander, D., Parent, M., O’Sullivan, S. S., Dovero, S., Lees, A. J., Bezard, E., et al. (2010). Maladaptive plasticity of serotonin axon terminals in levodopa-induced dyskinesia. Ann. Neurol. 68, 619–628. doi: 10.1002/ana.22097
Sanna, A., Follesa, P., Puligheddu, M., Cannas, A., Serra, M., Pisu, M. G., et al. (2020). Cerebellar continuous theta burst stimulation reduces levodopa-induced dyskinesias and decreases serum BDNF levels. Neurosci. Lett. 716:134653. doi: 10.1016/j.neulet.2019.134653
Shin, E., Garcia, J., Winkler, C., Björklund, A., and Carta, M. (2012a). Serotonergic and dopaminergic mechanisms in graft-induced dyskinesia in a rat model of Parkinson’s disease. Neurobiol. Dis. 47, 393–406. doi: 10.1016/j.nbd.2012.03.038
Shin, E., Tronci, E., and Carta, M. (2012b). Role of serotonin neurons in L-DOPA- and graft-induced dyskinesia in a rat model of Parkinson’s disease. Parkinsons Dis. 2012:370190. doi: 10.1155/2012/370190
Shin, E., Lisci, C., Tronci, E., Fidalgo, C., Stancampiano, R., Bjorklund, A., et al. (2014). The anti-dyskinetic effect of dopamine receptor blockade is enhanced in parkinsonian rats following dopamine neuron transplantation. Neurobiol. Dis. 62, 233–240. doi: 10.1016/j.nbd.2013.09.021
Silva, T. P., Poli, A., Hara, D. B., and Takahashi, R. N. (2016). Time course study of microglial and behavioral alterations induced by 6-hydroxydopamine in rats. Neurosci. Lett. 622, 83–87. doi: 10.1016/j.neulet.2016.04.049
Sinton, C. M., and Fallon, S. L. (1988). Electrophysiological evidence for a functional differentiation between subtypes of the 5-HT1 receptor. Eur. J. Pharmacol. 157, 173–181. doi: 10.1016/0014-2999(88)90380-9
Snyder, A. M., Zigmond, M. J., and Lund, R. D. (1986). Sprouting of serotoninergic afferents into striatum after dopamine-depleting lesions in infant rats: a retrograde transport and immunocytochemical study. J. Comp. Neurol. 245, 274–281. doi: 10.1002/cne.902450209
Stachowiak, M. K., Bruno, J. P., Snyder, A. M., Stricker, E. M., and Zigmond, M. J. (1984). Apparent sprouting of striatal serotonergic terminals after dopamine-depleting brain lesions in neonatal rats. Brain Res. 291, 164–167. doi: 10.1016/0006-8993(84)90665-6
Studer, L. (2017). Strategies for bringing stem cell-derived dopamine neurons to the clinic-The NYSTEM trial. Prog. Brain Res. 230, 191–212. doi: 10.1016/bs.pbr.2017.02.008
Svenningsson, P., Rosenblad, C., Af Edholm Arvidsson, K., Wictorin, K., Keywood, C., Shankar, B., et al. (2015). Eltoprazine counteracts L-DOPA-induced dyskinesias in Parkinson’s disease: a dose-finding study. Brain 138, 963–973. doi: 10.1093/brain/awu409
Tanaka, T., Doe, J. M., Horstmann, S. A., Ahmad, S., Ahmad, A., Min, S. J., et al. (2014). Neuroendocrine signaling via the serotonin transporter regulates clearance of apoptotic cells. J. Biol. Chem. 289, 10466–10475. doi: 10.1074/jbc.m113.482299
Tison, F., Nègre-Pages, L., Meissner, W. G., Dupouy, S., Li, Q., Thiolat, M. L., et al. (2013). Simvastatin decreases levodopa-induced dyskinesia in monkeys, but not in a randomized, placebo-controlled, multiple cross-over (“n-of-1”) exploratory trial of simvastatin against levodopa-induced dyskinesia in Parkinson’s disease patients. Parkinsonism Relat. Disord 19, 416–421. doi: 10.1016/j.parkreldis.2012.12.003
Towle, A. C., Criswell, H. E., Maynard, E. H., Lauder, J. M., Joh, T. H., Mueller, R. A., et al. (1989). Serotonergic innervation of the rat caudate following a neonatal 6-hydroxydopamine lesion: an anatomical, biochemical and pharmacological study. Pharmacol. Biochem. Behav. 34, 367–374. doi: 10.1016/0091-3057(89)90328-6
Tronci, E., Fidalgo, C., and Carta, M. (2015). Foetal cell transplantation for Parkinson’s disease: focus on graft-induced dyskinesia. Parkinsons Dis. 2015:563820. doi: 10.1155/2015/563820
Tronci, E., Napolitano, F., Muñoz, A., Fidalgo, C., Rossi, F., Björklund, A., et al. (2017). BDNF over-expression induces striatal serotonin fiber sprouting and increases the susceptibility to l-DOPA-induced dyskinesia in 6-OHDA-lesioned rats. Exp. Neurol. 297, 73–81. doi: 10.1016/j.expneurol.2017.07.017
Vermeiren, Y., and De Deyn, P. P. (2017). Targeting the norepinephrinergic system in Parkinson’s disease and related disorders: The locus coeruleus story. Neurochem. Int. 102, 22–32. doi: 10.1016/j.neuint.2016.11.009
Vermeiren, Y., Janssens, J., Van Dam, D., and De Deyn, P. P. (2018). Serotonergic dysfunction in amyotrophic lateral sclerosis and Parkinson’s disease: similar mechanisms, dissimilar outcomes. Front. Neurosci. 12:185. doi: 10.3389/fnins.2018.00185
Walker, M., Kuebler, L., Goehring, C. M., Pichler, B. J., and Herfert, K. (2019). Imaging SERT availability in a rat model of L-DOPA-induced dyskinesia. Mol. Imaging Biol. doi: 10.1007/s11307-019-01418-2 [Epub ahead of print].
Wang, Q., Chen, J., Li, M., Lv, S., Xie, Z., Li, N., et al. (2019). Eltoprazine prevents levodopa-induced dyskinesias by reducing causal interactions for theta oscillations in the dorsolateral striatum and substantia nigra pars reticulate. Neuropharmacology 148, 1–10. doi: 10.1016/j.neuropharm.2018.12.027
Wang, H. L., Zhang, S., Qi, J., Wang, H., Cachope, R., Mejias-Aponte, C. A., et al. (2019). Dorsal raphe dual serotonin-glutamate neurons drive reward by establishing excitatory synapses on VTA mesoaccumbens dopamine neurons. Cell Rep. 26, 1128.e7–1142.e7. doi: 10.1016/j.celrep.2019.01.014
Wang, S., Zhang, Q. J., Liu, J., Wu, Z. H., Wang, T., Gui, Z. H., et al. (2009). Unilateral lesion of the nigrostriatal pathway induces an increase of neuronal firing of the midbrain raphe nuclei 5-HT neurons and a decrease of their response to 5-HT1A receptor stimulation in the rat. Neuroscience 159, 850–861. doi: 10.1016/j.neuroscience.2008.12.051
Zhang, Q. J., Gao, R., Liu, J., Liu, Y. P., and Wang, S. (2007). Changes in the firing activity of serotonergic neurons in the dorsal raphe nucleus in a rat model of Parkinson’s disease. Sheng Li Xue Bao 59, 183–189.
Zhou, F. C., Bledsoe, S., and Murphy, J. (1991). Serotonergic sprouting is induced by dopamine-lesion in substantia nigra of adult rat brain. Brain Res. 556, 108–116. doi: 10.1016/0006-8993(91)90553-8
Keywords: dopamine, dyskinesia, glutamate, Levodopa, Parkinson, serotonin, striatum
Citation: Muñoz A, Lopez-Lopez A, Labandeira CM and Labandeira-Garcia JL (2020) Interactions Between the Serotonergic and Other Neurotransmitter Systems in the Basal Ganglia: Role in Parkinson’s Disease and Adverse Effects of L-DOPA. Front. Neuroanat. 14:26. doi: 10.3389/fnana.2020.00026
Received: 05 March 2020; Accepted: 28 April 2020;
Published: 04 June 2020.
Edited by:
Javier Blesa, Centro Integral en Neurociencias A.C. HM CINAC, SpainReviewed by:
Barbara Picconi, Università Telematica San Raffaele, ItalyCristina Miguelez, University of the Basque Country, Spain
Copyright © 2020 Muñoz, Lopez-Lopez, Labandeira and Labandeira-Garcia. This is an open-access article distributed under the terms of the Creative Commons Attribution License (CC BY). The use, distribution or reproduction in other forums is permitted, provided the original author(s) and the copyright owner(s) are credited and that the original publication in this journal is cited, in accordance with accepted academic practice. No use, distribution or reproduction is permitted which does not comply with these terms.
*Correspondence: Ana Muñoz, YW5hbWFyaWEubXVub3pAdXNjLmVz