- Department of Psychology and Institute for Neuroscience, University of Texas at Austin, Austin, TX, USA
Discovering that transcranial infrared laser stimulation produces beneficial effects on frontal cortex functions such as sustained attention, working memory, and affective state has been groundbreaking. Transcranial laser stimulation with low-power density (mW/cm2) and high-energy density (J/cm2) monochromatic light in the near-infrared wavelengths modulates brain functions and may produce neurotherapeutic effects in a nondestructive and non-thermal manner (Lampl, 2007; Hashmi et al., 2010). Barrett and Gonzalez-Lima (2013) provided the first controlled study showing that transcranial laser stimulation improves human cognitive and emotional brain functions. But for the field of low-level light/laser therapy (LLLT), development of a model of how luminous energy from red-to-near-infrared wavelengths modulates bioenergetics began with in vitro and in vivo discoveries in the last 40 years. Previous LLLT reviews have provided extensive background about historical developments, principles and applications (Rojas and Gonzalez-Lima, 2011, 2013; Chung et al., 2012). The purpose of this paper is to provide an update on LLLT's neurochemical mechanisms supporting transcranial laser stimulation for cognitive-enhancing applications. We will explain first LLLT's action on brain bioenergetics, briefly describe its bioavailability and dose-response, and finish with its beneficial effects on cognitive functions. Although our focus is on prefrontal-related cognitive functions, in principle LLLT should be able to modulate other brain functions. For example, stimulating different brain regions should affect different functions related to sensory and motor systems.
Brain Bioenergetics
The way that near-infrared lasers and light-emitting diodes (LEDs) interact with brain function is based on bioenergetics, a mechanism that is fundamentally different than that of other brain stimulation methods such as electric and magnetic stimulation. LLLT has been found to modulate the function of neurons in cell cultures, brain function in animals, and cognitive and emotional functions in healthy persons and clinical conditions. Photoneuromodulation involves the absorption of photons by specific molecules in neurons that activate bioenergetic signaling pathways after exposure to red-to-near-infrared light. The 600–1150 nm wavelengths allow better tissue penetration by photons because light is scattered at lower wavelengths and absorbed by water at higher wavelengths (Hamblin and Demidova, 2006). Over 25 years ago, it was found that molecules that absorb LLLT wavelengths are part of the mitochondrial respiratory enzyme cytochrome oxidase in different oxidation states (Karu et al., 2005). Thus, for red-to-near-infrared light, the primary molecular photoacceptor of photon energy is cytochrome oxidase (also called cytochrome c oxidase or cytochrome a-a3) (Pastore et al., 2000).
Therefore, photon energy absorption by cytochrome oxidase is well-established as the primary neurochemical mechanism of action of LLLT in neurons (Wong-Riley et al., 2005). The more the enzymatic activity of cytochrome oxidase increases, the more metabolic energy that is produced via mitochondrial oxidative phosphorylation. LLLT supplies the brain with metabolic energy in a way analogous to the conversion of nutrients into metabolic energy, but with light instead of nutrients providing the source for ATP-based metabolic energy (Mochizuki-Oda et al., 2002). If an effective near-infrared light energy dose is supplied, it stimulates brain ATP production (Lapchak and De Taboada, 2010) and blood flow (Uozumi et al., 2010), thereby fueling ATP-dependent membrane ion pumps, leading to greater membrane stability and resistance to depolarization, which has been shown to transiently reduce neuronal excitability (Konstantinovic et al., 2013). On the other hand, electromagnetic stimulation directly changes the electrical excitability of neurons.
A long-lasting effect is achieved by LLLT's up-regulating the amount of cytochrome oxidase, which enhances neuronal capacity for metabolic energy production that may be used to support cognitive brain functions. In mice and rats, memory has been improved by LLLT (Michalikova et al., 2008; Rojas et al., 2012a) and by methylene blue, a drug that at low doses donates electrons to cytochrome oxidase (Rojas et al., 2012b). Near-infrared light stimulates mitochondrial respiration by donating photons to cytochrome oxidase, because cytochrome oxidase is the main acceptor of photons from red-to-near-infrared light in neurons. By persistently stimulating cytochrome oxidase activity, transcranial LLLT induces post-stimulation up-regulation of the amount of cytochrome oxidase in brain mitochondria (Rojas et al., 2012a). Therefore, LLLT may lead to the conversion of luminous energy into metabolic energy (during light exposure) and to the up-regulation of the mitochondrial enzymatic machinery to produce more energy (after light exposure).
Bioavailability and Hormetic Dose-Response
The most abundant metalloprotein in nerve tissue is cytochrome oxidase, and its absorption wavelengths are well correlated with its enzymatic activity and ATP production (Wong-Riley et al., 2005). High LLLT bioavailability to the brain in vivo has been shown by inducing brain cytochrome oxidase activity transcranially, leading to enhanced extinction memory retention in normal rats (Rojas et al., 2012a) and improved visual discrimination in rats with impaired retinal mitochondrial function (Rojas et al., 2008). Our LLLT studies utilized varied wavelengths (633–1064 nm), daily doses (1–60 J/cm2), fractionation sessions (1–6), and power densities (2–250 mW/cm2) that identified effective LLLT parameters for rats and humans.
For example, we tested in rats the effects of different LLLT doses in vivo on brain cytochrome oxidase activity, at either 10.9, 21.6, 32.9 J/cm2, or no LLLT. Treatments were delivered for 20, 40, and 60 min via four 660-nm LED arrays with a power density of 9 mW/cm2. One day after the LLLT session, brains were extracted, frozen, sectioned, and processed for cytochrome oxidase histochemistry. A 10.9 J/cm2 dose increased cytochrome oxidase activity by 13.6%. A 21.6 J/cm2 dose produced a 10.3% increase. A non-significant cytochrome oxidase increase of 3% was found after the highest 32.9 J/cm2 dose. Responses of brain cytochrome oxidase to LLLT in vivo were characterized by hormesis, with a low dose being stimulatory, while higher doses were less effective.
The first demonstration that LLLT increased oxygen consumption in the rat prefrontal cortex in vivo was provided by Rojas et al. (2012a). Oxygen concentration in the cortex of rats was measured using fluorescence-quenching during LLLT at 9 mW/cm2 and 660 nm. LLLT induced a dose-dependent increase in oxygen consumption of 5% after 1 J/cm2 and 16% after 5 J/cm2. Since oxygen is used to form water within mitochondria in a reaction catalyzed by cytochrome oxidase, more cytochrome oxidase activity should lead to more oxygen consumption.
LLLT may offer some advantages over other types of stimulation, because LLLT non-invasively targets cytochrome oxidase, a key enzyme for energy production, with induced expression linked to energy demand. Hence LLLT is mechanistically specific and non-invasive, while transcranial magnetic stimulation may be non-specific, prolonged forehead electrical stimulation may produce muscle spasms, and deep brain or vagus nerve stimulations are invasive.
Cognitive and Emotional Functions
LLLT via commercial low-power sources (such as FDA-cleared laser diodes and LEDs) is a highly promising, affordable, non-pharmacological alternative for improving cognitive function. LLLT delivers safe doses of light energy that are sufficiently high to modulate neuronal functions, but low enough to not result in any damage (Wong-Riley et al., 2005). In 2002, the FDA approved LLLT for pain relief in cases of head and neck pain, arthritis and carpal tunnel syndrome (Fulop et al., 2010). LLLT has been used non-invasively in humans after ischemic stroke to improve neurological outcome (Lampl et al., 2007). It also led to improved recovery and reduced fatigue after exercise (Leal Junior et al., 2010). One LLLT stimulation session to the forehead, as reported by Schiffer et al. (2009), produced a significant antidepressant effect in depressed patients. No adverse side effects were found either immediately or at 2 or 4 weeks after LLLT. Thus, these beneficial LLLT treatments have been found to be safe in humans. Even though LLLT has been regarded as safe and received FDA approval for pain treatment, the use of transcranial lasers for cognitive augmentation should be restricted to research until further controlled studies support this application for clinical use.
We used transcranial laser stimulation to the forehead in a placebo-controlled, randomized study, to influence cognitive tasks related to the prefrontal cortex, including a psychomotor vigilance task (PVT) and a delayed match-to-sample (DMS) memory task (Barrett and Gonzalez-Lima, 2013). The PVT assesses sustained attention, with participants remaining vigilant during delay intervals, and pushing a button when a visual stimulus appears on a monitor. Our laser stimulation targeted prefrontal areas which are implicated in the sustained attentional processes of the PVT (Drummond et al., 2005). Similarly, the DMS task engages the prefrontal cortex as part of a network of frontal and parietal brain regions (Nieder and Miller, 2004).
Healthy volunteers received continuous wave near-infrared light intersecting cytochrome oxidase's absorption spectrum, delivered to the forehead using a 1064 nm low-power laser diode (also known as “cold laser”), which maximizes tissue penetration due to its long wavelength, and has been used in humans for other indications. The power density (or irradiance), 250 mW/cm2, as well as the cumulative energy density (or fluence), 60 J/cm2, were the same that showed beneficial psychological effects in Schiffer et al. (2009). This laser exposure produces negligible heat and no physical damage at the low power level used. This laser apparatus is used safely in a clinical setting by the supplier of the laser (Cell Gen Therapeutics, HD Laser Center, Dallas, TX). Reaction time in the PVT was improved by the laser treatment, as shown by a significant pre-post reaction time change relative to the placebo group. The DMS memory task also revealed significant enhancements in measures of memory retrieval latency and number of correct trials, when comparing the LLLT-treated with the placebo group (Figure 1). Self-reported positive and negative affective (emotional) states were also measured using the PANAS-X questionnaire before and 2 weeks after laser treatment. As compared to the placebo, treated subjects reported significantly improved affective states. We suggest that this kind of transcranial laser stimulation may serve as a non-invasive and efficacious method to augment cognitive brain functions related to attention, memory, and emotional functions.
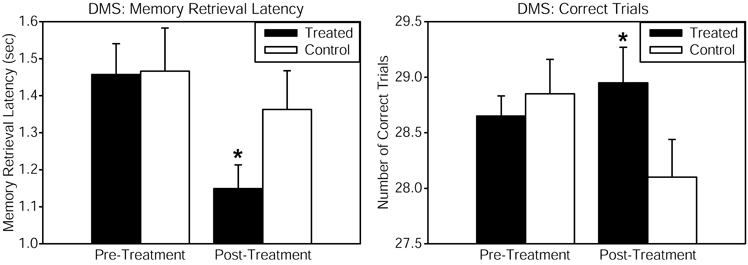
Figure 1. Cognitive performance in the delayed match-to-sample (DMS) memory task was improved after transcranial infrared stimulation to the right forehead. The DMS task involves presentation of a visual stimulus (grid pattern) on a screen. Then the stimulus disappears, and the participant must remember the stimulus through a delay. Then two choices appear, and the participant must decide which of these two is identical to the previous stimulus (the “match”). Treated subjects showed faster memory retrieval (left panel) and increased number of correct trials (right panel) out of 30 trials when attempting to choose the correct grid pattern. The function of frontal cortex regions, implicated in the attentional mode network utilized during this visuospatial memory task, was augmented by the laser treatment. Compared to baseline, this treatment also increased by 5% the oxyhemoglobin concentration of the prefrontal cortex as measured by near-infrared spectroscopy, both during the laser stimulation and during post-treatment DMS performance (in preparation). The data for the treated group consisted of n = 10 males and n = 10 females; the control group also consisted of n = 10 males and n = 10 females. *Significant treatment by pre-post score interaction, p < 0.05.
LLLT's bioenergetics mechanisms leading to cognitive augmentation may also be at play in its neuroprotective effects (Gonzalez-Lima et al., 2013). LLLT's stimulation of mitochondrial respiration should improve cellular function due to increased metabolic energy, as well as cellular survival after injury, due to the antioxidant effects of increases in cytochrome oxidase and superoxide dismutase (Rojas et al., 2008).
Laser transmittance of the 1064-nm wavelength at the forehead LLLT site was estimated in a post-mortem human specimen, which showed that approximately 2% of the light passed through the frontal bone. This yielded an absorption coefficient of a = 0.24, similar to the reported a = 0.22 transmittance through cranial bone for this wavelength (Bashkatov and Genina, 2006). Thus, we estimated that about 1.2 J/cm2 of the 60 J/cm2 LLLT dose applied reached the surface of the prefrontal cortex. This value is similar to 1 J/cm2, the peak effective LLLT dose in neuron cultures for increasing cytochrome oxidase activity (Rojas and Gonzalez-Lima, 2011).
Conclusions
Transcranial absorption of photon energy by cytochrome oxidase, the terminal enzyme in mitochondrial respiration, is proposed as the bioenergetic mechanism of action of LLLT in the brain. Transcranial LLLT up-regulates cortical cytochrome oxidase and enhances oxidative phosphorylation. LLLT improves prefrontal cortex-related cognitive functions, such as sustained attention, extinction memory, working memory, and affective state. Transcranial infrared stimulation may be used efficaciously to support neuronal mitochondrial respiration as a new non-invasive, cognition-improving intervention in animals and humans. This fascinating new approach should also be able to influence other brain functions depending on the neuroanatomical site stimulated and the stimulation parameters used.
References
Barrett, D. W., and Gonzalez-Lima, F. (2013). Transcranial infrared laser stimulation produces beneficial cognitive and emotional effects in humans. Neuroscience 230, 13–23. doi: 10.1016/j.neuroscience.2012.11.016
Bashkatov, A. N., and Genina, E. A. (2006). Optical properties of human cranial bone in the spectral range from 800 to 2000 nm. Proc. SPIE 6163, 616310. doi: 10.1117/12.697305
Chung, H., Dai, T., Sharma, S. K., Huang, Y. Y., Carroll, J. D., and Hamblin, M. R. (2012). The nuts and bolts of low-level laser (light) therapy. Ann. Biomed. Eng. 40, 516–533. doi: 10.1007/s10439-011-0454-7
Drummond, S. P., Bischoff-Grethe, A., Dinges, D. F., Ayalon, L., Mednick, S. C., and Meloy, M. J. (2005). The neural basis of the psychomotor vigilance task. Sleep 28, 1059–1068.
Fulop, A. M., Dhimmer, S., Deluca, J. R., Johanson, D. D., Lenz, R. V., Patel, K. B., et al. (2010). A meta-analysis of the efficacy of laser phototherapy on pain relief. Clin. J. Pain 26, 729–736. doi: 10.1097/AJP.0b013e3181f09713
Gonzalez-Lima, F., Barksdale, B. R., and Rojas, J. C. (2013). Mitochondrial respiration as a target for neuroprotection and cognitive enhancement. Biochem. Pharmacol. doi: 10.1016/j.bcp.2013.11.010. [Epub ahead of print].
Hamblin, M. R., and Demidova, T. N. (2006). Mechanisms of low level light therapy. Proc. SPIE 6140, 1–12. doi: 10.1117/12.646294
Hashmi, J. T., Huang, Y. Y., Osmani, B. Z., Sharma, S. K., Naeser, M. A., and Hamblin, M. R. (2010). Role of low-level laser therapy in neurorehabilitation. PM R 2, S292–S305. doi: 10.1016/j.pmrj.2010.10.013
Karu, T. I., Pyatibrat, L. V., Kolyakov, S. F., and Afanasyeva, N. I. (2005). Absorption measurements of a cell monolayer relevant to phototherapy: reduction of cytochrome c oxidase under near IR radiation. J. Photochem. Photobiol. B 81, 98–106. doi: 10.1016/j.jphotobiol.2005.07.002
Konstantinovic, L. M., Jelic, M. B., Jeremic, A., Stevanovic, V. B., Milanovic, S. D., and Filipovic, S. R. (2013). Transcranial application of near-infrared low-level laser can modulate cortical excitability. Lasers Surg. Med. 45, 648–653. doi: 10.1002/lsm.22190
Lampl, Y. (2007). Laser treatment for stroke. Expert Rev. Neurother. 7, 961–965. doi: 10.1586/14737175.7.8.961
Lampl, Y., Zivin, J. A., Fisher, M., Lew, R., Welin, L., Dahlof, B., et al. (2007). Infrared laser therapy for ischemic stroke: a new treatment strategy: results of the neurothera effectiveness and safety trial-1 (NEST-1). Stroke 38, 1843–1849. doi: 10.1161/STROKEAHA.106.478230
Lapchak, P. A., and De Taboada, L. (2010). Transcranial near infrared laser treatment (NILT) increases cortical adenosine-5'-triphosphate (ATP) content following embolic strokes in rabbits. Brain Res. 1306, 100–105. doi: 10.1016/j.brainres.2009.10.022
Leal Junior, E. C., Lopes-Martins, R. A., Frigo, L., De Marchi, T., Rossi, R. P., de Godoi, V., et al. (2010). Effects of low-level laser therapy (LLLT) in the development of exercise-induced skeletal muscle fatigue and changes in biochemical markers related to postexercise recovery. J. Orthop. Sports Phys. Ther. 40, 524–532. doi: 10.2519/jospt.2010.3294
Michalikova, S., Ennaceur, A., van Rensburg, R., and Chazot, P. L. (2008). Emotional responses and memory performance of middle-aged CD1 mice in a 3D maze: effects of low infrared light. Neurobiol. Learn. Mem. 89, 480–488. doi: 10.1016/j.nlm.2007.07.014
Mochizuki-Oda, N., Kataoka, Y., Cui, Y., Yamada, H., Heya, M., and Awazu, K. (2002). Effects of near-infra-red laser irradiation on adenosine triphosphate and adenosine diphosphate contents of rat brain tissue. Neurosci. Lett. 323, 207–210. doi: 10.1016/S0304-3940(02)00159-3
Nieder, A., and Miller, E. K. (2004). A parieto-frontal network for visual numerical information in the monkey. Proc. Natl. Acad. Sci. U.S.A. 101, 7457–7462. doi: 10.1073/pnas.0402239101
Pastore, D., Greco, M., and Passarella, S. (2000). Specific helium-neon laser sensitivity of the purified cytochrome c oxidase. Int. J. Radiat. Biol. 76, 863–870. doi: 10.1080/09553000050029020
Rojas, J. C., and Gonzalez-Lima, F. (2011). Low-level light therapy of the eye and brain. Eye Brain 3, 49–67. doi: 10.2147/EB.S21391
Rojas, J. C., Bruchey, A. K., and Gonzalez-Lima, F. (2012a). Low-level light therapy improves cortical metabolic capacity and memory retention. J. Alzheimers Dis. 32, 741–752. doi: 10.3233/JAD-2012-120817
Rojas, J. C., Bruchey, A. K., and Gonzalez-Lima, F. (2012b). Neurometabolic mechanisms for memory enhancement and neuroprotection of methylene blue. Prog. Neurobiol. 96, 32–45. doi: 10.1016/j.pneurobio.2011.10.007
Rojas, J. C., and Gonzalez-Lima, F. (2013). Neurological and psychological applications of transcranial lasers and LEDs. Biochem. Pharmacol. 86, 447–457. doi: 10.1016/j.bcp.2013.06.012
Rojas, J. C., Lee, J., John, J. M., and Gonzalez-Lima, F. (2008). Neuroprotective effects of near-infrared light in an in vivo model of mitochondrial optic neuropathy. J. Neurosci. 28, 13511–13521. doi: 10.1523/JNEUROSCI.3457-08.2008
Schiffer, F., Johnston, A. L., Ravichandran, C., Polcari, A., Teicher, M. H., Webb, R. H., et al. (2009). Psychological benefits 2 and 4 weeks after a single treatment with near infrared light to the forehead: a pilot study of 10 patients with major depression and anxiety. Behav. Brain Funct. 5, 46. doi: 10.1186/1744-9081-5-46
Uozumi, Y., Nawashiro, H., Sato, S., Kawauchi, S., Shima, K., and Kikuchi, M. (2010). Targeted increase in cerebral blood flow by transcranial near-infrared laser irradiation. Lasers Surg. Med. 42, 566–576. doi: 10.1002/lsm.20938
Keywords: cognitive enhancement, cytochrome oxidase, low-level light therapy, brain stimulation, photoneuromodulation
Citation: Gonzalez-Lima F and Barrett DW (2014) Augmentation of cognitive brain functions with transcranial lasers. Front. Syst. Neurosci. 8:36. doi: 10.3389/fnsys.2014.00036
Received: 31 January 2014; Accepted: 27 February 2014;
Published online: 14 March 2014.
Edited by:
Mikhail Lebedev, Duke University, USAReviewed by:
Julio C. Rojas, University of Texas Southwestern Medical Center, USAJohn Mitrofanis, University of Sydney, Australia
Copyright © 2014 Gonzalez-Lima and Barrett. This is an open-access article distributed under the terms of the Creative Commons Attribution License (CC BY). The use, distribution or reproduction in other forums is permitted, provided the original author(s) or licensor are credited and that the original publication in this journal is cited, in accordance with accepted academic practice. No use, distribution or reproduction is permitted which does not comply with these terms.
*Correspondence:Z29uemFsZXpsaW1hQHV0ZXhhcy5lZHU=