- 1Department of Rehabilitation Medicine, Keio University School of Medicine, Tokyo, Japan
- 2Department of Rehabilitation, Shonan Keiiku Hospital, Fujisawa, Japan
- 3Hokuto Social Medical Corporation, Obihiro, Japan
Barring a few studies, there are not enough established treatments to improve upper limb motor function in patients with severe impairments due to chronic stroke. This study aimed to clarify the effect of the kinesthetic perceptional illusion induced by visual stimulation (KINVIS) on upper limb motor function and the relationship between motor function and resting-state brain networks. Eleven patients with severe paralysis of upper limb motor function in the chronic phase (seven men and four women; age: 54.7 ± 10.8 years; 44.0 ± 29.0 months post-stroke) participated in the study. Patients underwent an intervention consisting of therapy using KINVIS and conventional therapeutic exercise (TherEX) for 10 days. Our originally developed KiNvis™ system was applied to induce KINVIS while watching the movement of the artificial hand. Clinical outcomes were examined to evaluate motor functions and resting-state brain functional connectivity (rsFC) by analyzing blood-oxygen-level-dependent (BOLD) signals measured using functional magnetic resonance imaging (fMRI). The outcomes of motor function (Fugle-Meyer Assessment, FMA) and spasticity (Modified Ashworth Scale, MAS) significantly improved after the intervention. The improvement in MAS scores for the fingers and the wrist flexors reached a minimum of clinically important differences. Before the intervention, strong and significant negative correlations between the motor functions and rsFC of the inferior parietal lobule (IPL) and premotor cortex (PMd) in the unaffected hemisphere was demonstrated. These strong correlations were disappeared after the intervention. A negative and strong correlation between the motor function and rsFC of the bilateral inferior parietal sulcus (IPS) significantly changed to strong and positive correlation after the intervention. These results may suggest that the combination approach of KINVIS therapy and TherEX improved motor functions and decreased spasticity in the paralyzed upper extremity after stroke in the chronic phase, possibly indicating the contribution of embodied-visual stimulation. The rsFC for the interhemispheric IPS and intrahemispheric IPL and PMd may be a possible regulatory factor for improving motor function and spasticity.
Clinical Trial Registration: www.ClinicalTrials.gov, identifier NCT01274117.
Introduction
Upper extremity motor function after stroke recovers in only 50% of all survivors at 6 months post-stroke (Kwakkel et al., 2003). Therefore, maximizing recovery of motor function in the upper extremity after stroke is socially meaningful (Langhorne and Legg, 2003), but is a challenge for the area of rehabilitation science. Constraint-induced movement therapy (CIMT) involves ipsilesional limb restraint with the training of the paretic arm (Wolf et al., 2006). There is moderate evidence of the therapeutic effects of CIMT; however, this therapy is adaptive for patients with more than moderate paralysis and not for patients with severe paralysis. In addition, mirror therapy (Dohle et al., 2009), mental practice (Page et al., 2007), and virtual reality therapy (Klamroth-Marganska et al., 2014) are shown to have beneficial effects with moderate-quality evidence (Pollock et al., 2014). All these therapies have been implemented in patients with dexterity (Kwakkel et al., 2003; Langhorne and Legg, 2003; Wolf et al., 2006; Page et al., 2007; Dohle et al., 2009; Klamroth-Marganska et al., 2014; Pollock et al., 2014). Recently, clinical trials for patients with stroke in the chronic phase were reported. The effect size of the mirror therapy (Colomer et al., 2016) or functional electrical stimulation (Carda et al., 2017) intervention may be insufficient. Additionally, clinical trials using the brain-machine interface have demonstrated a higher effect size (Ramos-Murguialday et al., 2013, 2019). Although a few influential results have been reported, other significant therapeutic approaches for patients with chronic stroke should be developed.
Nerve fiber tracking, which is performed using diffusion tensor tractography, is often used clinically and demonstrates the absence of fibers in the tract descending from the primary motor (M1) and somatosensory (S1) cortices to the brain stem. Correlation analyses between infarct volume (Saver et al., 1999) and location with motor impairment indicate that damage in the motor areas, corona radiata, and internal capsule decrease the probability of upper limb functional recovery (Binkofski et al., 1996; Shelton and Reding, 2001; Crafton et al., 2003; Schiemanck et al., 2008; Johansen-Berg et al., 2010; Moulton et al., 2015). Several functional magnetic resonance imaging (fMRI) studies have analyzed blood-oxygen-level-dependent (BOLD) signals as a measure of resting-state brain functional connectivity (rsFC) among brain sites (Carter et al., 2010; Urbin et al., 2014). However, these studies have not discussed the classification of the time course of recovery from a stroke or the recovery stages of sensory-motor function. In the acute phase after stroke, the inter- and intra-hemispheric rsFC differ depending on the severity of the stroke and become weak in patients with severe conditions (De Bruyn et al., 2018). In patients in the subacute-to-chronic phase after stroke, the rsFC between M1 of each hemisphere decreases compared with that in healthy control individuals (Zhang et al., 2016). In a previous study, the rsFC between S1 and other regions indicated an asymmetrical difference, and the index of asymmetry significantly correlated with motor function deficits (Frías et al., 2018).
We have conducted clinical studies including patients with severe motor paralysis to induce recovery of motor function using kinesthetic illusion induced by visual stimulation (KINVIS). KINVIS is defined as the psychological phenomenon in which a person who is resting feels as if a part of his/her own body is moving or feels the desire to move a body part while watching a movie of the body part that is moving. To establish the therapeutic utility of this phenomenon, we have developed a system that is clinically useful, named KiNvis™. In KINVIS therapy, the patient experiences KINVIS while neuromuscular electrical stimulation is applied to the muscle that is an agonist to the one whose movement is being watched in the movie. Therefore, KINVIS therapy indicates a combination of KINVIS with neuromuscular electrical stimulation. This system can be viewed as an intervention of virtual reality (augmented reality) using embodied-visual feedback, which can induce embodied cognitive change in self-body and physiological effects on motor-associated areas in the brain (Kaneko et al., 2007, 2015, 2016a,b; Aoyama et al., 2012). In other words, this may represent a cognitive stimulation to the embodied brain system for body ownership, a sense of agency, and kinesthetic perception. A specific brain network is activated during KINVIS (Kaneko et al., 2015). The first report of the physiological effects of embodied-visual feed-back indicated an enhancement of M1 excitability (Kaneko et al., 2007), which is sustained after KINVIS therapy (Kaneko et al., 2016a). Frequently, while the subject is experiencing KINVIS, a spontaneous muscular contraction can be observed in the agonist muscle that is shown in the movie (Itaguchi, 2018, unpublished data). We consider that these previous studies suggest that KINVIS may be associated with the drive of the nervous system for motor output. Moreover, a previous feasibility study showed that motor function immediately changes in patients with stroke exhibiting severe paralysis (Kaneko et al., 2016b). Therefore, we speculated that the repetitive application of KINVIS therapy in addition to conventional therapeutic exercise (TherEx) could produce a positive effect on motor function, with enhanced brain plasticity, in patients with stroke. We hypothesized that the connectivity of brain regions of interest (ROIs) that are associated with sensory-motor function and KINVIS might improve and induce changes in motor function.
Thus, the purpose of this study was to clarify the effect of repetitive KINVIS therapy in combination with TherEx on upper limb motor function and brain network function, defined as rsFC measured by BOLD signal analysis on fMRI, in patients with severe motor paralysis after stroke.
Materials and Methods
Study Design
This was a prospective case series study involving patients after stroke with hemiparesis.
Participants
Eleven patients participated in this study (seven men and four women; five with right hemiparesis and six with left hemiparesis; age: 54.7 ± 10.8 years; height: 162.5 ± 7.7 cm; weight: 65.2 ± 13.7 kg; Table 1). All patients provided written informed consent to participate in this study, which was approved by the local ethics committee (Shonan Keiiku Hospital, No. 17-0005) and conformed to the Declaration of Helsinki. This study was registered as a clinical trial with the University Hospital Medical Information Network in Japan (UMIN Critical Trial Registry UMIN000032286). The inclusion criteria were: (a) diagnosis of unilateral stroke, not involving the cortex; (b) Stroke impairment assessment set (SIAS) of the finger function 1A (ability to flex the paretic fingers voluntarily but not to extend them; Sonoda et al., 1993; Chino et al., 1996); (c) passive range of motion greater than −30° for metacarpophalangeal joint extension; (d) time from stroke onset to be more than 4 months; (e) ability to walk independently in daily life with or without assistance; (f) age older than 18 years; and (g) not receiving other special rehabilitation or treatment for upper extremity paralysis such as transcranial magnetic stimulation, repetitive facilitative exercise, and botulinum toxin within 3 months.
The exclusion criteria were: (a) inability to understand the purpose and task of this study; (b) severe internal disorder of the heart and metabolism; and (c) less than 0 score (never become more than 1) on the questionnaire of body ownership or kinesthetic sensation (as described below) during the intervention period.
Edinburgh Handedness Inventory (Oldfield, 1971) was used to assess patients’ handedness before the intervention onset. All participants were right-handed (the scores were 84.0 ± 17.6%).
As a supplementary experiment, 30 healthy individuals (17 men and 13 women; age: 25.2 ± 4.8 years; height: 165.2 ± 8.6 cm; weight: 59.2 ± 12.3 kg) were included for the measurements of functional magnetic resonance imaging, in addition to the patients. They had no neurological or psychiatric diseases and all participants were right-handed as indicated through the Edinburgh Handedness Inventory test (healthy group scores 82.9 ± 21.0%). MRI data acquisition was performed only once during this study period, between 4:00 PM and 5:30 PM. They were instructed to perform normal daily activities on the day of the MRI scan.
Interventions
The experiment consisted of 10 days of intervention on weekdays and evaluations before and after the intervention (Figure 1A). The intervention included KINVIS therapy and conventional therapeutic exercise (TherEX).
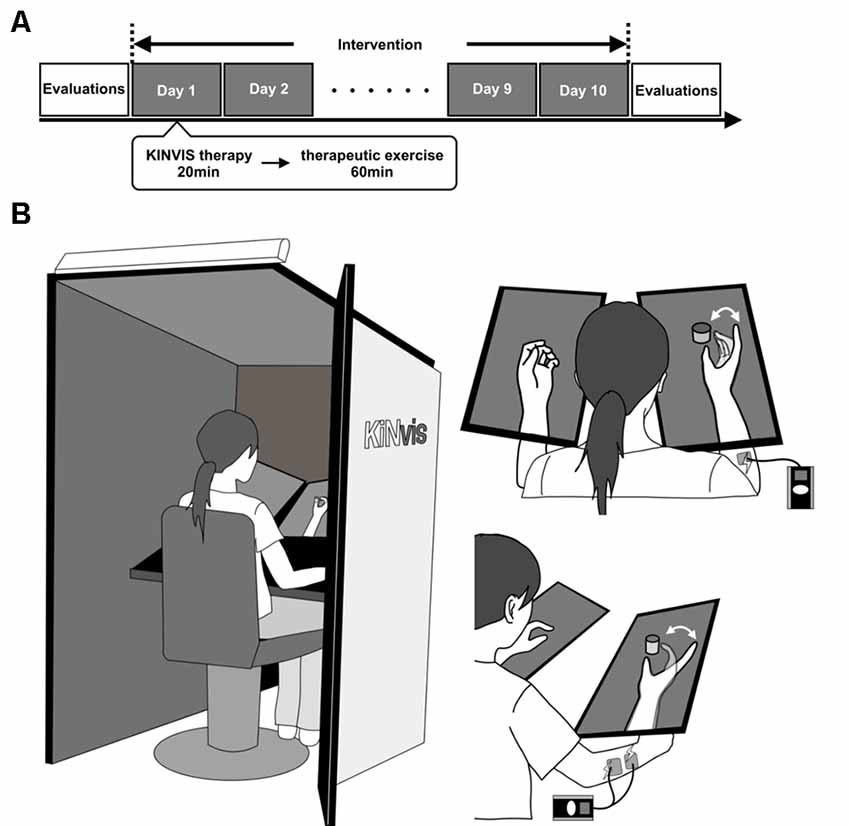
Figure 1. (A) Outline of the experimental plan. The experiment consisted of 10 days of intervention on weekdays and evaluations before and after the intervention. The intervention included conventional therapy for kinesthetic illusion induced by visual stimulation (KINVIS) therapy and therapeutic exercise (TherEX). KINVIS was applied for 20 min, and TherEX was applied after KINVIS therapy for 60 min by physical or occupational therapists. (B) KINVIS therapy set-up. KINVIS used the KiNvis™ System that consists of a table with two monitors, a chair for the patient to sit, both within a box to encourage concentration on the movie. Neuromuscular Electrical Stimulation was applied in combination with KINVIS. The patients’ arm was supported on a stand to avoid any somatosensory input.
KINVIS
The cognitive phenomenon of KINVIS can be described as the feeling of one’s body moving during sensory input, even though the body is actually in a resting state.
The subjects were applied a visual stimulation for 20 min based on our previous report using the KiNvis™ system (Kaneko et al., 2016a). Furthermore, we applied neuromuscular electrical stimulation (NMES; NM-F1, Ito Company Limited) in combination with KINVIS (Figure 1B) as a KINVIS therapy. The patients were seated at a chair with their forearm on the table. The hand movement of the unaffected side was recorded before the intervention. The movement task involved hand opening and closing. This task was executed using the unaffected side and was flipped to mirror the movement of the affected side. Their arm was supported on a stand to avoid any somatosensory input.
Surface electrodes for stimulation were placed bilaterally on the skin overlying the extensor digitorum communis (EDC) muscle. Electrical stimulation was applied at an intensity higher than the motor threshold of the muscle (motor threshold, 1.0–1.2 times higher; frequency, 20 Hz; pulse width, 50 μs), while watching the movie during the finger-extension phase. During KINVIS therapy, the patients were relaxed and were instructed not to move their hands. KINVIS therapy was applied at two sets of 10 min, for a total of 20 min per day. Participants answered a question on body ownership and kinesthetic sensation in each set. Specifically, the question on body ownership was: “I felt the hand in the screen like own hand as if it was part of my own body during watching the movie”; this was based on a previous study on rubber hand illusion (Botvinick, 2004). The question on kinesthetic sensation was: “I had a feeling that my hand is actually moving during watching the movie” (Kaneko et al., 2015). Patients selected a level to agree/disagree from the following 7-point Likert scale for each question, as follows: −3, strongly disagree; −2, disagree; −1, somewhat disagree; 0, neither agree nor disagree; 1, somewhat agree; 2, agree; 3, strongly agree. The sense of ownership and kinesthetic sensation in each patient were calculated from the median score on the 7-point Likert scale in a period of 10 days.
TherEX
TherEX was applied for 60 min by a physical or occupational therapist after KINVIS therapy. Generally, the TherEX was composed of a kind of task-oriented exercise for finger movement and proximal joint movement, and stretching flexor muscles. For each patient, individual exercises for upper extremities were selected to gradually increase the difficulty level in a conventional way. Since suppression of the spasticity for each subject’s finger and wrist flexors were manually realized after KINVIS therapy, execution of some task had been possible according to individual condition. For example, a simple pinch and release a small object (from 5 mm to several centimeter balls, columns, etc.), which was size the subject could execute it with relaxed the proximal muscles and the wrist flexion position, was chosen as a task. We did not prescribe a special approach (i.e., repetitive transcranial magnetic stimulation, transcranial direct current stimulation, CIMT). Each patient was instructed to self-exercise, such as exercising and stretching their fingers, as well as performing activities of daily living assisted by a therapist.
Outcome Measures
Clinical assessments of this study included measurements of upper limb motor function, spasticity, and actual use of the affected upper limb in activities of daily living. All clinical assessments were measured within an hour.
Fugl-Meyer assessment (FMA) was performed to assess upper extremity motor recovery. This test consisted of shoulder/elbow/forearm, wrist, hand movement and coordination. The FMA upper extremity motor score ranges from 0 to 66 (Platz et al., 2005). The Modified Ashworth Scale (MAS) was used to assess muscle tone in the 2nd to 5th finger flexor muscles, and wrist flexor muscles. The MAS is an ordinal scale with scores of 0, 1, 1+, 2, 3, 4 (Li et al., 2014). To calculate the mean value of MAS scores, score 1+ was transformed to 2, and scores 2, 3, and 4 were transformed to 3, 4, and 5.
The action research arm test (ARAT) was used to reflect motor function. The ARAT consist of four components; grasp, grip, pinch, and gross arm movement. This test involves transferring and picking up objects of different size. Each component’s score ranges from 0 to 4, and the total score ranges from 0 to 57 (Platz et al., 2005). The Box and Block Test (BBT) is one of the most common measures of motor function in stroke (Platz et al., 2005), and normative data are available for healthy adults (Mathiowetz et al., 1985). The score is calculated by the number of blocks transported in 60 s.
The motor activity log (MAL) assesses the actual use of upper limbs in daily living. This test is divided into the amount of use (AOU) and the quality of movement (QOM). The MAL is a self-report questionnaire inquiring about the amount and quality of daily use of the affected upper limb for 14 daily activities, such as holding a book and picking up a glass (Uswatte et al., 2005).
MRI Data Acquisition
Two conditions of MRI data were obtained. First, resting-state fMRI was performed to measure rsFC. During this session, participants were instructed to keep their eyes open and maintain wakefulness, without moving their head. For the resting-state fMRI scanning session, a gradient-echo echo-planar sequence was used (3.75 × 3.75 × 3.5 voxels; echo time = 40 ms; repetition time = 2,500 ms, flip angle = 85°), in order to collect BOLD contrast data of 244 slices. Second, a T1-weighted MRI scan was acquired, and participants were asked to keep their eyes closed during this session. T1-weighted structural images were acquired as an anatomical reference (0.4688 × 0.4688 × 1.4 mm voxels; echo time = 3.076 ms; repetition time = 8.368 ms; flip angle = 12°). All MRI data were obtained using a 1.5-T MRI scanner with a head coil (Optima 450w, GE Healthcare, Chicago, IL, USA). MRI data acquisition was performed before and after the intervention for patients.
Resting-State fMRI Data Preprocessing
All data preprocessing sessions and analyses were carried out using CONN toolbox (Whitfield-Gabrieli and Nieto-Castanon, 2012) implemented on MATLAB (Mathworks Inc., Natick, MA, USA). CONN is a conjunction in Statistical Parametric Mapping 12 (SPM12; Well-come Department of Imaging Neuroscience, London, UK); therefore, the following preprocessing pipeline is SPM12 compliant. Image preprocessing consisted of: (1) reducing the signal for the first four slices for magnetization equilibrium effects and adaptation of the subjects to the circumstances; (2) flipping of all structural/functional data of right-hand affected patients using non-rigid reflection along the x-axis, in order to align the affected hemisphere on the left side; (3) functional realignment and unwarping; (4) slice-timing correction applied to the images; (5) creation of binary masks in gray matter, white matter, and cerebrospinal fluid images using structural segmentation; (6) denoising using outlier detection thresholded 97th percentile in normative samples of framewise displacement; (7) normalization to the EPI image template conforming to the Montreal Neurological Institute (MNI) space (2 mm iso voxels); (8) spatial smoothing with an isotropic Gaussian kernel of 7-mm full width at half maximum; and (9) band-pass filtering with a setting of 0.01–0.08 Hz.
ROIs in This Intervention
Twenty ROIs associated with sensory-motor function and KINVIS were defined as spherical seed regions with a radius of 6 mm. ROIs were defined in the motor-related activation area, KINVIS activated area, and supramarginal gyrus (SMG), based on previous fMRI studies (Mayka et al., 2006; Gentile et al., 2013; Kaneko et al., 2015). The names and MNI coordinates of ROIs are shown in Table 2. Each of 10 ROIs was created in the MNI space on the affected and unaffected hemispheres.
Statistical Analyses
For motor function, a paired t-test was used to compare clinical assessments before and after the intervention. The statistical significance level was 5%. Statistical analyses were performed using SPSS, version 24.0 J (SPSS, Japan). Furthermore, we calculated the effect size of the clinical assessment scale using Cohen’s d statistics, and the magnitude of the difference between before and after the intervention was defined as small if d = 0.2, medium if d = 0.5, or large if d = 0.8, considering the clinical significance of the variables.
For rsFC scores, seed-based connectivity analysis was used to identify the brain regions temporally correlated using BOLD signal fluctuations in the ROIs. To represent the level of rsFC between each ROI and every location in the brain, this analysis used Z-scores which were the Fisher-transformed bivariate correlation coefficients between BOLD time-series averaged across all voxels within an ROI and an individual voxel.
In order to assess rsFC between an ROI and other regions at each measurement, we conducted one-sample t-test for Z-scores to search for ROI-to-Voxel with a particularly significant rsFC in each session (independently before and after the intervention), as reported by Zhang et al. (2016). Since Eklund et al. (2016) confirmed that the false positive rate is high when using the conventional threshold value, we searched for a site by the conservative threshold. As a result, threshold was set to the voxel level false-discovery-rate (FDR) corrected p < 0.01 and at cluster level FDR corrected p < 0.0001.
This study primarily focused on exploring whether the rsFC and motor functions would be indicated or whether the intervention in the patients with stroke would influence these relationships. Hence, Z-scores between significant clusters and ROIs were calculated to verify the correlation between Z-score and FMA upper extremity motor or ARAT total score. Similarly, for the stage of before intervention, the cluster mask of after intervention was used to calculate the Z-score between the same sites. Pearson’s product-moment correlation coefficient was used for this analysis. The statistical level was corrected to p < 0.025 using the Bonferroni method to avoid type 1 errors caused by verifying the correlation between ROI and motor function twice (i.e., FMA upper extremity motor and ARAT total score). In addition, if the correlation between Z-scores before or after the intervention and motor function was judged to be significant, testing the significance of the correlation coefficient was used to verify whether the correlation coefficient was significantly different. The significant threshold was corrected by the Bonferroni method to p < 0.05/n according to n times of tests.
Results
KINVIS was induced in all patients. The 7-Likert scale [median (IQR)] scores on body ownership and kinesthetic sensation were 2 (2–2) and 2 (1–2), respectively. These results suggest that self-body cognitive effects during KINVIS were detected subjectively.
Clinical Examination of Behavior
The results of clinical assessments are reported in Table 3. Regarding the FMA, the Shoulder/Elbow/Forearm score and, accordingly, the upper extremity motor score were significantly improved by the intervention (Shoulder/Elbow/Forearm: p = 0.003, effect size 0.63; upper extremity motor score: p = 0.003, effect size 0.65).
The intervention significantly improved the MAS scores of 2nd to 5th finger flexor muscles and wrist flexor muscles (2nd to 5th finger flexor muscles: p = 0.008, effect size 1.28; wrist flexor muscles: p = 0.001, effect size 1.01), the total ARAT score (p = 0.018, effect size 0.46), and the AOU and QOM scores of the MAL (AOU: p = 0.007, effect size 0.73, QOM: p = 0.008, effect size 0.49), while the BBT score showed no significant improvement.
Brain Functional Connectivity
Seed-based connectivity analysis of data acquired from 11 patients demonstrated several rsFC changes. Before the intervention period, there were several significant rsFC changes between an ROI in the affected and unaffected hemispheres, and other voxels (Table 4A, Figure 2A). For interhemispheric rsFC, five ROIs in the parietal cortex of the affected hemisphere showed significant rsFC with areas of the contralateral homologous site. In contrast, after the intervention, significant and positive rsFCs with contralateral homologous sites were additionally represented in the inferior parietal lobule (IPL), intra-parietal sulcus (IPS), and insula (Table 4A).
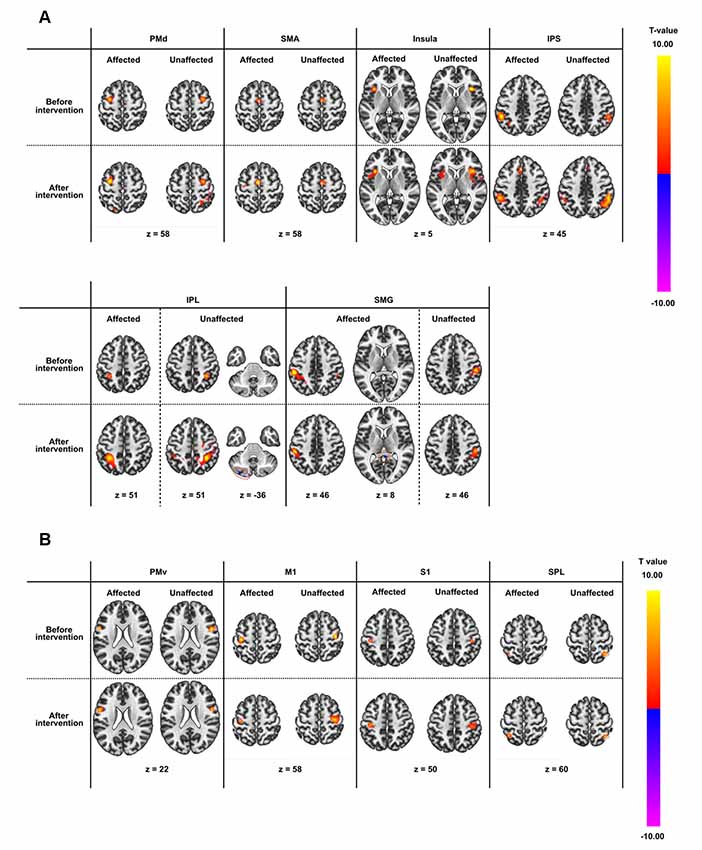
Figure 2. Results of seed-based functional connectivity in all regions-of-interest (ROIs). ROI names are defined in Table 2. Results before and after the intervention are displayed in rows, while those in the affected and unaffected hemispheres are displayed in columns. All connectivity maps were thresholded at voxel level false-discovery-rate (FDR) corrected at p < 0.01 and at cluster level FDR corrected at p < 0.0001. (A) ROIs showing significant intrahemispheric or interhemispheric resting-state brain functional connectivity (rsFC). (B) ROIs not showing significant intrahemispheric or interhemispheric rsFC apart from the rsFC to themselves.
Before the intervention, only the dorsal premotor cortex (PMd) in the affected hemisphere showed significant rsFC within the ipsilateral brain site [precuneus and lateral occipital cortex (LOC); Table 4B]. After the intervention, the PMd and supplementary motor area (SMA) in the affected hemisphere indicated significant rsFC with the frontal lobe and the parietal cortex (broad areas including S1 and parietal lobule for PMd; and S1, IPS and IPL for SMA) in the ipsilateral brain hemisphere (Table 4C). There was no significant intra-hemispheric rsFC of ROIs in the unaffected brain before the intervention. However, the PMd in the unaffected brain showed significant rsFC with the frontal lobe and parietal cortex after the intervention (Table 4C), similar to that observed for the PMd in the affected side after the intervention. The IPS and IPL in the unaffected side showed significant rsFC with the frontal lobe after the intervention, especially, we detected rsFC of the IPL with the motor areas of the superior frontal gyrus (SFG) and preCG (PMd). Furthermore, ROIs of the unaffected IPL and affected SMG showed significant rsFC with the cerebellum on the affected side. Figure 2B shows there was no connectivity change in PMv, M1, S1, and SPL.
There was a large number of significant intra- and inter-hemispheric rsFC in the healthy group. rsFC in patients after the intervention were common to rsFC represented in healthy group (Supplementary Figure S1, Table S1). The size of the region of the cluster was generally larger in the healthy group than the patients group. rsFC between IPL and SMG in the patient group did not contradict those in the healthy group.
Correlation Between Brain Functional Connectivity and Motor Function
The correlation between the Z-score and the motor functions was analyzed before and after the intervention and compared. The Z-score was calculated from the combination of significant ROI-to-cluster obtained from the results of the seed-based analysis. Table 5 indicates correlation coefficients and significances between functional connectivity from ROI-to-cluster and motor functions. In addition, Figure 3 shows the scatter plots of Z-score and the value of motor functions that demonstrated significant correlations among those shown in Table 5, and the results of examination for the significance of the correlation coefficients between stages of before and after the intervention.
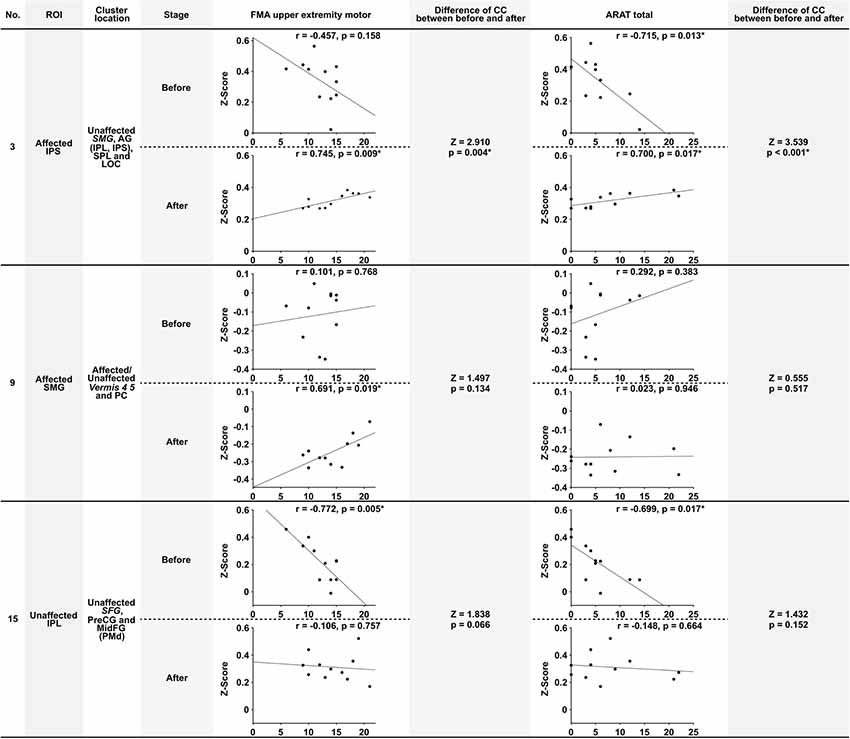
Figure 3. Scatter plots of significant correlation in Table 5, and the results of testing the significance of the correlation coefficient. This figure shows the scatter plots of the significant correlations shown in Table 5. The number (No), ROI name, and cluster location name of Columns are associated with Tables 4, 5. Stage means the assessment stage before and after the intervention. The correlation coefficient (r) and the probability value (p) shows above the scatter plot. The column of “Difference of CC (correlation coefficient) between before and after” shows the result of testing of the significance of the correlation coefficient (Z, Z-score; p, probability value). The significance probability defined p = 0.05/6 because a total of six tests were required. Values were marked *if they were below this threshold. Abbreviations: FMA upper extremity motor, the score of Fugl-Meyer assessment upper extremity motor function; ARAT total, Total score of Action research arm test; SMG, supramarginal gyrus; AG, angular gyrus; SPL, superior parietal lobule; MidFG, middle frontal cortex; PreCG, precentral gyrus; PC, cingulate gyrus posterior division.
Before the intervention, the connectivity of the bilateral parietal cortex was negatively correlated with ARAT total (Table 5, No. 3). Furthermore, the connectivity of IPL and PMd was also negatively correlated with the motor functions before the intervention (Table 5, No. 15). Connectivity between the bilateral IPS (including IPL, SPL, and LOC in the unaffected hemisphere) was positively correlated between the FMA upper extremity motor and ARAT total after the intervention. Moreover, connectivity between SMG in the affected hemisphere only and the affected or unaffected cerebellum vermis showed a positive correlation only after the intervention (Table 5, No. 9), although this correlation was not significant. Among the significant correlations in each stage, the connectivity that showed the significant difference before and after the intervention was that of the bilateral IPS (FMA upper extremity motor: Z = 2.910, p = 0.004; ARAT total: Z = 3.539, p < 0.001). Therefore, significant changes in the relationship between the movement functions and rsFC in the bilateral IPS after the intervention were demonstrated.
Discussion
The present research was the first prospective study demonstrating the effect of KINVIS therapy with TherEX on motor function and brain function in patients with stroke. As we expected from our preliminary study (Kaneko et al., 2016a), the motor functions examined with FMA and MAS improved after the therapeutic intervention period. The improvements in MAS reached a minimum of clinically important differences (Platz et al., 2005; Li et al., 2014). Furthermore, as the main result for rsFC, a significant change in the relationship between the motor functions and rsFC of bilateral IPS occurred parallel to the motor functional improvement. Although significant rsFC changes between an ROI and the clusters in each stage were also detected, they did not indicate direct comparison between stages. Clinically, the meaning of the differences in the detected significant pairs of the ROIs and the clusters in each stage was not indicted. The number of ROIs with significant rsFCs was limited before the intervention; however, 15 common pairs (Table 4A) of functionally connected ROIs were detected after the intervention. Therefore, we suggest that these pairs indicate significance in each stage. However, a future study including a control group should be designed with a direct comparison between stages.
The intervention adopted in the present study was “KINVIS,” which is a novel approach to hemiparesis developed by our group. From the results for the subjective score on the embodiment, body ownership, and KINVIS, this novel approach may influence the brain system associated with embodiment. We reported a number of fundamental studies regarding the physiological effect of this approach and the suspected clinical effects on paralytic extremities (Kaneko et al., 2007, 2015, 2016a,b; Aoyama et al., 2012). This technology can be viewed as a kind of virtual reality using embodied-visual feedback; however, it is not just virtual reality but can also induce embodied cognitive changes in one’s body and physiological effects on motor-associated areas in the brain. The cognitive effects induced during KINVIS therapy include body-ownership of the artificial body in the movie, kinesthetic perception, and/or a kind of desire or will to move one’s body. The point of this technology was to induce the illusory kinesthetic perception of the subject’s body, in contrast to the control condition in the previous experiment that involved a simple movement observation without such perception. Since our previous experiments indicated the physiological advantages of KINVIS as a therapeutic intervention for hemiparesis after stroke (Kaneko et al., 2007, 2015, 2016a,c; Aoyama et al., 2012), we conducted this first clinical trial.
An important feature of this study was that the participants were patients with severe hemiparesis and in the chronic phase after stroke. There are not many studies aimed at improving motor function in patients with chronic severe hemiparesis, as it is extremely difficult to apply a TherEX for hand and finger movement function. To compare the effect of various interventions to that of KINVIS, we searched PubMed for articles including the following key terms: stroke, chronic, upper limb, severe, and rehabilitation. As a result, we extracted 143 articles. Articles before 2014 were drawn from the Cochrane review (Pollock et al., 2014). There were 20 articles in which FMA or MAS were evaluated, and the effect size could be calculated. Since the patients in the present study were relatively severely paralyzed, articles in which the participants’ FMA score was less than 15 points were selected to discuss the effect size of interventions. Using these inclusion criteria, six articles were selected (Stinear et al., 2008; Ramos-Murguialday et al., 2013, 2019; Colomer et al., 2016; Grimm et al., 2016; Carda et al., 2017). Among those, the effect size was more than medium (Cohen’s d > 0.5) in only two articles. The effect size in our study was comparable to that of the previous six articles, indicating that the KINVIS therapy may be a powerful, effective approach for chronic, severe paralysis after stroke, and further clinical research is required. Importantly, the duration of intervention in our study was much shorter than that in the previous six studies. The duration of the intervention was at least 2 weeks in one study and more than 3 weeks in the other five studies. The intervention duration in our study was only 10 days, which is an advantage of our approach. The effect of long-term KINVIS therapy is not clear and should be assessed in future studies.
Our study also revealed a significant effect of the intervention on the spasticity of flexor muscles in the upper extremity, and the difference of MAS score reached the Minimal Clinically Important Difference (Platz et al., 2005; Li et al., 2014). As no previous study has indicated an effect on spasticity except for medication studies (Francis et al., 2004; Chen et al., 2005; Barros Galvão et al., 2014), this result is of great clinical importance. Moreover, the decrement in spasticity in the finger and wrist joint flexor muscles would now allow patients to proceed to TherEX, particularly in the case of patients with severely paralyzed upper extremities, who are in a sustained clenching state, making it impossible to apply any TherEX without KINVIS therapy. This decrement in spasticity suggests that KINVIS therapy is better applied before TherEX. There is currently no study reporting similar results, thus this new approach may be useful in improving paralysis therapy. One limitation of this study is the lack of control data; however, these positive changes in motor functions after the novel intervention strategy provide significant insight for future clinical studies.
As a noteworthy result indicated in the present study, a strong correlation between rsFC in the bilateral parietal cortex and the motor functions after the intervention period was demonstrated. This result possibly suggests that rsFC in the bilateral IPS in a severely paralyzed patient with stroke in the chronic phase may be a prognostic indicator for motor function recovery potential; controlled clinical trials may clarify this important matter in the near future. There was a negative correlation between rsFC of the bilateral IPS and motor function of the ARAT before the intervention period, and the change in the correlation towards the positive direction after the intervention. We estimate that the positive correlation observed after the intervention period may represent the capability a patient essentially possessed, which is either real or masked. Since the negative correlation between rsFC and motor function may be contaminated by the brain functional disruption owing to the stroke and/or negative adaptation to dis-use of the upper limb owing to sensory-motor functional paresis, we speculate the present intervention restored and advanced the sensory-motor and brain function in parallel. A similar phenomenon was demonstrated in a previous study from another group (Marumoto et al., 2013), and it may represent a common state in patients with stroke. The mechanism underlying these relationships is an interesting discussion point and it should be investigated in a future study. Also, connectivity between SMG in the affected hemisphere and the affected or unaffected cerebellum vermis showed a positive correlation with FMA only after the intervention. Furthermore, connectivity between the IPL and PMd in the unaffected hemisphere was also negatively correlated before the intervention. It would be clinically meaningful if the disappearance of this negative correlation in the unaffected hemisphere reflects the abnormal compensation of brain hyperactivity, as indicated in previous studies (Nowak et al., 2008; Dodd et al., 2017). If our novel approach can reduce contralesional hyperactivity, this would indicate its clinical potential and relevance. Besides, significantly increased connectivity was demonstrated in PMd with the parietal cortex in the affected hemisphere in both hemispheres after the intervention period. The anatomical fibers connecting the parietal cortex and PMd can be interpreted as the association fiber network (the superior longitudinal fasciculus fiber tracts) that includes the network predominantly involved when humans recognize changes in their position (Amemiya and Naito, 2016; Naito et al., 2016). The brain networks that were demonstrated to change in this study should be discussed, and whether these are associated with the reconstruction of body-schema alterations performed by KINVIS should be investigated in future studies. On the other hand, among these significant correlations, the connectivity that showed a significant difference between the stages before and after the intervention was that of the bilateral IPS only. We estimate that the rsFC bilaterally around the IPS may be key in connectivity, and is possibly associated with the functional improvement in the subject group recruited in this study. The pairs of brain areas where changes were indicated after simple TherEX in previous reports were homologous ROIs constituting the sensory-motor network (Carter et al., 2010; Urbin et al., 2014) and M1 (Carter et al., 2010, 2012; Wang et al., 2010; Westlake and Nagarajan, 2011; Urbin et al., 2014; Fan et al., 2015) only. Our results indicated homologous ROIs were correlated in the insula and areas of the parietal cortex (IPS, IPL, and SMG). Changes in these networks have not been previously reported, especially the change in the relationship between motor function and rsFC, which may be of clinical relevance.
Common brain areas between this and our previous study (Kaneko et al., 2015) that were associated with the effect of the KINVIS intervention were the SMA, PMd, SPL, IPL, and insula. These ROIs indicated a stronger intra-hemispheric correlation. We also found that the pairs of ROIs in the rostral-caudal direction, which may be connected via association fibers, were more strongly correlated after than before the intervention. The actual functional importance of this finding is not clear. However, the present results suggest that changes in intra-hemispheric rsFC in the uninvolved brain areas may be important for the recovery of motor function and spasticity. This neural plasticity was induced within only 10 days in patients in the chronic phase after stroke and occurred in parallel with the behavioral improvement. Our future objective is to decipher the network associated with this behavioral improvement.
Age-matched control trials are still needed in the future to gain further insight into the mechanisms involved in this phenomenon and to identify the brain networks that should be targeted in therapeutic approaches for the recovery of motor function. However, the functional improvement of the motor function and the indicated difference in the relationship between rsFC and motor function after combination approach of KINVIS therapy and TherEX in a severely paralyzed chronic phase patient with stroke were both clinically relevant and novel.
Conclusions
This prospective exploratory clinical trial provided the first evidence that a combination therapeutic approach, i.e., KINVIS therapy with TherEX, may improve the motor functions in patients with severe hemiparesis in the chronic phase after stroke, as predicted by our preliminary report (Kaneko et al., 2016b). Our results are comparable to those previously reported in studies including patients who were not as severely paralyzed as in our trial.
Furthermore, changes of the relationship (negative to positive) between the motor functions and the interhemispheric rsFC of the bilateral IPS, and disappearance of the significant negative correlation between the motor functions and the intra-hemispheric rsFC of IPL in the unaffected side and PMd were parallelly demonstrated with behavioral changes. rsFC for the interhemispheric IPS and intrahemispheric IPL and PMd may be a possible regulatory factor for improving motor function and spasticity.
Data Availability Statement
All datasets generated for this study are included in the article/Supplementary Material.
Ethics Statement
The studies involving human participants were reviewed and approved by Shonan Keiiku Hospital Ethics Committee, 4360, Endo, Fujisawa, Kanagawa, Japan. The patients/participants provided their written informed consent to participate in this study.
Author Contributions
KS, KA, and ML recruited patients and medically charged patients’ conditions. FK managed the quality of clinical interventions and examinations. MY and MO collected the clinical examinations data and analyzed data of clinical examination and fMRI data. FK and KS conceived and designed the study. FK and partially all other authors wrote the article. KS, KA, and ML critically reviewed the manuscript.
Funding
This work was supported by the Japan Agency for Medical Research and Development (AMED) under the projects of Development of Medical Devices and Systems for Advanced Medical Services (Grant Number JP 16he0402255h0003), and Japan Society for the Promotion of Science (JSPS) KAKENHI Grant-in-Aid for Scientific Research on Innovative Areas “Understanding brain plasticity on body representations to promote their adaptive functions” (Grant Number JP 17H05909).
Conflict of Interest
ML and FK are the founding scientists of Connect Inc., a commercial company for the development of rehabilitation devices since May 2018 (ML) and October 2018 (FK). This company does not have any relationship with the device or setup used in the current study.
The remaining authors declare that the research was conducted in the absence of any commercial or financial relationships that could be construed as a potential conflict of interest.
Acknowledgments
We also like to express our gratitude to Dr. Norihiro Suzuki and Radiology Department of Shonan Keiiku Hospital for their all academic support and encouragement.
Abbreviations
KINVIS, Kinesthetic perceptional illusion by visual stimulation; TherEX, Therapeutic exercise; rsFC, Resting-state brain functional connectivity; fMRI, Functional magnetic resonance imaging; CIMT, Constraint-induced movement therapy; M1, Primary motor; S1, Somatosensory; BOLD, Blood-oxygen-level-dependent; ROIs, Regions of interest; TFO, Time from onset; U/L, Upper Limb; SIAS, Stroke impairment assessment set; NMES, Neuromuscular electrical stimulation; EDC, Extensor digitorum communis; FMA, Fugl-Meyer assessment; MAS, Modified ashworth scale; ARAT, Action research arm test; BBT, Box and Block Test; MAL, Motor activity log; AOU, Amount of use; QOM, Quality of movement; MNI, Montreal Neurological Institute; SMG, Supramarginal gyrus; FDR, False discovery-rate; IPL, Inferior parietal lobule; IPS, Intra parietal sulcus; LOC, Lateral occipital cortex; PMd, Dorsal premotor cortex; SMA, Supplementary motor area; SFG, Superior frontal gyrus; SPL, Superior parietal lobule.
Supplementary Material
The Supplementary Material for this article can be found online at: https://www.frontiersin.org/articles/10.3389/fnsys.2019.00076/full#supplementary-material.
References
Amemiya, K., and Naito, E. (2016). Importance of human right inferior frontoparietal network connected by inferior branch of superior longitudinal fasciculus tract in corporeal awareness of kinesthetic illusory movement. Cortex 78, 15–30. doi: 10.1016/j.cortex.2016.01.017
Aoyama, T., Kaneko, F., Hayami, T., and Shibata, E. (2012). The effects of kinesthetic illusory sensation induced by a visual stimulus on the corticomotor excitability of the leg muscles. Neurosci. Lett. 514, 106–109. doi: 10.1016/j.neulet.2012.02.069
Barros Galvão, S. C., Borba Costa dos Santos, R., Borba dos Santos, P., Cabral, M. E., and Monte-Silva, K. (2014). Efficacy of coupling repetitive transcranial magnetic stimulation and physical therapy to reduce upper-limb spasticity in patients with stroke: a randomized controlled trial. Arch. Phys. Med. Rehabil. 95, 222–229. doi: 10.1016/j.apmr.2013.10.023
Binkofski, F., Seitz, R. J., Arnold, S., Classen, J., Benecke, R., Freund, H.-J. (1996). Thalamic metabolism and corticospinal tract integrity determine motor recovery in stroke. Ann. Neurol. 39, 460–470. doi: 10.1002/ana.410390408
Botvinick, M. (2004). Probing the neural basis of body ownership. Science 305, 782–783. doi: 10.1126/science.1101836
Carda, S., Biasiucci, A., Maesani, A., Ionta, S., Moncharmont, J., Clarke, S., et al. (2017). Electrically assisted movement therapy in chronic stroke patients with severe upper limb paresis: a pilot, single-blind, randomized crossover study. Arch. Phys. Med. Rehabil. 98, 1628.e2–1635.e2. doi: 10.1016/j.apmr.2017.02.020
Carter, A. R., Astafiev, S. V., Lang, C. E., Connor, L. T., Rengachary, J., Strube, M. J., et al. (2010). Resting interhemispheric functional magnetic resonance imaging connectivity predicts performance after stroke. Ann. Neurol. 67, 365–375. doi: 10.1002/ana.21905
Carter, A. R., Patel, K. R., Astafiev, S. V., Snyder, A. Z., Rengachary, J., Strube, M. J., et al. (2012). Upstream dysfunction of somatomotor functional connectivity after corticospinal damage in stroke. Neurorehabil. Neural Repair 26, 7–19. doi: 10.1177/1545968311411054
Chen, J. J., Wu, Y. N., Huang, S. C., Lee, H. M., and Wang, Y. L. (2005). The use of a portable muscle tone measurement device to measure the effects of botulinum toxin type a on elbow flexor spasticity. Arch. Phys. Med. Rehabil. 86, 1655–1660. doi: 10.1016/j.apmr.2005.03.019
Chino, N., Sonoda, S., Domen, K., Saitoh, E., and Kimura, A. (1996). “Stroke impairment assessment set (SIAS),” in Functional Evaluation of Stroke Patients, eds N. Chino and J. L. Melvin (Tokyo: Springer), 19–31.
Colomer, C., Noé, E., and Llorens, R. (2016). Mirror therapy in chronic stroke survivors with severely impaired upper limb function: a randomized controlled trial. Eur. J. Phys. Rehabil. Med. 52, 271–278.
Crafton, K. R., Mark, A. N., and Cramer, S. C. (2003). Improved understanding of cortical injury by incorporating measures of functional anatomy. Brain 126, 1650–1659. doi: 10.1093/brain/awg159
De Bruyn, N., Meyer, S., Kessner, S. S., Essers, B., Cheng, B., Thomalla, G., et al. (2018). Functional network connectivity is altered in patients with upper limb somatosensory impairments in the acute phase post stroke: a cross-sectional study. PLoS One 13:e0205693. doi: 10.1371/journal.pone.0205693
Dodd, K. C., Nair, V. A., and Prabhakaran, V. (2017). Role of the contralesional vs. ipsilesional hemisphere in stroke recovery. Front. Hum. Neurosci. 11:469. doi: 10.3389/fnhum.2017.00469
Dohle, C., Püllen, J., Nakaten, A., Küst, J., Rietz, C., and Karbe, H. (2009). Mirror therapy promotes recovery from severe hemiparesis: a randomized controlled trial. Neurorehabil. Neural Repair 23, 209–217. doi: 10.1177/1545968308324786
Eklund, A., Nichols, T. E., and Knutsson, H. (2016). Cluster failure: why fMRI inferences for spatial extent have inflated false-positive rates. Proc. Natl. Acad. Sci. U S A 113, 7900–7905. doi: 10.1073/pnas.1602413113
Fan, Y. T., Wu, C. Y., Liu, H. I., Lin, K. C., Wai, Y. Y., and Chen, Y. L. (2015). Neuroplastic changes in resting-state functional connectivity after stroke rehabilitation. Front. Hum. Neurosci. 9:546. doi: 10.3389/fnhum.2015.00546
Francis, H. P., Wade, D. T., Turner-Stokes, L., Kingswell, R. S., Dott, C. S., and Coxon, E. A. (2004). Does reducing spasticity translate into functional benefit? An exploratory meta-analysis. J. Neurol. Neurosurg. Psychiatry 75, 1547–1551. doi: 10.1136/jnnp.2003.025551
Frías, I., Starrs, F., Gisiger, T., Minuk, J., Thiel, A., and Paquette, C. (2018). Interhemispheric connectivity of primary sensory cortex is associated with motor impairment after stroke. Sci. Rep. 8:12601. doi: 10.1038/s41598-018-29751-6
Gentile, G., Guterstam, A., Brozzoli, C., and Ehrsson, H. H. (2013). Disintegration of multisensory signals from the real hand reduces default limb self-attribution: an fMRI study. J. Neurosci. 33, 13350–13366. doi: 10.1523/jneurosci.1363-13.2013
Grimm, F., Naros, G., and Gharabaghi, A. (2016). Closed-loop task difficulty adaptation during virtual reality reach-to-grasp training assisted with an exoskeleton for stroke rehabilitation. Front. Neurosci. 10:518. doi: 10.3389/fnins.2016.00518
Johansen-Berg, H., Scholz, J., and Stagg, C. J. (2010). Relevance of structural brain connectivity to learning and recovery from stroke. Front. Syst. Neurosci. 4:146. doi: 10.3389/fnsys.2010.00146
Kaneko, F., Blanchard, C., Lebar, N., Nazarian, B., Kavounoudias, A., and Romaiguère, P. (2015). Brain regions associated to a kinesthetic illusion evoked by watching a video of one’s own moving hand. PLoS One 10:e0131970. doi: 10.1371/journal.pone.0131970
Kaneko, F., Inada, T., Matsuda, N., Shibata, E., and Koyama, S. (2016a). Acute effect of visually induced kinesthetic illusion in patients with stroke: a preliminary report. Int. J. Neurorehabil. 3:212. doi: 10.4172/2376-0281.1000212
Kaneko, F., Shibata, E., Hayami, T., Nagahata, K., and Aoyama, T. (2016b). The association of motor imagery and kinesthetic illusion prolongs the effect of transcranial direct current stimulation on corticospinal tract excitability. J. Neuroeng. Rehabil. 13:36. doi: 10.1186/s12984-016-0143-8
Kaneko, F., Takahashi, R., Shibata, E., and Itaguchi, Y. (2016c). “Kinesthetic illusion induced by pairing of visual and peripheral nerve stimulation causes sustained enhancement of corticospinal tract excitability,” in Society for Neuroscience 2016, San Diego, CA, 12–16.
Kaneko, F., Yasojima, T., and Kizuka, T. (2007). Kinesthetic illusory feeling induced by a finger movement movie effects on corticomotor excitability. Neuroscience 149, 976–984. doi: 10.1016/j.neuroscience.2007.07.028
Klamroth-Marganska, V., Blanco, J., Campen, K., Curt, A., Dietz, V., Ettlin, T., et al. (2014). Three-dimensional, task-specific robot therapy of the arm after stroke: a multicentre, parallel-group randomised trial. Lancet Neurol. 13, 159–166. doi: 10.1016/S1474-4422(13)70305-3
Kwakkel, G., Kollen, B. J., van der Grond, J., and Prevo, A. J. (2003). Probability of regaining dexterity in the flaccid upper limb: impact of severity of paresis and time since onset in acute stroke. Stroke 34, 2181–2186. doi: 10.1161/01.str.0000087172.16305.cd
Langhorne, P., and Legg, L. (2003). Evidence behind stroke rehabilitation. J. Neurol. Neurosurg. Psychiatry 74, iv18–iv21. doi: 10.1136/jnnp.74.suppl_4.iv18
Li, F., Wu, Y., and Li, X. (2014). Test-retest reliability and inter-rater reliability of the Modified Tardieu Scale and the Modified Ashworth Scale in hemiplegic patients with stroke. Eur. J. Phys. Rehabil. Med. 50, 9–15.
Marumoto, K., Koyama, T., Hosomi, M., Takebayashi, T., Hanada, K., Ikeda, S., et al. (2013). Diffusion tensor imaging predicts the outcome of constraint-induced movement therapy in chronic infarction patients with hemiplegia: a pilot study. Restor. Neurol. Neurosci. 31, 387–396. doi: 10.3233/RNN-120285
Mathiowetz, V., Volland, G., Kashman, N., and Weber, K. (1985). Adult norms for the Box and Block Test of manual dexterity. Am. J. Occup. Ther. 39, 386–391. doi: 10.5014/ajot.39.6.386
Mayka, M. A., Corcos, D. M., Leurgans, S. E., and Vaillancourt, D. E. (2006). Three-dimensional locations and boundaries of motor and premotor cortices as defined by functional brain imaging: a meta-analysis. Neuroimage 31, 1453–1474. doi: 10.1016/j.neuroimage.2006.02.004
Moulton, E., Amor-Sahli, M., Perlbarg, V., Pires, C., Crozier, S., Galanaud, D., et al. (2015). Axial diffusivity of the corona radiata at 24 hours post-stroke: a new biomarker for motor and global outcome. PLoS One 10:e0142910. doi: 10.1371/journal.pone.0142910
Naito, E., Morita, T., and Amemiya, K. (2016). Body representations in the human brain revealed by kinesthetic illusions and their essential contributions to motor control and corporeal awareness. Neurosci. Res. 104, 16–30. doi: 10.1016/j.neures.2015.10.013
Nowak, D. A., Grefkes, C., Manuel Dafotakis, M., Eickhoff, S., Küst, J., Hans Karbe, H., et al. (2008). Effects of low-frequency repetitive transcranial magnetic stimulation of the contralesional primary motor cortex on movement kinematics and neural activity in subcortical stroke. Arch. Neurol. 65, 741–747. doi: 10.1001/archneur.65.6.741
Oldfield, R. C. (1971). The assessment and analysis of handedness: the Edinburgh inventory. Neuropsychologia 9, 97–113. doi: 10.1016/0028-3932(71)90067-4
Page, S. J., Levine, P., and Leonard, A. (2007). Mental practice in chronic stroke results of a randomized, placebo-controlled trial. Stroke 38, 1293–1297. doi: 10.1161/01.str.0000260205.67348.2b
Platz, T., Eickhof, C., Van Kaick, S., Engel, U., Pinkowski, C., Kalok, S., et al. (2005). Impairment-oriented training or Bobath therapy for severe arm paresis after stroke: a single-blind, multicentre randomized controlled trial. Clin. Rehabil. 19, 714–724. doi: 10.1191/0269215505cr904oa
Pollock, A., Farmer, S. E., Brady, M. C., Langhorne, P., Mead, G. E., Mehrholz, J., et al. (2014). Interventions for improving upper limb function after stroke. Cochrane Database Syst. Rev. 12:CD10820. doi: 10.1002/14651858.CD010820.pub2
Ramos-Murguialday, A., Broetz, D., Rea, M., Läer, L., Yilmaz, Ö., Brasil, F. L., et al. (2013). Brain-machine interface in chronic stroke rehabilitation: a controlled study. Ann. Neurol. 74, 100–108. doi: 10.1002/ana.23879
Ramos-Murguialday, A., Curado, M. R., Broetz, D., Yilmaz, Ö., Brasil, F. L., Liberati, G., et al. (2019). Brain-machine interface in chronic stroke: randomized trial long-term follow-up. Neurorehabil. Neural Repair 33, 188–198. doi: 10.1177/1545968319827573
Saver, J. L., Johnston, K. C., Homer, D., Wityk, R., Koroshetz, W., Truskowski, L. L., et al. (1999). Infarct volume as a surrogate or auxiliary outcome measure in ischemic stroke clinical trials. Stroke 30, 293–298. doi: 10.1161/01.str.30.2.293
Schiemanck, S. K., Kwakkel, G., Post, M. W. M., Kappelle, L. J., and Prevo, A. J. H. (2008). Impact of internal capsule lesions on outcome of motor hand function at one year post-stroke. J. Rehabil. Med. 40, 96–101. doi: 10.2340/16501977-0130
Shelton, F. N. A. P., and Reding, M. J. (2001). Effect of lesion location on upper limb motor recovery after stroke. Stroke 32, 107–112. doi: 10.1161/01.str.32.1.107
Sonoda, S., Saitoh, E., Domen, K., Chino, N., and Kimura, A. (1993). Stroke impairment assessment set (SIAS). (3). Observation of motor recovery. Jpn. J. Rehabil. Med. 30, 273–278. doi: 10.2490/jjrm1963.30.315
Stinear, C. M., Barber, P. A., Coxon, J. P., Fleming, M. K., and Byblow, W. D. (2008). Priming the motor system enhances the effects of upper limb therapy in chronic stroke. Brain. 131, 1381–1390. doi: 10.1093/brain/awn051
Urbin, M. A., Hong, X., Lang, C. E., and Carter, A. R. (2014). Resting-state functional connectivity and its association with multiple domains of upper-extremity function in chronic stroke. Neurorehabil. Neural Repair 28, 761–769. doi: 10.1177/1545968314522349
Uswatte, G., Taub, E., Morris, D., Vignolo, M., and McCulloch, K. (2005). Reliability and validity of the upper-extremity Motor Activity Log-14 for measuring real-world arm use. Stroke 36, 2493–2496. doi: 10.1161/01.str.0000185928.90848.2e
Wang, L., Yu, C., Chen, H., Qin, W., He, Y., Fan, F., et al. (2010). Dynamic functional reorganization of the motor execution network after stroke. Brain 133, 1224–1238. doi: 10.1093/brain/awq043
Westlake, K. P., and Nagarajan, S. S. (2011). Functional connectivity in relation to motor performance and recovery after stroke. Front. Syst. Neurosci. 5:8. doi: 10.3389/fnsys.2011.00008
Whitfield-Gabrieli, S., and Nieto-Castanon, A. (2012). Conn: a functional connectivity toolbox for correlated and anticorrelated brain networks. Brain Connect. 2, 125–141. doi: 10.1089/brain.2012.0073
Wolf, S. L., Winstein, C. J., Miller, J. P., Taub, E., Uswatte, G., Morris, D., et al. (2006). Effect of constraint-induced movement therapy on upper extremity function 3 to 9 months after stroke: the EXCITE randomized clinical trial. JAMA 296, 2095–2104. doi: 10.1001/jama.296.17.2095
Zhang, Y., Li, K. S., Ning, Y. Z., Fu, C. H., Liu, H. W., Han, X., et al. (2016). Altered structural and functional connectivity between the bilateral primary motor cortex in unilateral subcortical stroke: a multimodal magnetic resonance imaging study. Medicine 95:e4534. doi: 10.1097/md.0000000000004534
Keywords: stroke, rehabilitation, kinesthetic illusion, virtual reality, body ownership, embodied visual feedback, resting-state brain functional connectivity, mirror visual feedback
Citation: Kaneko F, Shindo K, Yoneta M, Okawada M, Akaboshi K and Liu M (2019) A Case Series Clinical Trial of a Novel Approach Using Augmented Reality That Inspires Self-body Cognition in Patients With Stroke: Effects on Motor Function and Resting-State Brain Functional Connectivity. Front. Syst. Neurosci. 13:76. doi: 10.3389/fnsys.2019.00076
Received: 31 July 2019; Accepted: 27 November 2019;
Published: 17 December 2019.
Edited by:
James W. Grau, Texas A&M University, United StatesReviewed by:
Soha Saleh, Kessler Foundation, United StatesBirgitta Langhammer, OsloMet—Oslo Metropolitan University, Norway
Copyright © 2019 Kaneko, Shindo, Yoneta, Okawada, Akaboshi and Liu. This is an open-access article distributed under the terms of the Creative Commons Attribution License (CC BY). The use, distribution or reproduction in other forums is permitted, provided the original author(s) and the copyright owner(s) are credited and that the original publication in this journal is cited, in accordance with accepted academic practice. No use, distribution or reproduction is permitted which does not comply with these terms.
*Correspondence: Fuminari Kaneko, Zi1rYW5la29Aa2Vpby5qcA==