- 1Section on Craniofacial Genetic Disorders, Eunice Kennedy Shriver National Institute of Child Health and Human Development (NICHD), National Institutes of Health (NIH), Bethesda, MD, United States
- 2Department of Biomedical Engineering, University of Utah, Salt Lake City, UT, United States
- 3School of Dentistry, University of Alberta, Edmonton, AB, Canada
- 4Molecular Genomics Core, Eunice Kennedy Shriver National Institute of Child Health and Human Development (NICHD), National Institutes of Health (NIH), Bethesda, MD, United States
- 5Bioinformatics and Scientific Programming Core, Eunice Kennedy Shriver National Institute of Child Health and Human Development (NICHD), National Institutes of Health (NIH), Bethesda, MD, United States
Mouse and human genetic studies indicate key roles of the Wnt10a ligand in odontogenesis. Previous studies have identified effectors and regulators of the Wnt signaling pathway actively expressed during key stages of tooth morphogenesis. However, limitations in multiplexing and spatial resolution hindered a more comprehensive analysis of these signaling molecules. Here, profiling of transcriptomes using fluorescent multiplex in situ hybridization and single-cell RNA-sequencing (scRNA-seq) provide robust insight into the synchronized expression patterns of Wnt10a, Dkk1, and Sost simultaneously during tooth development. First, we identified Wnt10a transcripts restricted to the epithelium at the stage of tooth bud morphogenesis, contrasting that of Sost and Dkk1 localization to the dental mesenchyme. By embryonic day 15.5 (E15.5), a marked shift of Wnt10a expression from dental epithelium to mesenchyme was noted, while Sost and Dkk1 expression remained enriched in the mesenchyme. By postnatal day 0 (P0), co-localization patterns of Wnt10a, Dkk1, and Sost were observed in both terminally differentiating and secreting odontoblasts of molars and incisors. Interestingly, Wnt10a exhibited robust expression in fully differentiated ameloblasts at the developing cusp tip of both molars and incisors, an observation not previously noted in prior studies. At P7 and 14, after the mineralization of dentin and enamel, Wnt10a expression was limited to odontoblasts. Meanwhile, Wnt modulators showed reduced or absent signals in molars. In contrast, strong signals persisted in ameloblasts (for Wnt10a) and odontoblasts (for Wnt10a, Sost, and Dkk1) towards the proximal end of incisors, near the cervical loop. Our scRNA-seq analysis used CellChat to further contextualize Wnt pathway-mediated communication between cells by examining ligand-receptor interactions among different clusters. The co-localization pattern of Wnt10a, Dkk1, and Sost in both terminally differentiating and secreting odontoblasts of molars and incisors potentially signifies the crucial ligand-modulator interaction along the gradient of cytodifferentiation starting from each cusp tip towards the apical region. These data provide cell type-specific insight into the role of Wnt ligands and mediators during epithelial-mesenchymal interactions in odontogenesis.
Introduction
Wnt signaling provides critical cues in many processes of embryogenesis, including organ development, tissue homeostasis, and wound repair (Tamura and Nemoto, 2016). Several Wnt signaling components, including Wnt ligands, receptors, transducers, transcription factors, and antagonists, are expressed in the dental epithelium and mesenchyme during tooth development in humans and mice (Kornsuthisopon et al., 2022). Following patterning morphogenesis, Wnt10a is strongly expressed in pre- and fully-differentiated odontoblasts (Yamashiro et al., 2007), with expression levels maintained in odontoblasts adjacent to Hertwig’s epithelial root sheath (HERS). Wnt10a deficiency causes enamel hypoplasia, leading to a flattened crown and taurodontic roots in mice and humans (Yang et al., 2015).
Several extracellular Wnt antagonists (Malinauskas and Jones, 2014) expressed in the tooth organ either bind directly to or compete with Wnt ligands for binding to the co-receptors, Lrp5 and Lrp6. Dickkopf (Dkk) and Sclerostin (Sost) proteins are secreted Wnt antagonists that block the binding of Wnt ligands to Lrp5 and Lrp6 receptors. Inhibition of epithelial Wnt/β-catenin through Dkk1 overexpression arrests tooth morphogenesis at early or late bud stage (Han et al., 2011). A previous study reported that Sost expression was restricted to odontoblasts at the dentin matrix-secreting stages (Naka and Yokose, 2011). Sost overexpression in human pulp-derived odontoblast-like cells inhibited odontogenic differentiation (Liao et al., 2019). Nevertheless, mice lacking the Sost gene exhibit alterations only in periodontal bone and cementum, with no other abnormalities observed in tooth structure (Kuchler et al., 2014).
Wnt agonist therapies have been studied to stimulate Wnt signaling for the propagation of dentin-pulp regeneration (Neves et al., 2017; Ali et al., 2019; Birjandi et al., 2020). Mice lacking the Sost gene show significantly improved pulp healing following injury (Collignon et al., 2017). Inhibition of DKK1 and SOST using a bispecific antibody has shown superior bone repair activity to that of monotherapy (Florio et al., 2016). Modulating components of the Wnt signaling pathway holds great promise in the field of tooth regeneration. Therefore, it is imperative to gain a comprehensive understanding of the spatiotemporal expression patterns during odontogenesis in embryonic and early postnatal developmental stages. The primary aim of this study is to conduct a robust spatiotemporal profiling of Wnt mediators, specifically Sost and Dkk1, along with the Wnt effector ligand, Wnt10a, during odontogenesis, utilizing multiplex, high spatial resolution technology. Furthermore, our study aims to explore if the expression pattern of these three genes differs between non-growing molars and continuously self-renewing incisors. Profiling of transcriptomes using fluorescent multiplex in situ hybridization and single-cell RNA-sequencing (scRNA-seq) provides a distinctive insight into the synchronized expression pattern of Wnt10a, Dkk1, and Sost during tooth development not previously conducted.
Materials and methods
Animals and embryo collection
All animal procedures were approved by the National Institutes of Health, National Institute of Child Health and Human Development Animal Care and Use Committee (ACUC), under Animal Study Protocol #21-031. Mice were housed in a temperature-controlled room under artificial lighting with access to food and water ad libitum. Healthy fertile male mice were mated with the same strain C57BL/6J female mice to generate embryos and pups at different developmental stages. Embryonic day 0.5 (E 0.5) was identified as the day on which the presence of a vaginal plug was confirmed. Six embryonic and four postnatal stages, regardless of their sex, at each developmental stage (E12.5, E13.5, E14.5, E15.5, E17.5, E18.5, postnatal day 0 (P0), P1, P7, P14 (n = 3 biological replicates and n = 3 technical replicates in each, Total number of pregnant mice used = 30) were used in this study. Mice were euthanized by an overdose of carbon dioxide followed by cervical dislocation.
Histology preparation
E12.5 to E18.5 embryos, and P0/P1, P7, P14 (n = 3) mice pups were used for morphological observation using hematoxylin and eosin (HE) staining. Whole embryo/pup heads were fixed in 10% neutral buffered formalin (NBF) (Azer Scientific, Cat No. NAF-4-G) for 24 h and dehydrated and processed, paraffin-embedded, and serially sectioned (4.5 μm) in the coronal (molar) and sagittal (incisor) plane. Stages at P0 or later were decalcified using EDTA (0.5M, pH 8.0, Quality Biological, Cat No. 351-027-101). The sections were deparaffinized, rehydrated, stained with H&E, and imaged on an AxioScan.Z1 slide scanner (Zeiss).
Fluorescent multiplex mRNA in situ hybridization (RNAscope)
E12.5 to E18.5 embryos, and P0/P1, P7, P14 mice (n = 3) were used for RNAscope analysis. RNAscope Probe-Mm-Wnt10a, Sost, Dkk1 (Advanced Cell Diagnostics; ACD, 401061, 410031, 402521) were designed. RNAscope® Multiplex Fluorescent Detection Kit v2 (ACD, 323110) was used to explore the expression pattern of Wnt10a, Sost, and Dkk1 following the manufacturer’s protocol. RNAscope slides were imaged on an AxioScan.Z1 slide scanner (Zeiss) with Plan-apochromat 40x/0.95 objective in four fluorescent channels (DAPI, Cy3, Cy5, Cy7).
Tooth organ dissection, single cell dissociation
E15.5 embryos and P0/P1 mice pups, n = 1 (collected and pooled 6 tooth organs in 1 sample) were used for single-cell dissociation. Mice embryos/pups were dissected out and transferred into ice-cold phosphate-buffered saline (PBS) in a 10 cm petri dish. Tooth organs were carefully dissected (maxillary and mandibular molars) of each respective stage and were collected in a 1.5 mL tube with 1X PBS with 0.04% bovine serum albumin (BSA) on ice. Samples were collected from mice embryos/pups in each respective age group. Harvested tooth organs were cut into small pieces in ice-cold 1X PBS with 0.04% BSA on ice. For isolating the single cells, tissue pellets were then incubated in 1 mL of TrypLE express enzyme (Thermo Fisher Scientific, Waltham, MA) for 30 min at 37 °C. Cells were collected by passing the suspension through a cell strainer, followed by centrifugation.
Single-cell RNA-sequencing (scRNA-seq)
Libraries from isolated cells were prepared using the Chromium Next Single Cell 3’ Reagent Kit v3.1 (Dual Index −10x Genomics—protocol CG000315 Rev C), aiming for a total of 8,000 cells per sample. In brief, cell suspensions were loaded onto the Chromium Controller microfluidic device to be partitioned into bar code-containing Gel Bead-In-Emulsions (GEMs). Samples were incubated, and poly-adenylated mRNAs were reverse-transcribed into cDNAs containing a unique molecular identifier (UMI) and a cell barcode unique to each GEM. The GEMs were broken using a recovery agent to produce a bulk pool of barcoded molecules. Additional amplification of the cDNA was performed before proceeding to fragmentation, end repair, A-tailing, and ligation. A sample dual index PCR was performed in order to add the P5, i5, i7, and P7 sequences to the final scRNA-seq library. Finally, samples were pooled, and the library was sequenced using the Illumina NovaSeq 6,000 with the SP reagent Kit v.1.5, resulting in 28 × 90 bp paired-end reads.
Bioinformatics analysis
Cell Ranger (10X Genomics) was used to process reads into a barcode x count matrix by aligning to the mm10 mouse assembly and using the refdata-gex-mm10-2020-A annotation provided by 10X Genomics. This was performed for each of the samples (P1 mandibular molar, P1 maxillary molar, E15.5 mandibular molar, E15.5 maxillary molar). In total, ∼110,000 cells across all samples, ranging from 7,379 to 20,076 per sample, were obtained from Cell Ranger using its default filtering. We further used the scDblFinder package (McGinnis et al., 2019) with default parameters to identify and remove doublets from analysis in each of the samples. Cells were further filtered based on the percentage of counts associated with mitochondrial genes and the number of detected genes per cell, and we removed cells >3 median absolute deviations (MADs) from the median of the total population using the scuttle package (McCarthy et al., 2017). This resulted in 32,288 cells total (ranging from 1,545 to 6,737 cells per sample). Data normalization, scaling, dimensionality reduction, highly variable feature detection, population sub-setting, data integration, and marker detection were all performed with the Seurat v4 (Hao 2021) package based on the standard workflow. Specifically, the steps were the following: normalization with SCTransform (Hafemeister and Satija, 2019; Choudhary and Satija, 2022) on each sample independently using the glmGamPoi method; integration of all samples using SelectIntegrationFeatures with 3,000 features and FindIntegrationAnchors with cca reduction and 5 anchor features; clustering on 40 principal components with a resolution of 1.0; and FindMarkers using Wilcoxon test. Cell types were assigned post-analysis to clusters after inspection of the marker genes. Cells identified as immune response, muscle, endothelial cells, neural, and myeloid cells were then removed, and the remaining cells were clustered and annotated using the same methods and parameters as above.
Cluster identities were assigned based on previously published gene markers (Chiba et al., 2020; Krivanek et al., 2020; Hermans et al., 2022; Jing et al., 2022) (Supplementary Figure S6).
CellChat analysis
New Seurat objects were generated, filtered, and clustered using the PopsicleR package for the E15.5 and P1 maxillary molar datasets (Grandi et al., 2022). We followed the standard workflow, filtering out cells with less than 200 features and more than 6,500 (E15.5) or 7,500 (P1), >20% (E15.5) or 25% (P1) mitochondrial, and >50% (E15.5) or 45% (P1) ribosomal genes. Doublets were removed at a threshold of 0.35 (E15.5) or 0.38 (P1). Normalization was performed using default values, followed by regression based on cell cycle genes. We then clustered the dataset at 0.6 resolution and annotated clusters as described above.
Individual CellChat objects were generated from the resultant Seurat objects and analyzed following the single dataset analysis tutorial for each (Jin et al., 2021). Querying the WNT signaling pathway network, we generated several plots to visualize predicted interactions within and between annotated clusters. For both E15.5 and P1 maxillary molar datasets, we generated circle plots and chord diagrams to visualize cellular communication and scatter plots to identify signaling roles for cells using network centrality analysis, focused on the WNT signaling pathway network only.
Results
Wnt10a is expressed in dental epithelium while Wnt modulators, Dkk1 and Sost, are expressed in the dental mesenchyme during the early stages of tooth development
To confirm the morphological features of the molar and incisor tooth organ at each developmental time point, we performed H&E staining of Formalin-Fixed Paraffin-Embedded (FFPE) embryo head specimens. During the initiation of tooth development [E12.5], a localized thickening of the oral epithelium is visualized in the presumptive molariform field (Supplementary Figure S1). To evaluate the spatiotemporal mRNA expression of Wnt10a, Sost, and Dkk1 in the molar and incisor tooth organ we performed RNAscope at each developmental time point using FFPE tissue. Wnt10a transcripts were detected in the oral and presumptive dental epithelium but were absent in the mesenchyme of maxillary and mandibular molars (Supplementary Figure S1). Dkk1 was expressed in the mesenchyme of molars, and Sost expression was minimal or absent (Supplementary Figure S1). Similar expression patterns were observed in the incisors (Supplementary Figure S2).
By E13.5, the epithelial bud had developed by invaginating into the condensed mesenchyme. The enamel knot (EK) started to appear in first molars at this stage (Figure 1A, Supplementary Figure S3A). Wnt10a transcripts were detected throughout the molar bud epithelium with the strongest expression in the EK (Figure 1A1, Supplementary Figure S3B). In the dental epithelium, stronger expression was observed towards the buccal side (red arrowhead in Figure 1A1, Supplementary Figure S3B), while Sost and Dkk1 were expressed within the mesenchyme (Figure 1A2, A3, Supplementary Figure S3C, D). At this stage, we found relatively intense expression of Wnt10a along the labial border of the epithelial invagination in incisors (white arrow in Supplementary Figure S2).
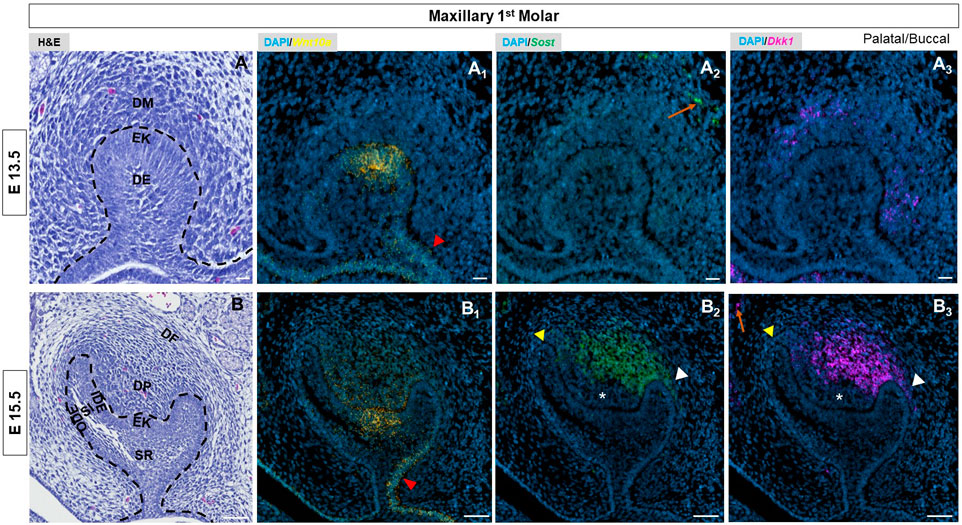
FIGURE 1. (A, B) E13.5 & E15.5 maxillary first molar tooth organs of wild type mice were analyzed using hematoxylin and eosin (HE) staining. RNAscope in-situ hybridization of Wnt10a, Sost and Dkk1 with positive staining in (A1, B1) yellow dots (A2, B2) green dots and (A3, B3) violet dots respectively, on coronal sections of the maxillary first molar tooth organ from wild-type mice at E13.5 & E15.5. The red arrowhead indicates stronger expression of Wnt10a towards the buccal side of the dental epithelium. Yellow arrowhead shows weaker signals of Sost and Dkk1 towards the longer palatal cervical loop. White arrowhead shows strong signals of Sost and Dkk1 towards a shorter buccal cervical loop. The absence of Sost and Dkk1 from the coronal papilla area adjacent to the EK is indicated by a white asterisk. Enriched expression of Sost and Dkk1 along the future alveolar bone regions around the tooth organ is marked by an orange arrow. Scale bar, (A, A1-3) 20 µm (B, B1-3) 50 µm. DE-Dental epithelium, DM-Dental mesenchyme, EK-Enamel knot, OD-Odontoblast, AM-Ameloblast, SR-Stellate reticulum, SI-Stellate Intermedium, IDE-Inner dental epithelium, ODE- Outer dental epithelium, DP-Dental papilla, DF-Dental follicle.
At the cap stage of molars, [E14.5] (Supplementary Figure S1) Wnt10a was exclusively found in the EK area with a slightly stronger signal along the buccal side (Supplementary Figure S1). Enriched expression of both Sost and Dkk1 was observed in the dental papilla (DP) mesenchyme with a noted absence from the coronal papilla area adjacent to the EK (white asterisk in Supplementary Figure S1). A weaker signal of Sost and Dkk1 was noted surrounding the longer palatal cervical loop (CL) (yellow arrowhead) compared to the shorter buccal CL of the maxillary molar (white arrowhead in Supplementary Figure S1).
Wnt10a expression shifts from the epithelium to mesenchyme
At E15.5, the molars and incisors have started to develop into a bell shape (Figure 1B, Supplementary Figure S2, Supplementary Figure S3E). During this stage, Wnt10a expression had begun shifting to surrounding mesenchymal cells adjacent to the EK (Figure 1B1, Supplementary Figure S3F). We observed a diffuse expression pattern of Wnt10a within the EK region. In addition, Wnt10a expression was noted in the palatal/lingual region of dental epithelium along with intense expression in the buccal dental epithelium of molars (red arrowhead in Figure 1B1, Supplementary Figure S3F). Sost and Dkk1 transcripts in molars did not change from E14.5. The concurrent expression of Sost and Dkk1 was absent in the coronal papilla adjacent to the EK (white asterisk in Figure 1B2, B3, Supplementary Figure S3G, H). A weaker expression of Sost and Dkk1 around the longer palatal CL (yellow arrowhead) compared to the shorter buccal side of molars (white arrowhead) was noted (Figure 1B2, B3, Supplementary Figure S3G, H). In incisors, Sost and Dkk1 were expressed in the mesenchyme closer to the EK region (Supplementary Figure S2). Sagittal sections of molars at E16.5 and E17.5 showed patchy expression patterns of Wnt mediators in the DP mesenchyme, with noted absence in areas with intense Wnt10a transcripts (Supplementary Figure S5).
Wnt10a and wnt modulators are co-expressed during odontoblast differentiation and dentinogenesis
At E17.5 and 18.5 - during the late bell stage (Supplementary Figure S1), (Figure 2A, Supplementary Figure S4A),- Wnt10a expression was strongly localized to the inner dental epithelium (IDE) and terminally-differentiating odontoblasts corresponding to the future developing cusps of the maxillary molar (Figure 2A1, Supplementary Figure S1). Sost and Dkk1 transcripts in the maxillary molars remained consistent in strength and localization to previous stages (Supplementary Figure S1), (Figure 2A2, A3). In the mandibular molar, maxillary, and mandibular incisors, Wnt10a transcription corresponded to the gradient of terminally differentiating ameloblasts and odontoblasts at the cusp tip regions (Supplementary Figure S2; Supplementary Figure S4). At E17.5, Sost and Dkk1 transcripts were detected strongly throughout the DP of mandibular molars (Supplementary Figure S1). In the incisors at E17.5 and mandibular molars at E18.5, both Wnt mediators were localized to the pre-odontoblasts and terminally-differentiating odontoblasts at the developing cusp tips (Supplementary Figure S2; Supplementary Figure S4C, D).
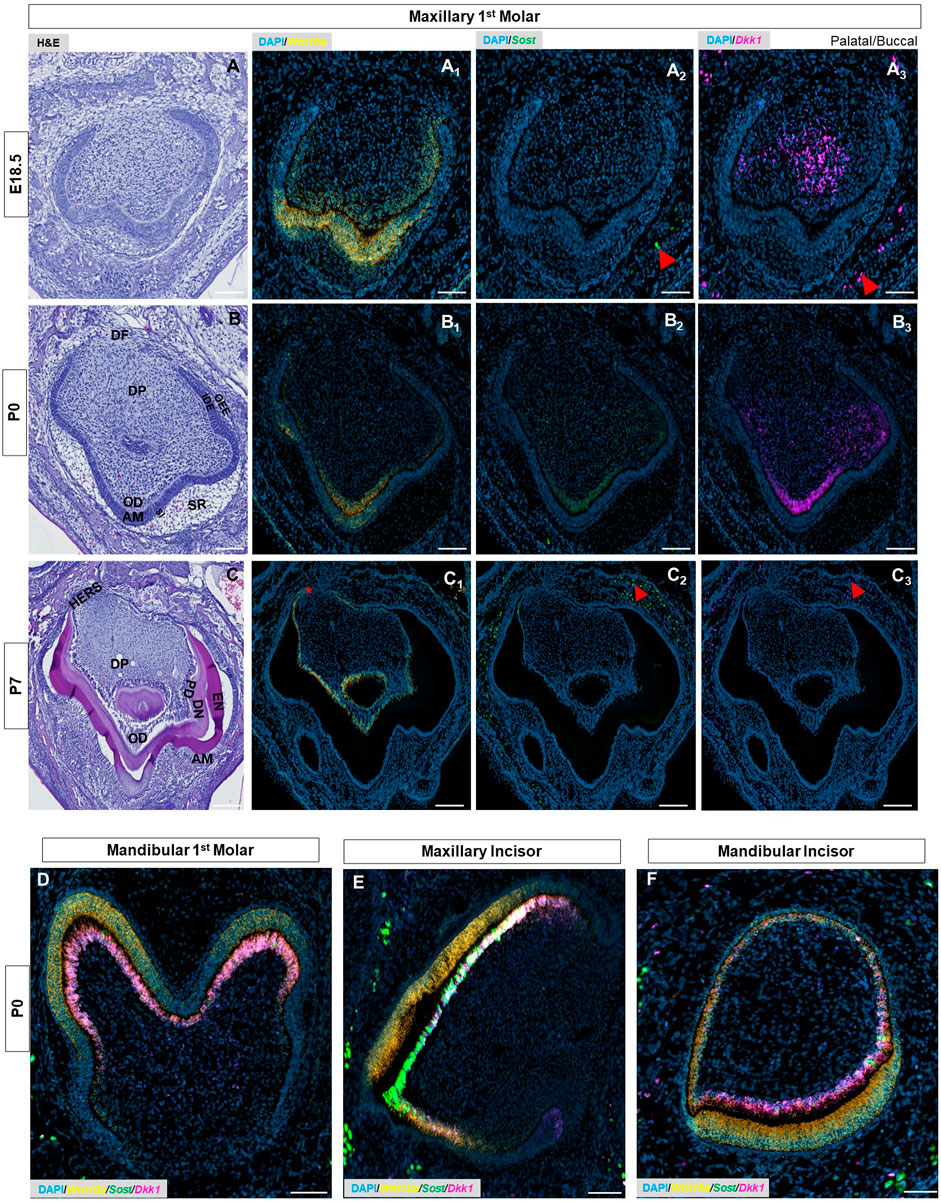
FIGURE 2. (A–C) E18.5, P0 & P7 maxillary first molar tooth organs of wild-type mice were analyzed using hematoxylin and eosin (HE) staining. RNAscope in-situ hybridization of Wnt10a, Sost, and Dkk1 with positive staining in (A1-C1) yellow dots (A2-C2) green dots, and (A3-C3) violet dots respectively, on coronal sections of the maxillary first molar tooth organ from wild-type mice at E18.5, P0 & P7. (D–F) Merged RNAscope in-situ hybridization of Wnt10a, Sost, and Dkk1 in coronal sections of the mandibular first molar, maxillary and mandibular incisor tooth organ from wild-type mice at P0. The red asterisk shows a strong signal of Wnt10a in Elongated HERS. Red arrowhead shows strong signals of Sost and Dkk1 within the osteocytes of developing bone. Scale bar, 100 µm. OD-Odontoblast, AM-Ameloblast, SR-Stellate reticulum, SI-Stellate intermedium, IDE-Inner dental epithelium, ODE- Outer dental epithelium, DP-Dental papilla, DF-Dental follicle, EN-Enamel, DN-Dentin, PD-Pre-dentin, HERS-Hertwig’s epithelial root sheath.
At P0, secretory odontoblasts were aligned perpendicular to the basement membrane (Figure 2B, Supplementary Figure S4E), and deposited predentin was evident in mandibular molars (black asterisk in Supplementary Figure S4E). Enamel formation was observed at the labial surface of the mandibular incisors (yellow arrowhead in Supplementary Figure S6). Sost and Dkk1 were strongly localized along the secretory odontoblast layer of molars and incisors (Figure 2B2, B3, Supplementary Figure S4G,H), (Supplementary Figure S6). Wnt10a was expressed intensely by the terminally differentiated ameloblasts and odontoblasts along the developing cusp tip of molars and incisors (Figure 2B1), (Supplementary Figure S6). Weaker Wnt10a expression was noted in the ameloblasts located at the occlusal groove of mandibular molars (red arrow in Supplementary Figure S4F). Likewise, diminished Wnt10a, Sost, and Dkk1 expression was noted in odontoblasts located at the occlusal groove region (white arrow in Supplementary Figure S4F-H). This multiplex in situ analysis at P0 gives us a clear picture of the co-localization pattern of Wnt10a, Dkk1, and Sost in terminally differentiating and secretary odontoblasts of molars and incisors (Figures 2D–F).
Wnt10a transcripts localized to a single odontoblast layer and wnt modulator gene expression decreases following mineralization of enamel and dentin in the tooth organ
Advanced mineralization of enamel and dentin occurs in molars and incisors by P7 (Figure 2C, Supplementary Figure S4I), (Figure 3A). Wnt10a transcripts were primarily localized to odontoblasts along with a weaker signal in the reduced dental epithelium (RDE) (Figure 2C1, Supplementary Figure S4J). Elongated HERS in molars showed a strong signal of Wnt10a (red asterisk in Figure 2C1, Supplementary Figure S4J). Lower Sost expression was exclusively present in some areas of the RDE with a few punctate signals of Sost and Dkk1 observed in odontoblasts near the molar HERS (Figure 2C2, C3, Supplementary Figure S4K, L). Maxillary incisors showed a strong expression of Wnt10a in ameloblasts and odontoblasts along the proximal end of the CL (red arrowhead in Figure 3A1, A2). Intense signals of Sost and Dkk1 in the odontoblast layer toward the labial CL of maxillary incisors at P7 (yellow and white arrowhead in Figure 3A3-A6). At P14 we observed weak Wnt10a expression in odontoblasts and neither Sost nor Dkk1 was found in molars (Supplementary Figure S7). At P14, intense signals of Wnt10a were continuously present in ameloblasts and odontoblasts towards the proximal end of incisors near the CL (red arrowhead in Figure 3B1, B2). We observed an abundance of transcripts of Sost and Dkk1 in proximal odontoblasts near the CL in incisors (yellow and white arrowhead in Figure 3B3, B6).
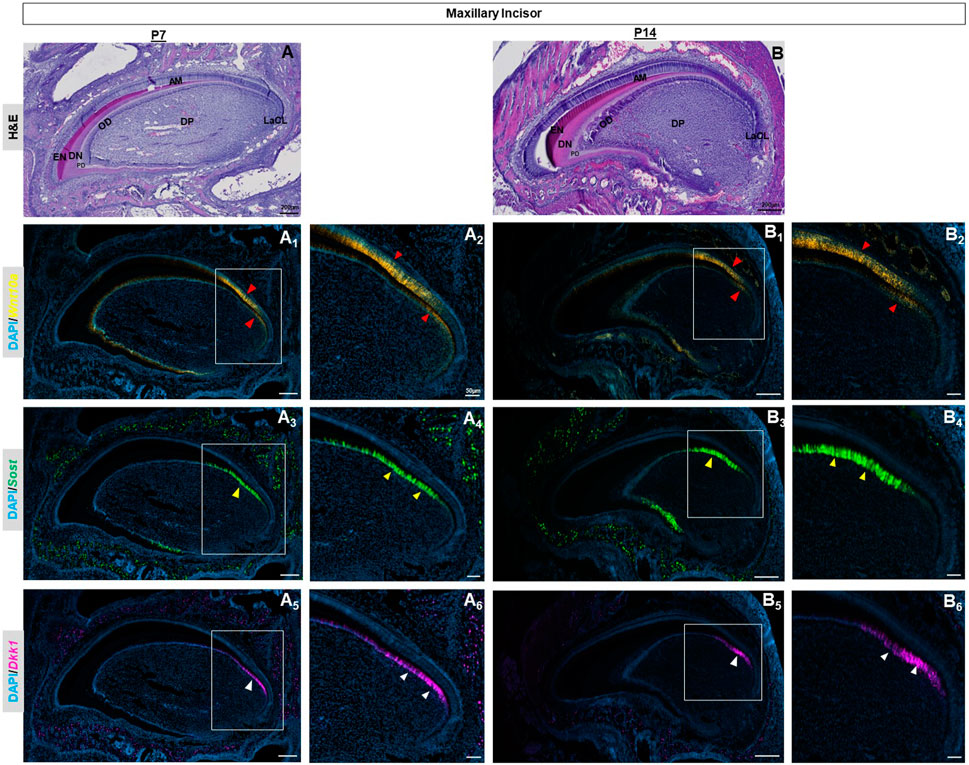
FIGURE 3. P7 & P14 maxillary incisor tooth organs of wild-type mice were analyzed using hematoxylin and eosin (HE) staining (A, B). RNAscope in-situ hybridization of Wnt10a, Sost and Dkk1 with positive staining in yellow dots (A1, A2, B1, B2) green dots (A3, A4, B3, B4), and violet dots (A5, A6, B5, B6) respectively, on sagittal sections of the maxillary incisor tooth organ from wild-type mice at P7 & P14. Intense signals of Wnt10a in ameloblasts and odontoblasts towards the proximal end of incisors near the CL are shown by the red arrowhead. The abundance of transcripts of Sost and Dkk1 in proximal odontoblasts near the CL in incisors is marked by yellow and white arrowheads respectively. Scale bar, 200 µm (A, B, A1,3,5, B1,3,5), 50 µm (A2,4,6, B2,4,6), OD-Odontoblast, AM-Ameloblast, DP-Dental papilla, EN-Enamel, DN-Dentin, PD-Pre-dentin, DP-Dental papilla, LaCL-Labial cervical loop.
Enriched expression of Sost and Dkk1 was also detected along the future alveolar bone regions around the tooth organ from E12.5 to E15.5 (orange arrow in Figure 1, Supplementary Figure S1-3). During later stages, intense expression of Sost and Dkk1 was found within the osteocytes of developing bone (red arrowhead in Figure 2, Supplementary Figure S4). Dkk1 and Sost expression generally overlapped in expression patterns, and close spatial association was observed throughout development from E14.5 to P0 (Figure 4).
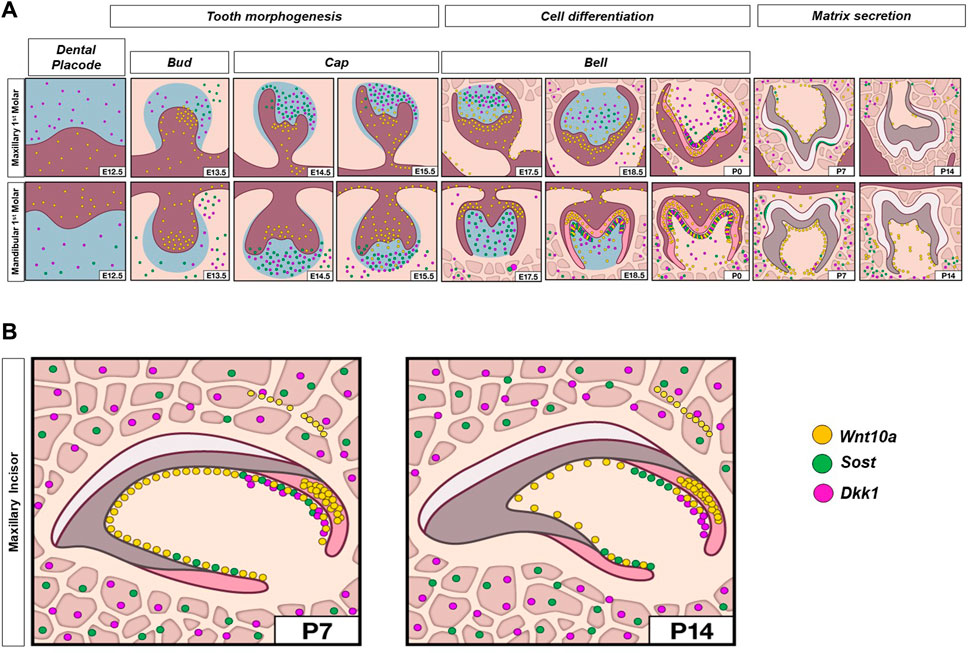
FIGURE 4. Schematic representation of spatiotemporal expression patterns of Wnt10a, Sost, and Dkk1 with positive staining in yellow dots, green dots, and violet dots respectively, on coronal sections of the maxillary and mandibular first molar (A), and sagittal sections of maxillary incisor tooth organ (B) of wild-type mice at different developmental stages.
To profile Wnt signaling genes using single-cell RNA sequencing (scRNA-seq) we first collected the maxillary tooth organ from E15.5 and P1 stages, isolated single cells, and sequenced individual cell transcriptomes. Following this we identified clusters containing dental cell populations in E15.5 and P1 maxillary molars (Figure 5A, A’). Consistent with our RNAscope data, at E15.5, Wnt10a was expressed by epithelial-mesenchymal cells and SR/SI. By P1, Wnt10a expression was concentrated in odontoblast and ameloblasts. Dkk1 was absent from epithelial cells at E15.5 and in the ameloblasts at P1 (Figure 5B). Furthermore, we profiled commonly studied Wnt ligands, intermediates, and receptors known to play a role in tooth development. Wnt4 and Wnt5b expression was restricted to the epithelium, while Wnt11 and mediators Dkk1, Dkk2, and Dkk3 were found only in mesenchyme at both stages. In contrast, Wnt5a and Sostdc1 were detected in both epithelial and mesenchymal cells at both stages. Along with Wnt10a, Wnt6 was associated only with epithelium at E15.5 and both epithelium and mesenchyme at P1 (Supplementary Figure S8, 9).
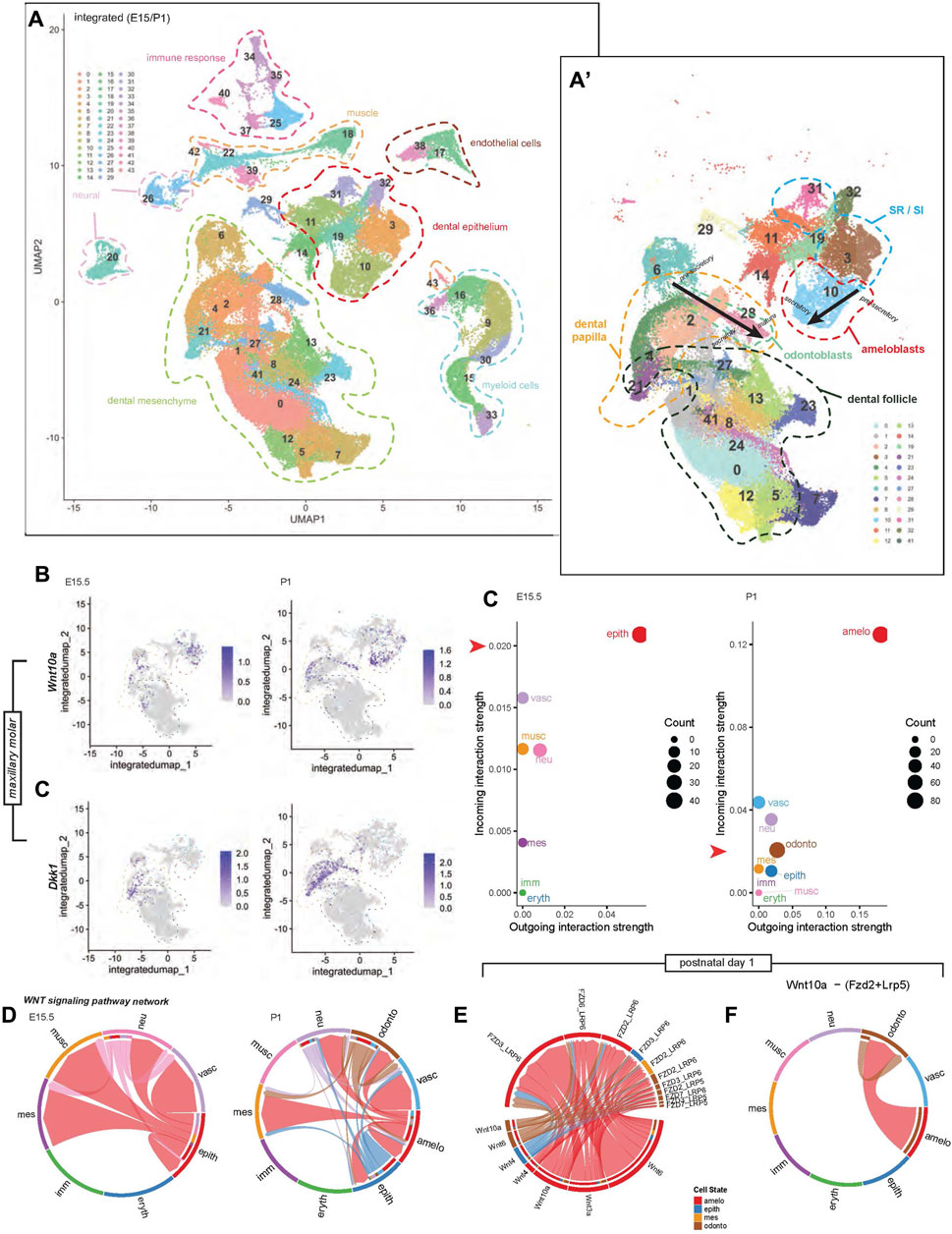
FIGURE 5. Integrated single-cell transcriptomic profile of murine maxillary first molars at developmental stages E15.5 and P1. (A) Uniform manifold approximation and projection (UMAP) highlights 44 unique cell cluster identities. (A′) Sub-clustering of only tooth-specific cell identities enables higher-resolution distinction between key cell types and lineage states; black arrows indicate maturation projection, from pre-secretory, secretory, to mature odontoblasts and ameloblasts. (B) Stage-specific feature plots of Wnt10a and Dkk1 expression indicate dynamic spatiotemporal cell cluster enrichment in different cell populations. Low-dimensional depiction of cell clusters in an incoming-outgoing signaling strength at E15.5 and P1 using CellChat. The red arrow along the y-axis of each scatter plot aligns with 0.02 on each plot, highlighting the difference in scale of the incoming interaction strength between plots. (C) Similarly, the scale of outgoing interaction strength has a greater range in the P1 plot compared with E15.5. (D) Chord diagrams representing the Wnt signaling pathway network between clusters at E15.5 and P1 reconstructed with CellChat. (E) Chord diagrams plotting the strength of Wnt signaling between Wnt ligands and receptors at P1. (F) Chord diagrams show Wnt10a-(Fzd2+ Lrp6) signaling strength outgoing from ameloblasts and odontoblasts to other cell clusters at P1. Additional colored bars in the inner concentric circles of panels (D–F) indicate cell types targeted by outgoing signaling, as these plots are directional. Chord width indicates the strength of flow between the cell types represented in each chord plot. Colors of chord plot links and scatter plot points are coded according to labels along the outside border of the E15.5 and P1 plots in (D), indicating the identity of the outgoing signal. Note: The color corresponding to each cell type is not identical between E15.5 and P1. An example interpretation of panel (D) is as follows: At P1, the red link connecting the ameloblast cluster to the odontoblast cluster represents ligands produced by ameloblasts that have known receptors in odontoblasts. The absence of outgoing links from the blue vascular cluster shows that it is not regulating anything but is regulated by other cells (mostly by ameloblasts). However, the ameloblast cluster regulates much more than just vasculature, with the majority of its regulation coming from itself. The abundance of red links in this chord plot overall indicates that the ameloblast cluster is doing the majority of the regulation, and dominates the incoming signal of all types, including to itself.
Finally, we used the CellChat tool to predict the contribution of the different cell types to cell–cell Wnt pathway signaling at a lower clustering resolution, differentiating between odontoblast, ameloblast, and other clusters at P1 only. At E15.5, the epithelial cluster is the predominant signal sender and receiver, interacting with the mesenchymal cluster. At P1, the ameloblast-containing cluster had the strongest outgoing and incoming interactions with the odontoblast cluster (Figure 5C). Dental cell-cell interactions at E15.5 were mainly between epithelium-epithelium and epithelium-mesenchyme. At P1, diversification of predicted inter-cluster interactions was reflected by Wnt pathway connections within the ameloblast cluster, between ameloblast-odontoblast, ameloblast-epithelium, and ameloblast-mesenchyme clusters (Figure 5D). At P1, notable Wnt signaling genes associated with ameloblasts and odontoblasts were Wnt6, Wnt10a, Wnt3a, Wnt4, and the corresponding receptors, shown in Figure 5E. The strongest predicted Wnt pathway interaction in our dataset was that of Wnt10a-Frz2-Lrp5, which was found within odontoblast and between ameloblast-odontoblast clusters (Figure 5F).
Discussion
The present study provides unique insight into the spatiotemporal relationship of Wnt signaling pathway molecules, which, until now, have not been evaluated across the entire timeline of dental development. This sheds light on the potential interplay between these molecules in facilitating proper tooth morphogenesis. Previous studies have shown that WNT10A phenotypic changes involve microdontia of dentition, defective cusp, and root morphology (Bohring et al., 2009; Mues et al., 2014; Xu et al., 2017). Dkk1 knockout mice exhibited two significant phenotypes: the absence of anterior head structures and malformed forelimbs (Mukhopadhyay et al., 2001). Elevated expression of Dkk1 in odontoblasts and dental pulp cells hindered the formation of dentin in mandibular molars and led to pronounced dentin resorption (Han et al., 2011). Sost-deficient mice demonstrated an increased width of the cementum and a reduction in the periodontal space width (Kuchler et al., 2014). A better understanding of the dynamic gene expression patterns of Wnt ligands and modulators in dentinogenesis could provide critical information for the development of improved treatments for vital pulp therapy and dentin regeneration.
Our observations differ from prior in-vivo studies that reported isolated spatiotemporal expression patterns of Sost and Dkk1 (Fjeld et al., 2005; Naka and Yokose, 2011). It was demonstrated that SOST protein presence was limited to odontoblasts engaged in pre-dentin secretion as well as osteoblasts (Naka and Yokose, 2011). Additionally, at the E13 stage, Dkk1 transcripts were noted in the epithelial bud of molar tooth germs (Fjeld et al., 2005). Surprisingly, our findings indicate that Dkk1 and Sost transcripts were detected in the adjacent mesenchymal tissue, dental papilla cells, and odontoblasts throughout the developmental stages of both molars and incisors, ranging from E12.5 to P7. Previous studies have shown that the Sost gene, acts as an inhibitor of canonical Wnt signaling and is known to play a role in regulating bone mass (Li et al., 2005; Poole et al., 2005; Power et al., 2010). In this study, we have confirmed the presence of Sost in dental papilla cells, as well as in secretory odontoblasts located adjacent to the ameloblasts in odontogenesis. Both osteocytes and odontoblasts are responsible for producing the mineralizing matrix and have mechanosensing functions (Lanyon, 1993; Allard et al., 2000). Our research clearly demonstrates that Sost also serves as a marker for dental papilla and odontoblast cells during tooth morphogenesis and dentin matrix deposition. Further investigations are necessary to elucidate the mechanisms governing the expression of Sost in odontoblasts.
Our data are consistent with earlier studies that implicate Wnt10a as potentially involved not only in tooth bud initiation but also in patterning, odontoblast differentiation, and root furcation development (Yamashiro et al., 2007; Zhang et al., 2014; Yang et al., 2015; Xu et al., 2017; Yu et al., 2020; Yoshinaga et al., 2023). Furthermore, our results build upon prior observations by demonstrating that Wnt10a mRNA was prominently present not only in terminally differentiated odontoblasts but also in the ameloblasts along the developing cusp tip of both molars and incisors. Robust expression of Wnt10a is noted in our study along the gradient cytodifferentiation of ameloblasts at the developing cusp tip of both molars and incisors, suggesting its involvement in the process of crown morphogenesis and amelogenesis. The co-localization pattern of Wnt10a, Dkk1, and Sost in both terminally differentiating and secreting odontoblasts of molars and incisors potentially signifies the crucial interaction between Wnt ligands and mediators originating from each cusp tip and extending towards the apical region. Moreover, there was a decrease in the expression of Wnt10a in the ameloblasts, along with reduced expression of Wnt10a, Sost, and Dkk1 in the odontoblasts, particularly at the occlusal groove of mandibular molars. This provides further evidence of their crucial involvement in the process of tooth morphogenesis. Following the mineralization of enamel and dentin at P14, there was a noticeable decline in Wnt10a signals, with neither Sost nor Dkk1 being detected in molars. It indicates that Wnt10a and modulators play a vital role in the deposition of mineralized tissue in tooth development. In addition, prior studies have suggested that Wnt10a (Li et al., 2023; Hara et al., 2021) and Sost (Collignon et al., 2017) play roles in activating odontoblasts and promoting the differentiation of reparative odontoblasts in response to tooth injury and direct pulp capping. This clearly indicates their role in facilitating mineralized tissue deposition not only in normal dentinogenesis but also in reparative dentinogenesis. We speculate that Sost and Dkk1 become inactive once the mineralized tissue is deposited. However, it is important to note that Wnt ligands and modulators have the potential to be reactivated during the process of reparative dentinogenesis (Li et al., 2023; Hara et al., 2021; Collignon et al., 2017). At P14, high expression of Wnt10a transcripts was seen in ameloblasts and odontoblasts along with Sost and Dkk1 in odontoblasts at the proximal end of the growing incisor. These data substantiate the requirement of Wnt10a, Sost, and Dkk1 by odontoblasts during the active phases of matrix synthesis, particularly evident at the apical end of continuously erupting incisors.
Our scRNA-seq analysis revealed overlapping or individual expression signatures of Wnt ligands, receptors, mediators, and inhibitors in the dental epithelium and/or mesenchyme, inferring their respective involvement in the differentiation of various cells during odontogenesis. scRNA-seq analysis revealed distinct patterns of expression of Wnt10a and Dkk1 at E15.5 and P1, corroborating and validating our in-situ data. This novel dataset now provides a valuable resource for understanding shifts in gene expression profiles between epithelium and mesenchyme, one of the fundamental developmental interactions responsible for the patterning and morphogenesis of tooth organs. Of note, our dataset did not detect sufficient Sost expression in the samples collected, which we attribute to relatively low expression that was not detected at the sequencing coverage levels used here. Thus, we could not draw specific conclusions regarding Sost from our scRNA-seq. Similarly, the expression of Wnt10a and Dkk1 in scRNA-seq was lower than that observed in situ. This may be due to the common drop-out effect where expressed transcripts are not detected because of technical sequencing coverage limitations, or not capturing intact, fully dissociated cells of interest in droplets (Qiu, 2022). Furthermore, the limits of resolution in preparing samples at developmental time points may mean that the E15.5 used for scRNA-seq and E15.5 used for hybridization could still differ from each other by a matter of hours, which may be sufficiently different from each other given the dynamic nature of development.
Several studies have used Wnt agonist therapies to stimulate Wnt signaling for dentin-pulp regeneration (Neves et al., 2017; Ali et al., 2019; Birjandi et al., 2020). Here, the spatiotemporal interrogation of dynamic expression patterns of multiplexed Wnt10a, Sost, and Dkk1 during tooth morphogenesis reveals novel insight into the critical modulation of Wnt signaling requisite for proper homeostatic signaling, differentiation, and patterning. Further studies of the molecular mechanisms involving Wnt10a, Sost, and Dkk1 in dentinogenesis and reactionary/reparative dentin formation may pave the way toward the development of multi-target strategies for dentin regeneration.
Data availability statement
The data presented in the study are deposited in the GEO repository (https://www.ncbi.nlm.nih.gov/geo/), accession number GSE247224.
Ethics statement
The animal study was approved by All animal procedures were approved by the National Institutes of Health, National Institute of Child Health and Human Development Animal Care and Use Committee (ACUC), under Animal Study Protocol #21-031. The study was conducted in accordance with the local legislation and institutional requirements.
Author contributions
RR: Conceptualization, Data curation, Formal Analysis, Investigation, Methodology, Software, Validation, Visualization, Writing–original draft, Writing–review and editing. JP: Data curation, Investigation, Methodology, Software, Validation, Writing–review and editing. DR: Data curation, Formal Analysis, Investigation, Methodology, Software, Writing–review and editing. PC: Data curation, Project administration, Validation, Visualization, Writing–review and editing. FK: Project administration, Validation, Writing–review and editing. FF: Data curation, Formal Analysis, Investigation, Software, Writing–review and editing. JI: Data curation, Formal Analysis, Investigation, Software, Writing–review and editing. GF: Data curation, Formal Analysis, Investigation, Software, Writing–review and editing. RD: Data curation, Formal Analysis, Investigation, Methodology, Software, Writing–review and editing. RD’S: Conceptualization, Funding acquisition, Investigation, Resources, Supervision, Validation, Visualization, Writing–review and editing.
Funding
The author(s) declare financial support was received for the research, authorship, and/or publication of this article. This work was supported by National Institute of Child Health and Human Development (NICHD) Research Grant Identifier ZIA HD009015 to RD and 1ZICHD008986-04 to RD’S.
Acknowledgments
We acknowledge the technical and graphic assistance of Drs. Vincent Schram, Sergey Leiken and Elena Makareeva and Ms. Nichole Swan (NICHD, NIH). We thank Celia Tomas and other NICHD animal facility staff for assistance with animal husbandry and breeding.
Conflict of interest
The authors declare that the research was conducted in the absence of any commercial or financial relationships that could be construed as a potential conflict of interest.
Publisher’s note
All claims expressed in this article are solely those of the authors and do not necessarily represent those of their affiliated organizations, or those of the publisher, the editors and the reviewers. Any product that may be evaluated in this article, or claim that may be made by its manufacturer, is not guaranteed or endorsed by the publisher.
Supplementary material
The Supplementary Material for this article can be found online at: https://www.frontiersin.org/articles/10.3389/fphys.2023.1316635/full#supplementary-material
References
Ali M., Okamoto M., Komichi S., Watanabe M., Huang H., Takahashi Y., et al. (2019). Lithium-containing surface pre-reacted glass fillers enhance hDPSC functions and induce reparative dentin formation in a rat pulp capping model through activation of Wnt/β-catenin signaling. Acta biomater. 96, 594–604. doi:10.1016/j.actbio.2019.06.016
Allard B., Couble M. L., Magloire H., Bleicher F. (2000). Characterization and gene expression of high conductance calcium-activated potassium channels displaying mechanosensitivity in human odontoblasts. J. Biol. Chem. 275 (33), 25556–25561. doi:10.1074/jbc.M002327200
Birjandi A. A., Suzano F. R., Sharpe P. T. (2020). Drug repurposing in Dentistry; towards application of small molecules in dentin repair. Int. J. Mol. Sci. 21 (17), 6394. doi:10.3390/ijms21176394
Bohring A., Stamm T., Spaich C., Haase C., Spree K., Hehr U., et al. (2009). WNT10A mutations are a frequent cause of a broad spectrum of ectodermal dysplasias with sex-biased manifestation pattern in heterozygotes. Am. J. Hum. Genet. 85 (1), 97–105. doi:10.1016/j.ajhg.2009.06.001
Chiba Y., Saito K., Martin D., Boger E. T., Rhodes C., Yoshizaki K., et al. (2020). Single-cell RNA-sequencing from mouse incisor reveals dental epithelial cell-type specific genes. Front. Cell Dev. Biol. 8, 841. doi:10.3389/fcell.2020.00841
Choudhary S., Satija R. (2022). Comparison and evaluation of statistical error models for scRNA-seq. Genome Biol. 23 (1), 27. doi:10.1186/s13059-021-02584-9
Collignon A. M., Amri N., Lesieur J., Sadoine J., Ribes S., Menashi S., et al. (2017). Sclerostin deficiency promotes reparative dentinogenesis. J. Dent. Res. 96 (7), 815–821. doi:10.1177/0022034517698104
Fjeld K., Kettunen P., Furmanek T., Kvinnsland I. H., Luukko K. (2005). Dynamic expression of Wnt signaling-related Dickkopf1, -2, and -3 mRNAs in the developing mouse tooth. Dev. Dyn. 233 (1), 161–166. doi:10.1002/dvdy.20285
Florio M., Gunasekaran K., Stolina M., Li X., Liu L., Tipton B., et al. (2016). A bispecific antibody targeting sclerostin and DKK-1 promotes bone mass accrual and fracture repair. Nat. Commun. 7, 11505. doi:10.1038/ncomms11505
Grandi F., Caroli J., Romano O., Marchionni M., Forcato M., Bicciato S. (2022). popsicleR: a R package for pre-processing and quality control analysis of single cell RNA-seq data. J. Mol. Biol. 434 (11), 167560. doi:10.1016/j.jmb.2022.167560
Hafemeister C., Satija R. (2019). Normalization and variance stabilization of single-cell RNA-seq data using regularized negative binomial regression. Genome Biol. 20 (1), 296. doi:10.1186/s13059-019-1874-1
Han X. L., Liu M., Voisey A., Ren Y. S., Kurimoto P., Gao T., et al. (2011). Post-natal effect of overexpressed DKK1 on mandibular molar formation. J. Dent. Res. 90 (11), 1312–1317. doi:10.1177/0022034511421926
Hara M., Horibe K., Mori H., Nakamura H. (2021). The role of canonical Wnt signaling in dentin bridge formation. J. Oral Biosci. 63 (2), 199–209. doi:10.1016/j.job.2021.03.003
Hermans F., Bueds C., Hemeryck L., Lambrichts I., Bronckaers A., Vankelecom H. (2022). Establishment of inclusive single-cell transcriptome atlases from mouse and human tooth as powerful resource for dental research. Front. Cell Dev. Biol. 10, 1021459. doi:10.3389/fcell.2022.1021459
Jin S., Guerrero-Juarez C. F., Zhang L., Chang I., Ramos R., Kuan C. H., et al. (2021). Inference and analysis of cell-cell communication using CellChat. Nat. Commun. 12 (1), 1088. doi:10.1038/s41467-021-21246-9
Jing J., Feng J., Yuan Y., Guo T., Lei J., Pei F., et al. (2022). Spatiotemporal single-cell regulatory atlas reveals neural crest lineage diversification and cellular function during tooth morphogenesis. Nat. Commun. 13 (1), 4803. doi:10.1038/s41467-022-32490-y
Kornsuthisopon C., Photichailert S., Nowwarote N., Tompkins K. A., Osathanon T. (2022). Wnt signaling in dental pulp homeostasis and dentin regeneration. Archives oral Biol. 134, 105322. doi:10.1016/j.archoralbio.2021.105322
Krivanek J., Soldatov R. A., Kastriti M. E., Chontorotzea T., Herdina A. N., Petersen J., et al. (2020). Dental cell type atlas reveals stem and differentiated cell types in mouse and human teeth. Nat. Commun. 11 (1), 4816. doi:10.1038/s41467-020-18512-7
Kuchler U., Schwarze U. Y., Dobsak T., Heimel P., Bosshardt D. D., Kneissel M., et al. (2014). Dental and periodontal phenotype in sclerostin knockout mice. Int. J. oral Sci. 6 (2), 70–76. doi:10.1038/ijos.2014.12
Lanyon L. E. (1993). Osteocytes, strain detection, bone modeling and remodeling. Calcif. tissue Int. 53 (1), S102–S106. doi:10.1007/BF01673415
Li X., Zhang Y., Kang H., Liu W., Liu P., Zhang J., et al. (2005). Sclerostin binds to LRP5/6 and antagonizes canonical Wnt signaling. J. Biol. Chem. 280 (20), 19883–19887. doi:10.1074/jbc.M413274200
Li Y., Wu M., Xing X., Li X., Shi C. (2023). Effect of Wnt10a/β-catenin signaling pathway on promoting the repair of different types of dentin-pulp injury. In Vitro Cell Dev. Biol. Anim. 59 (7), 486–504. doi:10.1007/s11626-023-00785-z
Liao C., Ou Y., Wu Y., Zhou Y., Liang S., Wang Y. (2019). Sclerostin inhibits odontogenic differentiation of human pulp-derived odontoblast-like cells under mechanical stress. J. Cell. physiology 234 (11), 20779–20789. doi:10.1002/jcp.28684
Malinauskas T., Jones E. Y. (2014). Extracellular modulators of Wnt signalling. Curr. Opin. Struct. Biol. 29, 77–84. doi:10.1016/j.sbi.2014.10.003
McGinnis C. S., Murrow L. M., Gartner Z. J. (2019). DoubletFinder: doublet detection in single-cell RNA sequencing data using artificial nearest neighbors. Cell Syst. 8 (4), 329–337. doi:10.1016/j.cels.2019.03.003
Mues G., Bonds J., Xiang L., Vieira A. R., Seymen F., Klein O., et al. (2014). The WNT10A gene in ectodermal dysplasias and selective tooth agenesis. Am. J. Med. Genet. Part A 164A (10), 2455–2460. doi:10.1002/ajmg.a.36520
Mukhopadhyay M., Shtrom S., Rodriguez-Esteban C., Chen L., Tsukui T., Gomer L., et al. (2001). Dickkopf1 is required for embryonic head induction and limb morphogenesis in the mouse. Dev. Cell 1 (3), 423–434. doi:10.1016/s1534-5807(01)00041-7
Naka T., Yokose S. (2011). Spatiotemporal expression of sclerostin in odontoblasts during embryonic mouse tooth morphogenesis. J. Endod. 37 (3), 340–345. doi:10.1016/j.joen.2010.11.025
Neves V. C., Babb R., Chandrasekaran D., Sharpe P. T. (2017). Promotion of natural tooth repair by small molecule GSK3 antagonists. Sci. Rep. 7, 39654. doi:10.1038/srep39654
Poole K. E., van Bezooijen R. L., Loveridge N., Hamersma H., Papapoulos S. E., Löwik C. W., et al. (2005). Sclerostin is a delayed secreted product of osteocytes that inhibits bone formation. FASEB J. 19 (13), 1842–1844. doi:10.1096/fj.05-4221fje
Power J., Poole K. E., van Bezooijen R., Doube M., Caballero-Alías A. M., Lowik C., et al. (2010). Sclerostin and the regulation of bone formation: effects in hip osteoarthritis and femoral neck fracture. J. bone mineral Res. 25 (8), 1867–1876. doi:10.1002/jbmr.70
Qiu P. (2020). Embracing the dropouts in single-cell RNA-seq analysis. Nat. Commun. 11 (1), 1169. doi:10.1038/s41467-020-14976-9
Tamura M., Nemoto E. (2016). Role of the Wnt signaling molecules in the tooth. Jpn. Dent. Sci. Rev. 52 (4), 75–83. doi:10.1016/j.jdsr.2016.04.001
Xu M., Horrell J., Snitow M., Cui J., Gochnauer H., Syrett C. M., et al. (2017). WNT10A mutation causes ectodermal dysplasia by impairing progenitor cell proliferation and KLF4-mediated differentiation. Nat. Commun. 8, 15397. doi:10.1038/ncomms15397
Yamashiro T., Zheng L., Shitaku Y., Saito M., Tsubakimoto T., Takada K., et al. (2007). Wnt10a regulates dentin sialophosphoprotein mRNA expression and possibly links odontoblast differentiation and tooth morphogenesis. Differentiation 75 (5), 452–462. doi:10.1111/j.1432-0436.2006.00150.x
Yang J., Wang S. K., Choi M., Reid B. M., Hu Y., Lee Y. L., et al. (2015). Taurodontism, variations in tooth number, and misshapened crowns in Wnt10a null mice and human kindreds. Mol. Genet. genomic Med. 3 (1), 40–58. doi:10.1002/mgg3.111
Yoshinaga K., Yasue A., Mitsui S. N., Minegishi Y., Oyadomari S., Imoto I., et al. (2023). Effects of Wnt10a and Wnt10b double mutations on tooth development. Genes 14 (2), 340. doi:10.3390/genes14020340
Yu M., Liu Y., Wang Y., Wong S. W., Wu J., Liu H., et al. (2020). Epithelial Wnt10a is essential for tooth root furcation morphogenesis. J. Dent. Res. 99 (3), 311–319. doi:10.1177/0022034519897607
Keywords: tooth morphogenesis, cell differentiation, Wnt signaling modulators, odontoblasts, ameloblasts, dentinogenesis
Citation: Raju R, Piña JO, Roth DM, Chattaraj P, Kidwai FK, Faucz FR, Iben J, Fridell G, Dale RK and D’Souza RN (2024) Profiles of Wnt pathway gene expression during tooth morphogenesis. Front. Physiol. 14:1316635. doi: 10.3389/fphys.2023.1316635
Received: 10 October 2023; Accepted: 13 November 2023;
Published: 10 January 2024.
Edited by:
Christian Morsczeck, University of Regensburg, GermanyReviewed by:
Noriaki Ono, University of Texas Health Science Center at Houston, United StatesWanida Ono, University of Texas Health Science Center at Houston, United States
Copyright © 2024 Raju, Piña, Roth, Chattaraj, Kidwai, Faucz, Iben, Fridell, Dale and D’Souza. This is an open-access article distributed under the terms of the Creative Commons Attribution License (CC BY). The use, distribution or reproduction in other forums is permitted, provided the original author(s) and the copyright owner(s) are credited and that the original publication in this journal is cited, in accordance with accepted academic practice. No use, distribution or reproduction is permitted which does not comply with these terms.
*Correspondence: Rena N. D’Souza, rena.d’souza@nih.gov