- 1Environmental Science and Health Research Group, Facultad de Medicina Humana, Universidad Nacional del Centro del Perú, Huancayo, Peru
- 2Facultad de Ingeniería Ambiental y de Recursos Naturales, Universidad Nacional del Callao, Callao, Peru
- 3Facultad de Ingeniería Química, Universidad Nacional del Centro del Perú, Huancayo, Peru
- 4Facultad de Zootecnia, Universidad Nacional del Centro del Perú, Huancayo, Peru
High Andean rivers are fragile ecosystems in the face of various threats, including heavy metal contamination. The objective of this study was to evaluate the potential ecological risk of heavy metals in surface sediment of lotic systems in the central region of Peru. Composite samples of surface sediments were collected from the Chía and Miraflores rivers and the concentrations of heavy metals were determined. The ecological risk analysis was carried out based on the contamination indexes and confirmed by the modified degree of contamination (mCd). The concentration of heavy metals in the sediment of the Chía river was in the following descending order: Fe > Mn > Zn > V > Pb > Cr > Ni > Cu > Mo > Hg, y en el río Miraflores fue: Fe > Mn > Zn > Ni > V > Cr > Cu > Pb > Hg > Mo. The mean concentration of Cu, Cr, Fe, Mn, Mo, Ni, Pb, and V in the sediment samples in both rivers did not exceed the threshold values of the continental crust concentration, nor the interim sediment quality guidelines of the Canadian Council of Ministers of the Environment. However, the mean concentration of Hg exceeded the guideline values in the Miraflores river and the likely effect (0.7 mg.kg−1) adverse effects. The values of the enrichment factor (EF), contamination factor (CF), geoaccumulation index (Igeo), and pollution load index (PLI) indicated low contamination in the sediments of the rivers studied, being confirmed by the modified degree of contamination (mCd). Finally, the risk assessment showed that heavy metals in the sediments presented a low potential ecological risk.
1 Introduction
Water quality is high on the global sustainability agenda, especially in rivers, as it is directly related to human wellbeing (Töre et al., 2021; Uddin et al., 2021; Nayak et al., 2023). The conditions of these aquatic ecosystems have deteriorated over time due to heavy metal inputs from anthropogenic and natural sources (Zhang et al., 2022; Heikkinen et al., 2023). Metallic elements released into rivers by human activities such as urban wastewater discharges, mining and industrial emissions, intensive agriculture and livestock farming alter the balance of aquatic ecosystems (Redwan and Elhaddad, 2017; Luo et al., 2019; Ali et al., 2022; Zhang et al., 2023). Natural sources also contribute significant concentrations of heavy metals to water bodies (Duncan et al., 2018; Dendievel et al., 2022). For example, in areas with volcanic activity, it is possible to find high concentrations of boron and arsenic in water bodies (Ali and Khan, 2018). Heavy metals accumulated in sediments can mobilize to the water column and increase their bioavailability in both matrices and influence their bioaccumulation and biomagnification in aquatic flora and fauna (Ustaoglu and Tepe, 2019; Algül and Beyhan, 2020; Ambrosino et al., 2023). Several factors influence the accumulation and spatial distribution of heavy metals in sediments (Wei and Wen, 2012), such as solubility, mobility, adsorption, bioavailability, precipitation, and cation dynamics (Bakshe and Jugade, 2021). However, the degree of risk from heavy metals will vary depending on concentration levels and source (Sojka et al., 2023).
Heavy metals can enter the human body through a wide range of food chains and reach toxic amounts (Jaishankar et al., 2014; Isroni and Maulida, 2022; Peñaloza et al., 2023). Prolonged exposure to heavy metals can alter biochemical processes, lead to their accumulation in the liver and kidneys and induce toxicity in many organs of the body with carcinogenic, teratogenic, and cardiovascular consequences (Algül and Beyhan, 2020; Bakshe and Jugade, 2021; Alam et al., 2023). Over the past decades, many studies have reported on sediment contamination by heavy metals, source identification, distribution pattern, degree of contamination, and health risks (Saiful et al., 2015; Engin et al., 2017; Yi et al., 2017; Ibrahim et al., 2019); however, few have attempted to establish a link between them. The degree of sediment contamination by heavy metals and the risks they pose can be assessed using individual and complex indices such as contamination factor (CF), enrichment factor (EF), modified contamination index (mCd), and potential ecological risk (Ri; Bian et al., 2016; Jia et al., 2017; Libralato et al., 2018; Li et al., 2020; Wieringa et al., 2022), as they allow associating pollution patterns to their potential sources (Wang et al., 2017).
In Peru, heavy metal contamination of rivers is a major concern not only because it degrades these ecosystems and restricts their use, but also because it threatens human health. Specifically, specific research experiences oriented to evaluate the ecological risk of heavy metals in Andean aquatic ecosystems are scarce (Custodio et al., 2021b). The Chía and Miraflores rivers, located in the central region of Peru, are of vital importance for the various ecosystem services they provide. The predominant activities dependent on these water bodies are mainly fish farming, water for human consumption and water for irrigation and recreation. Currently, there is no known research on the level of contamination and ecological risks of heavy metals in sediments of these rivers. Therefore, it is of utmost importance to generate relevant information on river sediment quality conditions for water quality protection and control. In this study, the degree of contamination and the potential ecological risk of sediment by heavy metals have been evaluated to obtain a comprehensive understanding of the pressure that these ecosystems are experiencing. In addition, the results will allow decision makers to propose and implement action measures to protect and conserve these ecosystems and reduce information gaps aligned with Sustainable Development Goal 6 (Clean Water and Sanitation). In this sense, the objective of this study is to evaluate the potential ecological risk of heavy metals in surface sediment of lotic systems in the central region of Peru.
2 Materials and methods
2.1 Study area
The Chía and Miraflores rivers are located in the Mantaro river watershed in Peru between parallels 10°34′30″ and 13°35′30″ south latitude, and meridians 73°55′00″ and 76°40′30″ west longitude (Figure 1). Both rivers are characterized by fish farming activities, but downstream they are strongly influenced by agricultural and urban activities. The Mantaro basin has a climate that varies according to altitude. In the study area of the Chía and Miraflores rivers, the climate is temperate and cold, with low humidity. The average air temperature is lowest in July and highest in November. In areas above 4,000 masl the temperature is around 4.3°C, in areas between 3,000 and 4,000 masl the temperature varies between 8.1 and 10.4°C and in areas between 2,000 and 3,000 masl the temperature varies between 14.6 and 17.4°C (Custodio et al., 2021a). Rainfall occurs gradually from September onwards, intensifies in February and March and decreases in April to reach minimum values in June (Ministry of Agriculture, 2010). The sampling sites were established according to the existing sources of contamination, such as wastewater discharges from fish farms, runoff from agricultural areas and urban wastewater. In both rivers, three sampling sites were established with three sampling sub-sites in each sector.
2.2 Sample collection and laboratory analysis
Surface sediment samples (0–10 cm depth) from the Chia and Miraflores rivers were collected using a stainless steel device, according to standard protocol (USEPA, 2001) in June 2022. Samples from each zone were mixed following the protocol of Condor et al. (2021) to obtain composite samples; these were transferred to the laboratory in cold chain for analysis (APHA, 2012). Sediment samples were dried at 40°C, ground and sieved with an 85 μm mesh sieve to obtain uniform samples. Heavy metal extraction was performed according to the protocol of Custodio et al. (2022) from 1 g of sediment per sample. Finally, the total concentrations of Ni, Co, Cd, Cr, Cu, Zn, Pb, V, Sb, and As were analyzed with an inductively coupled plasma mass spectrometer (ICP-MS, Perkin Elmer NexION 1000). Quality control and quality assurance was performed by applying standard laboratory methods (APHA, 2012).
2.3 Heavy metal contamination and ecological risk assessment methods
The ecological risk due to heavy metals was evaluated based on contamination indices, such as the enrichment factor (EF), contamination factor (CF), geoaccumulation index (Igeo), pollution load index (PLI), modified degree of contamination (mCd), and potential ecological risk (Ri). In addition, the site rank index (SRI) was applied to integrate the EF, Cf, and Igeo results obtained for each metal evaluated. This integrated value was then compared with the complex indices, such as PLI, mCd, and Ri (Omwene et al., 2018). In the calculation of these indicators, the upper continental crust values were considered because they provide a reference value for the Hg (Turekian and Wedepohl, 1961).
2.3.1 Enrichment factor
The FE estimates the anthropogenic impact of metals on sediments. It usually uses iron as a marker to differentiate or normalize the natural and anthropogenic components in the collected sample (Abrahim and Parker, 2008; Fernández et al., 2018). The EF is calculated using Equation 1.
Donde, sample es la concentración de metal en el sedimento, Cm background es la concentración del metal en la corteza continental, sample es la concentración de hierro en el sedimento y CFe background es la concentración de hierro en la corteza superior continental.
Where, Cm sample is the concentration of metal in the sediment, Cm background is the concentration of metal in the continental crust, CFe sample is the concentration of iron in the sediment and CFe background is the concentration of iron in the continental upper crust. An EF <1 qualifies as not enriched, 1 <EF <3 low enrichment, 3 <EF <5 moderate enrichment, 5 <EF <10 moderately severe enrichment, 10 <EF <25 severe enrichment, 25 <EF <50 very severe enrichment and EF > 50 extremely severe enrichment (El-amier et al., 2017; Omwene et al., 2018).
2.3.2 Contamination factor
Cf is the ratio of the concentration of each metal in the sediment to the background value (Turekian and Wedepohl, 1961). This index was used to quantify the metal contamination status of the sediment for each metal evaluated (Hakanson, 1980; El-amier et al., 2017) and is calculated by the Equation 2.
Where, Cm sample is the metal concentration in the sediment, and Cm background is the metal concentration in the upper continental crust. A CF <1 indicates a low contamination factor, 1 <CF <3 moderate contamination factor, 3 <CF <6 considerable contamination factor, and CF ≥ 6 very high contamination factor (El-amier et al., 2017).
2.3.3 Geoaccumulation index
Igeo determines the difference of metal content in the samples and background values that exist naturally in the continental crust (Turekian and Wedepohl, 1961). This index establishes the sediment contamination levels for each metal evaluated (Kowalska et al., 2018; Ranjbar et al., 2020) which is calculated by Equation 3.
Where, Cn is the metal concentration in the sediments, Bn is the metal concentration in the upper continental crust, and 1.5 is a factor applied to minimize the effect of possible variations in background values. Igeo is classified into seven levels: Igeo ≤ 0, level 0 (not contaminated); 0 <Igeo <1, level 1 (not contaminated to moderately contaminated); 1 <Igeo <2, level 2 (moderately contaminated); 2 <Igeo <3, level 3 (moderately to heavily contaminated); 3 <Igeo <4, level 4 (heavily contaminated); 4 <Igeo <5 level 5 (heavily to extremely contaminated); Igeo > 5, level 6 (extremely contaminated; Müller, 1979).
2.3.4 Pollution load index
The PLI determines the degree of sediment contamination by heavy metals and the degree of impairment in the environment (Tomlinson et al., 1980; Mohiuddin et al., 2011). The PLI is calculated using the Equation 4.
Where, CFn is the contamination factor for each metal, and n is the number of metals evaluated. A value of PLI = 0 indicates good ecosystem condition, PLI = 1 indicates baseline levels of contaminants in the ecosystem, and PLI > 1 indicates progressive deterioration of ecosystem quality (Tomlinson et al., 1980).
2.3.5 Modified degree of contamination (mCd)
The mCd evaluates the degree of global contamination of sediments, integrating all the heavy metals evaluated in the ecosystem (Abrahim and Parker, 2008). The mCd is calculated using the Equation 5.
Where, CF is the contamination factor, i is the “ith” metal, and n is the number of metals evaluated. mCd is classified in the ranges: mCd ≤ 1.5, from uncontaminated to very low degree of contamination; 1. 5 <mCd ≤ 2, low degree of contamination; 2 <mCd ≤ 4, moderate degree of contamination; 4 <mCd ≤ 8, high degree of contamination; 8 <mCd ≤ 16, very high degree of contamination; 16 <mCd ≤ 32, extremely high degree of contamination; mCd > 32, ultra-high degree of contamination.
2.3.6 Potential ecological risk (Ri)
The Ri results from the sum of the ecological risk factors (Er) calculated for each heavy metal evaluated. This index determines the overall degree of ecological risk of heavy metals in sediment samples (Hakanson, 1980). Ri is calculated using the Equation 6.
Where, Tr is the biological toxic factor for each metal, and i is the “ith” metal. Ri is classified in the ranges: Ri ≤ 95 low potential ecological risk; 95 <Ri ≤ 190 moderate potential ecological risk; 190 <Ri ≤ 380 considerable potential ecological risk and Ri > 380 very high potential ecological risk (Ranjbar et al., 2020).
2.3.7 Site rank index (SRI)
The SRI indicates the state of sediment contamination by comparing the level of metal contamination of the sampling sites under the same metric avoiding the comparison in different classifications of each contamination index (CF and Igeo; Omwene et al., 2018; Zhang et al., 2020). The SRI is calculated using Equation 7.
Where, S is the number of sampling sites, n is the site contamination rank in ascending order (at each value used: CF and Igeo), and i is the “ith” metal. An SRI <median - SD indicates low contamination; median - DS <SRI <median, moderate contamination; median <SRI <median + DS, high contamination; median + DS <SRI <SRI, severe contamination (Omwene et al., 2018).
2.4 Statistical analysis
Data analysis and processing were performed using R software and R-Studio (Version 4.1.3). The Shapiro-Wilk test was used to assess whether the data have a normal distribution. A cluster analysis was performed using the Euclidean distancing method, Ward's linkage and a standardization of values using Z-scores. A principal component analysis (PCA) was performed to identify possible sources of metal contamination in the sediments of both rivers.
3 Results
3.1 Analysis and distribution of metals in sediments
Descriptive statistics for metals in sediment from the Chía and Miraflores rivers, continental crust (CC) reference values (Turekian and Wedepohl, 1961) and Canadian Council of Ministers of the Environment (ISQG-CCME) interim sediment quality guidelines (Canadian Council of Ministers of the Environment, 2001) values are presented in Table 1. The mean concentration of Cu, Cr, Fe, Mn, Mo, Ni, Pb, and V in sediment samples in the Chía and Miraflores rivers did not exceed the threshold values of the continental crust concentration, nor the ISQG-CCME values. The mean Hg concentration in the Chía river (0.16 ± 0.01 mg.kg−1) did not exceed the threshold value of the continental crust concentration (0.4 mg.kg−1), but it was between the TEL and PEL values of the ISQG-CCME, being located in the range of possibility where adverse effects occasionally occur. Regarding the mean Hg concentration in the Miraflores river (1.01 ± 0.03 mg.kg−1), this exceeded the threshold value of the continental crust, in addition to exceeding the PEL value (0.7 mg.kg−1) of the ISQG-CCME, which means that it is above the range of possibility where adverse effects occur frequently. The mean Zn concentration in the Miraflores river did not exceed any of the comparison standards. While in the Chía river, the mean Zn concentration (120.12 ± 1.86 mg.kg−1) only exceeded the threshold value of the continental crust.
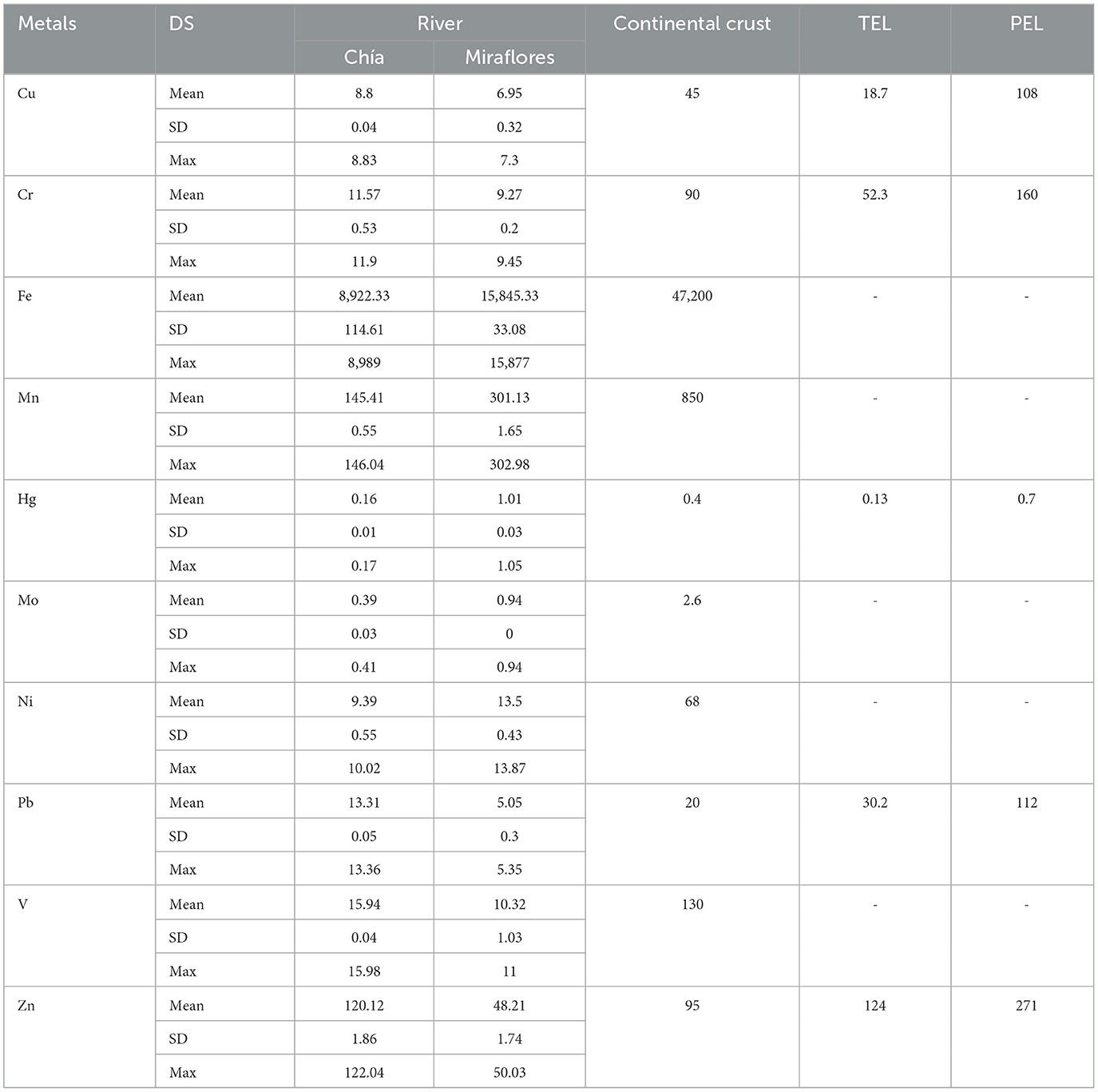
Table 1. Descriptive statistics (DS) of metals concentrations (mg/kg) in sediments of rivers and international reference values [Continental crust (Turekian and Wedepohl, 1961), threshold effect level (TEL), and probable effect level (PEL) of the interim sediment Quality guidelines (Canadian Council of Ministers of the Environment, 2001)].
The decreasing order of heavy metal concentration in the sediments of the Chía river was: Fe > Mn > Zn > V > Pb > Cr > Ni > Cu > Mo > Hg, and in the Miraflores river it was: Fe > Mn > Zn > Ni > V > Cr > Cu > Pb > Hg > Mo (Supplementary Table 1). With respect to the metals that exceeded any of the comparison standards, the highest concentration of Hg (1.05 mg.kg−1) was recorded in the middle zone of the Miraflores river. While the highest concentration of Zn (122.04 mg.kg−1) was recorded in the highest zone of the Chía river. In the specific case of Hg, it is observed that it has exceeded at least one of the standards in each of the rivers, revealing the ease of adsorption on surfaces. These results are supported by Ullrich et al. (2010) who report that in aquatic environments Hg is mostly bound to sediments. In addition, it is the metal that occupies the last places in terms of concentration levels in the sediments of the rivers studied. This behavior of Hg is due to the fact that its occurrence in nature is not common and its complex dynamics in ecosystems does not allow it to be easily detected by common analytical techniques (Velásquez et al., 2021; Cheng et al., 2023). In addition, the chemical speciation of Hg determines its mobility and toxicity in sediments where microbial methylation can occur (Beckers and Rinklebe, 2017; Pavithra et al., 2023).
3.2 Cluster analysis and identification of potential source of contamination
The dendrogram obtained by the Ward method shows the clustering of metal concentrations in the sediments (Figure 2). Cluster analysis shows the clustering of heavy metals in each of the rivers studied (Figure 2). The clustering procedure highlighted clusters in which the sampling sites have similar characteristics. The first cluster groups sampling sites in the upper and middle part of each river and the second cluster groups sampling sites in the lower part. In addition, it allows the metals with the highest concentrations in the Chía river (V, Cr, Cu, Pb, and Zn) to be separated from those found in the Miraflores river (Ni, Mo, Hg, Fe, and Mn). On the other hand, the principal component analysis (PCA) was performed to identify the potential source of contamination, through the grouping and behavior of the metals evaluated. The data were analyzed taking into account the sample adequacy measure KMO (0.55) and Bartlett's test of sphericity (p-value = 0.01). Components 1 and 2 covered 99.3% of the total variance (PC1: 98.1% and PC2: 1.2%; Supplementary Table 2). According to the contribution of each metal in the principal components, all are above 0.94 in PC1, where Fe, Pb, Mn, and Hg have the highest contribution values (0.99), while Cr and V show the highest contributions in PC2 (0.036 and 0.037, respectively; Supplementary Table 3). The high contributions found in PC1 and the high degree of variability shown by this PCA may be due to the high degree of association shown by certain groups of metals and the clear distinction between the two rivers studied. This situation is also observed in Figure 3, where there is a clear differentiation between the Chía and Miraflores rivers with respect to the heavy metals evaluated. The distribution of these metals in each river would reveal the possible specific anthropogenic contribution in each body of water, which could be due to the main activities practiced in these ecosystems.
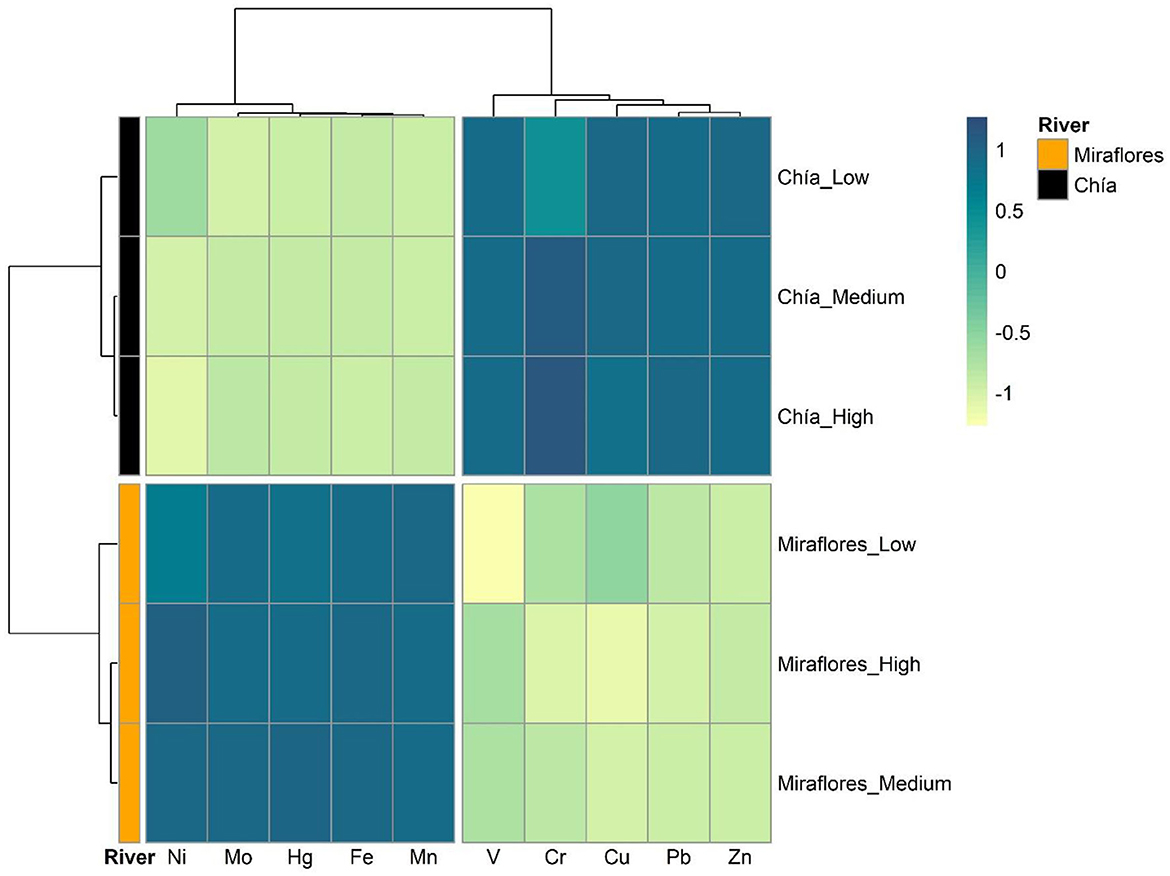
Figure 2. Dendrogram showing the clustering of sampling sites according to the concentration of metals in the sediments of the Miraflores and Chía rivers.
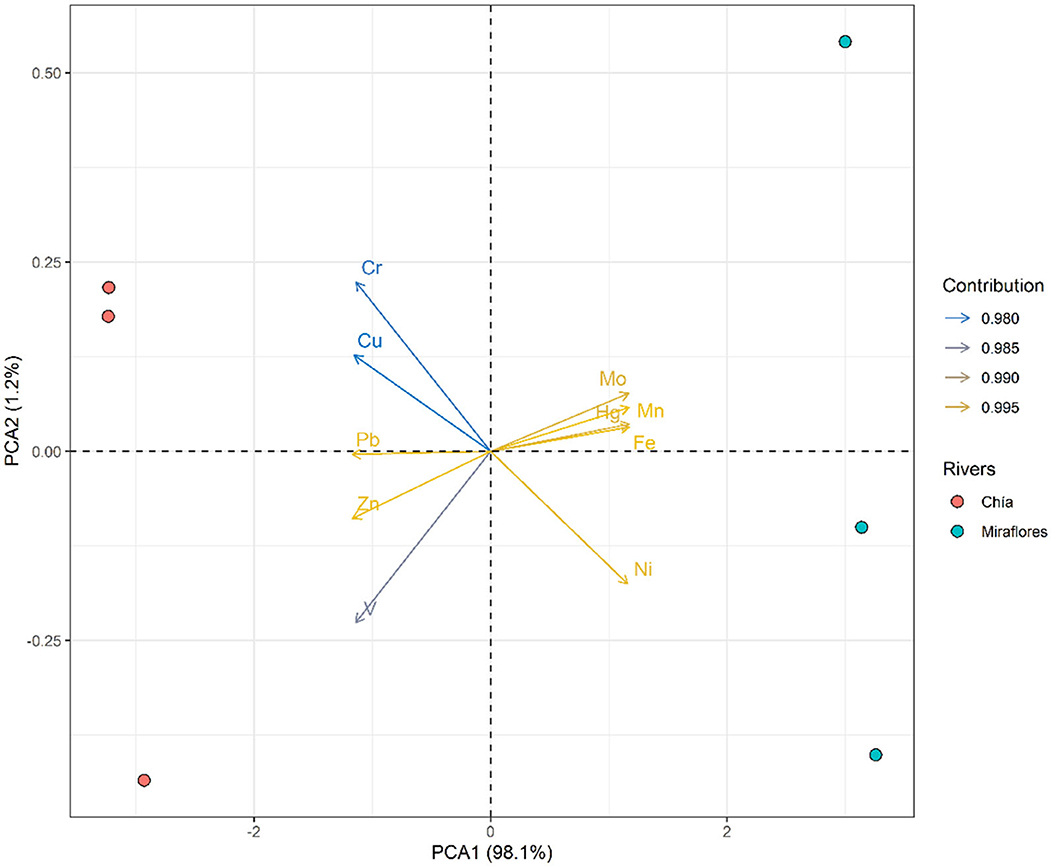
Figure 3. Principal component analysis based on the concentration of metals in sediment of Chía and Miraflores rivers.
3.3 Assessment of contamination and potential ecological risk by metals in sediments
Table 2 shows the results obtained from the individual contamination indexes obtained for each metal, according to river. The highest EF values were found for Hg, Pb, and Zn, both in the Chía and Miraflores rivers. However, when categorizing these values qualitatively, we can note that for the Chía river, minor enrichment (1 <EF <3) of Cu and Hg, moderate enrichment (3 <EF <5) of Pb and moderately severe enrichment (5 <EF <10) of Zn have been found. While the other metals have presented values categorized as absence of enrichment (1 <EF). On the other hand, in the Miraflores river, minor enrichment has been observed due to the presence of Mn and Mo and moderately severe enrichment due to the presence of Hg.
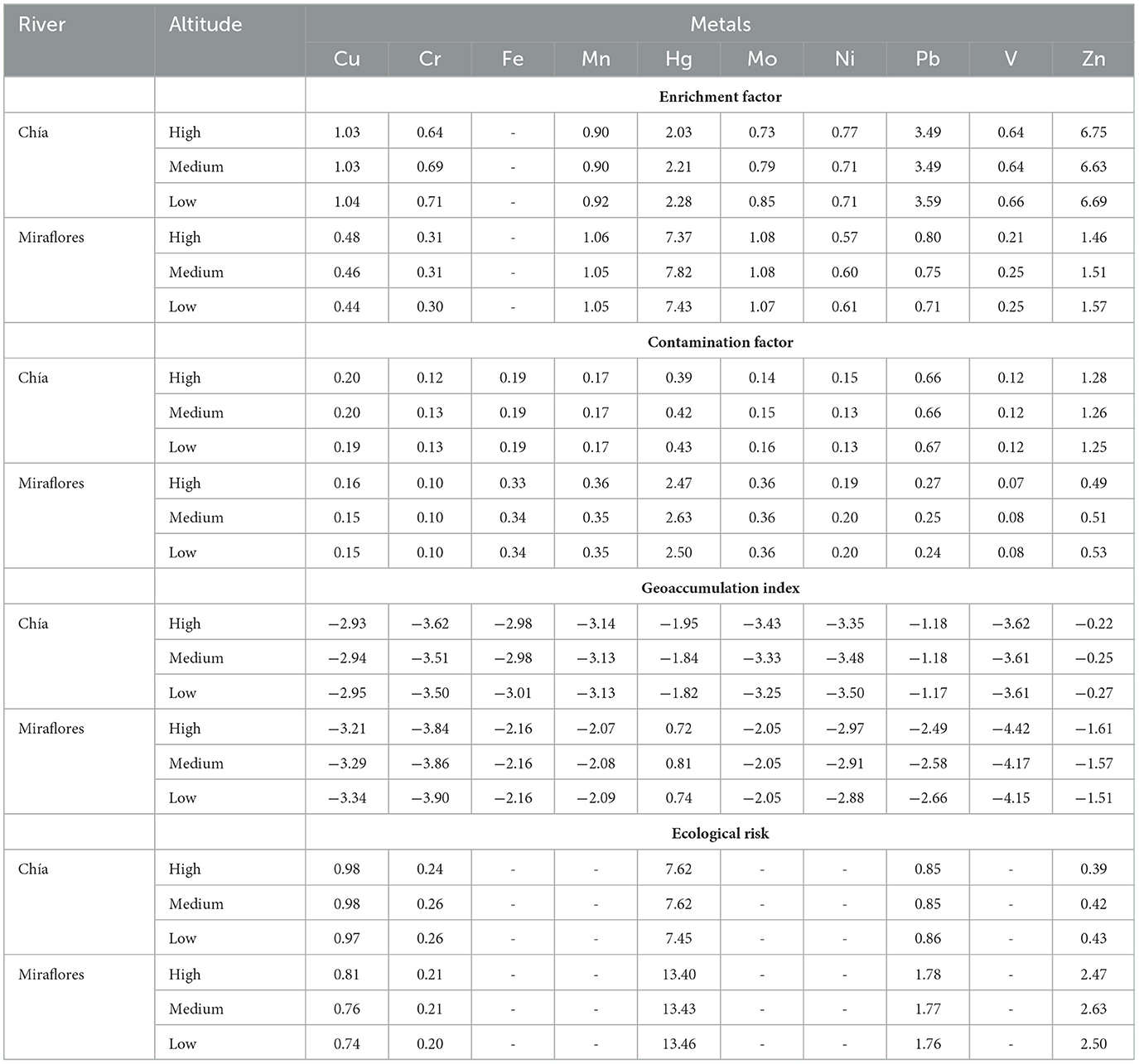
Table 2. Enrichment factor, contamination factor, geoaccumulation index, and ecological risk of metals in sediment of Chía and Miraflores rivers.
In the case of CF values, the highest levels were found for Hg and Zn (CF-Hg> 2, CF-Zn > 1). When categorizing these values, moderate contamination levels (1 <CF <3) of Hg and Zn were determined for the Miraflores and Chia rivers, respectively; while for the rest of the metals evaluated, contamination levels categorized as low were found. In the case of the Igeo values, only positive values were found for Hg levels in the Miraflores river. However, these were <1, categorized as not contaminated to moderately contaminated. For the rest of the metals, the Igeo category corresponded to not contaminated. It should be noted that these results found by the individual indices are in agreement with those found in Table 1, where Hg and Zn were determined to have exceeded at least one of the standards considered. However, the individual analysis of each metal allows us to observe certain anomalies in the levels of Cu and Pb for the Chía river, which added to the levels of Zn contamination could be part of a mix of metals anthropogenically contributed to this body of water.
Table 3 shows the complex contamination indexes evaluated by river, such as PLI, mCd, and Ri and the Site Rank Index (SRI) generated from EF and Cf. Regarding the complex indexes such as PLI and mCd, these have shown values below 0.5 for both rivers; qualifying as sediments without contamination and very low degree of contamination, respectively. In the same sense, the values of ecological risk and potential ecological risk are categorized as low ecological risk (Er <40) and low potential ecological risk (Ri <95). These results are due to the fact that all these complex indices consider the contribution of all the metals evaluated, and probably, the low contribution of contamination observed in metals such as Cu, Cr, Fe, Mn, Mo, Ni, and V, which means that the global index does not present considerable contamination categories. Likewise, when calculating the SRI for the most representative individual indices (EF and CF), values above 50 are observed, which after being categorized (Supplementary Tables 4, 5) show moderate (50 <SRI-EF <63) and high (63 <SRI-EF <77) enrichment levels for the Miraflores river and moderate and severe enrichment (SRI-EF > 77) for the Chía river; as well as moderate (59 <SRI-CF <65) and severe (SRI-CF > 71) contamination levels, because both indices, in their individual calculation, have categorized Hg and Zn at their highest impact levels. These results also reveal the need to implement action measures to protect and conserve these dynamic systems (Matta et al., 2022) so that, in the intervention area, the diversity of uses (in fish farming, irrigation, and human consumption) of these ecosystems is not restricted and does not affect the economy of local residents.
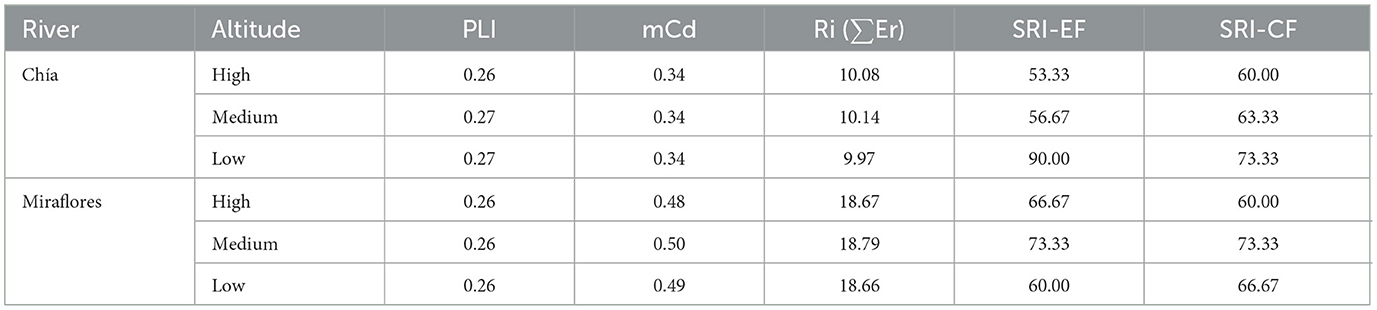
Table 3. Pollution load index (PLI), modified contamination degree (mCd), potential ecological risk (Ri), site rank index (SRI) for enrichment factor (EF), and contamination factor (Cf) of metals in sediment of Chía and Miraflores rivers.
4 Discussion
4.1 Analysis and distribution of metals in sediments
Heavy metal contamination is a widespread concern due to the adverse impacts on ecosystems and human health (Ting et al., 2020; Fisher et al., 2021; Liu et al., 2023). The results show that contamination of lotic systems varies by downstream sector, and could increase due to the transport of pollutants through runoff from agricultural areas and those with mining operations. Heavy metals entering these aquatic systems tend to adsorb on suspended particles and deposit in sediments, which could cause long-term effects on local benthic organisms and other species (Zeng et al., 2018; Liu et al., 2022). In this study, the concentration of heavy metals in sediment samples from the Chía and Miraflores rivers, determined based on upper continental crust reference values (Taylor and Mclennan, 1995) and the Interim Sediment Quality Guidelines at their Threshold Effect Levels and Probable Effect Levels (Canadian Council of Ministers of the Environment, 2004) did not exceed the threshold values, except for Hg and Zn. In comparison to the heavy metal content of the Mantaro river basin, the metal contents found in the present study are lower due to the different industrial structure and economic development (Custodio et al., 2021b).
Most of the mean heavy metal concentrations in the sediments of the Chia and Miraflores rivers did not exceed the threshold values of the continental crust concentration, nor the ISQG-CCME values. However, the mean Hg concentration in both rivers revealed the possibility of adverse effects. The impact of relatively intense human activities in the area downstream of both rivers may be the main reason for the distribution of heavy metals. For example, the mean concentration of Zn and Hg exceeded the threshold value of the interim sediment quality guidelines. These results are in agreement with other studies reporting that sediments are the main sink for heavy metals in the aquatic environment (Wei et al., 2023), where sediment quality could reflect the degree of environmental contamination status on a longer time scale (Qian et al., 2021). In addition, unlike other pollutants, metals do not biodegrade and are toxic and bioaccumulative (Zeng and Wu, 2013; Ranjbar et al., 2017; Sojka et al., 2022). However, the metals that exceeded some of the comparison standards were Hg in the Miraflores river and Zn in the Chía river. Hg is the metal with the lowest concentration level in sediments, because its occurrence in nature is not common and its complex dynamics in ecosystems does not allow it to be easily detected by common analysis techniques (Velásquez et al., 2021; Cheng et al., 2023).
The pattern of heavy metal concentrations found in the rivers of this study differs from that reported by Monroy et al. (2014) in water bodies with similar characteristics. The concentrations of metals in the sediments of the Chía and Miraflores rivers were lower; however, wastewater discharges significantly affect the water column, sediments and fish. Therefore, source and risk assessments of sediment contamination in aquatic ecosystems are essential for effective management. Rainbow trout farming is one of the main activities in the central highlands of Peru. However, several studies have reported that in aquaculture, the use of feed additives containing metals or antibiotics can cause adverse environmental impacts in receiving water bodies (Seiler and Berendonk, 2012; Jaishankar et al., 2014; Wang et al., 2019). Metal ions can be released from these feed additives or antibiotics and adsorbed on suspended particles, subsequently deposited and accumulated in sediments, and from there, distributed to other compartments such as the water column and aquatic fauna.
Spatial variation in heavy metal levels was revealed through cluster analysis. River sectors with similar characteristics were grouped together, while those with different characteristics had distant links. This behavior allowed disaggregating the metals with higher concentrations found in the Chia river in relation to those found in the Miraflores river. Principal component analysis was used to explore the possible sources of sediment contamination by heavy metals. PCA generated a vector matrix plotted on a two-dimensional axis. The high contributions found in PC1 and the high degree of variability shown by this PCA may be due to the high degree of association presented by some groups of metals and the clear difference between the two rivers studied. The distribution of these metals in each river would reveal the possible specific anthropogenic contribution in each water body, which could be due to the main activities practiced in these ecosystems. These results coincide with other studies of similar environments that report that Zn, Cr, Ni, Hg, and Cu contamination is mainly related to industrial activities (Chen et al., 2016; Meng et al., 2021). In general, Hg is an important component of agrochemicals, pesticides and herbicides (Wang et al., 2023), Cu and Zn mainly come from animal excrement, as they are micronutrients added to animal feed as growth promoters (Yang et al., 2020). Cr and Pb come from the electroplating and transportation industries (Chen et al., 2016; Jin et al., 2022) as a result of wear and tear on vehicle parts, use of lubricants and gasoline, and from automobile manufacturing (as additives for airbag detonators), and as a feed additive (Zhang et al., 2022). Natural sources are another important source of metal contaminants in sediments when the pH is low (Luo et al., 2019). Low pH can increase metal solubility and influence metal concentrations in river sediments (Bai et al., 2016).
4.2 Assessment of contamination and potential ecological risk by metals in sediments
The individual contamination indices obtained for each metal in the Chía and Miraflores rivers showed different levels of contamination. The enrichment indexes of Hg, Pb, and Zn in both rivers were the highest. However, when categorizing these values, minor enrichment of Cu and Hg, moderate enrichment of Pb and moderately severe enrichment of Zn were found in the Chía river. In the Miraflores river, minor enrichment of Mn and Mo and moderately severe enrichment due to the presence of Hg were found, indicating that the study area of both rivers is impacted by human activities (agriculture, industry, urbanization, etc.). In contrast, other heavy metals evaluated were in a safe state. The CF showed that Hg and Zn presented the highest levels of contamination. By categorizing these values, moderate levels of Hg and Zn contamination were determined for the Miraflores and Chía rivers, respectively; while for the rest of the heavy metals, low levels of contamination were determined for the Miraflores and Chía rivers, respectively; while for the rest of the heavy metals, low levels of contamination were determined for the Miraflores and Chía rivers, respectively.
The Igeo values for Hg in the Miraflores River showed values <1, categorized as not contaminated to moderately contaminated. The Igeo of the rest of the metals qualified as uncontaminated. However, the individual analysis of each metal allows us to observe certain important levels of Cu and Pb contamination for the Chía river, which, added to the levels of Zn contamination, could be part of a mix of metals anthropogenically contributed to the water body. For instance, Hahn et al. (2018) indicates that sediments become contaminated due to the release of metals from soils by leaching and soil erosion processes. The results found are supported by other studies that refer that Cu, Pb, and Zn tend to be rapidly diluted and transported long distances once they are released into the water body and finally deposited in the sediment (Zhuang et al., 2021; Nagarajan et al., 2023). These findings also indicate that Hg is the main contributor to heavy metal pollution in the Miraflores river (Zahra et al., 2014; Ke et al., 2017; Li et al., 2022).
Complex contamination indices such as PLI and mCd, showed values below 0.5 for both rivers, qualifying as sediments with no contamination and very low degree of contamination, respectively. In general, the PLI index reflects the non-negligible integrated contamination of the studied heavy metals in the river sediments. These results are supported by Ali et al. (2016) who refer that PLI can provide some insight to populations about sediment quality. As well as, information for decision makers about the pollution status of the study area. In the same sense, the values of ecological risk and potential ecological risk are categorized as low ecological risk and low ecological risk potential. The results also indicate that the contribution of all the heavy metals evaluated determines the degree of contamination of the sediments (Omwene et al., 2018; Zhang et al., 2020), and a potential effect on organisms (Ranjbar et al., 2020). However, heavy metal contamination of both rivers is related to the human activities that take place in them (Liu et al., 2022). These results suggest that the ecological risk from metals in the sediments of the Miraflores river comes mainly from Hg, as it is the main contributor.
5 Conclusion
The constant population growth and development around the Chía and Miraflores rivers goes hand in hand with the strong pressure experienced by these ecosystems and the demand for good quality water. This study revealed that the concentrations of heavy metals in the sediments of both rivers did not exceed the reference values and thresholds of the upper continental crust and the provisional guidelines on sediment quality, respectively; with the exception of Hg in the Miraflores river and Zn in the Chía river. The application of statistical ordination methods revealed a clear partition in the importance of certain metals for each river, where the difference in metallic elements was determined by Hg, Pb, and Zn. The EF and Cf values showed that the most important metals were Hg, Pb, and Zn with moderate to moderately severe enrichment and moderate contamination. However, applying complex indices such as PLI, mCd, and Ri, very low levels of contamination and/or low ecological risk were found, which shows the importance of the contribution of the other metals that were not relevant in the analyses, since these are global indices. The SRI revealed that the Miraflores river has moderate to high enrichment levels and the Chía river has moderate to severe levels, mainly influenced by Hg and Zn concentrations. Finally, this study revealed that the main contributions of metallic elements come from various anthropogenic activities. Therefore, it is recommended that stricter control and monitoring measures be directed to point sources of heavy metals in order to reduce the pressure of these sources of contamination on Andean aquatic ecosystems.
Data availability statement
The original contributions presented in the study are included in the article/Supplementary material, further inquiries can be directed to the corresponding author.
Author contributions
MC: Conceptualization, Formal analysis, Investigation, Methodology, Supervision, Visualization, Writing—original draft, Writing—review & editing. AF: Data curation, Formal analysis, Investigation, Writing—review & editing. HD: Investigation, Methodology, Visualization, Writing—review & editing. FC: Investigation, Writing—review & editing. JH: Investigation, Methodology, Writing—review & editing.
Funding
The author(s) declare that no financial support was received for the research, authorship, and/or publication of this article.
Acknowledgments
We thank the Water Research Laboratory for allowing us to use their equipment and materials to carry out the sampling phase.
Conflict of interest
The authors declare that the research was conducted in the absence of any commercial or financial relationships that could be construed as a potential conflict of interest.
Publisher's note
All claims expressed in this article are solely those of the authors and do not necessarily represent those of their affiliated organizations, or those of the publisher, the editors and the reviewers. Any product that may be evaluated in this article, or claim that may be made by its manufacturer, is not guaranteed or endorsed by the publisher.
Supplementary material
The Supplementary Material for this article can be found online at: https://www.frontiersin.org/articles/10.3389/frwa.2023.1295712/full#supplementary-material
References
Abrahim, G. M. S., and Parker, R. J. (2008). Assessment of heavy metal enrichment factors and the degree of contamination in marine sediments from Tamaki Estuary, Auckland, New Zealand. Environ. Monitor. Assess. 136, 227–238. doi: 10.1007/s10661-007-9678-2
Alam, M., Rohani, M. F., and Hossain, M. S. (2023). Heavy metals accumulation in some important fish species cultured in commercial fish farm of Natore, Bangladesh and possible health risk evaluation. Emerg. Contaminants 9, 100254. doi: 10.1016/j.emcon.2023.100254
Algül, F., and Beyhan, M. (2020). Concentrations and sources of heavy metals in shallow sediments in Lake Bafa, Turkey. Sci. Rep. 10, 1–12. doi: 10.1038/s41598-020-68833-2
Ali, H., and Khan, E. (2018). What are heavy metals? Long-standing controversy over the scientific use of the term ‘heavy metals'–proposal of a comprehensive definition. Toxicol. Environ. Chem. 100, 6–19. doi: 10.1080/02772248.2017.1413652
Ali, M. M., Ali, M. L., Islam, M. S., and Rahman, M. Z. (2016). Preliminary assessment of heavy metals in water and sediment of Karnaphuli River, Bangladesh. Environ. Nanotechnol. Monit. Manage. 5, 27–35. doi: 10.1016/j.enmm.2016.01.002
Ali, M. M., Ali, M. L., Rakib, M. R. J., Islam, M. S., Bhuyan, M. S., Senapathi, V., et al. (2022). Seasonal behavior and accumulation of some toxic metals in commercial fishes from Kirtankhola Tidal River of Bangladesh—a health risk taxation. Chemosphere 301, 134660. doi: 10.1016/j.chemosphere.2022.134660
Ambrosino, M., El-Saadani, Z., Khatita, A. A., Mingqi, W., Palarea-Albaladejo, J., and Cicchella, D. (2023). Geochemical speciation, ecological risk and assessment of main sources of potentially toxic elements (PTEs) in stream sediments from Nile River in Egypt. Water 15, 132308. doi: 10.3390/w15132308
APHA (2012). Standard Methods for the Examination of Water and Wastewater. American Public Health Association, 541.
Bai, J., Jia, J., Zhang, G., Zhao, Q., Lu, Q., Cui, B., et al. (2016). Spatial and temporal dynamics of heavy metal pollution and source identification in sediment cores from the short-term flooding riparian wetlands in a Chinese Delta. Environ. Pollut. 219, 379–388. doi: 10.1016/j.envpol.2016.05.016
Bakshe, P., and Jugade, R. (2021). Distribution, association, and ecological risk evaluation of heavy metals and influencing factors in major industrial stream sediments of Chandrapur District, Central India. Water Air Soil Pollut. 232, 3. doi: 10.1007/s11270-021-05040-3
Beckers, F., and Rinklebe, J. (2017). Cycling of mercury in the environment: sources, fate, and human health implications: a review. Crit. Rev. Environ. Sci. Technol. 47, 693–794. doi: 10.1080/10643389.2017.1326277
Bian, B., Zhou, Y., and Fang, B. B. (2016). Distribution of heavy metals and benthic macroinvertebrates: impacts from typical inflow river sediments in the Taihu Basin, China. Ecol. Indicat. 69, 348–359. doi: 10.1016/j.ecolind.2016.04.048
Canadian Council of Ministers of the Environment (2001). Canadian Sediment Quality Guidelines for the Protection of Aquatic Life. Sediment Quality. Environment Canada Guidelines and Standards Division.
Canadian Council of Ministers of the Environment (2004). Soil, Ground Water and Sediment Standards for Use Under Part XV. 1 of the Environmental Protection Act. Queen's Printer for Ontario.
Chen, T., Chang, Q., Liu, J., Clevers, J. G. P. W., and Kooistra, L. (2016). Identification of soil heavy metal sources and improvement in spatial mapping based on soil spectral information: a case study in Northwest China. Sci. Tot. Environ. 565, 155–164. doi: 10.1016/j.scitotenv.2016.04.163
Cheng, Y., Paolo, F., Albarico, J. B., Chen, C. F., and Chen, C. W. (2023). Pollution sources and ecological risks of potentially toxic metals in sediments from a multi-functional Hsingda harbor in southwestern Taiwan. Region. Stud. Mar. Sci. 58, 102780. doi: 10.1016/j.rsma.2022.102780
Condor, A., Custodio, M., Chanamé, F., Cuadrado, W., and Peñaloza, R. (2021). Heavy metals and arsenic in water, sediment and the muscle of oncorhynchus mykiss from the Tishgo river in the central andes of Peru. J. Ecol. Eng. 22, 156–166. doi: 10.12911/22998993/134045
Custodio, M., Espinoza, C., Peñaloza, R., Peralta-Ortiz, T., Sánchez-Suárez, H., Ordinola-Zapata, A., et al. (2022). Microbial diversity in intensively farmed lake sediment contaminated by heavy metals and identification of microbial taxa bioindicators of environmental quality. Sci. Rep. 12, 1–12. doi: 10.1038/s41598-021-03949-7
Custodio, M., Fow, A., Chanamé, F., Orellana-Mendoza, E., Peñaloza, R., Alvarado, J. C., et al. (2021b). Ecological risk due to heavy metal contamination in sediment and water of natural wetlands with tourist influence in the central region of Peru. Water 13, 162256. doi: 10.3390/w13162256
Custodio, M., Peñaloza, R., Alvarado, J., Chanamé, F., and Maldonado, E. (2021a). Surface water quality in the mantaro river watershed assessed after the cessation of anthropogenic activities due to the COVID-19 pandemic. Pol. J. Environ. Stud. 30, 3005–3018. doi: 10.15244/pjoes/130988
Dendievel, A. M., Grosbois, C., Ayrault, S., Evrard, O., Coynel, A., Debret, M., et al. (2022). Key factors influencing metal concentrations in sediments along western European rivers: a long-term monitoring study (1945–2020). Sci. Tot. Environ. 805, 149778. doi: 10.1016/j.scitotenv.2021.149778
Duncan, A. E., de Vries, N., and Nyarko, K. B. (2018). Assessment of heavy metal pollution in the sediments of the river Pra and its tributaries. Water Air Soil Pollut. 229, 6. doi: 10.1007/s11270-018-3899-6
El-amier, Y. A., Elnaggar, A. A., and El-alfy, M. A. (2017). Egyptian journal of basic and applied sciences evaluation and mapping spatial distribution of bottom sediment heavy metal contamination in Burullus lake, Egypt. Egypt. J. Basic Appl. Sci. 4, 55–66. doi: 10.1016/j.ejbas.2016.09.005
Engin, M. S., Uyanik, A., and Cay, S. (2017). Investigation of trace metals distribution in water, sediments and wetland plants of Kizilirmak Delta, Turkey. Int. J. Sediment Res. 32, 90–97. doi: 10.1016/j.ijsrc.2016.03.004
Fernández, S., Daiana, M., Carbone, M. E., Villagran, D. M., and Marcovecchio, J. E. (2018). Toxic metals in a highly urbanized industry-impacted estuary (Bahia Blanca Estuary, Argentina): spatio-temporal analysis based on GIS. Environ. Earth Sci. 77, 5. doi: 10.1007/s12665-018-7565-5
Fisher, M. B., Guo, A. Z., Wren Tracy, J., Prasad, S. K., Cronk, R. D., Browning, E. G., et al. (2021). Occurrence of lead and other toxic metals derived from drinking-water systems in three West African countries. Environ. Health Perspect. 129, 7804. doi: 10.1289/EHP7804
Hahn, J., Opp, C., Evgrafova, A., Groll, M., Zitzer, N., and Laufenberg, G. (2018). Impacts of dam draining on the mobility of heavy metals and arsenic in water and basin bottom sediments of three studied dams in Germany. Sci. Tot. Environ. 640–641, 1072–1081. doi: 10.1016/j.scitotenv.2018.05.295
Hakanson, L. (1980). An ecological risk index for aquatic pollution control. A sedimentological approach. Water Res. 14, 975–1001. doi: 10.1016/0043-1354(80)90143-8
Heikkinen, A., Nygren, A., and Custodio, M. (2023). The slow violence of mining and environmental suffering in the andean waterscapes. Extract. Industr. Soc. 14, 101254. doi: 10.1016/j.exis.2023.101254
Ibrahim, M. I. A., Mohamed, L. A., Mahmoud, M. G., Shaban, K. S., Fahmy, M. A., and Ebeid, M. H. (2019). Potential ecological hazards assessment and prediction of sediment heavy metals pollution along the Gulf of Suez, Egypt. Egypt. J. Aquatic Res. 45, 329–335. doi: 10.1016/j.ejar.2019.12.003
Isroni, W., and Maulida, N. (2022). Bioacumulation of heavy metals Pb and Hg in green shells (Perna Viridis) in pasuruan waters based on different seasons. IOP Conf. Ser. Earth Environ. Sci. 1036, 12097. doi: 10.1088/1755-1315/1036/1/012097
Jaishankar, M., Tseten, T., Anbalagan, N., Mathew, B. B., and Beeregowda, K. N. (2014). Toxicity, mechanism and health effects of some heavy metals. Interdiscipl. Toxicol. 7, 60–72. doi: 10.2478/intox-2014-0009
Jia, Y., Wang, L., Qu, Z., and Yang, Z. (2017). Distribution, contamination and accumulation of heavy metals in water, sediments, and freshwater shellfish from Liuyang river, Southern China. Environ. Sci. Pollut. Res. 25, 712–720. doi: 10.1007/s11356-017-1068-x
Jin, B., Wang, J., Lou, W., Wang, L., Xu, J., Pan, Y., et al. (2022). Pollution, ecological risk and source identification of heavy metals in sediments from the Huafei river in the eastern suburbs of Kaifeng, China. Int. J. Environ. Res. Publ. Health 19, 1811259. doi: 10.3390/ijerph191811259
Ke, X., Gui, S., Huang, H., Zhang, H., Wang, C., and Guo, W. (2017). Ecological risk assessment and source identification for heavy metals in surface sediment from the Liaohe river protected area, China. Chemosphere 175, 473–481. doi: 10.1016/j.chemosphere.2017.02.029
Kowalska, J. B., Mazurek, R., Gasiorek, M., and Zaleski, T. (2018). Pollution indices as useful tools for the comprehensive evaluation of the degree of soil contamination–a review. Environ. Geochem. Health 40, 2395–2420. doi: 10.1007/s10653-018-0106-z
Li, X., Bing, J., Zhang, J., Guo, L., Deng, Z., Wang, D., et al. (2022). Ecological risk assessment and sources identification of heavy metals in surface sediments of a river–reservoir system. Sci. Tot. Environ. 842, 156683. doi: 10.1016/j.scitotenv.2022.156683
Li, Y., Chen, H., and Teng, Y. (2020). Source apportionment and source-oriented risk assessment of heavy metals in the sediments of an urban river-lake system. Sci. Tot. Environ. 737, 140310. doi: 10.1016/j.scitotenv.2020.140310
Libralato, G., Minetto, D., Lofrano, G., Guida, M., Carotenuto, M., Aliberti, F., et al. (2018). Toxicity assessment within the application of in situ contaminated sediment remediation technologies: a review. Sci. Tot. Environ. 621, 85–94. doi: 10.1016/j.scitotenv.2017.11.229
Liu, N., Liu, H., Wu, P., Meng, W., Li, X., and Chen, X. (2022). Distribution characteristics and potential pollution assessment of heavy metals (Cd, Pb, Zn) in reservoir sediments from a historical artisanal zinc smelting area in southwest China. Environ. Sci. Pollut. Res. 29, 14288–14298. doi: 10.1007/s11356-021-16824-9
Liu, P., Wu, Q., Hu, W., Tian, K., Huang, B., and Zhao, Y. (2023). Comparison of heavy metals in riverine and estuarine sediments in the lower Yangtze river: distribution, sources, and ecological risks. Environ. Technol. Innov. 30, 103076. doi: 10.1016/j.eti.2023.103076
Luo, L., Mei, K., Qu, L., Zhang, C., Chen, H., Wang, S., et al. (2019). Assessment of the geographical detector method for investigating heavy metal source apportionment in an urban watershed of eastern China. Sci. Tot. Environ. 653, 714–722. doi: 10.1016/j.scitotenv.2018.10.424
Matta, G., Kumar, A., Nayak, A., and Kumar, P. (2022). Appraisal of spatial–temporal variation and pollution source estimation of Ganga river system through pollution indices and environmetrics in Upper Ganga Basin. Appl. Water Sci. 12, 1–11. doi: 10.1007/s13201-021-01552-9
Meng, L., Zhao, L., Liu, W., Lian, J., and Chao, L. (2021). Risk assessment of bioavailable heavy metals in the water and sediments in the Yongding New River, North China. Environ. Monitor. Assess. 193, 6. doi: 10.1007/s10661-021-09367-6
Ministry of Agriculture (2010). Assessment of Surface Water Resources in the Mantaro River Watershed. Lima: Ministry of Agriculture.
Mohiuddin, K. M., Ogawa, Y., Zakir, H. M., Otomo, K., and Shikazono, N. (2011). Heavy metals contamination in water and sediments of an urban river in a developing country. Int. J. Environ. Sci. Tech. 8, 723–736. doi: 10.1007/BF03326257
Monroy, M., Maceda-Veiga, A., and de Sostoa, A. (2014). Metal concentration in water, sediment and four fish species from lake Titicaca reveals a large-scale environmental concern. Sci. Tot. Environ. 487, 233–244. doi: 10.1016/j.scitotenv.2014.03.134
Müller, G. (1979). Heavy metals in the sediment of the rhine-changes seitt. Umschauin Wissenschaft Und Technik 79, 778–783.
Nagarajan, R., Eswaramoorthi, S. G., Anandkumar, A., and Ramkumar, M. (2023). Geochemical fractionation, mobility of elements and environmental significance of surface sediments in a Tropical River, Borneo. Mar. Pollut. Bullet. 192, 115090. doi: 10.1016/j.marpolbul.2023.115090
Nayak, A., Matta, G., Uniyal, D. P., Kumar, A., Kumar, P., and Pant, G. (2023). Assessment of potentially toxic elements in groundwater through interpolation, pollution indices, and chemometric techniques in Dehradun in Uttarakhand State. Environ. Sci. Pollut. Res. 23, 123456789. doi: 10.1007/s11356-023-27419-x
Omwene, P. I., Öncel, M. S., Çelen, M., and Kobya, M. (2018). Heavy metal pollution and spatial distribution in surface sediments of Mustafakemalpaşa stream located in the world's largest borate basin (Turkey). Chemosphere 208, 782–792. doi: 10.1016/j.chemosphere.2018.06.031
Pavithra, K., Grace, P., SundarRajan, P., Senthil Kumar, A., and Rangasamy, G. (2023). Mercury sources, contaminations, mercury cycle, detection and treatment techniques: a review. Chemosphere 312, 137314. doi: 10.1016/j.chemosphere.2022.137314
Peñaloza, R., Cacciuttolo, C., Cano, D., and Solorzano, F. (2023). Human health risk assessment for exposure to heavy metals via dietary intake of rainbow trout in the influence area of a smelting facility located in Peru. Toxics 11, 764. doi: 10.3390/toxics11090764
Qian, B., Tang, C., Yang, Y., and Xiao, X. (2021). Pollution characteristics and risk assessment of heavy metals in the surface sediments of Dongting lake water system during normal water period. Eur. J. Remote Sens. 54, 211–221. doi: 10.1080/22797254.2020.1763207
Ranjbar, J., Bakhtiyari, A. R., Toosi, A. S., and Jadot, C. (2017). Spatial distribution, ecological and health risk assessment of heavy metals in marine surface sediments and coastal seawaters of fringing coral reefs of the Persian Gulf, Iran. Chemosphere 185, 1090–1111. doi: 10.1016/j.chemosphere.2017.07.110
Ranjbar, J., Soumita Mitra, A., Raudonyte-Svirbutavičiene, E., and Bakhtiari, A. R. (2020). Large-scale evaluation of deposition, bioavailability and ecological risks of the potentially toxic metals in the sediment cores of the hotspot coral reef ecosystems (Persian Gulf, Iran). J. Hazard. Mater. 400, 122988. doi: 10.1016/j.jhazmat.2020.122988
Redwan, M., and Elhaddad, E. (2017). Heavy metals seasonal variability and distribution in lake Qaroun sediments, El-Fayoum, Egypt. J. Afri. Earth Sci. 134, 48–55. doi: 10.1016/j.jafrearsci.2017.06.005
Saiful, I., Ahmed, M. K., and Habibullah-Al-Mamun, M. (2015). Geochemical speciation and risk assessment of heavy metals in sediments of a river in Bangladesh. Soil Sediment Contaminat. 24, 639–655. doi: 10.1080/15320383.2015.997869
Seiler, C., and Berendonk, T. U. (2012). Heavy metal driven co-selection of antibiotic resistance in soil and water bodies impacted by agriculture and aquaculture. Front. Microbiol. 3:1–10. doi: 10.3389/fmicb.2012.00399
Sojka, M., Jaskuła, J., Barabach, J., Ptak, M., and Zhu, S. (2022). Heavy metals in lake surface sediments in protected areas in Poland: concentration, pollution, ecological risk, sources and spatial distribution. Sci. Rep. 12, 1–16. doi: 10.1038/s41598-022-19298-y
Sojka, M., Ptak, M., Jaskuła, J., and Krasniqi, V. (2023). Ecological and health risk assessments of heavy metals contained in sediments of Polish Dam Reservoirs. Int. J. Environ. Res. Publ. Health 20, 10324. doi: 10.3390/ijerph20010324
Taylor, S. R., and Mclennan, S. M. (1995). The geochemical the continental evolution crust. Rev. Mineral. Geochem. 33, 241–265.
Ting, F., Wenxuan, L., Guanjun, H., Kai, C., Xiuxia, Z., Jing, L., et al. (2020). Fractionation and ecological risk assessment of trace metals in surface sediment from the Huaihe River, Anhui, China. Hum. Ecol. Risk Assess. 26, 147–161. doi: 10.1080/10807039.2018.1497476
Tomlinson, D. L., Wilson, J. G., Harris, C. R., and Jeffrey, D. W. (1980). Problems in the assessment of heavy-metal levels in estuaries and the formation of a pollution index. Helgolander Meeresunters 33, 566–575.
Töre, Y., Ustaoglu, F., Tepe, Y., and Kalipci, E. (2021). Levels of toxic metals in edible fish species of the Tigris River (Turkey); threat to public health. Ecol. Indicat. 123, 107361. doi: 10.1016/j.ecolind.2021.107361
Turekian, K. K., and Wedepohl, K. H. (1961). Distribution of the elements in some major units of the Earth's crust. Geol. Soc. Am. Bullet. 72, 175–192.
Uddin, M. G., Nash, S., and Olbert, A. I. (2021). A review of water quality index models and their use for assessing surface water quality. Ecol. Indicat. 122, 107218. doi: 10.1016/j.ecolind.2020.107218
Ullrich, S. M., Tanton, T. W., and Abdrashitova, S. A. (2010). Mercury in the aquatic environment: a review of factors affecting methylation. Crit. Rev. Environ. Sci. Technol. 31, 241–293. doi: 10.1080/20016491089226
USEPA (2001). Methods for Collection, Storage and Manipulation of Sediment for Chemical and Toxicological Analyses: Technical Manual. Washington, DC: U.S. Environmental Protection Agency.
Ustaoglu, F., and Tepe, Y. (2019). Water quality and sediment contamination assessment of Pazarsuyu Stream, Turkey using multivariate statistical methods and pollution indicators. Int. Soil Water Conserv. Res. 7, 47–56. doi: 10.1016/j.iswcr.2018.09.001
Velásquez, M., Vega, C., Corvera, R., Pillaca, M., Thomas, E., Stewart, P., et al. (2021). Mercury in soils impacted by Alluvial gold mining in the Peruvian Amazon. J. Environ. Manag. 288, 112364. doi: 10.1016/j.jenvman.2021.112364
Wang, N., Han, J., Wei, Y., Li, G., and Sun, Y. (2019). Potential ecological risk and health risk assessment of heavy metals and metalloid in soil around Xunyang Mining Areas. Sustainability 11, 184828. doi: 10.3390/su11184828
Wang, Q., Huang, X., and Zhang, Y. (2023). Heavy metals and their ecological risk assessment in surface sediments of the Changjiang River Estuary and Contiguous East China Sea. Sustainability 15, 54323. doi: 10.3390/su15054323
Wang, Y., Wang, R., Fan, L., Chen, T., Bai, Y., Yu, Q., et al. (2017). Assessment of multiple exposure to chemical elements and health risks among residents near Huodehong lead-zinc mining area in Yunnan, Southwest China. Chemosphere 174, 613–627. doi: 10.1016/j.chemosphere.2017.01.055
Wei, C., and Wen, H. (2012). Geochemical baselines of heavy metals in the sediments of two large freshwater lakes in China: implications for contamination character and history. Environ. Geochem. Health 34, 737–748. doi: 10.1007/s10653-012-9492-9
Wei, H., Wang, Y., Liu, J., and Zeng, R. (2023). Heavy metal in river sediments of Huanghua City in water diversion area from Yellow River, China: contamination, ecological risks, and sources. Water 15, w15010058. doi: 10.3390/w15010058
Wieringa, N., van der Lee, G. H., de Baat, M. L., Kraak, M. H. S., and Verdonschot, P. F. M. (2022). Contribution of sediment contamination to multi-stress in lowland waters. Sci. Tot. Environ. 844, 157045. doi: 10.1016/j.scitotenv.2022.157045
Yang, J., Wang, J., Qiao, P., Zheng, Y., Yang, J., Chen, T., et al. (2020). Identifying factors that influence soil heavy metals by using categorical regression analysis: a case study in Beijing, China. Front. Environ. Sci. Eng. 14, 2. doi: 10.1007/s11783-019-1216-2
Yi, Y., Tang, C., Yi, T., Yang, Z., and Zhang, S. (2017). Health risk assessment of heavy metals in fish and accumulation patterns in food web in the Upper Yangtze River, China. Ecotoxicol. Environ. Saf. 145, 295–302. doi: 10.1016/j.ecoenv.2017.07.022
Zahra, A., Hashmi, M. Z., Malik, R. N., and Ahmed, Z. (2014). Enrichment and geo-accumulation of heavy metals and risk assessment of sediments of the Kurang Nallah-Feeding Tributary of the Rawal Lake Reservoir, Pakistan. Sci. Tot. Environ. 470–471, 925–933. doi: 10.1016/j.scitotenv.2013.10.017
Zeng, H., and Wu, J. (2013). Heavy metal pollution of lakes along the mid-lower reaches of the Yangtze River in China: intensity, sources and spatial patterns. Int. J. Environ. Res. Publ. Health 10, 793–807. doi: 10.3390/ijerph10030793
Zeng, Y., Lai, Z., Yang, W., and Li, H. (2018). Distribution of heavy metals in surface sediments from the Pearl River Outlets, South China: five-year monitoring program. Fresenius Environmental Bulletin 27, 574–583.
Zhang, H., Zeng, H., Jiang, Y., Xie, Z., Xu, X., Ding, M., et al. (2020). Using the compound system to synthetically evaluate the enrichment of heavy metal(loid)s in a Subtropical Basin, China. Environ. Pollut. 256, 113396. doi: 10.1016/j.envpol.2019.113396
Zhang, J., Wang, M., Ren, K., Yan, K., Liang, Y., Yuan, H., et al. (2023). The relationship between mountain wetland health and water quality: a case study of the Upper Hanjiang River Basin, China. J. Environ. Manag. 346, 118998. doi: 10.1016/j.jenvman.2023.118998
Zhang, L., Tan, X., Chen, H., Liu, Y., and Cui, Z. (2022). Effects of agriculture and animal husbandry on heavy metal contamination in the aquatic environment and human health in Huangshui River Basin. Water 14, 40549. doi: 10.3390/w14040549
Keywords: contamination, ecological risk, heavy metals, surface sediment, lotic systems, aquatic environment
Citation: Custodio M, Fow A, De la Cruz H, Chanamé F and Huarcaya J (2024) Potential ecological risk from heavy metals in surface sediment of lotic systems in central region Peru. Front. Water 5:1295712. doi: 10.3389/frwa.2023.1295712
Received: 17 September 2023; Accepted: 29 December 2023;
Published: 11 January 2024.
Edited by:
Pramod Kumar Pandey, Directorate of Coldwater Fisheries Research (ICAR), IndiaReviewed by:
Venkatramanan Senapathi, Alagappa University, IndiaGagan Matta, Gurukul Kangri University, India
Copyright © 2024 Custodio, Fow, De la Cruz, Chanamé and Huarcaya. This is an open-access article distributed under the terms of the Creative Commons Attribution License (CC BY). The use, distribution or reproduction in other forums is permitted, provided the original author(s) and the copyright owner(s) are credited and that the original publication in this journal is cited, in accordance with accepted academic practice. No use, distribution or reproduction is permitted which does not comply with these terms.
*Correspondence: María Custodio, mcustodio@uncp.edu.pe