- 1Hotchkiss Brain Institute, University of Calgary, Calgary, AB, Canada
- 2Alberta Children's Hospital Research Institute, University of Calgary, Calgary, AB, Canada
- 3Department of Neurology, Ahmanson-Lovelace Brain Mapping Center, University of California, Los Angeles, Los Angeles, CA, United States
- 4Department of Bioengineering, University of California, Los Angeles, Los Angeles, CA, United States
- 5Division of Developmental Paediatrics, Department of Paediatrics and Child Health, Red Cross Memorial Children's Hospital, University of Cape Town, Cape Town, South Africa
- 6Neuroscience Institute, University of Cape Town, Cape Town, South Africa
- 7Department of Psychiatry and Mental Health, University of Cape Town, Cape Town, South Africa
- 8South African Medical Research Council (SAMRC), Unit of Risk and Resilience in Mental Disorders, University of Cape Town, Cape Town, South Africa
- 9Department of Psychiatry and Biobehavioural Sciences, University of California, Los Angeles, Los Angeles, CA, United States
- 10The Semel Institute for Neuroscience and Human Behaviour, University of California, Los Angeles, Los Angeles, CA, United States
- 11David Geffen School of Medicine, University of California, Los Angeles, Los Angeles, CA, United States
- 12Department of Paediatrics and Child Health, Red Cross War Memorial Children's Hospital, University of Cape Town, Cape Town, South Africa
- 13South African Medical Research Council (SAMRC), Unit on Child and Adolescent Health, University of Cape Town, Cape Town, South Africa
- 14Department of Radiology, University of Calgary, Calgary, AB, Canada
Introduction: Tobacco and alcohol are the two most common substances used during pregnancy, and both can disrupt neurodevelopment, resulting in cognitive and behavioral deficits including language difficulties. Previous studies show that children with prenatal substance exposure exhibit microstructural alterations in major white matter pathways, though few studies have investigated the impact of prenatal substance exposure on white matter microstructure and language skills during the toddler years.
Methods: In this study, 93 children (34 exposed to alcohol and/or tobacco) aged 23 years from the Drakenstein Child Health Study, South Africa, completed Expressive and Receptive Communication assessments from the Bayley Scales of Infant and Toddler Development, Third Edition (BSID-III) and underwent diffusion MRI scans. Diffusion images were preprocessed, and 11 major white matter tracts were isolated. Fractional anisotropy (FA) and mean diffusivity (MD) were extracted for each white matter tract. Linear regression was used to examine differences between the tobacco/alcohol exposed group and unexposed controls for FA, MD, and language scores, as well as relationships between brain metrics and language. There were no significant group differences in language scores or FA.
Results: Children with alcohol or tobacco exposure had lower average MD in the splenium of the corpus callosum compared to unexposed controls. Significant interactions between prenatal substance exposure and language scores were seen in 7 tracts but did not survive multiple comparisons correction.
Discussion: Our findings show that prenatal alcohol and/or tobacco exposure appear to alter the relationship between white matter microstructure and early language skills in this population of toddlers, potentially laying the basis of language deficits observed later in older children with prenatal substance exposure, which may have implications for learning and interventions.
1 Introduction
Alcohol and tobacco consumption are common in pregnancy, and both substances can cross the placenta and exert maladaptive effects on the developing fetus (Behnke and Eyler, 1993; Veazey et al., 2013; Caputo et al., 2016). It is estimated that upwards of ~10% of pregnant individuals consume alcohol and 1.7% consume tobacco worldwide (Lange et al., 2018; Popova et al., 2023). This prevalence is higher in some countries like South Africa, where ~20% of people report using alcohol (Petersen Williams et al., 2014), and ~5% use tobacco (Phaswana-Mafuya et al., 2019) during pregnancy in some regions.
Alcohol is a teratogen that can disrupt neuronal proliferation and migration and can cause cell death in the fetal brain (Miller, 1986; Veazey et al., 2013). In addition to its direct effects on fetal brain development, alcohol causes decreased umbilical artery blood flow which induces hypoxia in the fetus (Jones et al., 1981; Mukherjee and Hodgen, 1982; Bosco and Diaz, 2012) and can lead to growth retardation (Abel, 1984, 1985; Lo et al., 2017). As a result, individuals with prenatal alcohol exposure (PAE) may demonstrate cognitive and behavioral deficits (Lees et al., 2020; Popova et al., 2023) as well as neuroanatomical changes such as reduced gray matter and white matter volume and altered white matter microstructure (Colby et al., 2012; Donald et al., 2015; Kar et al., 2021; Moore and Xia, 2022).
Maternal tobacco use during pregnancy can also result in impaired fetal growth, and reduced gestation time leading to premature birth (Ward et al., 2007). Nicotine reduces the availability of nutrients and oxygen to the fetus by acting as a powerful vasoconstrictor (Espy et al., 2011). Additionally, neurodevelopment is disrupted when nicotine (rather than endogenous acetylcholine) binds to nicotinic acetylcholine receptors (nAChRs) during gestation (Navarro et al., 1989; Heath and Picciotto, 2009). Studies have also shown lower total cortical volume and surface area (Gonzalez et al., 2023) and white matter alterations in the uncinate fasciculus, corticospinal tract, and the genu of the corpus callosum (Jacobsen et al., 2007; Roos et al., 2021) for children with prenatal tobacco exposure (PTE). PTE has been associated with decreased cognitive performance in children (Ernst et al., 2001; Margolis et al., 2021) and reduced language abilities (Hernández-Martínez et al., 2017). PAE and PTE frequently co-occur (Dukes et al., 2017).
Evidence is mixed when investigating the extent to which PAE or PTE affect language development (Peixinho et al., 2022). Several studies demonstrate that moderate or heavy PAE impairs language skills in early childhood (Fried and Watkinson, 1990; Fried et al., 1992; Hendricks et al., 2019), though others show no major deficits (Hendricks et al., 2020; Lindinger et al., 2022). Longitudinal studies indicate language difficulties at 2-6 years of age in children with PAE and PTE, but no difference when the children were 4-6 years old (Fried and Watkinson, 1990; Fried et al., 1992). Few studies have investigated language skills in PTE. Additionally, a cohort study by Hernández-Martínez et al., 2017 found that infants with PTE had impaired language scores related to auditory function including vocalizations, sound discrimination, and prelinguistic vocalizations. The lack of clarity in previous studies highlights the importance of studying the effects of PAE and/or PTE (collectively termed prenatal substance exposure, PSE) in early childhood, as well as the underlying neural mechanisms of language development.
Magnetic resonance imaging (MRI) offers the capability to non-invasively examine brain structure (Yen et al., 2023). Moreover, white matter microstructure specifically can be assessed using diffusion imaging (dMRI) (Mürner-Lavanchy et al., 2018). Fractional anisotropy (FA) and mean diffusivity (MD) are metrics that are sensitive to microstructural properties including local fiber density, coherence, orientation, and myelination (Basser and Jones, 2002; Beaulieu, 2002). Previous literature has established a well-recognized relationship between white matter microstructure and early language skills (Mills et al., 2013; Mürner-Lavanchy et al., 2018; Walton et al., 2018), reflected by positive relationships between language/reading abilities and FA, and negative relationships with MD in major white matter tracts including the arcuate fasciculus, uncinate fasciculus, inferior fronto-occipital fasciculus, and the corpus callosum (Vandermosten et al., 2012, 2015; Saygin et al., 2013; Walton et al., 2018; Sket et al., 2019; Zuk et al., 2021).
Few studies have examined how PSE affects the relationship between white matter microstructure and language abilities, focusing only on PAE (Gómez et al., 2022; Ostertag et al., 2023). Gómez et al. (2022) found negative associations between speeded naming and FA in the left superior longitudinal fasciculus and left inferior longitudinal fasciculus in 7-16 year olds with PAE. Ostertag et al. (2023) reported altered developmental trajectories in the arcuate fasciculus of preschool aged children with PAE, and a significant FA-by-age-by-group interaction for the left arcuate in predicting speeded naming scores, and right arcuate FA predicting phonological processing scores (Ostertag et al., 2023). Treit et al. (2013) reported significant associations between changes in MD in the superior fronto-occipital fasciculus and superior longitudinal fasciculus, with changes in reading abilities over time in children aged 5-13 years. The relationship between early language skills and diffusion metrics in toddlers with PAE remains unknown.
It is important to understand how PSE affects white matter microstructure and early language skills in toddlers to inform early interventions and mitigate difficulties as the children get older. The current study aimed to investigate white matter microstructure and language, and the relationships between them, in a cohort of South African toddlers with PSE (defined as PAE, PTE, or both).
2 Methods
2.1 Participants
Ninety three children aged 2–3 years (58 males, mean = 2.77 +/−0.14 years) were included in this study. In total, nine children had PAE (but not PTE), 11 children had PTE (and no PAE), 14 children had both PAE and PTE, and 59 children had neither PTE nor PAE (Table 1). Children were part of the larger Drakenstein Child Health Study (DCHS); a multidisciplinary longitudinal birth cohort study in Western Cape, South Africa (Stein et al., 2015; Donald et al., 2018). The DCHS aims to investigate the early-life determinants of infant and child development and cognition (Donald et al., 2018). The Western Cape is culturally and linguistically heterogenous and characterized by low socioeconomic status (Donald et al., 2018). A total of 1143 children were included in DCHS. 121 children were recruited for MRI scans at 2–3 years. Of those, n = 28 did not provide high quality data for analysis. Informed parent/guardian consent was obtained. The DCHS was approved by the University of Cape Town Human Research Ethics Committee (401/2009). The neuroimaging sub-study was approved by the University of Cape Town Human Research Ethics Committee (UCT HREC, Reference 525/2012).
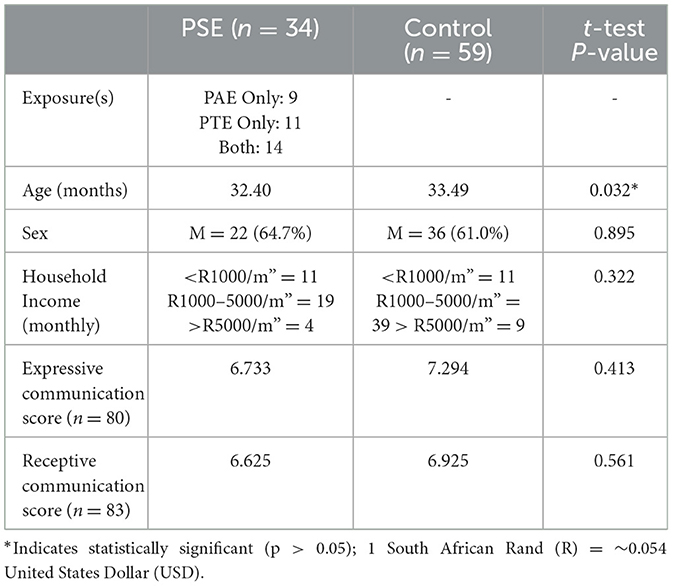
Table 1. Participant demographics and language scores. PSE: children with PAE and/or PTE. Control: unexposed children.
PSE was evaluated using parental questionnaires as well as the Alcohol, Smoking and Substance Involvement Screening Test (ASSIST), a tool developed by the World Health Organization to screen for substance use in adults (WHO ASSIST Working Group, 2002) and has been validated in previous studies (Humeniuk et al., 2008; Roos et al., 2021). Twenty three children had confirmed PAE, with a minimum score of 11 on ASSIST; six children had low exposure, 10 had moderate exposure, five had high exposure, and 2 had no ASSIST scores, but PAE was confirmed by parents. Twenty five participants had PTE, 17 of which had moderate exposure (ASSIST score ≥ 6) and 8 had high exposure (ASSIST score ≥ 28); no children had low exposure to PTE. Children with confirmed prenatal exposure to substances other than alcohol and tobacco (e.g., cannabis, cocaine) were excluded. Additional exclusion criteria included children who: were born < 36 weeks gestation, had genetic and/or congenital disorders, or had contraindications to MRI. Children spoke English, Afrikaans, Shona, or isiXhosa.
2.2 Language assessments
Trained assessors administered the Expressive and Receptive Communication subtests from the Bayley Scales of Infant and Toddler Development, Third Edition (BSID-III; Bayley, 2012) to assess child early language and communication skills. Expressive Communication assesses skills such as gesturing and naming objects and pictures. Receptive Communication assesses the child's ability to recognize and identify sounds, objects, and pictures. Assessments were translated from English (forward and back translation) and completed in the participant's preferred language.
2.3 Image acquisition
Children underwent diffusion MRI in a 3T Siemens Skyra MRI scanner with a 32-channel head coil at Groote Schuur Hospital in Cape Town, South Africa. DTI data was acquired using voxel size = 1.8 × 1.8 × 2.0 mm, TR = 7,800 ms; TE = 92 ms, with 30 gradient diffusion directions at b = 1,000 s/mm2, and one b = 0 s/mm2; one full protocol was acquired in each of the anterior-posterior and posterior-anterior phase encoding directions. All scans were performed during natural sleep. Further details about the MRI image acquisition are described in Wedderburn et al. (2020).
2.4 Image processing
DTI data was preprocessed using ExploreDTI, a graphical toolbox for processing, analyzing, and visualizing MR data that is written in MATLAB (Leemans et al., 2009; MATLAB version: 9.6.0.1472908, The MathWorks Inc, 2022). Our image preprocessing including correction for Gibbs ringing, subject motion, eddy current, and EPI-based susceptibility distortions. The diffusion tensor was calculated via the REKINDLE method (Tax et al., 2015), and whole brain tractography was performed with the following parameters: seedpoint resolution = 2 × 2 × 2 mm3, FA threshold = 0.15, fiber length = 50–500 mm, angle threshold = 30°, step size = 1 mm. Semiautomated tractography was used to delineate 11 major white matter tracts associated with language processing: the bilateral longitudinal fasciculus (ILF), bilateral uncinate fasciculus (UF), bilateral inferior fronto-occipital fasciculus (IFOF), bilateral arcuate fasciculus (AF), and three components of the corpus callosum (CC)—genu, body, and splenium (Figure 1) (Smits et al., 2014; Kargar and Jalilian, 2024). Mean FA and MD were extracted for each tract in each individual.
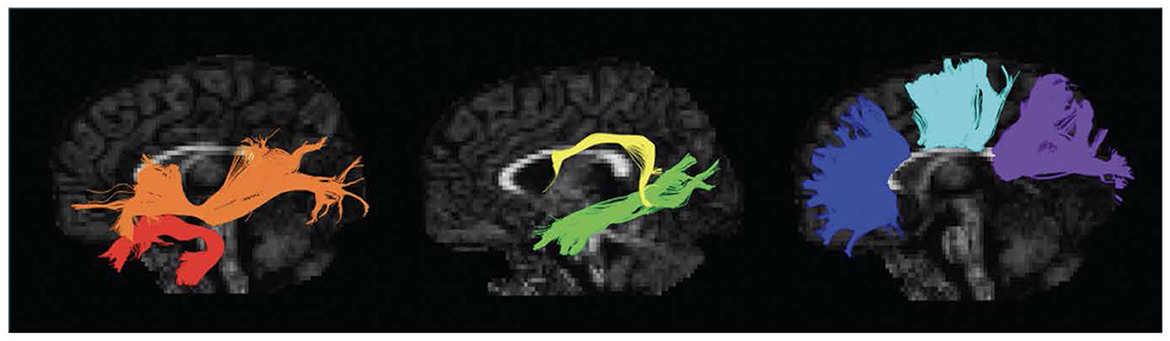
Figure 1. Left sagittal view of isolated white matter tracts. Red, Uncinate Fasciculus (UF); Orange, Inferior Fronto-Occipital Fasciculus (IFOF); Yellow, Arcuate Fasciculus (AF); Green, Inferior Longitudinal Fasciculus (ILF); Blue, Genu of the Corpus Callosum (CC); Aqua, Body of the Corpus Callosum (CC); Purple, Splenium of the Corpus Callosum (CC).
Two prior studies examined brain diffusion properties in an overlapping sample of participants using tract-based spatial statistics (TBSS). Here, we chose to use tractography so we could focus our analysis on specific tracts a priori, analyze those tracts as a whole, and because tractography is less sensitive to small errors in registration across participants than TBSS.
2.5 Statistical analysis
All statistical analysis was completed using R studio (v4.2.1, R Core Team, 2021). Expressive and Receptive Communication scores, age, and FA and MD of each tract were compared between groups using t-tests. Sex differences between groups were assessed using a chi-squared test. Linear regression models were used to test associations between white matter microstructure (FA, MD) and early language skills, as well as the extent to which PSE moderates these associations. Receptive or Expressive Communication scores were the dependent variable, with age, sex, median household income (average household income per month), FA or MD (one at a time), PSE, and the interaction term between DTI metrics and PSE as independent variables, according to the following:
Receptive/Expressive Communication ~ age + sex + household income + FA/MD + FA/MD * PSE.
A total of 44 models were tested: 11 white matter tracts x two diffusion metrics (FA, MD) x two language scores (Receptive Communication, Expressive Communication).
Child's primary language was considered as an additional covariate, but no significant correlations were observed with communication scores during preliminary analysis, so this factor was excluded from subsequent models. False discovery rate (FDR) was implemented to correct for multiple comparisons at q < 0.05. FDR correction was completed separately for Expressive and Receptive Communication models. A total of 22 comparisons were made using FDR for each effect (ex: household income, PSE, PSE x brain interaction).
Post hoc analyses were performed to examine the effects of PAE and PTE separately, compared to controls with no exposure to either substance. The same linear regression models were used for PAE and PTE as were used for PSE.
Given the known relationship between cognition and language, we also investigated whether the two groups had different cognitive scores on the BSID-III Cognitive Scale, and if PSE influenced the relationship between white matter microstructure and Cognitive Scores.
3 Results
3.1 Language scores
An independent samples t-test showed no significant differences in Expressive (p = 0.41) or Receptive (p = 0.56). Communication scores between the PSE and control groups (Table 1).
3.2 White matter microstructure
The arcuate fasciculus could not be delineated in every individual, so the right AF had 89 data points and the left AF had 83 data points. All other tracts were delineated in all individuals. There were no significant group differences of FA in any white matter tract (Figure 2). The PSE group had lower MD in the left IFOF (p = 0.030) and the splenium of the CC (p = 0.002) (Figure 3). After FDR corrections, only the differences in the splenium remained significant (q = 0.034).
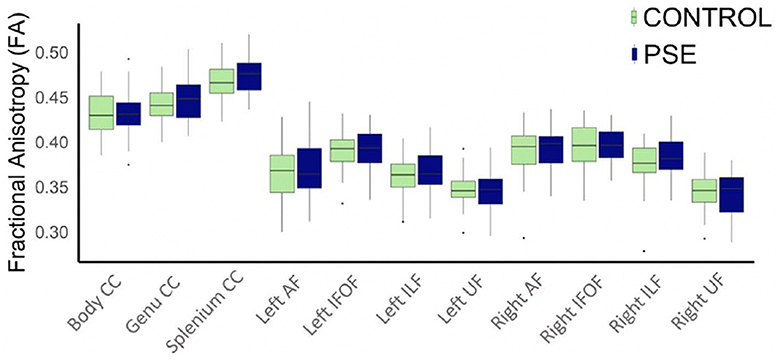
Figure 2. Boxplot displaying mean FA values of the white matter tracts for prenatal substance exposure (PSE; defined as prenatal alcohol and/or tobacco exposure) and control groups. FA values were directly pulled from the white matter for each participant interest variables have not been regressed out. No group differences in FA were significant. ILF, inferior longitudinal fasciculus; UF, uncinate fasciculus; IFOF, inferior fronto-occipital fasciculus; AF, arcuate fasciculus; CC, corpus callosum.
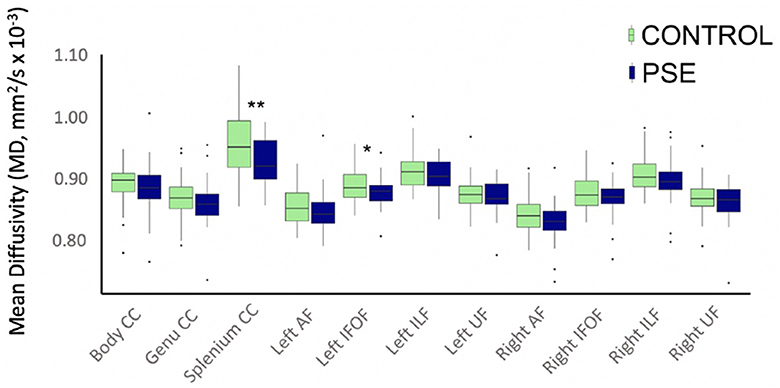
Figure 3. Boxplot displaying mean MD values of the white matter tracts for prenatal substance exposure (PSE; defined as prenatal alcohol and/or tobacco exposure) and control groups. MD values were directly pulled from the white matter for each participant interest variables have not been regressed out. MD was significantly lower in the PSE group compared to controls in the left IFOF (p = 0.030; q = 0.33) and the splenium of the CC (p = 0.002, q = 0.034). ILF, inferior longitudinal fasciculus; UF, uncinate fasciculus; IFOF, inferior fronto-occipital fasciculus; AF, arcuate fasciculus; CC, corpus callosum.
3.3 Substance exposure
PSE had both a main effect on language scores and a significant interaction with diffusion metrics in 9 of the 44 models (Tables 2, 3). Expressive Communication and FA models had significant PSE and FA interactions in the left IFOF (p = 0.015), and the body of the CC (p = 0.035), such that the PSE group had a more positive relationship between FA and Expressive Communication scores than the control group (Figure 4). There was an interaction effect between MD and PSE for Expressive Communication and MD in the bilateral ILF (L = 0.032; R = 0.037), right IFOF (p = 0.015), genu (p = 0.009) and body (p = 0.020) of the CC, and right AF (p = 0.014), such that the PSE group had a more negative relationship between MD and Expressive Communication than controls (Figure 5).
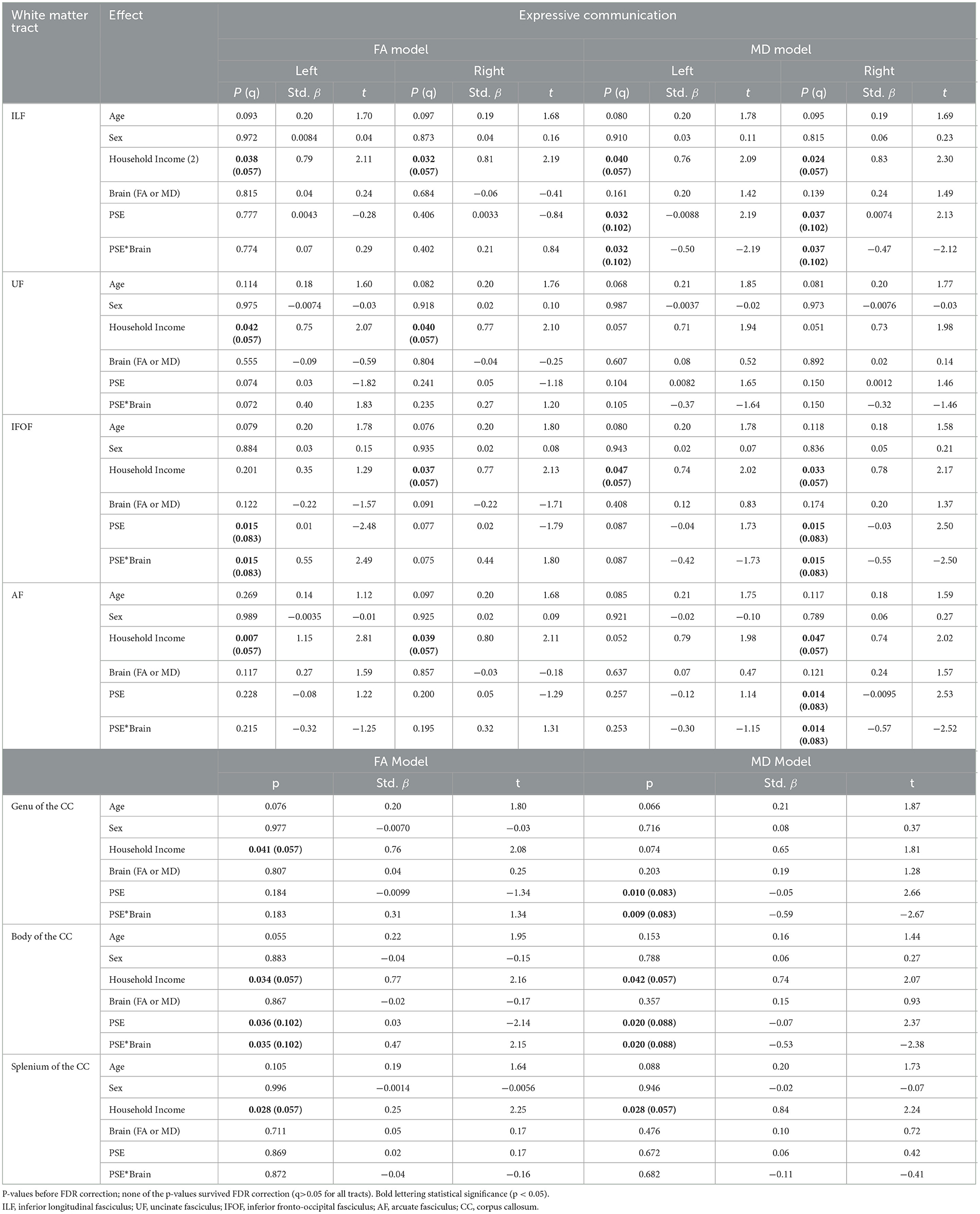
Table 2. The main effect of prenatal substance exposure (PSE), as well as the interaction effect of PSE and brain metrics for Expressive Communication models skills in 11 white matter tracts with p-values (p), standardized beta (Std. β), and test statistic (t).
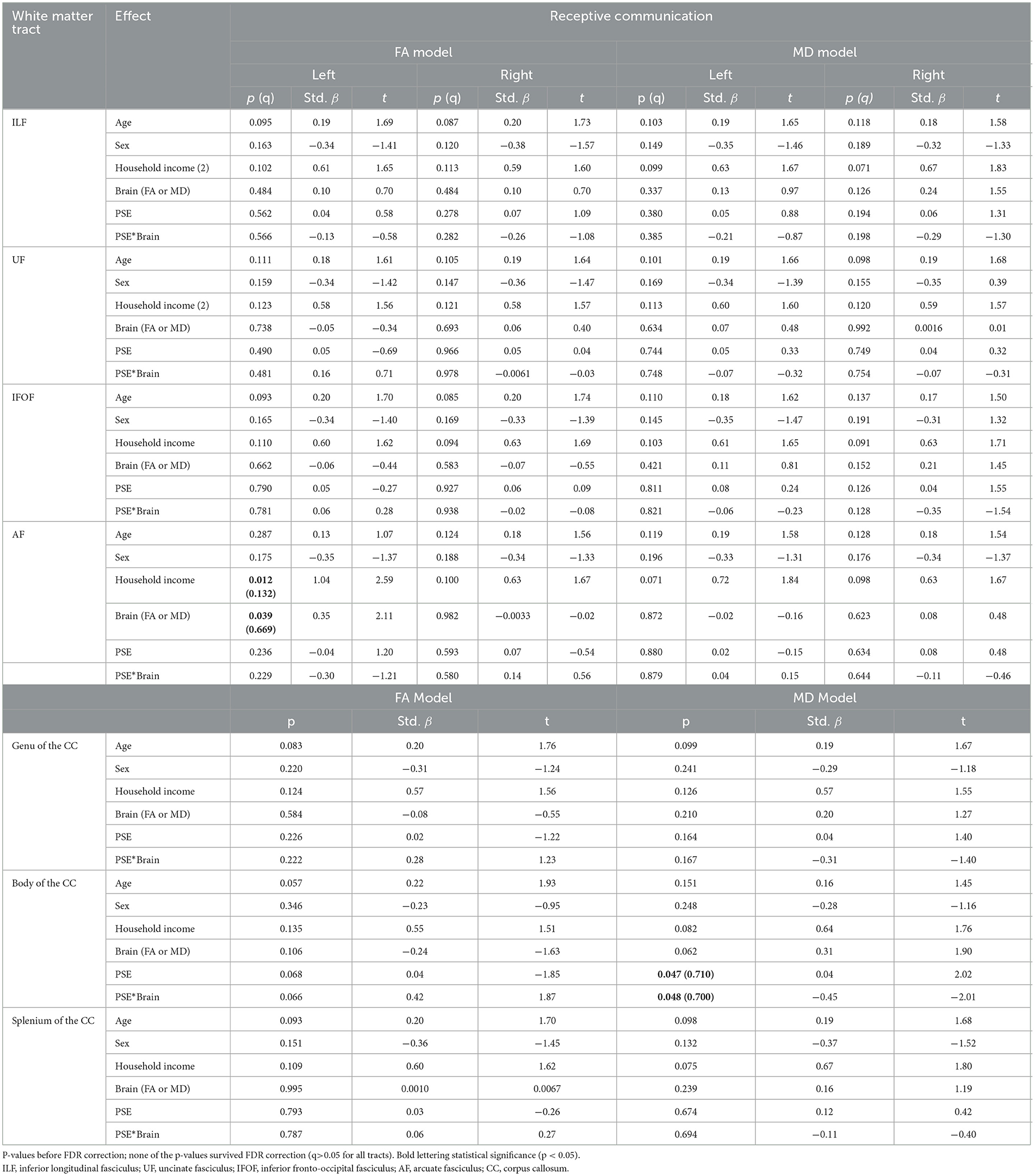
Table 3. The main effect of prenatal substance exposure (PSE), as well as the interaction effect of PSE and brain metrics for Receptive Communication models skills in 11 white matter tracts with p-values (p), standardized beta (Std. β), and the t-statistic.
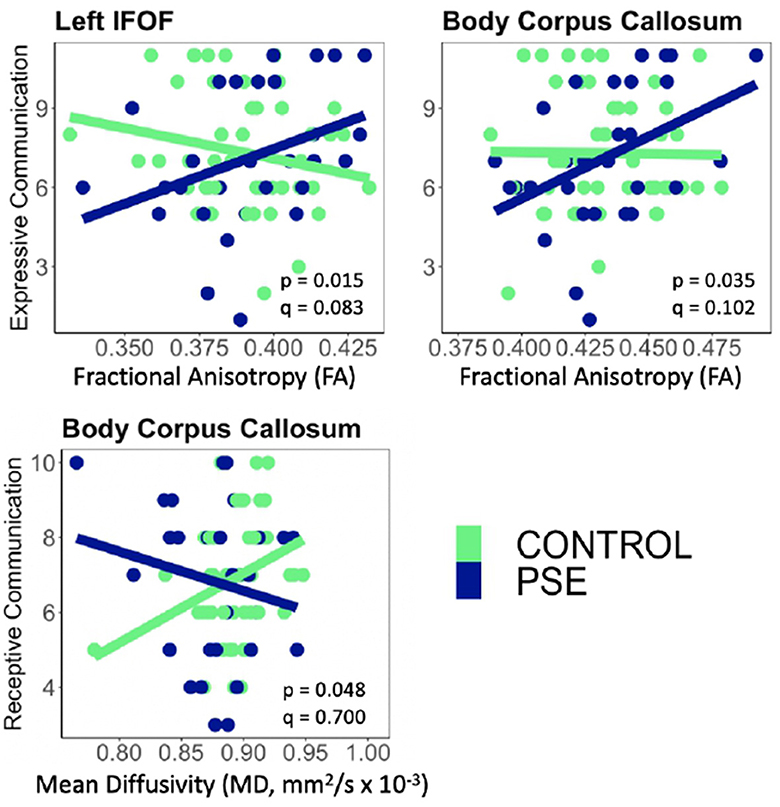
Figure 4. There were significant interactions between Prenatal Substance Exposure (PSE; defined as prenatal alcohol and/or tobacco exposure) and FA for Expressive Communication scores in the left IFOF and body of the CC. PSE had an interaction with MD in the body of the CC for Receptive Communication scores. Interaction effects did not survive multiple comparisons correction (q > 0.05 for all). The top row of plots displays FAxPSE interactions for Expressive Communication while the bottom row shows MDxPSE interactions for Receptive Communication. PSE group (n = 34) can be visualized in blue, and the control group (n = 59) is shown in green. IFOF, Inferior Fronto-Occipital Fasciculus.
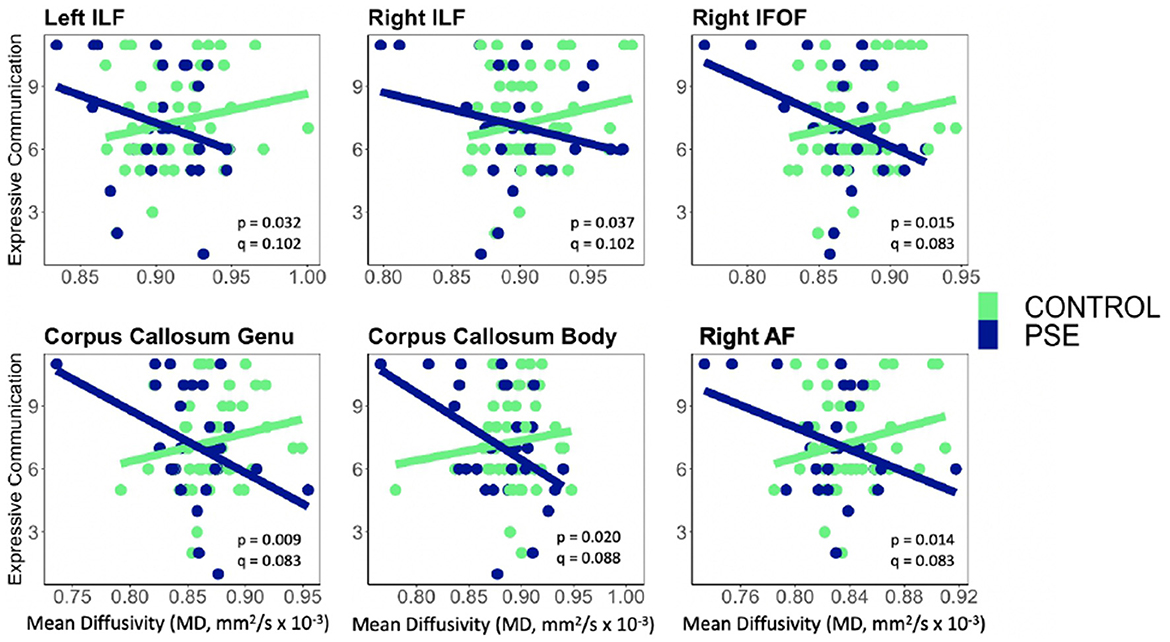
Figure 5. Prenatal Substance Exposure (PSE; defined as prenatal alcohol and/or tobacco exposure)had an interaction with MD in the bilateral ILF, right IFOF, genu and body of the CC, and right AF for Expressive Communication scores. None of these interaction effects survived multiple comparisons correction (q > 0.05 for all). PSE group (n = 34) can be visualized in blue, and the control group (n = 59) is shown in green. ILF, Inferior Longitudinal Fasciculus; IFOF, Inferior Fronto-Occipital Fasciculus; AF, Arcuate Fasciculus.
There were no significant interactions between PSE and FA for Receptive Communication models. There was an interaction effect between PSE and MD for Receptive Communication scores in the body of the CC (p = 0.048) similarly to the PSE and MD interactions with Expressive Communication. None of the interactions or main effects survived multiple comparison correction (all q > 0.05).
3.4 Socioeconomic status
Household income had a positive main effect on Expressive Communication for FA in all tracts, and for MD in all tracts except the bilateral UF, genu of the CC, and left AF (p = 0.007–0.038; q = 0.057–0.132). There was a main effect of household income in only the left AF for the Receptive Communication models.
3.5.1. Post hoc analyses–PAE and PTE
For Expressive Communication, PAE had a main effect and interaction effect with MD in the right IFOF (p = 0.017; 0.017, respectively), genu (p = 0.005; 0.005) and body (p = 0.019; 0.018) of the CC, and right AF (p = 0.020; 0.020). For Receptive Communication, PAE had a main effect and interaction with FA in the left AF (p = 0.048; 0.043, respectively). PAE had a main effect and interaction with MD for Receptive Communication in the body of the CC (p = 0.044, p = 0.045, respectively) (Supplementary Tables 1, 2). Similar to our main findings, children with PAE exhibited a more negative relationship between MD and Expressive and Receptive Communication scores, and a more positive relationship between FA and Receptive Communication scores, than controls.
PTE had main and interaction effects for the Receptive Communication and FA model in the body of the CC (p = 0.049; 0.046, respectively), and only an interaction effect in the right AF (p = 0.047) (Supplementary Tables 3, 4). Following the same trend as the PSE group and PAE independently, participants in the PTE group showed a positive relationship between FA and Receptive Communication scores and controls.
3.5.2. Post hoc analyses—cognitive scores
BSID-III Cognitive Scores did not differ between groups. There were no significant interaction effects between PSE and measures of white matter microstructure for the Cognitive Score models, nor were there main effects of PSE on brain metrics.
The previously seen effects in our main analysis became stronger when controlling for Cognitive Score as a covariate. PSE had a main effect and a significant interaction with diffusion metrics in more tracts than in our main analysis. The PSE main effect and PSE and FA interaction effect was significant in the left UF (p = 0.005, p = 0.004, respectively), left IFOF (p = 0.005, p = 0.004), genu (p = 0.022, p = 0.020) and body (p = 0.009, p = 0.008) of the CC for Expressive Communication scores. There were main effects of PSE and interaction effects of PSE and MD for Expressive Communication in the left UF (p = 0.026, p = 0.027, respectively), right UF (p = 0.017, p = 0.017), left IFOF (p = 0.013, p = 0.014), right IFOF (p = 0.003, p = 0.003), genu (p < 0.001, p < 0.001) and body (p = 0.047, p = 0.048) of the CC, and the right AF (p = 0.010, p = 0.010). PSE had a main effect and interaction with FA for Receptive Communication scores in the genu (p = 0.021, p = 0.019, respectively) and body (p = 0.007, p = 0.006) of the CC. PSE had a significant main effect on Receptive Communication scores and MD in the left IFOF (p = 0.048).
4 Discussion
Here, we show subtle differences in white matter microstructure in children with PSE, as well as a moderating effect of PSE on brain-language relationships, though these did not survive correction for multiple comparisons. In PSE, higher FA and/or lower MD were associated with better language performance in toddlers with PSE, while in controls, this relationship was weak and in the opposite direction. This altered relationship between white matter microstructure and language abilities may reflect early brain differences underlying language challenges that become more evident as children get older.
The PSE group had lower MD in the splenium of the corpus callosum compared to unexposed controls, and a nominally significant difference of MD in the IFOF that did not survive FDR correction. Since MD decreases with age across childhood (Lebel and Deoni, 2018), lower MD could suggest a premature pattern of white matter development. Indeed, altered development patterns have been noted in children with PAE across several prior studies (Kar et al., 2022; Donald et al., 2024). While most studies in older children with PAE report lower FA and/or higher MD compared to unexposed controls (Donald et al., 2015), a few studies have found higher FA and lower MD in brain white matter in 2–7-year-old children with PAE (Kar et al., 2021; Roos et al., 2021; Donald et al., 2024), and even older children with PAE (Lebel et al., 2008; Gómez et al., 2022). In two studies with overlapping datasets but using different methods, similar findings of higher FA and lower MD were observed. Very few studies have looked at white matter microstructure in children with PTE, and results have been somewhat mixed. Jacobsen et al. (2007) found higher FA in the genu of the corpus callosum in adolescents with PTE (many of whom were also current smokers), but young boys prenatally exposed to tobacco and methamphetamine had lower baseline FA and faster decreases in FA compared to unexposed controls when examined longitudinally (Chang et al., 2016). Further research, particularly longitudinal research, is needed to better understand the trajectories of FA and MD in children with PSE.
There were no significant group differences in Expressive or Receptive Communication scores. The existing literature shows conflicting results, especially in younger children, with some studies reporting worse expressive and receptive communication performance in young children with PAE (Fried and Watkinson, 1988, 1990; McGee et al., 2009; Davies et al., 2011), and others finding no language differences in the toddler years (Greene et al., 1990; Kaplan-Estrin et al., 1999; Hendricks et al., 2020). In general, the studies that found no language differences included participants with low-moderate PAE (Greene et al., 1990; Kaplan-Estrin et al., 1999), while studies of children with higher PAE reported worse language skills (McGee et al., 2009). This perhaps reflects a dose-dependent relationship, with language impairments being more evident in children with worse exposures. Our sample had a wide range of PAE, and moderate-to-high levels of PTE, though we did not have enough participants at each exposure level to test a dose-dependent relationship. Future studies are needed to examine the role of the amount of PSE on language difficulties in toddlers.
Higher FA and lower MD were associated with better language skills in the PSE group, while the control group showed weaker and opposite relationships. In typically developing older children and adults, higher FA and lower MD in several white matter tracts are consistently associated with better language performance (Ivanova et al., 2016; Mürner-Lavanchy et al., 2018), which is consistent with the relationships observed here in children with PSE. Indeed, subtle structural alterations in white matter may underlie language performance in the children with PSE and lead to the associations observed here. However, in contrast to our results, Gómez et al. (2022) reported a negative association between a language score component closely associated with speeded naming, and FA, in the genu of the corpus callosum in older children with PAE. This may reflect a complex role of age in these relationships, which is in line with altered development trajectories in children with PAE (Kar et al., 2022; Donald et al., 2024). Young children with PAE tend to have slower decreases of MD over time in several white matter tracts compared to unexposed controls, which may indicate reduced brain plasticity and/or a prolonged period of brain development, both have implications for cognitive outcomes and language abilities (Lebel et al., 2012; Kar et al., 2022; Donald et al., 2024). Longitudinal studies will help better elucidate these brain-language relationships across age.
In this study, household income was significantly associated with language scores, which is in line with prior studies of unexposed children (Noble et al., 2005; Schwab and Lew-Williams, 2016). Hemingway et al. (2020) found that PAE explained a higher proportion of variance in brain structure and function compared to other risk factors including socioeconomic status. Other studies have found attenuated or absent associations between current socioeconomic status (caregiver's educational attainment, occupation, and household income) and brain metrics in children with PAE (McLachlan et al., 2020; Uban et al., 2020). This may reflect the pre- to post-natal changes in socioeconomic factors for these children, who were primarily no longer living with their birth families. All participants in this study lived with their biological mother and came from households where incomes were lower than the mean annual household income for Statistics South Africa (2017) (R138,168/y, equivalent to ~$7,500/y USD; Statistics South Africa, 2017). Most previous studies investigating the effects of PAE and PTE were conducted on individuals coming from families with higher incomes, which may influence developmental outcomes of PAE (Uban et al., 2020). The geographical region and group of people that were investigated in the current study is one of the most impacted populations affected by PSE, making it an important cohort to study. It is possible that the influence of household income on brain and language outcomes varies at different levels of PSE and income, and/or when children remain in the same families (Hendricks et al., 2020).
We observed similar trends for the effects of PAE and PTE independently, as we did for the effects of PSE altogether. This suggests that at these levels, and in this population, PTE has similar effects on the brain-language relationship as PAE. It is worth noting, however, that many previous studies of PAE included participants with co-occurring PTE and/or other stubstances (Hendricks et al., 2019; Roos et al., 2021; Kar et al., 2022; Donald et al., 2024). Future, larger studies may be able to examine the interaction between the two exposures to provide more insight into combined or separate prenatal exposures.
Our results appear to reflect language abilities specifically, rather than more general intellectual ability, as the supplementary analysis for cognitive scores showed no effects. Furthermore, when adding cognitive scores as a covariate in our language models, we saw the same trends as in our main analysis but with stronger relationships.
One limitation of this study is the small sample size, preventing us from examining interactions between alcohol and tobacco exposure. This also limited our ability to detect significant moderation effects, and none of these survived multiple comparison corrections.
In conclusion, we show that PSE appears to moderate the brain-language relationship in early life. While language deficits have not yet emerged, the altered brain-language relationship in the toddler years could be the basis of language challenges in older children with PSE. Substance-exposed children could face different challenges than unexposed children as they begin to engage in more complicated language activities including learning to read. Furthermore, this highlights the importance of developing early interventions for children with PSE to mitigate the development of language challenges.
Data availability statement
The data analyzed in this study is subject to the following licenses/restrictions: Data will be made available on request. Requests to access these datasets should be directed to a2lyc3R5LmRvbmFsZEB1Y3QuYWMuemE=.
Ethics statement
The studies involving humans were approved by University of Cape Town Human Research Ethics Committee. The studies were conducted in accordance with the local legislation and institutional requirements. Written informed consent for participation in this study was provided by the participants' legal guardians/next of kin.
Author contributions
CS: Conceptualization, Data curation, Formal analysis, Investigation, Methodology, Validation, Visualization, Writing – original draft, Writing – review & editing. MG: Conceptualization, Data curation, Formal analysis, Investigation, Methodology, Project administration, Supervision, Validation, Visualization, Writing – review & editing. BG: Conceptualization, Data curation, Methodology, Visualization, Writing – review & editing. SJ: Conceptualization, Data curation, Visualization, Writing – review & editing. CW: Data curation, Writing – review & editing, Funding acquisition. AR: Data curation, Writing – review & editing. SS: Methodology, Writing – review & editing, Data curation. NH: Data curation, Methodology, Project administration, Writing – review & editing. KN: Conceptualization, Writing – review & editing. RW: Conceptualization, Writing – review & editing. HZ: Conceptualization, Writing – review & editing, Funding acquisition. DS: Conceptualization, Writing – review & editing, Funding acquisition. KD: Conceptualization, Data curation, Funding acquisition, Investigation, Methodology, Project administration, Validation, Visualization, Writing – review & editing. CL: Conceptualization, Data curation, Formal analysis, Funding acquisition, Investigation, Methodology, Resources, Supervision, Validation, Visualization, Writing – original draft, Writing – review & editing.
Funding
The author(s) declare financial support was received for the research, authorship, and/or publication of this article. This study was funded by the Jacobs Foundation, and Bill and Melinda Gates Foundation (OPP 1017641) and this particular sub-study was supported through a US Brain and Behavior Foundation Independent Investigator grant (24467) awarded to KD. KD and additional aspects of the research were supported by the SA National Research Foundation (NRF), an Academy of Medical Sciences Newton Advanced Fellowship (NAF002/1001) funded by the UK Government's Newton Fund, by NIAAA via (R21AA023887 and R01 AA026834-01), by the Collaborative Initiative on Fetal Alcohol Spectrum Disorders (CIFASD) developmental grant (U24 AA014811). Additional support for HZ and DS was provided by the Medical Research Council of South Africa. CW was supported by the Wellcome Trust through a Research Training Fellowship [203525/Z/16/Z]. AR was supported by the SA NRF. MG was supported by the Hotchkiss Brain Institute. CL receives salary support from the Canada Research Chairs Program.
Acknowledgments
We greatly thank the parents and children who participated in this study. We would also like to thank the study staff in Paarl, the study data team, and the clinical and administrative staff of the Western Cape Government Health Department at Paarl Hospital and at the clinics for support of the study.
Conflict of interest
The authors declare that the research was conducted in the absence of any commercial or financial relationships that could be construed as a potential conflict of interest.
The author(s) declared that they were an editorial board member of Frontiers, at the time of submission. This had no impact on the peer review process and the final decision.
Publisher's note
All claims expressed in this article are solely those of the authors and do not necessarily represent those of their affiliated organizations, or those of the publisher, the editors and the reviewers. Any product that may be evaluated in this article, or claim that may be made by its manufacturer, is not guaranteed or endorsed by the publisher.
Supplementary material
The Supplementary Material for this article can be found online at: https://www.frontiersin.org/articles/10.3389/fnint.2024.1438888/full#supplementary-material
References
Abel, E. L. (1984). Prenatal effects of alcohol. Drug Alcohol Depend. 14, 1–10. doi: 10.1016/0376-8716(84)90012-7
Abel, E. L. (1985). Prenatal effects of alcohol on growth: a brief overview. Fed. Proc. 44, 2318–2322.
Basser, P. J., and Jones, D. K. (2002). Diffusion-tensor MRI: theory, experimental design and data analysis - a technical review. NMR Biomed. 15, 456–467. doi: 10.1002/nbm.783
Beaulieu, C. (2002). The basis of anisotropic water diffusion in the nervous system—A technical review. NMR Biomed. 15, 435–455. doi: 10.1002/nbm.782
Behnke, M., and Eyler, F. D. (1993). The consequences of prenatal substance use for the developing fetus, newborn, and young child. Int. J. Addict. 28, 1341–1391. doi: 10.3109/10826089309062191
Bosco, C., and Diaz, E. (2012). Placental hypoxia and foetal development versus alcohol exposure in pregnancy. Alcohol Alcohol. 47, 109–117. doi: 10.1093/alcalc/agr166
Caputo, C., Wood, E., and Jabbour, L. (2016). Impact of fetal alcohol exposure on body systems: a systematic review. Birth Defects Res. 108, 174–180. doi: 10.1002/bdrc.21129
Chang, L., Oishi, K., Skranes, J., Buchthal, S., Cunningham, E., Yamakawa, R., et al. (2016). Sex-specific alterations of white matter developmental trajectories in infants with prenatal exposure to methamphetamine and tobacco. JAMA Psychiat. 73, 1217–1227. doi: 10.1001/jamapsychiatry.2016.2794
Colby, J. B., Smith, L., O'Connor, M. J., Bookheimer, S. Y., Van Horn, J. D., and Sowell, E. R. (2012). White matter microstructural alterations in children with prenatal methamphetamine/polydrug exposure. Psychiatry Res. 204, 140–148. doi: 10.1016/j.pscychresns.2012.04.017
Davies, L., Dunn, M., Chersich, M., Urban, M., Chetty, C., Olivier, L., et al. (2011). Developmental delay of infants and young children with and without fetal alcohol spectrum disorder in the Northern Cape Province, South Africa. Afr. J. Psychiatry. 14, 298–305. doi: 10.4314/ajpsy.v14i4.7
Donald, K. A., Eastman, E., Howells, F. M., Adnams, C., Riley, E. P., Woods, R. P., et al. (2015). Neuroimaging effects of prenatal alcohol exposure on the developing human brain: A magnetic resonance imaging review. Acta Neuropsychiatr. 27, 251–269. doi: 10.1017/neu.2015.12
Donald, K. A., Hendrikse, C. J., Roos, A., Wedderburn, C. J., Subramoney, S., Ringshaw, J. E., et al. (2024). Prenatal alcohol exposure and white matter microstructural changes across the first 6–7 years of life: a longitudinal diffusion tensor imaging study of a South African birth cohort. NeuroImage: Clini. 41:103572. doi: 10.1016/j.nicl.2024.103572
Donald, K. A., Hoogenhout, M., du Plooy, C. P., Wedderburn, C. J., Nhapi, R. T., Barnett, W., et al. (2018). Drakenstein Child Health Study (DCHS): investigating determinants of early child development and cognition. BMJ Paediatrics Open 2:e000282. doi: 10.1136/bmjpo-2018-000282
Dukes, K., Tripp, T., Willinger, M., Odendaal, H., Elliott, A. J., Kinney, H. C., et al. (2017). Drinking and smoking patterns during pregnancy: development of group-based trajectories in the Safe Passage Study. Alcohol 62, 49–60. doi: 10.1016/j.alcohol.2017.03.001
Ernst, M., Moolchan, E. T., and Robinson, M. L. (2001). Behavioral and neural consequences of prenatal exposure to nicotine. J. Am. Acad. Child & Adolesc. Psychiatry 40, 630–641. doi: 10.1097/00004583-200106000-00007
Espy, K. A., Fang, H., Johnson, C., Stopp, C., Wiebe, S. A., and Respass, J. (2011). Prenatal tobacco exposure: developmental outcomes in the neonatal period. Dev. Psychol. 47, 153–156. doi: 10.1037/a0020724
Fried, P. A., O'Connell, C. M., and Watkinson, B. (1992). 60- and 72-month follow-up of children prenatally exposed to marijuana, cigarettes, and alcohol: cognitive and language assessment. J. Dev. Behav. Pediat. 13, 383–391. doi: 10.1097/00004703-199212000-00001
Fried, P. A., and Watkinson, B. (1988). 12- and 24-month neurobehavioural follow-up of children prenatally exposed to marihuana, cigarettes and alcohol. Neurotoxicol. Teratol. 10, 305–313. doi: 10.1016/0892-0362(88)90032-3
Fried, P. A., and Watkinson, B. (1990). 36- and 48-month neurobehavioral follow-up of children prenatally exposed to marijuana, cigarettes, and alcohol. J. Dev. Behav. Pediat. 11, 49–58. doi: 10.1097/00004703-199004000-00003
Gómez, M. J. C., Beaulieu, C., McMorris, C. A., Gibbard, B., Tortorelli, C., and Lebel, C. (2022). Frontoparietal and temporal white matter diffusion MRI in children and youth with prenatal alcohol exposure. Alcoholism 46, 1808–1818. doi: 10.1111/acer.14929
Gonzalez, M. R., Uban, K. A., Tapert, S. F., and Sowell, E. R. (2023). Prenatal tobacco exposure associations with physical health and neurodevelopment in the ABCD cohort. Health Psychol. 42, 856–867. doi: 10.1037/hea0001265
Greene, T., Emhart, C. B., Martier, S., Sokol, R., and Ager, J. (1990). Prenatal alcohol exposure and language development. Alcoholism 14, 937–945. doi: 10.1111/j.1530-0277.1990.tb01842.x
Heath, C. J., and Picciotto, M. R. (2009). Nicotine-induced plasticity during development: Modulation of the cholinergic system and long-term consequences for circuits involved in attention and sensory processing. Neuropharmacology 56, 254–262. doi: 10.1016/j.neuropharm.2008.07.020
Hemingway, S. J. A., Davies, J. K., Jirikowic, T., and Olson, E. M. (2020). What proportion of the brain structural and functional abnormalities observed among children with fetal alcohol spectrum disorder is explained by their prenatal alcohol exposure and their other prenatal and postnatal risks? Adv. Pediat. Res. 7:41.
Hendricks, G., Malcolm-Smith, S., Adnams, C., Stein, D. J., and Donald, K. A. M. (2019). Effects of prenatal alcohol exposure on language, speech and communication outcomes: a review longitudinal studies. Acta Neuropsychiatr. 31, 74–83. doi: 10.1017/neu.2018.28
Hendricks, G., Malcolm-Smith, S., Stein, D. J., Zar, H. J., Wedderburn, C. J., Nhapi, R. T., et al. (2020). Prenatal alcohol exposure is associated with early motor, but not language development in a South African cohort. Acta Neuropsychiatr. 32, 145–152. doi: 10.1017/neu.2019.51
Hernández-Martínez, C., Voltas Moreso, N., Ribot Serra, B., Arija Val, V., Escribano Macías, J., and Canals Sans, J. (2017). Effects of prenatal nicotine exposure on infant language development: a cohort follow up study. Matern. Child Health J. 21, 734–744. doi: 10.1007/s10995-016-2158-y
Humeniuk, R., Ali, R., Babor, T. F., Farrell, M., Formigoni, M. L., Jittiwutikarn, J., et al. (2008). Validation of the alcohol, smoking and substance involvement screening test (ASSIST). Addiction 103, 1039–1047. doi: 10.1111/j.1360-0443.2007.02114.x
Ivanova, M. V., Isaev, D. Y., Dragoy, O. V., Akinina, Y. S., Petrushevskiy, A. G., Fedina, O. N., et al. (2016). Diffusion-tensor imaging of major white matter tracts and their role in language processing in aphasia. Cortex 85, 165–181. doi: 10.1016/j.cortex.2016.04.019
Jacobsen, L. K., Picciotto, M. R., Heath, C. J., Frost, S. J., Tsou, K. A., Dwan, R. A., et al. (2007). Prenatal and adolescent exposure to tobacco smoke modulates the development of white matter microstructure. J. Neurosci. 27, 13491–13498. doi: 10.1523/JNEUROSCI.2402-07.2007
Jones, P. J. H., Leichter, J., and Lee, M. (1981). Placental blood flow in rats fed alcohol before and during gestation. Life Sci. 29, 1153–1159. doi: 10.1016/0024-3205(81)90204-6
Kaplan-Estrin, M., Jacobson, S. W., and Jacobson, J. L. (1999). Neurobehavioral effects of prenatal alcohol exposure at 26 months. Neurotoxicol. Teratol. 21, 503–511. doi: 10.1016/S0892-0362(99)00031-8
Kar, P., Reynolds, J. E., Gibbard, W. B., McMorris, C., Tortorelli, C., and Lebel, C. (2022). Trajectories of brain white matter development in young children with prenatal alcohol exposure. Hum. Brain Mapp. 43, 4145–4157. doi: 10.1002/hbm.25944
Kar, P., Reynolds, J. E., Grohs, M. N., Gibbard, W. B., McMorris, C., Tortorelli, C., et al. (2021). White matter alterations in young children with prenatal alcohol exposure. Dev. Neurobiol. 81, 400–410. doi: 10.1002/dneu.22821
Kargar, Y., and Jalilian, M. (2024). Anatomo-functional profile of white matter tracts in relevance to language: a systematic review. J. Neurolinguistics 69:101175. doi: 10.1016/j.jneuroling.2023.101175
Lange, S., Probst, C., Rehm, J., and Popova, S. (2018). National, regional, and global prevalence of smoking during pregnancy in the general population: a systematic review and meta-analysis. The Lancet Global Health 6, e769–e776. doi: 10.1016/S2214-109X(18)30223-7
Lebel, C., and Deoni, S. (2018). The development of brain white matter microstructure. Neuroimage 182, 207–218. doi: 10.1016/j.neuroimage.2017.12.097
Lebel, C., Mattson, S. N., Riley, E. P., Jones, K. L., Adnams, C. M., May, P. A., et al. (2012). A Longitudinal study of the long-term consequences of drinking during pregnancy: heavy in utero alcohol exposure disrupts the normal processes of brain development. J. Neurosci. 32, 15243–15251. doi: 10.1523/JNEUROSCI.1161-12.2012
Lebel, C., Rasmussen, C., Wyper, K., Walker, L., Andrew, G., Yager, J., et al. (2008). Brain diffusion abnormalities in children with fetal alcohol spectrum disorder. Alcohol. Clin. Exp. Res. 32, 1732–1740. doi: 10.1111/j.1530-0277.2008.00750.x
Leemans, A., Jeurissen, B., Sijbers, J., and Jones, D. K. (2009). “ExploreDTI: A graphical toolbox for processing, analyzing, and visualizing diffusion MR data,” in 17th Annual Meeting of Intl Soc Mag Reson Med, 17.
Lees, B., Mewton, L., Jacobus, J., Valadez, E. A., Stapinski, L. A., Teesson, M., et al. (2020). Association of prenatal alcohol exposure with psychological, behavioral, and neurodevelopmental outcomes in children from the adolescent brain cognitive development study. Am. J. Psychiatry 177, 1060–1072. doi: 10.1176/appi.ajp.2020.20010086
Lindinger, N. M., Jacobson, S. W., Davidson, L., Conradie, S., Dodge, N. C., Molteno, C. D., et al. (2022). Reading impairment in adolescents with fetal alcohol spectrum disorders. Scientific Stud. Read. 26, 469–488. doi: 10.1080/10888438.2022.2054717
Lo, J. O., Schabel, M. C., Roberts, V. H. J., Wang, X., Lewandowski, K. S., Grant, K. A., et al. (2017). First trimester alcohol exposure alters placental perfusion and fetal oxygen availability affecting fetal growth and development in a non-human primate model. Am. J. Obstet. Gynecol. 216, 302.e1–302.e8. doi: 10.1016/j.ajog.2017.01.016
Margolis, A. E., Pagliaccio, D., Ramphal, B., Banker, S., Thomas, L., Robinson, M., et al. (2021). Prenatal environmental tobacco smoke exposure alters children's cognitive control circuitry: a preliminary study. Environ. Int. 155:106516. doi: 10.1016/j.envint.2021.106516
McGee, C. L., Bjorkquist, O. A., Riley, E. P., and Mattson, S. N. (2009). Impaired language performance in young children with heavy prenatal alcohol exposure. Neurotoxicol. Teratol. 31, 71–75. doi: 10.1016/j.ntt.2008.09.004
McLachlan, K., Zhou, D., Little, G., Rasmussen, C., Pei, J., Andrew, G., et al. (2020). Current socioeconomic status correlates with brain volumes in healthy children and adolescents but not in children with prenatal alcohol exposure. Front. Human Neurosci. 14:223. doi: 10.3389/fnhum.2020.00223
Miller, M. W. (1986). Effects of alcohol on the generation and migration of cerebral cortical neurons. Science 233, 1308–1311. doi: 10.1126/science.3749878
Mills, B. D., Lai, J., Brown, T. T., Erhart, M., Halgren, E., Reilly, J., et al. (2013). White matter microstructure correlates of narrative production in typically developing children and children with high functioning autism. Neuropsychologia 51, 1933–1941. doi: 10.1016/j.neuropsychologia.2013.06.012
Moore, E. M., and Xia, Y. (2022). Neurodevelopmental trajectories following prenatal alcohol exposure. Front. Hum. Neurosci. 15:695855. doi: 10.3389/fnhum.2021.695855
Mukherjee, A. B., and Hodgen, G. D. (1982). Maternal ethanol exposure induces transient impairment of umbilical circulation and fetal hypoxia in monkeys. Science 218, 700–702. doi: 10.1126/science.6890235
Mürner-Lavanchy, I. M., Kelly, C. E., Reidy, N., Doyle, L. W., Lee, K. J., Inder, T., et al. (2018). White matter microstructure is associated with language in children born very preterm. NeuroImage. Clinical 20, 808–822. doi: 10.1016/j.nicl.2018.09.020
Navarro, H. A., Seidler, F. J., Eylers, J. P., Baker, F. E., Dobbins, S. S., Lappi, S. E., et al. (1989). Effects of prenatal nicotine exposure on development of central and peripheral cholinergic neurotransmitter systems. Evidence for cholinergic trophic influences in developing brain. J. Pharmacol. Exp. Therapeut. 251, 894–900.
Noble, K. G., Norman, M. F., and Farah, M. J. (2005). Neurocognitive correlates of socioeconomic status in kindergarten children. Dev. Sci. 8, 74–87. doi: 10.1111/j.1467-7687.2005.00394.x
Ostertag, C., Reynolds, J. E., Kar, P., Dewey, D., Gibbard, W. B., Tortorelli, C., et al. (2023). Arcuate fasciculus and pre-reading language development in children with prenatal alcohol exposure. Front. Neurosci. 17:1174165. doi: 10.3389/fnins.2023.1174165
Peixinho, J., Toseeb, U., Mountford, H. S., Bermudez, I., and Newbury, D. F. (2022). The effects of prenatal smoke exposure on language development—A systematic review. Infant Child Dev. 31:e2331. doi: 10.1002/icd.2331
Petersen Williams, P., Jordaan, E., Mathews, C., Lombard, C., and Parry, C. D. H. (2014). Alcohol and other drug use during pregnancy among women attending midwife obstetric units in the Cape Metropole, South Africa. Adv. Prev. Med. 2014:e871427. doi: 10.1155/2014/871427
Phaswana-Mafuya, N., Peltzer, K., and Pengpid, S. (2019). Maternal tobacco use during pregnancy in South Africa: results from a national population-based survey. Int. J. Prev. Med. 10:99. doi: 10.4103/ijpvm.IJPVM_212_18
Popova, S., Charness, M. E., Burd, L., Crawford, A., Hoyme, H. E., Mukherjee, R. A. S., et al. (2023). Fetal alcohol spectrum disorders. Nat. Rev. Dis. Primers 9, 1–21. doi: 10.1038/s41572-023-00420-x
R Core Team (2021). R: A Language and Environment for Statistical Computing. Vienna: R Foundation for Statistical Computing. Available at: https://www.R-project.org/
Roos, A., Wedderburn, C. J., Fouche, J.-P., Subramoney, S., Joshi, S. H., Woods, R. P., et al. (2021). Central white matter integrity alterations in 2-3-year-old children following prenatal alcohol exposure. Drug Alcohol Depend. 225:108826. doi: 10.1016/j.drugalcdep.2021.108826
Saygin, Z. M., Norton, E. S., Osher, D. E., Beach, S. D., Cyr, A. B., Ozernov-Palchik, O., et al. (2013). Tracking the roots of reading ability: white matter volume and integrity correlate with phonological awareness in prereading and early-reading kindergarten children. J. Neurosci. 33, 13251–13258. doi: 10.1523/JNEUROSCI.4383-12.2013
Schwab, J. F., and Lew-Williams, C. (2016). Language learning, socioeconomic status, and child-directed speech. Wiley Interdiscip. Rev. Cogn. Sci. 7, 264–275. doi: 10.1002/wcs.1393
Sket, G. M., Overfeld, J., Styner, M., Gilmore, J. H., Entringer, S., Wadhwa, P. D., et al. (2019). Neonatal White matter maturation is associated with infant language development. Front. Hum. Neurosci. 13:434. doi: 10.3389/fnhum.2019.00434
Smits, M., Jiskoot, L. C., and Papma, J. M. (2014). White matter tracts of speech and language. Seminars in Ultrasound, CT and MRI 35, 504–516. doi: 10.1053/j.sult.2014.06.008
Statistics South Africa (2017). Living Conditions of Household in South Africa. Available at: https://www.statssa.gov.za/publications/P0310/P03102014.pdf
Stein, D. J., Koen, N., Donald, K. A., Adnams, C. M., Koopowitz, S., Lund, C., et al. (2015). Investigating the psychosocial determinants of child health in Africa: The Drakenstein Child Health Study. J. NEUROSCI. METH. 252, 27–35. doi: 10.1016/j.jneumeth.2015.03.016
Tax, C. M. W., Otte, W. M., Viergever, M. A., Dijkhuizen, R. M., and Leemans, A. (2015). REKINDLE: Robust extraction of kurtosis INDices with linear estimation. Magnetic Reson. Med. 73, 794–808. doi: 10.1002/mrm.25165
The MathWorks Inc (2022). MATLAB version 9.6.0.1472908. Natick, Massachusetts: The MathWorks Inc. Available at: https://www.mathworks.com
Treit, S., Lebel, C., Baugh, L., Rasmussen, C., Andrew, G., and Beaulieu, C. (2013). Longitudinal MRI reveals altered trajectory of brain development during childhood and adolescence in fetal alcohol spectrum disorders. J. Neurosci. 33, 10098–10109. doi: 10.1523/JNEUROSCI.5004-12.2013
Uban, K. A., Kan, E., Wozniak, J. R., Mattson, S. N., Coles, C. D., and Sowell, E. R. (2020). The relationship between socioeconomic status and brain volume in children and adolescents with prenatal alcohol exposure. Front. Hum. Neurosci. 14:85. doi: 10.3389/fnhum.2020.00085
Vandermosten, M., Boets, B., Wouters, J., and Ghesquière, P. (2012). A qualitative and quantitative review of diffusion tensor imaging studies in reading and dyslexia. Neurosci. Biobehav. Rev. 36, 1532–1552. doi: 10.1016/j.neubiorev.2012.04.002
Vandermosten, M., Vanderauwera, J., Theys, C., De Vos, A., Vanvooren, S., Sunaert, S., et al. (2015). A DTI tractography study in pre-readers at risk for dyslexia. Dev. Cogn. Neurosci. 14, 8–15. doi: 10.1016/j.dcn.2015.05.006
Veazey, K. J., Muller, D., and Golding, M. C. (2013). Prenatal alcohol exposure and cellular differentiation. Alcohol Res.: Curr. Rev. 35, 77–85.
Walton, M., Dewey, D., and Lebel, C. (2018). Brain white matter structure and language ability in preschool-aged children. Brain Lang. 176, 19–25. doi: 10.1016/j.bandl.2017.10.008
Ward, C., Lewis, S., and Coleman, T. (2007). Prevalence of maternal smoking and environmental tobacco smoke exposure during pregnancy and impact on birth weight: Retrospective study using Millennium Cohort. BMC Public Health 7:81. doi: 10.1186/1471-2458-7-81
Wedderburn, C. J., Subramoney, S., Yeung, S., Fouche, J.-P., Joshi, S. H., Narr, K. L., et al. (2020). Neuroimaging young children and associations with neurocognitive development in a South African birth cohort study. NeuroImage 219, 116846–116846. doi: 10.1016/j.neuroimage.2020.116846
WHO ASSIST Working Group (2002). The alcohol, smoking and substance involvement screening test (ASSIST): Development, reliability and feasibility. Addiction 97, 1183–1194. doi: 10.1046/j.1360-0443.2002.00185.x
Yen, C., Lin, C.-L., and Chiang, M.-C. (2023). Exploring the Frontiers of Neuroimaging: a review of recent advances in understanding brain functioning and disorders. Life 13:1472. doi: 10.3390/life13071472
Keywords: diffusion tensor imaging (DTI), early language, prenatal tobacco exposure, prenatal alcohol exposure (PAE), development
Citation: Scholten C, Ghasoub M, Geeraert B, Joshi S, Wedderburn CJ, Roos A, Subramoney S, Hoffman N, Narr K, Woods R, Zar HJ, Stein DJ, Donald K and Lebel C (2024) Prenatal tobacco and alcohol exposure, white matter microstructure, and early language skills in toddlers from a South African birth cohort. Front. Integr. Neurosci. 18:1438888. doi: 10.3389/fnint.2024.1438888
Received: 26 May 2024; Accepted: 12 August 2024;
Published: 02 September 2024.
Edited by:
Avantika Mathur, Vanderbilt University, United StatesReviewed by:
Vincent Frouin, Neurospin, FranceJulia M. Stephen, Mind Research Network (MRN), United States
Copyright © 2024 Scholten, Ghasoub, Geeraert, Joshi, Wedderburn, Roos, Subramoney, Hoffman, Narr, Woods, Zar, Stein, Donald and Lebel. This is an open-access article distributed under the terms of the Creative Commons Attribution License (CC BY). The use, distribution or reproduction in other forums is permitted, provided the original author(s) and the copyright owner(s) are credited and that the original publication in this journal is cited, in accordance with accepted academic practice. No use, distribution or reproduction is permitted which does not comply with these terms.
*Correspondence: Mohammad Ghasoub, bW9oYW1tYWQuZ2hhc291YkB1Y2FsZ2FyeS5jYQ==; Catherine Lebel, Y2xlYmVsQHVjYWxnYXJ5LmNh
†These authors share senior authorship