- 1 Institute of Neuroscience and Medicine – Neuromodulation, Research Center Jülich, Jülich, Germany
- 2 Department of Stereotactic and Functional Neurosurgery, University of Cologne, Cologne, Germany
Chronic subjective tinnitus is characterized by abnormal neuronal synchronization in the central auditory system. As shown in a controlled clinical trial, acoustic coordinated reset (CR) neuromodulation causes a significant relief of tinnitus symptoms along with a significant decrease of pathological oscillatory activity in a network comprising auditory and non-auditory brain areas, which is often accompanied with a significant tinnitus pitch change. Here we studied if the tinnitus pitch change correlates with a reduction of tinnitus loudness and/or annoyance as assessed by visual analog scale (VAS) scores. Furthermore, we studied if the changes of the pattern of brain synchrony in tinnitus patients induced by 12 weeks of CR therapy depend on whether or not the patients undergo a pronounced tinnitus pitch change. Therefore, we applied standardized low-resolution brain electromagnetic tomography (sLORETA) to EEG recordings from two groups of patients with a sustained CR-induced relief of tinnitus symptoms with and without tinnitus pitch change. We found that absolute changes of VAS loudness and VAS annoyance scores significantly correlate with the modulus, i.e., the absolute value, of the tinnitus pitch change. Moreover, as opposed to patients with small or no pitch change we found a significantly stronger decrease in gamma power in patients with pronounced tinnitus pitch change in right parietal cortex (Brodmann area, BA 40), right frontal cortex (BA 9, 46), left temporal cortex (BA 22, 42), and left frontal cortex (BA 4, 6), combined with a significantly stronger increase of alpha (10–12 Hz) activity in the right and left anterior cingulate cortex (ACC; BA 32, 24). In addition, we revealed a significantly lower functional connectivity in the gamma band between the right dorsolateral prefrontal cortex (BA 46) and the right ACC (BA 32) after 12 weeks of CR therapy in patients with pronounced pitch change. Our results indicate a substantial, CR-induced reduction of tinnitus-related auditory binding in a pitch processing network.
Introduction
Non-pulsatile subjective tinnitus is an auditory phantom phenomenon that is present in up to 15% of the population (Eggermont and Roberts, 2004). Tinnitus generation is considered to have a central basis, typically being initiated by damage to the peripheral hearing system (Eggermont and Roberts, 2004; Weisz et al., 2005, 2006). Cortical map reorganization is related to tinnitus (Robertson and Irvine, 1989; Muhlnickel et al., 1998; Lockwood et al., 2002; Moller, 2003; Yang et al., 2011). However, according to an MEG study, map reorganization cannot explain the emergence of tinnitus in a satisfactory manner (Weisz et al., 2006). Another important tinnitus-related phenomenon is the emergence of pathological neural synchrony (Ochi and Eggermont, 1997; Norena and Eggermont, 2003; Seki and Eggermont, 2003; Weisz et al., 2005, 2007; De Ridder et al., 2011b). In general, pathologically increased delta activity emerges in cortical regions deprived of afferent input (Steriade, 2006). In the context of tinnitus, pathologically enhanced neuronal synchronization was observed in the primary auditory cortex of animals following damage to the inner ear (Ochi and Eggermont, 1997; Norena and Eggermont, 2003; Seki and Eggermont, 2003) as well as in tinnitus patients (Llinas et al., 1999; Weisz et al., 2005, 2007; De Ridder et al., 2011b). Apart from a significant reduction of alpha band power (Weisz et al., 2005) abnormally strong synchrony was observed in tinnitus patients in the delta band (Weisz et al., 2005), theta band (De Ridder et al., 2011b), and gamma band (Weisz et al., 2007), e.g., in temporal areas. Several studies confirmed that pathological neural synchronization is related to the tinnitus percept (Dohrmann et al., 2007; Kahlbrock and Weisz, 2008; De Ridder et al., 2011b). However, a conscious perception of the tinnitus requires the involvement of larger networks of brain areas relevant to awareness and salience (De Ridder et al., 2011a).
Indeed, apart from auditory cortical areas also non-auditory areas such as amygdala, cingulate cortex, and parahippocampus are involved in the tinnitus generation, in particular, in patients with tinnitus distress (Rauschecker, 2005; Vanneste et al., 2010; De Ridder et al., 2011a). Furthermore, an altered functional interaction between non-auditory and auditory areas with abnormal synchrony seems to be a hallmark of tinnitus distress (Schlee et al., 2009a).
To specifically counteract the electrophysiological correlate of tinnitus, i.e., the pathological neural synchrony, in a previous proof of concept study we have used a non-invasive desynchronizing stimulation technique, acoustic coordinated reset (CR) neuromodulation (Tass et al., 2012). CR neuromodulation has been developed computationally (Tass, 1999, 2003b) and is essentially based on fundamental dynamical self-organization principles (Tass, 2003a, b) as well as on the intimate relationship between neuronal dynamics and connectivity (Yuste and Bonhoeffer, 2004). As shown in theoretical and computational studies, CR neuromodulation causes a desynchronization (Tass, 2003b) which in turn shifts neural networks with spike timing-dependent plasticity (Gerstner et al., 1996; Markram, 1997) from a synchronized state with strong synaptic connectivity to a desynchronized state with weak connectivity (Tass and Majtanik, 2006; Hauptmann et al., 2007; Tass and Hauptmann, 2009). By doing so, the network unlearns pathological connectivity and pathological synchrony. Put otherwise, it undergoes an anti-kindling (Tass and Majtanik, 2006). CR neuromodulation has initially been developed for the application to electrical deep brain stimulation (DBS) in Parkinson’s disease (Tass, 2003b). For this, brief electrical high-frequency pulse trains are sequentially delivered via different stimulation contacts of an implanted lead. In animal experiments acute desynchronizing effects (Neiman et al., 2007) as well as long-lasting desynchronizing after-effects (Tass et al., 2009) of electrical CR have been verified.
As shown computationally, due to the underlying biophysics and non-linear dynamics, CR neuromodulation can be applied invasively with electrical stimuli as well as non-invasively by means of sensory, e.g., auditory stimuli (Popovych and Tass, 2012; Tass and Popovych, 2012). Accordingly, in a proof of concept study the initially DBS-oriented CR concept was transformed into a non-invasive, acoustic treatment for subjective tonal tinnitus (Tass et al., 2012). Relying on the tonotopic organization of the central auditory system, the electrical brief high-frequency pulse trains were replaced by CR tones with different frequencies centered around the patient’s individual tinnitus frequency. The CR approach aims at a desynchronization of a synchronized focus in the tonotopically organized auditory cortex located in an area corresponding to the dominant tinnitus frequency and the belonging tinnitus spectrum (Norena et al., 2002). To this end, we sequentially delivered CR tones, where the goal of each CR tone is to cause a phase reset or at least a soft phase reset (i.e., a phase reset achieved by an iterated administration of that CR tone (see Tass, 2002) of the pathological slow-wave oscillation in the delta frequency band in a neuronal sub-population tonotopically related to the frequency of the particular CR tone (Tass et al., 2012).
In a prospective, randomized, single blind, placebo-controlled proof of concept trial in 63 patients with chronic tonal tinnitus (“RESET study”) CR treatment turned out to be safe and well-tolerated and resulted in a highly significant decrease of tinnitus loudness and symptoms as measured by VAS and tinnitus questionnaire (TQ) scores (Tass et al., 2012). Furthermore, as shown by means of EEG recordings, after 12 weeks of treatment with acoustic CR neuromodulation pathologically elevated delta and gamma activity were both decreased in a network of brain areas comprising primary and secondary auditory cortex as well as non-auditory, e.g., prefrontal areas (Tass et al., 2012). By the same token, the tinnitus-related reduction of alpha activity was reversed and alpha activity re-increased in auditory and prefrontal areas (Tass et al., 2012). In a relevant number of patients CR neuromodulation induced a tinnitus pitch change predominantly lowering tinnitus frequencies (Tass et al., 2012). Theoretical studies have predicted long-lasting (Tass and Majtanik, 2006) and cumulative (Hauptmann and Tass, 2009) desynchronizing effects of CR neuromodulation.
We here study if the tinnitus pitch change, observed in the RESET study, correlates with a reduction of tinnitus loudness and/or annoyance, and if the changes of the pattern of brain synchrony in tinnitus patients depends on whether or not the patients undergo a pronounced tinnitus pitch change. For this, we compare two groups of patients with very similar tinnitus relief, but significantly different tinnitus pitch change. According to previous studies different perceptual characteristics of tinnitus may be coded by an altered network activity of multiple parallel overlapping dynamic neural networks (De Ridder et al., 2011a). Also, it has been shown that different auditory modalities may be coded by different mechanisms and also by spatially separate neuronal networks: sound identification and sound localization were shown to depend on specialized and spatially distinct pathways (Clement et al., 1999; Alain et al., 2001; Banai et al., 2011). Clement et al. (1999) proposed that pitch and loudness are processed in separate modules of the auditory memory. Developmental trajectories of the abilities to detect auditory amplitude and frequency modulation were also shown to be distinct (Banai et al., 2011). Moreover, experiments in non-human primates indicate that an activation of primary sensory areas is not sufficient for the generation of the percept, and associative areas located in, e.g., frontal and parietal lobes are involved in sensory perception (Romo and Salinas, 2003; Lemus et al., 2009a, b). In summary, following current notions on parallel processing of different aspects of auditory information in general and in phantom perception in particular, we might expect differences in the change of brain synchrony in auditory, but also in non-auditory areas between our two patient groups, treated with acoustic CR neuromodulation and differing in the amount of their tinnitus pitch change.
The long-lasting and cumulative therapeutic (i.e., clinical) effects of acoustic CR neuromodulation along with its long-lasting desynchronizing effect on the pathological, tinnitus-related neuronal synchrony, both observed in our proof of concept study (Tass et al., 2012), are in accordance with these theoretical predictions. However, the CR-induced tinnitus pitch change was not predicted theoretically. In fact, the pitch change indicates CR-induced neuroplastic changes. We shall discuss possible mechanisms that may cause a CR-induced tinnitus pitch change below.
Materials and Methods
Participants
The current work is based on existing data from patients who participated in a multicentric randomized, controlled clinical trial on Acoustic CR Neuromodulation in the Treatment of Chronic Tinnitus, performed in Germany between 2009 and 2010 (“RESET study1,” Identifier: NCT00927121; Tass et al., 2012). For the present study patients were selected based on the following criteria: (i) EEG data had to be available for both baseline and the 12 weeks visit; (ii) clinical data, i.e., VAS loudness, VAS annoyance, and matched tinnitus pitch, had to be available for both base line and the 12 weeks visit. From the total population of 63 patients one patient dropped out of the study before the 12 weeks visit. For another patient there were no EEG data available, and in two patients matched tinnitus pitch could not be determined as tinnitus was absent at the 12 weeks visit. Thus 59 patients were selected for further analysis. All patients suffered from chronic subjective tonal tinnitus.
Treatment
Patients were stimulated for 12 weeks using a portable acoustic device and comfortable earphones (Tass et al., 2012). In the RESET study patients were randomly allocated to receive acoustic CR neuromodulation (group G1–G4) or placebo stimulation (G5): G1 (n = 22), G2 (n = 12), G3 (n = 12), G4 (n = 12), and G5 (n = 5). G1–G3 all received stimulation for 4–6 h every day. G4 and G5 all received stimulation for 1 h max every day. Stimulation signals were generated based on a specific formula reflecting the logarithmic tonotopic organization of the auditory cortex and on the matched tinnitus (frequency ft) with an equal number of tones placed below and above the tinnitus frequency (except for placebo). Stimulation tones were perceived by patients as equally loud and just super-threshold. Four tones per cycle were played in random order with three stimulation cycles followed by two silent cycles. The four tones are based on a patient specific list of frequencies (see Tass et al., 2012): G1, G3, G4 (f1 to f4); G2 (G2, prior to each cycle four frequencies are chosen from f1 to f12, with the constraint that each cycle has to contain one frequency from each of the four groups f1 to f3, f4 to f6, f7 to f9, and f10 to f12); G5 based on a modified tinnitus frequency [fp = 0.7071 ft/(2n), fp within 300–600 Hz]. The stimulation tones are equidistantly placed on a logarithmic scale within the interval [0.5·ft, 2·ft] for G1–G4 and within [0.5·fp, 2·fp] for G5. Cycle repetition rate was 1.5 Hz for G1, G2, G4, and G5 whereas in G3 the rate was harmonized to the patient’s specific EEG data (Tass, 2003b; Tass et al., 2009; based on the highest peak in the delta frequency band). A readjustment of stimulation parameters was performed at each visit, provided the matched tinnitus frequency had changed. Visits took place after 1, 4, 8, 12, and 16 weeks. Data for this paper come from baseline and the 12 weeks visit as EEG recordings for all patients were performed at these visits. At each visit, tinnitus loudness and annoyance were assessed off-stimulation (at least 2.5 h after cessation of CR neuromodulation) and consecutively on-stimulation (15 min after turning on CR neuromodulation) using a VAS scale for loudness (VAS-L) and annoyance (VAS-A) ranging from 0 to 100. In this study we only use the off-stimulation VAS scores, because EEG recordings were performed off-stimulation. A pure tone matching procedure was used to determine the best matching tinnitus pitch (from 100 to 10,000 Hz). Patients were instructed to match the frequency of a pure tone to the perceived pitch of their tinnitus. During this procedure intensity and frequency of the matching tone were controlled by the patient. Tinnitus matching started either well below or well above the patient’s tinnitus frequency. The patient had to adjust the matching tone to his/her tinnitus. Patients had to confirm a best matching pitch at least twice. The matching tone was repeatedly interrupted to facilitate the comparison between matching tone and tinnitus.
In general, the CR treatment was safe and well-tolerated and resulted in a highly significant decrease of tinnitus symptoms as measured by VAS and TQ scores (Tass et al., 2012): After 12 weeks of therapy a strong significant reduction (29.6–37.3 points) of VAS-L/VAS-A in G1 and G3 in the on-stimulation condition (p ≤ 0.01 compared to baseline) was observed. This significant effect persisted in the off-stimulation condition for G1/G3 VAS-L/VAS-A (18.0–28.8 points p < 0.004). G2 (the noisy CR group) and G4 (stimulation time of 1 h/day) showed less pronounced reduction of tinnitus symptoms (for more details see Tass et al., 2012). In contrast, the placebo group G5 showed neither on- nor off-stimulation significant changes in VAS-L/VAS-A scores after 12 weeks. TQ scores were significantly reduced compared to baseline in G1–G4 with the strongest improvements in G1 and G3 (Tass et al., 2012). In contrast, there were no significant changes in TQ score in the placebo group G5.
Data Collection
Each patient underwent two recording sessions: on day 1 before start of treatment and after 12 weeks, minimum 2 h after stopping the last stimulation session. Subjects were instructed to retain from caffeinated beverages on the day of the recording to exclude caffeine induced changes of EEG activity. Patients were seated in upright position in a comfortable chair. EEG recordings were obtained in a dimly lit room in a Faraday cage. EEG data were collected from 128 surface electrodes using a HydroCel Geodesic Sensor Net. All electrodes were referenced to Cz. The EEG signals were amplified with the Net Amps 200 amplifier (Electrical Geodesics Inc, Eugene, USA), digitized at 1 kHz and analogous bandpass filtered from 0.1 to 400 Hz. Recordings were performed in the awake state during alternating 2 min intervals with eyes closed and eyes open. For all patients we selected the eyes closed data for further analysis, since they were less affected by artifacts. Photogrammetry was performed for all subjects using Geodesic Photogrammetry System, and the individual head shape was modeled for every subject in each EEG session. Offline the scalp EEG was re-referenced to an average reference. Signals were additionally digitally filtered with a 0.8–130 Hz digital filter. Each EEG recording was corrected for blink and eye movements in BESA using the surrogate model approach in BESA (Brain Electrical Source Analysis, MEGIS Software, 5.3 version; Scherg et al., 2002). Recordings were further analyzed in MATLAB (The Mathworks, Natick, MA, USA) using EEGLAB2. All EEG segments that contained large muscle or other artifacts were removed. The mean length of the recordings after artifact correction was 3 min 24 s ± 23 s.
Data Analysis
Pearson correlation was performed between changes of VAS-L, VAS-A, matched tinnitus pitch (i.e., difference before and after CR treatment) and the corresponding modulus of the change of matched tinnitus pitch. We set out to investigate how spontaneous oscillatory brain activity changes in patients with pronounced tinnitus pitch change as opposed to patients with zero or minimal pitch change. Accordingly, to investigate differences in the changes of spontaneous oscillatory brain activity between patients with pronounced and with minimal/zero tinnitus pitch change, we created two groups of patients with a sustained relief of tinnitus symptoms as measured by VAS-L and VAS-A: (i) pitch change (PC) group, (ii) no tinnitus pitch change (NPC) or minimal tinnitus pitch change group. Sustained, clinically relevant relief of tinnitus symptoms, i.e., the minimal clinically important difference (MCID), was defined as ΔVAS ≤ -10 points (n = 34; Adamchic et al., submitted). This group of patients was divided into two equally large sub-populations based on the modulus of the individual pitch change ratio: Patients in the NPC group had a modulus of the pitch change ratio < 0.3, whereas patients in the PC group had pitch change ratio≥ 0.3. Pitch change ratio was defined as (modified pitch at 12 weeks visit/initial pitch at baseline) -1. Thus, 50% of patients were classified to the PC group and 50% to the NPC group. According to the significant positive correlation between VAS-L or VAS-A and the modulus of the change of the matched tinnitus pitch (see Results), the PC group had a greater VAS improvement (ΔVAS-L = -29.4 ± 10.8, n = 17) compared to the NPC group (ΔVAS-L = -25.1 ± 18.9, n = 17). To exclude the influence of different magnitudes of the reduction of tinnitus symptoms between the PC and NPC group on the further analysis, we excluded the patient with the greatest VAS-L improvement from PC and the patient with the smallest VAS-L improvement from NPC, in this way creating two groups (PC, n = 16 and NPC, n = 16) with a rather similar reduction of tinnitus symptoms, but significantly different matched pitch changes (Table 1).
None of the patients from the placebo group was classified to belong to PC. A current density analysis of the recorded electrical activity was performed in 3-D Talairach/MNI space using the sLORETA software package (Pascual-Marqui, 2002). sLORETA computed three-dimensional linear solutions for the EEG inverse problem with a three-shell spherical head model adapted to the Talairach human brain atlas digitized at the Brain Imaging Center of the Montreal Neurological Institute. sLORETA images represent the electrical activity of each voxel in terms of the amplitude of the computed current source density (CSD) in that voxel (μA/mm2). LORETA has been validated using functional magnetic resonance imaging (fMRI) and positron emission tomography (PET; Pascual-Marqui et al., 1994; Vitacco et al., 2002; Mulert et al., 2004; Pizzagalli et al., 2004; Zumsteg et al., 2005). Also, it was shown that even deep structures with subcallosal cingulate and mesial hippocampal foci could be correctly identified by LORETA (Pizzagalli et al., 2004; Zumsteg et al., 2005). sLORETA is an advanced, further improved version of LORETA (Pascual-Marqui et al., 2002). The solution space used in this study was restricted to the gray matter voxels that belonged to cortical and hippocampal regions, comprising a total of 6430 voxels at a spatial resolution of 5 mm. The effects of the differential change of the matched tinnitus frequency on the spontaneous brain activity as assessed by sLORETA in the PC and the NPC group were revealed between the two groups with a voxel-by-voxel paired-groups comparison of the current density distribution applying t-statistical non-parametric mapping (SnPM; Robertson and Irvine, 1989). For this, a paired-groups design (A1–A2) = (B1–B2) was used, where A1 and A2 stand for the CSDs in the PC group (before vs. after CR therapy, respectively) and B1 and B2 stand for the CSDs in the NPC group (before vs. after CR therapy, respectively). In this way, we detected brain regions where oscillatory activity was specifically reduced or increased after 12 weeks of CR treatment in the PC group as opposed to the corresponding changes in the NPC group. The significance levels used were p < 0.05. sLORETA source localization was performed on the basis of fixed frequency bands: delta (1–3 Hz), theta (4–7 Hz), alpha1 (8–9 Hz), alpha2 (10–12 Hz), beta1 (13–18 Hz), beta2 (19–21 Hz), beta3 (22–30 Hz), and gamma (30–45 Hz). Put otherwise, previously we have shown that CR therapy induces significant power changes in different frequency bands and specific brain areas (Tass et al., 2012). We here study, how these changes depend on whether or not the tinnitus pitch changes strongly.
To study whether the CSD changes, that showed up as significant differences between PC and NPC groups in particular Brodmann areas (BAs), were correlated with tinnitus pitch changes or VAS changes, CSDs were extracted from spherical volumes of interest (radius 5 mm) centered on the voxels with maximal significance value in each of these BAs. These CSD values were used for a partial correlation analysis with data from matched tinnitus pitch, VAS-L and VAS-A in all 59 patients. By using partial correlations we measure the relationship of two variables with the effect of a control variable being removed. In our case we correlated changes of CSD extracted from spherical volumes of interest for a certain frequency band with pitch changes, controlling for VAS and vice versa, thus obtaining correlations specifically for pitch and VAS. Additionally, we assess functional connectivity as a pattern of statistical dependencies between separate brain regions. For this, we do not make any specific assumptions concerning the directional interactions between the brain regions under study. Measures of linear dependence (coherence-type) between two multivariate time series may be expressed as the sum of the lagged linear and instantaneous linear dependences (Pascual-Marqui, 2007b). However, any measure of instantaneous dependence is likely to be contaminated with a non-physiological contribution due to volume conduction (Pascual-Marqui, 2007b). Pascual-Marqui (2007a) introduced a technique that removes the instantaneous, non-physiological contribution resulting from volume conduction. Accordingly, this measure of dependence can be applied to a large number of brain areas simultaneously. We calculated lagged linear connectivity (lagged coherence) for the same frequency bands as used for the sLORETA analysis. Calculations of lagged linear connectivity were performed using regions of interest (ROI), constructed for each hemisphere by defining all voxels located within a radius of 5 mm around designated seed points. Seed points were placed in the BAs that showed significant differences between PC and NPC and were defined as the voxel with the highest significance in a selected BA (see Results). In other words, we constrained our connectivity analysis to those brain areas which showed differential effects of CR therapy depending on whether or not tinnitus pitch changed strongly. Connectivity data were subjected to repeated-measures ANOVA (rmANOVA) with the within-group factor Time (1, base line; 2, after 12 weeks of acoustic CR neuromodulation) and between-group factor Group with two levels: PC and NPC. Where rmANOVA showed significant effects, post hoc t-tests were applied.
Results
Baseline characteristics of the patient population are presented in Table 2.
No significant correlation was observed between changes in VAS-L or VAS-A and tinnitus pitch change ratio when all patients (n = 59) were included into the analysis (Table 3).
However, in patients with decreased tinnitus pitch the pitch change ratio positively correlated with both a decrease in VAS-L (r = 0.37, p = 0.01; n = 46) and VAS-A (r = 0.48, p < 0.01; n = 46; Figure 1).
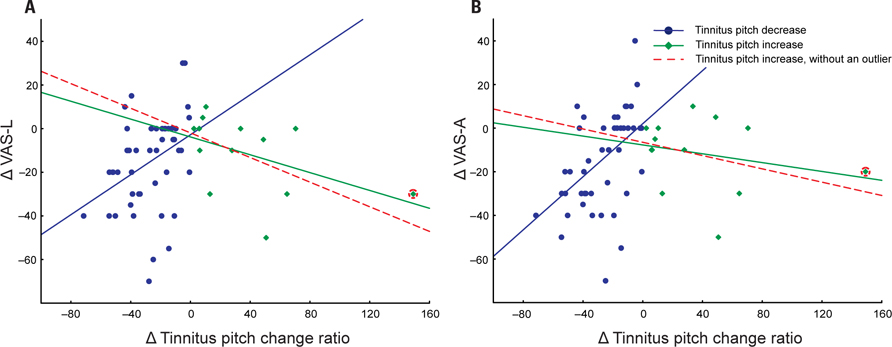
FIGURE 1. Changes in VAS-L (A) and VAS-A (B) as a function of a tinnitus pitch change ratio. Patients are divided into two groups: (1) tinnitus pitch decrease (blue, n = 46); (2) tinnitus pitch increase (green, n = 13 or red dashed line after removal of an outlier, n = 12). An outlier patient is marked by the red circle.
A complementary trend was observed in patients with an increase in their tinnitus pitch (Figure 1). However, possibly due to the low number of patients (n = 13), the correlation between the increase of the tinnitus pitch and the VAS-L and VAS-A changes did not reach significance: correlation between the increase of the tinnitus pitch change ratio and VAS changes were r = -0.37, p = 0.11 for VAS-L and r = -0.25, p = 0.42 for VAS-A. One patient (an “outlier”) had a much greater tinnitus pitch change ratio, i.e., = 1.49 than all other patients. However inclusion of this patient into the analysis set did not dramatically affect the results (Figure 1). Based on these findings, the modulus of the tinnitus pitch change was used for further analysis. A reduction of the tinnitus symptoms negatively correlated with the modulus of the tinnitus pitch change (Figure 2; Table 3). In general, we found a high correlation between the CR-induced changes in ΔVAS-L and ΔVAS-A, i.e., r = 0.92 (baseline to 12 weeks, p < 0.01).
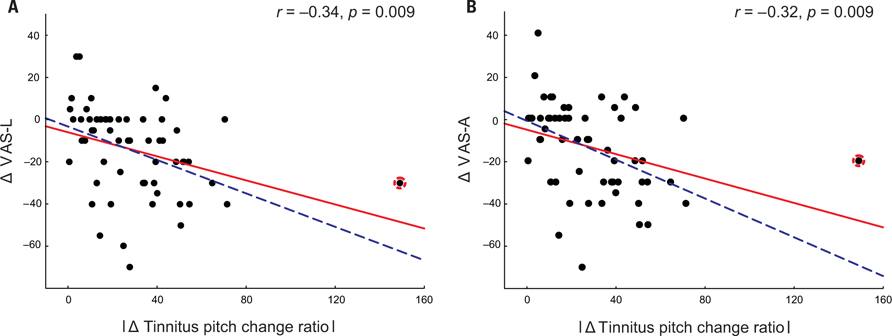
FIGURE 2. Changes in VAS-L (A) and VAS-A (B) as a function of the modulus tinnitus pitch change ratio with all patients included (n = 59, solid red line) or with the outlier (marked by the red circle) excluded from the analysis (n = 58, dashed blue line).
Standardized low resolution brain electromagnetic tomography revealed regions were oscillatory activity was specifically reduced or increased after 12 weeks of treatment in the PC group as compared to the changes in the NPC group. A significantly different effect on the power of the oscillatory activity after 12 weeks of CR therapy between the two groups was observed in the gamma band in PC compared to NPC in the following areas: left parietal cortex (BA 40), right frontal cortex (BA 9, 46), left frontal cortex (BA 4, 6), and left temporal cortex (BA 22, 42; Figure 3). In these brain areas gamma band power decreased significantly more strongly in the PC group.
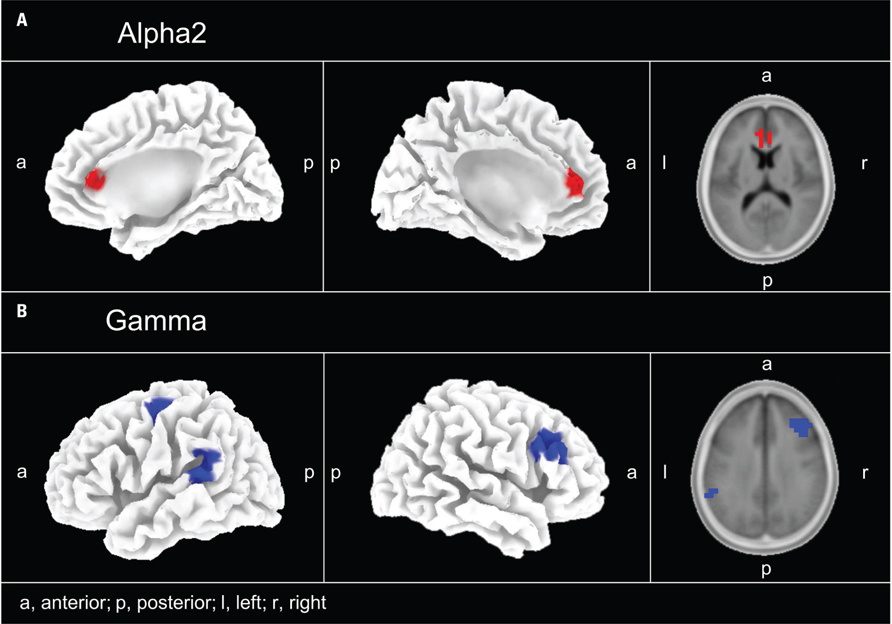
FIGURE 3. EEG source localization (sLORETA) power maps for the PC–NPC paired-groups comparison. (A) Alpha2 (10–12 Hz) increased (color-coded red) in the right and left anterior cingulate cortex (BA 24, 32). (B) Gamma band power decreased (color-coded blue) with pitch change in the left parietal (BA 40), left temporal (BA 22, 42), right frontal (BA 9, 46), and left frontal (BA 4, 6) cortex.
In addition, we found a differential effect of the CR therapy on the power of the oscillatory activity in the alpha2 band: The power of alpha2 increased significantly more strongly in the PC as compared to the NPC group in the right anterior cingulate cortex (ACC; BA 32, 24). No significant results were found for any other frequency bands. BAs containing significant voxels are listed in Table 4.
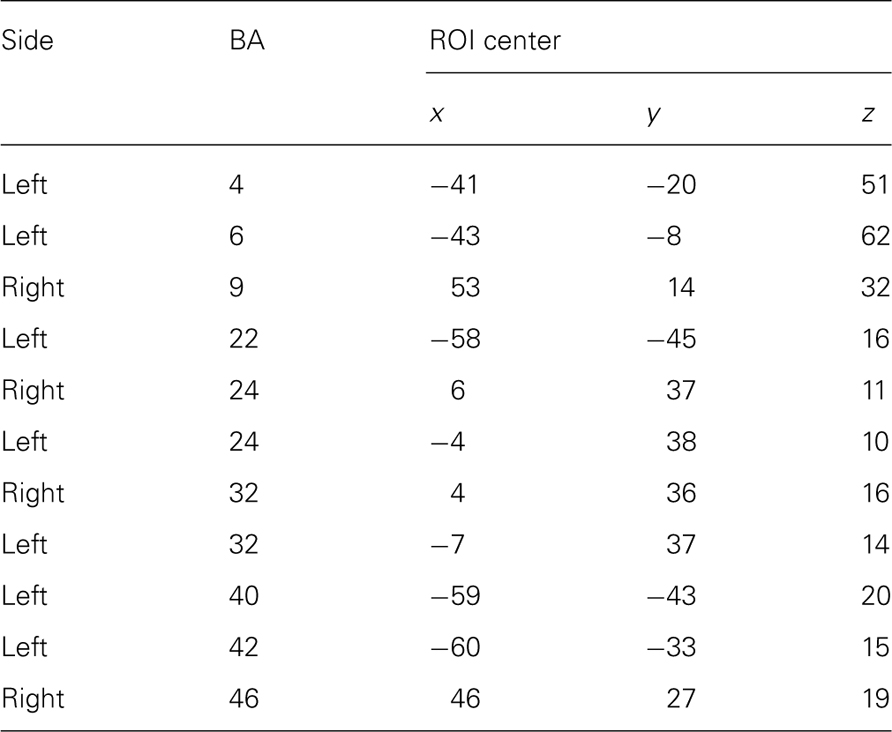
Table 4. Locations (Talairach coordinates) of maximal t-values in each BA containing significant voxels revealed by comparing oscillatory power changes between groups (PC and NPC) by means of sLORETA.
The modulus of the tinnitus pitch change ratio correlated negatively (controlling for VAS-L, i.e., statistically ruling out the influence of VAS-L) with the CSD changes in the gamma band in the left temporal cortex r = - 0.31, p = 0.02 (volumes of interest center x -58, y -45, z 16; BA 22). Changes in the VAS-A negatively correlated with changes in CSD (controlling for the modulus of the tinnitus pitch change ratio, i.e., statistically ruling out the influence of the modulus of the tinnitus pitch change ratio) in the alpha2 band in the right frontal cortex r = -0.33, p = 0.01 (volumes of interest center x 6, y 37, z 11; BA 24; Figure 4). The correlation between modulus of the pitch change ratio and CSD gamma power remained significant (r = -0.29, p = 0.03, n = 58) also after removing the outlier from the complete (n = 59) dataset.
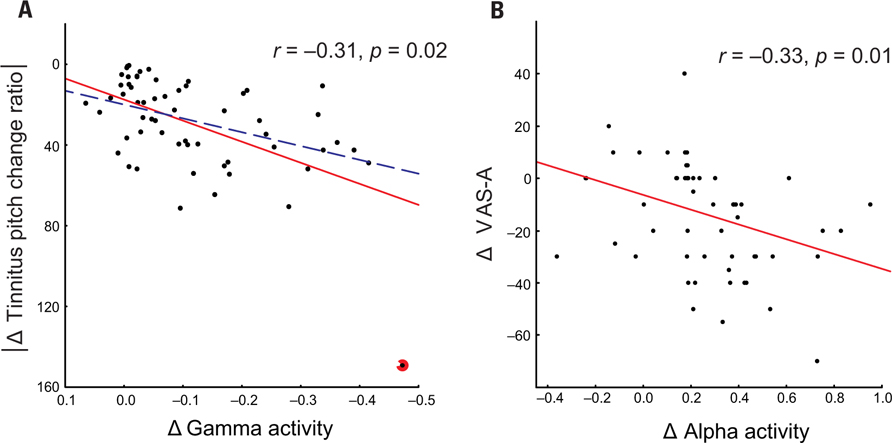
FIGURE 4. Significant negative partial correlation between relative change of CSD gamma power and modulus of tinnitus pitch change ratio (A) with all patients included (r = -0.31, p = 0.02, solid red line) or with the outlier excluded (r = -0.29, p = 0.03, dashed blue line).The outlier patient is marked by the red circle. A significant negative correlation (r = -0.33, p = 0.01, n = 59) between relative change of anterior cingulate CSD alpha power and difference in VAS-A (B).
No further significant correlations between clinical data and CSD were found for other regions and bands.
Repeated-measures ANOVA of functional connectivity values between BAs containing significant voxels revealed a significant interaction of Group (PC vs. NPC, F = 5.3, p = 0.03) for the right BA 32 – BA 46 connectivity. Subsequent post hoc t-tests showed a significantly lower linear connectivity at the end of 12 weeks than at the baseline (p = 0.007). Comparison between NPC and PC at the 12 weeks visit revealed significantly lower functional connectivity in the gamma band between the right dorsolateral prefrontal cortex (DLPFC; BA 46) and the right ACC (BA 32) in the PC group (p = 0.008; Figure 5). Accordingly no significant differences in functional connectivity were found between PC and NPC at baseline (p > 0.05).
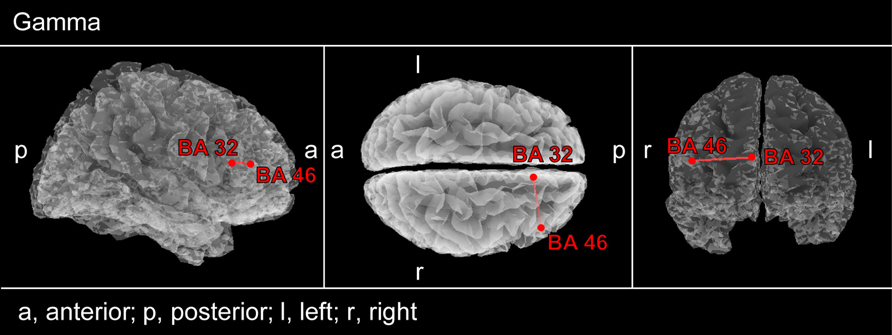
FIGURE 5. Connectivity contrast analysis between PC and NPC patients. rmANOVA analysis was applied to those brain areas which showed differential effects of CR therapy depending on whether or not tinnitus pitch changed strongly (see Figure 3). Decreased gamma lagged linear connectivity can be seen in PC patients between right ACC (BA 32) and DLPFC (BA 46).
Discussion
To our knowledge, this is the first study in a group of tinnitus patients that reveals an association of therapy-induced changes in tinnitus pitch to both a reduction of tinnitus symptoms and changes in oscillatory brain activity.
Notably, we observe a significant correlation between long-lasting CR therapy-induced changes of tinnitus pitch and changes of tinnitus loudness and annoyance. These findings are in agreement with a study conducted by Baguley et al. (2005) who showed that intravenous infusion of lidocaine in tinnitus patients leads to a short-term reduction of tinnitus loudness and annoyance paralleled by a tinnitus pitch reduction of roughly similar size. In that study, the measurement of pitch change, however, was performed with VAS and no analysis of an interdependence between changes in tinnitus loudness or annoyance and tinnitus pitch was performed. Another, substantial difference to our study is that changes of pitch as well as reduction in VAS-L and VAS-A disappeared 20 min after lidocaine injection, whereas VAS and matched tinnitus pitch measurements in our study were performed minimally 2.5 h after cessation of the acoustic CR neuromodulation.
In another study, investigating the treatment of tinnitus with chronic electrical neurostimulation of the vestibulocochlear nerve, the transformation of the tinnitus spectrum per se was perceived by patients as pleasant (Bartels et al., 2007). The significant correlation between changes in tinnitus loudness, tinnitus annoyance and changes in tinnitus pitch, obtained in our study, demonstrates the relation of the perceived CR therapy-induced tinnitus pitch change to the change of tinnitus symptoms.
Significant group differences in oscillatory brain activity are a reduction in gamma power over the left superior temporal gyrus, the left supramarginal gyrus and the left premotor cortex and the right DLPFC and an accompanying enhancement in alpha2 power in frontal lobe in patients with tinnitus pitch change. Human functional imaging studies indicate that supramarginal gyrus (left > right) is a part of a distributed and dynamic brain network that subserves pitch memory (Gaab et al., 2003). The area with reduced gamma activity in the left temporal lobe also roughly overlaps with the planum temporale (PT), a large region, located in the superior temporal plane posterior to Heschl’s gyrus (Westbury et al., 1999). PT was proposed to be a computational interface segregating incoming sound patterns that are then used for comparison with the previously stored patterns (Griffiths and Warren, 2002). The association of a CSD change in this area with changes in tinnitus pitch suggests that changes in the left superior temporal region might be more associated with changes in processing of tinnitus pitch per se. This region might not be the locus of conscious perception of pitch change, but instead a relay-station necessary for processing and gating pitch related information to higher-order cortical areas involved in the tinnitus aversive network.
Another region with lower gamma in the PC group roughly corresponds to the DLPFC. The right DLPFC was proposed to be a part of the tinnitus network related to the affective components of tinnitus (Schlee et al., 2009a, b; Vanneste et al., 2010; Langguth et al., 2011). It is also a part of a fronto-parietal network proposed to perform the retrieval and comparison of incoming auditory information (Arnott et al., 2005). Frontal regions were also shown to play a role in the maintenance of tonal patterns and auditory working memory, e.g., experimental evidence even suggests that the prefrontal cortex has cells related to auditory memory (Bodner et al., 1996; Chao and Knight, 1996; Celsis et al., 1999). Thus the prefrontal cortex could be one of the higher-order centers in the tinnitus aversive network that integrates sensory (e.g., tinnitus pitch) aspects of tinnitus possibly coming from PT with other modalities (Jastreboff, 1990; Mirz et al., 2000). An increase in alpha2 oscillatory brain activity in the PC group was found in a region corresponding to ACC. This is in line with data reported by Vanneste and De Ridder (2011) where the stimulation of DLPFC in tinnitus patients resulted in an increase of alpha activity in ACC. Moreover in our study CSD changes in the anterior cingulate area negatively correlated with changes in tinnitus annoyance. These results correspond to data obtained from patients suffering with pain where a correlation between changes in ACC activity and pain perception was observed (Davis et al., 1997).
Furthermore, we found a decreased connectivity in the gamma band between DLPFC and ACC regions in patients with tinnitus pitch change. The DLPFC has anatomical connections to the ACC permitting functional connectivity between these two areas (Pandya et al., 1981; Petrides and Pandya, 1999). In particular, gamma band oscillations appear to be crucial for the binding of information originating from different sources into coherent percept (Singer and Gray, 1995; Schulte et al., 2002; Herrmann et al., 2010). This finding is in line with previous data showing a modulation of activity in the ACC as a result of rTMS or transcranial direct current stimulation of the prefrontal cortex over the DLPFC (Paus et al., 2001; Vanneste and De Ridder, 2011). Remarkably decreased functional connectivity in the gamma band was also found between the right DLPFC and ACC after transcranial direct current stimulation of DLPFC (Vanneste and De Ridder, 2011). Premotor cortex was proposed to have hidden sensory function, however it’s relation to tinnitus is not yet clear (de Lafuente and Romo, 2002).
In summary, our study revealed changes in a distributed brain network which involved the left superior temporal and supramarginal gyrus, dorsolateral prefrontal region, and the ACC. CSD in the gamma band positively correlated with the modulus of the tinnitus pitch change in the region of the superior temporal gyrus (BA 22) indicating that this region may be related to processing and gating tinnitus pitch related information to higher-order cortical hubs of the tinnitus network. The ACC, in turn, could be more associated with affective tinnitus distress, being one of the higher-order centers in tinnitus network.
What mechanism may turn a greater tinnitus pitch change into a greater change of tinnitus annoyance and loudness? Different auditory modalities were proposed to be coded by different mechanisms and spatially separate brain networks (Clement et al., 1999; Alain et al., 2001; Banai et al., 2011). Thus, one can assume that different perceptual characteristics of tinnitus (e.g., pitch and loudness) could also be coded by spatially and functionally parallel and overlapping brain networks. This notion would be in line with the notion that tinnitus arises as the result of altered activity in multiple parallel overlapping dynamic brain networks (De Ridder et al., 2011b). Thus, tinnitus characteristic pitch associated with a negative emotional context related to tinnitus, can lead to the formation of a strong, aversive memory trace of this particular sound in a separate brain network specific for pitch processing and memory. This may at the same time be paralleled by increased synchronized neural activity, indicating its more intensive involvement, in the brain network subserving perceptual modality, i.e., pitch related to negative emotions. According to the binding theory (Singer and Gray, 1995; Schulte et al., 2002; Herrmann et al., 2010) different features of an object have to be combined into a coherent percept to be consciously perceived. Oscillatory neural activity in the gamma band have been proposed to be a representation of this binding process (Singer and Gray, 1995; Schulte et al., 2002; Herrmann et al., 2010). From the standpoint of auditory binding, the sLORETA results obtained in our study can be interpreted to reflect a substantial, CR-induced reduction of a tinnitus-related auditory binding process. Moreover persistent tinnitus would support this aversive emotional association and hinders its decline (De Ridder et al., 2011a). In this network superior temporal gyrus and/or PT could play the role of a tinnitus pitch processing and gating center and constitute a distinctive part of a pitch aversive network. After having been processed by this pitch hub, the information might enter a non-specific network comprising DLPFC for sensory information integration and ACC for associative and emotional processing. A change in tinnitus pitch might break the synchrony in one part of the tinnitus network of brain areas that otherwise supports aversive emotional associations and prevents from a decline of the memory of the tinnitus. In the absence of a reinforcement these aversive associations may gradually diminish and, finally, fade away. A destruction of such aversive emotional associations may, in turn, contribute to the reduction in chronic tinnitus distress.
As shown here, the amount of tinnitus pitch change determines in which way the amount of pathological (gamma) and physiological (alpha) synchrony in a network of tinnitus-related brain areas together with the interactions between different hubs within that network change. However, the question of the underlying physiological mechanism of the CR-induced tinnitus pitch change still remains open. Given the tonotopic organization of the primary auditory cortex, a CR-induced tinnitus pitch change may likely correspond to a spatial shift of the synchronized, tinnitus-related synchronous focus within the primary auditory cortex. Based on the dynamical mechanisms of action of CR neuromodulation (Tass, 2003a, b; Tass and Majtanik, 2006; Hauptmann et al., 2007; Tass and Hauptmann, 2009) asymmetries of both the stimulation and the network subjected to stimulation might cause such an effect. For instance, in an elegant modeling study on the emergence of the Zwicker tone (Zwicker, 1964; Franosch et al., 2003), an auditory after-effect, the impact of a spatial gradient of the lateral inhibition in the auditory cortex on the neuronal dynamics in the central auditory cortex has been explored. It was shown that a gradient of the lateral inhibition has significant impact on the neuronal dynamics and, in particular, that a noise reduction mechanism together with a dominantly unilateral inhibition is able to explain the emergence of the Zwicker tone. A gradient of the lateral inhibition combined with a spatially equidistant delivery of acoustic stimuli might explain a spatial shift of a synchronous focus in the primary auditory cortex. By the same token, in case of a spatially well-balanced lateral inhibition asymmetries of the arrangement of the CR tones (with respect to the patient’s individual tonotopic organization of the primary auditory cortex) might also cause a spatial shift of the synchronous focus in the primary auditory cortex. This issue will be in the focus of forthcoming theoretical and experimental studies.
Our results support the idea that tinnitus might be a consequence of altered activity in multiple parallel and overlapping dynamic networks. In this network elementary sensory dimensions may be represented by segregated mechanisms and networks that probably involve cortical areas encoding sensory features of the stimulus as well as prefrontal and cingulate regions necessary for perception.
Additional studies are required to further deepen our understanding of the involvement of pitch processing networks in the pathophysiology of tinnitus. Such studies will further elaborate which brain areas are critically involved in pitch processing in auditory phantom perception and how these areas interact with or depend on areas required for the percept to reach consciousness.
Conflict of Interest Statement
Dr. Peter A. Tass and Dr. Christian Hauptmann have a contractual relationship with ANM Adaptive Neuromodulation GmbH.
Footnotes
References
Alain, C., Arnott, S. R., Hevenor, S., Graham, S., and Grady, C. L. (2001). “What” and “where” in the human auditory system. Proc. Natl. Acad. Sci. U.S.A. 98, 12301–12306.
Arnott, S. R., Grady, C. L., Hevenor, S. J., Graham, S., and Alain, C. (2005). The functional organization of auditory working memory as revealed by fMRI. J. Cogn. Neurosci. 17, 819–831.
Baguley, D. M., Jones, S., Wilkins, I., Axon, P. R., and Moffat, D. A. (2005). The inhibitory effect of intravenous lidocaine infusion on tinnitus after translabyrinthine removal of vestibular schwannoma: a double-blind, placebo-controlled, crossover study. Otol. Neurotol. 26, 169–176.
Banai, K., Sabin, A. T., and Wright, B. A. (2011). Separable developmental trajectories for the abilities to detect auditory amplitude and frequency modulation. Hear. Res. 280, 219–227.
Bartels, H., Staal, M. J., Holm, A. F., Mooij, J. J., and Albers, F. W. (2007). Long-term evaluation of treatment of chronic, therapeutically refractory tinnitus by neurostimulation. Stereotact. Funct. Neurosurg. 85, 150–157.
Bodner, M., Kroger, J., and Fuster, J. M. (1996). Auditory memory cells in dorsolateral prefrontal cortex. Neuroreport 7, 1905–1908.
Celsis, P., Boulanouar, K., Doyon, B., Ranjeva, J. P., Berry, I., Nespoulous, J. L., and Chollet, F. (1999). Differential fMRI responses in the left posterior superior temporal gyrus and left supramarginal gyrus to habituation and change detection in syllables and tones. Neuroimage 9, 135–144.
Chao, L. L., and Knight, R. T. (1996). Prefrontal and posterior cortical activation during auditory working memory. Brain Res. Cogn. Brain Res. 4, 27–37.
Clement, S., Demany, L., and Semal, C. (1999). Memory for pitch versus memory for loudness. J. Acoust. Soc. Am. 106, 2805–2811.
Davis, K. D., Taylor, S. J., Crawley, A. P., Wood, M. L., and Mikulis, D. J. (1997). Functional MRI of pain- and attention-related activations in the human cingulate cortex. J. Neurophysiol. 77, 3370–3380.
de Lafuente, V., and Romo, R. (2002). A hidden sensory function for motor cortex. Neuron 36, 785–786.
De Ridder, D., Elgoyhen, A. B., Romo, R., and Langguth, B. (2011a). Phantom percepts: tinnitus and pain as persisting aversive memory networks. Proc. Natl. Acad. Sci. U.S.A. 108, 8075–8080.
De Ridder, D., Van Der Loo, E., Vanneste, S., Gais, S., Plazier, M., Kovacs, S., Sunaert, S., Menovsky, T., and Van De Heyning, P. (2011b). Theta-gamma dysrhythmia and auditory phantom perception. J. Neurosurg. 114, 912–921.
Dohrmann, K., Weisz, N., Schlee, W., Hartmann, T., and Elbert, T. (2007). Neurofeedback for treating tinnitus. Prog. Brain Res. 166, 473–554.
Eggermont, J. J., and Roberts, L. E. (2004). The neuroscience of tinnitus. Trends Neurosci. 27, 676–682.
Franosch, J. M., Kempter, R., Fastl, H., and Van Hemmen, J. L. (2003). Zwicker tone illusion and noise reduction in the auditory system. Phys. Rev. Lett. 90, 178103.
Gaab, N., Gaser, C., Zaehle, T., Jancke, L., and Schlaug, G. (2003). Functional anatomy of pitch memory – an fMRI study with sparse temporal sampling. Neuroimage 19, 1417– 1426.
Gerstner, W., Kempter, R., Van Hemmen, J. L., and Wagner, H. (1996). A neuronal learning rule for sub-millisecond temporal coding. Nature 383, 76–81.
Griffiths, T. D., and Warren, J. D. (2002). The planum temporale as a computational hub. Trends Neurosci. 25, 348–353.
Hauptmann, C., Popovych, O., and Tass, P. A. (2007). Desynchronizing the abnormally synchronized neural activity in the subthalamic nucleus: a modeling study. Expert Rev. Med. Devices 4, 633–650.
Hauptmann, C., and Tass, P. A. (2009). Cumulative and after-effects of short and weak coordinated reset stimulation: a modeling study. J. Neural Eng. 6, 016004.
Herrmann, C. S., Frund, I., and Lenz, D. (2010). Human gamma-band activity: a review on cognitive and behavioral correlates and network models. Neurosci. Biobehav. Rev. 34, 981–992.
Jastreboff, P. J. (1990). Phantom auditory perception (tinnitus): mechanisms of generation and perception. Neurosci. Res. 8, 221–254.
Kahlbrock, N., and Weisz, N. (2008). Transient reduction of tinnitus intensity is marked by concomitant reductions of delta band power. BMC Biol. 6, 4. doi: 10.1186/1741-7007-6-4
Langguth, B., Landgrebe, M., Kleinjung, T., Sand, G. P., and Hajak, G. (2011). Tinnitus and depression. World J. Biol. Psychiatry 12, 489–500.
Lemus, L., Hernandez, A., and Romo, R. (2009a). Neural codes for perceptual discrimination of acoustic flutter in the primate auditory cortex. Proc. Natl. Acad. Sci. U.S.A. 106, 9471–9476.
Lemus, L., Hernandez, A., and Romo, R. (2009b). Neural encoding of auditory discrimination in ventral premotor cortex. Proc. Natl. Acad. Sci. U.S.A. 106, 14640–14645.
Llinas, R. R., Ribary, U., Jeanmonod, D., Kronberg, E., and Mitra, P. P. (1999). Thalamocortical dysrhythmia: a neurological and neuropsychiatric syndrome characterized by magnetoencephalography. Proc. Natl. Acad. Sci. U.S.A. 96, 15222–15227.
Lockwood, A. H., Salvi, R. J., and Burkard, R. F. (2002). Current concepts: tinnitus. N. Eng. J. Med. 347, 904–910.
Markram, H. (1997). Regulation of synaptic efficacy by coincidence of postsynaptic APs and EPSPs. Science 275, 213–215.
Mirz, F., Gjedde, A., Ishizu, K., and Pedersen, C. B. (2000). Cortical networks subserving the perception of tinnitus – a PET study. Acta Otolaryngol. Suppl. 543, 241–243.
Muhlnickel, W., Elbert, T., Taub, E., and Flor, H. (1998). Reorganization of auditory cortex in tinnitus. Proc. Natl. Acad. Sci. U.S.A. 95, 10340–10343.
Mulert, C., Jager, L., Schmitt, R., Bussfeld, P., Pogarell, O., Moller, H. J., Juckel, G., and Hegerl, U. (2004). Integration of fMRI and simultaneous EEG: towards a comprehensive understanding of localization and time-course of brain activity in target detection. Neuroimage 22, 83–94.
Neiman, A. B., Russell, D. F., Yakusheva, T. A., Dilullo, A., and Tass, P. A. (2007). Response clustering in transient stochastic synchronization and desynchronization of coupled neuronal bursters. Phys. Rev. E Stat. Nonlin. Soft Matter Phys. 76, 021908.
Norena, A., Micheyl, C., Chery-Croze, S., and Collet, L. (2002). Psychoacoustic characterization of the tinnitus spectrum: implications for the underlying mechanisms of tinnitus. Audiol. Neurootol. 7, 358–369.
Norena, A. J., and Eggermont, J. J. (2003). Changes in spontaneous neural activity immediately after an acoustic trauma: implications for neural correlates of tinnitus. Hear. Res. 183, 137–153.
Ochi, K., and Eggermont, J. J. (1997). Effects of quinine on neural activity in cat primary auditory cortex. Hear. Res. 105, 105–118.
Pandya, D. N., Van Hoesen, G. W., and Mesulam, M. M. (1981). Efferent connections of the cingulate gyrus in the rhesus monkey. Exp. Brain Res. 42, 319–330.
Pascual-Marqui, R. D. (2002). Standardized low-resolution brain electromagnetic tomography (sLORETA): technical details. Methods Find. Exp. Clin. Pharmacol. 24(Suppl. D), 5–12.
Pascual-Marqui, R. D. (2007a). Discrete, 3D distributed, linear imaging methods of electric neuronal activity. Part 1: exact, zero error localization. arXiv:0710.3341 [math-ph], http://arxiv.org/pdf/0710.3341
Pascual-Marqui, R. D. (2007b). Instantaneous and lagged measurements of linear and nonlinear dependence between groups of multivariate time series: frequency decomposition. arXiv:0711.1455 [stat.ME], http://arxiv.org/abs/0711.1455
Pascual-Marqui, R. D., Esslen, M., Kochi, K., and Lehmann, D. (2002). Functional imaging with low-resolution brain electromagnetic tomography (LORETA): a review. Methods Find. Exp. Clin. Pharmacol. 24(Suppl. C), 91–95.
Pascual-Marqui, R. D., Michel, C. M., and Lehmann, D. (1994). Low resolution electromagnetic tomography:a new method for localizing electrical activity in the brain. Int. J. Psychophysiol. 18, 49–65.
Paus, T., Castro-Alamancos, M. A., and Petrides, M. (2001). Corticocortical connectivity of the human mid-dorsolateral frontal cortex and its modulation by repetitive transcranial magnetic stimulation. Eur. J. Neurosci. 14, 1405–1411.
Petrides, M., and Pandya, D. N. (1999). Dorsolateral prefrontal cortex: comparative cytoarchitectonic analysis in the human and the macaque brain and corticocortical connection patterns. Eur. J. Neurosci. 11, 1011–1036.
Pizzagalli, D. A., Oakes, T. R., Fox, A. S., Chung, M. K., Larson, C. L., Abercrombie, H. C., Schaefer, S. M., Benca, R. M., and Davidson, R. J. (2004). Functional but not structural subgenual prefrontal cortex abnormalities in melancholia. Mol. Psychiatry 9, 325, 393–405.
Popovych, O. V., and Tass, P. A. (2012). Desynchronizing electrical and sensory coordinated reset neuromodulation. Front. Hum. Neurosci. 6:58. doi: 10.3389/fnhum.2012.00058
Rauschecker, J. P. (2005). Neural encoding and retrieval of sound sequences. Ann. N. Y. Acad. Sci. 1060, 125–135.
Robertson, D., and Irvine, D. R. (1989). Plasticity of frequency organization in auditory cortex of guinea pigs with partial unilateral deafness. J. Comp. Neurol. 282, 456–471.
Romo, R., and Salinas, E. (2003). Flutter discrimination: neural codes, perception, memory and decision making. Nat. Rev. Neurosci. 4, 203–218.
Scherg, M., Ille, N., Bornfleth, H., and Berg, P. (2002). Advanced tools for digital EEG review: virtual source montages, whole-head mapping, correlation, and phase analysis. J. Clin. Neurophysiol. 19, 91–112.
Schlee, W., Hartmann, T., Langguth, B., and Weisz, N. (2009a). Abnormal resting-state cortical coupling in chronic tinnitus. BMC Neurosci. 10, 11. doi: 10.1186/1471-2202-10-11
Schlee, W., Mueller, N., Hartmann, T., Keil, J., Lorenz, I., and Weisz, N. (2009b). Mapping cortical hubs in tinnitus. BMC Biol. 7, 80. doi: 10.1186/1741-7007-7-80
Schulte, M., Knief, A., Seither-Preisler, A., and Pantev, C. (2002). Different modes of pitch perception and learning-induced neuronal plasticity of the human auditory cortex. Neural Plast. 9, 161–175.
Seki, S., and Eggermont, J. J. (2003). Changes in spontaneous firing rate and neural synchrony in cat primary auditory cortex after localized tone-induced hearing loss. Hear. Res. 180, 28–38.
Singer, W., and Gray, C. M. (1995). Visual feature integration and the temporal correlation hypothesis. Annu. Rev. Neurosci. 18, 555–586.
Steriade, M. (2006). Grouping of brain rhythms in corticothalamic systems. Neuroscience 137, 1087–1106.
Tass, P., Silchenko, A., Hauptmann, C., Barnikol, U., and Speckmann, E. J. (2009). Long-lasting desynchronization in rat hippocampal slice induced by coordinated reset stimulation. Phys. Rev. E Stat. Nonlin. Soft Matter Phys. 80, 011902.
Tass, P. A. (1999). Phase Resetting in Medicine and Biology: Stochastic Modelling and Data Analysis. Berlin: Springer Verlag.
Tass, P. A. (2002). Desynchronization of brain rhythms with soft phaseresetting techniques. Biol. Cybern. 87, 102–115.
Tass, P. A. (2003a). Desynchronization by means of a coordinated reset of neural sub-populations – a novel technique for demand-controlled deep brain stimulation. Prog. Theor. Phys. Suppl. 150, 281–296.
Tass, P. A. (2003b). A model of desynchronizing deep brain stimulation with a demand-controlled coordinated reset of neural subpopulations. Biol. Cybern. 89, 81–88.
Tass, P. A., Adamchic, I., Freund, H.-J., Von Stackelberg, T., and Hauptmann, C. (2012). Counteracting tinnitus by acoustic coordinated reset neuromodulation. Restor. Neurol. Neurosci. 30, 137–159.
Tass, P. A., and Hauptmann, C. (2009). Anti-kindling achieved by stimulation targeting slow synaptic dynamics. Restor. Neurol. Neurosci. 27, 589–609.
Tass, P. A., and Majtanik, M. (2006). Long-term anti-kindling effects of desynchronizing brain stimulation: a theoretical study. Biol. Cybern. 94, 58–66.
Tass, P. A., and Popovych, O. P. (2012). Unlearning tinnitus-related cerebral synchrony with acoustic coordinated reset stimulation – theoretical concept and modelling. Biol. Cybern. 106, 27–36.
Vanneste, S., and De Ridder, D. (2011). Bifrontal transcranial direct current stimulation modulates tinnitus intensity and tinnitus-distress-related brain activity. Eur. J. Neurosci. 34, 605–614.
Vanneste, S., Plazier, M., Der Loo, E., De Heyning, P. V., Congedo, M., and De Ridder, D. (2010). The neural correlates of tinnitus-related distress. Neuroimage 52, 470–480.
Vitacco, D., Brandeis, D., Pascual-Marqui, R., and Martin, E. (2002). Correspondence of event-related potential tomography and functional magnetic resonance imaging during language processing. Hum. Brain Mapp. 17, 4–12.
Weisz, N., Hartmann, T., Dohrmann, K., Schlee, W., and Norena, A. (2006). High-frequency tinnitus without hearing loss does not mean absence of deafferentation. Hear. Res. 222, 108–114.
Weisz, N., Moratti, S., Meinzer, M., Dohrmann, K., and Elbert, T. (2005). Tinnitus perception and distress is related to abnormal spontaneous brain activity as measured by magnetoencephalography. PLoS Med. 2, e153. doi: 10.1371/journal. pmed.0020153
Weisz, N., Muller, S., Schlee, W., Dohrmann, K., Hartmann, T., and Elbert, T. (2007). The neural code of auditory phantom perception. J. Neurosci. 27, 1479–1484.
Westbury, C. F., Zatorre, R. J., and Evans, A. C. (1999). Quantifying variability in the planum temporale: a probability map. Cereb. Cortex 9, 392–405.
Yang, S., Weiner, B. D., Zhang, L. S., Cho, S. J., and Bao, S. (2011). Homeostatic plasticity drives tinnitus perception in an animal model. Proc. Natl. Acad. Sci. U.S.A. 108, 14974–14979.
Yuste, R., and Bonhoeffer, T. (2004). Genesis of dendritic spines: insights from ultrastructural and imaging studies. Nat. Rev. Neurosci. 5, 24–34.
Zumsteg, D., Wennberg, R. A., Treyer, V., Buck, A., and Wieser, H. G. (2005). H2(15)O or 13NH3 PET and electromagnetic tomography (LORETA) during partial status epilepticus. Neurology 65, 1657–1660.
Keywords: tinnitus, coordinated reset neuromodulation, pitch, phantom perception, gamma band activity, electroencephalography
Citation: Adamchic I, Hauptmann C and Tass PA (2012) Changes of oscillatory activity in pitch processing network and related tinnitus relief induced by acoustic CR neuromodulation. Front. Syst. Neurosci. 6:18. doi: 10.3389/fnsys.2012.00018
Received: 23 December 2011; Accepted: 06 March 2012;
Published online: 05 April 2012.
Edited by:
Jos J. Eggermont, University of Calgary, CanadaReviewed by:
Jos J. Eggermont, University of Calgary, CanadaBerthold Langguth, University of Regensburg, Germany
Sven Vanneste, University Hospital Antwerp, Belgium
Copyright: © 2012 Adamchic, Hauptmann and Tass. This is an open-access article distributed under the terms of the Creative Commons Attribution Non Commercial License, which permits noncommercial use, distribution, and reproduction in other forums, provided the original authors and source are credited.
*Correspondence: Ilya Adamchic, Institute of Neuroscience and Medicine – Neuromodulation, Research Center Jülich, 52425 Jülich, Germany. e-mail:aS5hZGFtY2hpY0Bmei1qdWVsaWNoLmRl