- 1British Heart Foundation Centre for Cardiovascular Science, The Queen’s Medical Research Institute, University of Edinburgh, Edinburgh, UK
- 2Neonatal Unit, Simpson Centre for Reproductive Health, Royal Infirmary of Edinburgh, Edinburgh, UK
- 3Department of Translational Research in Psychiatry, Max-Planck Institute of Psychiatry, Munich, Germany
Background: Preterm birth associates with a substantially increased risk of later cardiovascular disease and neurodevelopmental disorders. Understanding underlying mechanisms will facilitate the development of screening and intervention strategies to reduce disease risk. Changes in DNA methylation have been proposed as one mechanism linking the early environment with later disease risk. We tested the hypothesis that preterm birth associates with altered DNA methylation in genes encoding insulin-like growth factor 2 (IGF2) and FK506-binding protein 5 (FKBP5), which appear particularly vulnerable to early life adversity.
Methods: Fifty preterm infants were seen and assessed at birth, term equivalent age, 3 months and 1-year corrected ages; 40 term infants were seen at birth, 3 months and 1 year. Saliva was collected for DNA extraction at birth, term, and 1 year. Pyrosequencing of bisulfite-converted DNA was performed to measure DNA methylation at specific CpG sites within the IGF2 and FKBP5 loci.
Results: Weight and head circumference was reduced in preterm infants at all time points. Preterm infants had a higher percentage body fat at term-corrected age, but this difference was not persistent. DNA methylation at the differentially methylated region (DMR) of IGF2 (IGF2DMR2) and FKBP5 was lower in preterm infants at birth- and term-corrected age compared to term infants at birth. IGF2DMR2 and FKBP5 methylation was related to birthweight SD score in preterm infants. Among preterm infants, social deprivation was an independent contributor toward reducing DNA methylation at IGF2DMR2 at birth- and term-corrected age and maternal smoking was associated with reduced DNA methylation at FKBP5 at birth. There were no persistent differences in DNA methylation at 1 year of age.
Conclusion: Changes in DNA methylation were identified at key regions of IGF2/H19 and FKBP5 in preterm infants in early life. Potential contributing factors include maternal smoking and social deprivation. However, these changes did not persist at 1 year of age and further longitudinal studies are required to determine any associations between altered DNA methylation in the perinatal period of individuals born preterm and their long-term health.
Introduction
Epidemiological evidence linking low birthweight with an increased risk of cardiovascular disease as well as developmental neuropsychiatric disorders (1) has led to the concept of “early life programming.” This proposes that exposure to adverse conditions during critical stages of early development results in a change in the offspring structural and functional phenotype (2). Preterm birth acts as a profound challenge in early life. There is now substantial evidence that prematurity associates with risk factors for cardiovascular disease in adulthood, including hypertension and insulin resistance (3–5). Furthermore, preterm birth is closely associated with neurodevelopmental disorders including cognitive impairment and autism spectrum disorder (6). This has important implications for public health, since worldwide, 15 million infants are born preterm every year, and survival rates have increased markedly over recent years (7).
Factors acting during intrauterine development, which may be important in mediating programing effects in infants born small at term, include undernutrition and glucocorticoid overexposure. These may be of particular importance in infants born preterm. In addition, preterm infants are vulnerable to these factors acting in early postnatal life (4). Following birth, many preterm infants develop a cumulative protein and energy deficit and exhibit early postnatal growth failure (8). Preterm infants are additionally exposed to repeated stressful and often painful procedures during a period of rapid brain maturation, and several studies have shown an impact of these procedures on neurodevelopment and hypothalamic–pituitary–adrenal (HPA) axis activity (9, 10).
Understanding the mechanisms by which prematurity associates with long-term effects on health would facilitate the development of effective screening and intervention strategies. Changes in DNA methylation have been proposed as one mechanism linking early life events and later disease risk (11), and genome-wide profiling has revealed DNA methylation differences between preterm and term infants in early life (12–14). Exposure to an adverse environment in early life has repeatedly been associated with altered DNA methylation at a gene of particular importance for fetal growth: the imprinted gene insulin-like growth factor 2 (IGF2). IGF2 is a key growth factor, particularly in early development. IGF2 expression is controlled by DNA methylation at a number of differentially methylated regions (DMRs) (15) and altered IGF2 DNA methylation has been reported following exposure to altered maternal nutrition including severe famine (16, 17).
The early life environment can also impact on the normal functioning of the HPA axis, with implications for neurodevelopment. Exposure to an adverse environment pre- or postnatally has been associated with altered DNA methylation at a number of genes important in determining HPA axis function, including the glucocorticoid receptor (GR) and 11beta-hydroxysteroid dehydrogenase type 2 (11β-HSD2). Differences in DNA methylation at GR and 11β-HSD2 in placenta have been reported in association with infant behavioral development (18); however, we have previously reported that DNA methylation at GR and 11β-HSD2 is extremely low in individuals exposed to an adverse early life environment and is, therefore, unlikely to impact on gene expression (17). FK506-binding protein 5 (FKBP5) encodes a co-chaperone of GR and is induced following stress exposure through GR binding to specific genomic response elements; in turn, FKBP5 protein binds to the GR complex, reducing its affinity for cortisol and decreasing nuclear translocation (19). Thus, it is an important component of the stress response. A functional polymorphism in FKBP5 intron 2 alters mRNA and protein induction following GR activation (20), such that the allele associated with stronger FKBP5 mRNA induction associates with GR resistance and an increased risk of a number of psychiatric disorders following childhood trauma (20). Exposure to childhood trauma leads to allele-specific epigenetic changes, with a GR-binding-induced decrease in DNA methylation within a functional glucocorticoid response element in intron 7, specifically, in carriers of the risk allele (20). Further, DNA methylation of FKBP5 in placenta associates with infant arousal scores (21).
In this study, we tested the hypothesis that preterm birth, a profound stressor in early life, associates with altered DNA methylation at the candidate loci IGF2 and FKBP5, which may be particularly vulnerable to early life adversity, and examined whether any changes were persistent over the first year of life.
Materials and Methods
Cohort
Fifty preterm (<32 weeks gestation) and 40 term (37–42 weeks gestation) infants were recruited within the first week of life from the Simpson Center for Reproductive Health, Edinburgh, UK, with informed written parental consent. Ethical approval was obtained from the South East Scotland Research Ethics Committee (Reference 11/AL/0329). NHS management approval was obtained (Lothian R&D Project number 2011/R/NE/03). Perinatal samples were collected under the Edinburgh Reproductive Tissue BioBank (ERTBB) (West of Scotland Research Ethics Service Reference 09/S0704/3). All parents gave written informed consent and all studies were performed in accordance with the declaration of Helsinki. Infant samples were collected under the framework of the ERTBB following an amendment to ethical approval (Reference AM07/1). Demographic details were obtained during clinic visits and from hospital records. All of the preterm infants were admitted to the neonatal unit; of these, six infants died. In three of these infants, saliva for buccal cells was not collected after birth due to clinical instability and DNA was of poor quality in a fourth infant. Five of the term infants were also admitted to the neonatal unit for a short period (respiratory distress syndrome, weight loss, and hemolysis from Rhesus isoimmunization) but none required follow-up. Preterm infants were seen at birth, at term-corrected age, and at 3 months and 1-year corrected ages; term infants were seen at birth, 3 months, and 1 year. All visits occurred in the afternoon, supervised by one researcher (Chinthika Piyasena).
Growth and Body Composition
Weight, length, and occipitofrontal head circumference (OFC) was measured by one trained researcher. Percentage body fat mass was measured by air displacement plethysmography in preterm infants at term-corrected age and 3 months corrected age, and in term infants at birth and 3 months using the PEAPOD Body Composition System (COSMED, Chicago, IL, USA). Skin fold thickness (subscapular and triceps) was measured at 1 year by the same trained researcher. Term infants were measured at a median of 2 days (range 0–8) after birth, at 3 months (13.3 weeks; range 10.4–16.9), and 1 year (52.3 weeks; range 48.1–57.4). Preterm infants were measured at term-corrected age at a median of 40 (range 35 + 0 to 44 + 1)-corrected weeks, at 3 months corrected (13.4 weeks; range 10.3–18.3) and 1 year corrected (53.9 weeks; range 52.1–68.7).
Analysis of DNA Methylation
Saliva was collected using the Oragene DNA (OG-250) kits and saliva sponges CS-1 and extracted using prepIT-2LP (DNA Genotek, Ottawa, ON, Canada). DNA was quantified using the Qubit 2.0 Fluorometer (Life Technologies, Paisley, UK). Five hundred nanograms of DNA were bisulfite converted using the EZ DNA Methylation Gold Kit (Zymo Research Corporation, CA, USA). Pyrosequencing was performed to analyze DNA methylation for DMRs controlling IGF2 expression: IGF2 DMR2 (IGF2DMR2, n = 9 CpGs) and the H19 imprinting control region (H19ICR, n = 8 CpGs) as previously described (22). Primers were purchased from Invitrogen (Life Technologies, Paisley, UK). DNA was amplified using the AmpliTaq Gold 360 kit (Applied Biosystems, Warrington, UK) and pyrosequencing performed using PyroMark Q24Gold reagents on a PyroMark Q24 Pyrosequencer (Qiagen, Crawley, UK). Data were analyzed using PyroMark Q24 1.0.10. Percentage DNA methylation is expressed as the average across all CpGs in each of the two loci in IGF2.
Methylation analysis of three CpGs in FKBP5 intron 7, two of which are located in consensus GRE motif (CpG 2 and 3), was performed in triplicates using a protocol adapted from Klengel et al. (20). One hundred twenty nanograms genomic DNA was bisulfite converted using the EZ DNA Methylation Kit (Zymo Research Corporation, CA, USA). Bisulfite converted DNA was amplified in a 50 µl reaction mix (4–10 µl DNA; each bisulfite specific Primer with a final concentration of 0.2 µM, FKBP5int7_P1_F: GTTGTTTTTGGAATTTAAGGTAATTG, and FKBP5int7_P1_R_biot: biotin-TCTCTTACCTCCAACACTACTACTAAAA) using the Kapa HIFI Uracil + Hot start Ready Mix (Kapa Biosystems Inc., Wilmington, DE, USA). Cycling conditions of the touchdown PCR were 98°C for 5 min, 2× (98°C – 40 s, 62°C – 30 s, 72°C – 60 s), 5× (98°C – 40 s, 60°C – 30 s, 72°C – 60 s), 8× (98°C – 40 s, 58°C – 30 s, 72°C – 60 s), 34× (98°C – 40 s, 56°C – 30 s, 72°C – 60 s), 72°C for 1 min and cooling to 4°C. Pyrosequencing of FKBP5 CpGs was performed on a PyroMark Q96 ID system (QIAGEN GmbH, Hilden) using PyroMark Q96Gold reagents with the following sequencing primer: FKBP5int7_P1_S2: 5′-GTTGATATATAGGAATAAAATAAGA-3′ for CpG1 and CpG2 and FKBP5int7_P1_S3: 5′-TGGAGTTATAGTGTAGGTTTT-3′ for CpG3. PyroMark Q96 ID Software 2.5 (QIAGEN GmbH, Hilden) was used to calculate percentage methylation.
The legends for Figures 1 and 2 state the number of values/measurements analyzed, excluding samples, which were discarded because of insufficient DNA and/or poor pyrosequencing quality. Additionally, we were unable to collect saliva from several babies for various reasons, although these babies still underwent anthropometry.
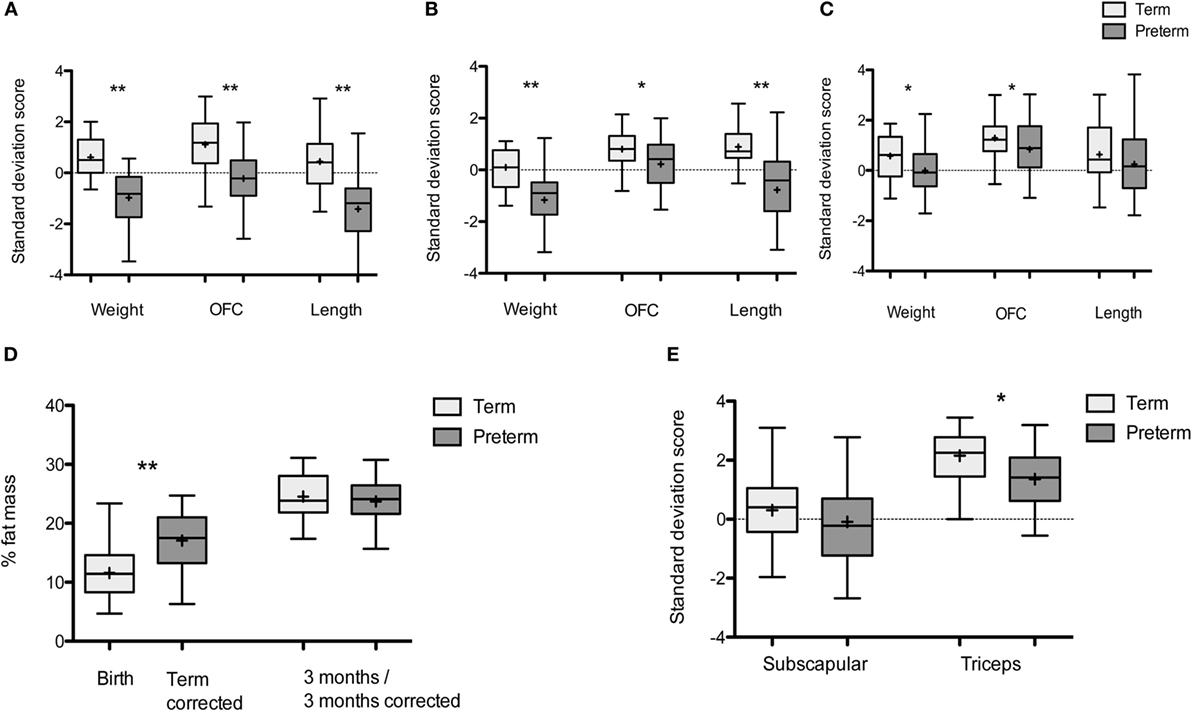
Figure 1. Growth of term and preterm infants over the first year: box and whisker plots: Tukey with line at median (+ indicates mean). (A) Weight, OFC, and length at birth- and term-corrected age. Term infants: n = 40, preterm infants: n = 43. (B) Weight, OFC, and length at 3 months/3 months-corrected age. Term infants n = 35, preterm infants n = 35. (C) Weight, OFC, and length at 1 year/1-year corrected. Term infants n = 35, preterm infants n = 42. (D) % fat mass in preterm and term infants at birth- and term-corrected age and 3 months of age. Term infants: n = 32 at birth and 30 at 3 months, preterm infants: n = 21 at term-corrected age and 32 at 3 months corrected. (E) Skinfold thickness in term and preterm infants at 1 year/1-year corrected. Term infants n = 32, preterm infants, n = 31 (n denotes the number of measurements obtained). *p < 0.05, **p < 0.001.
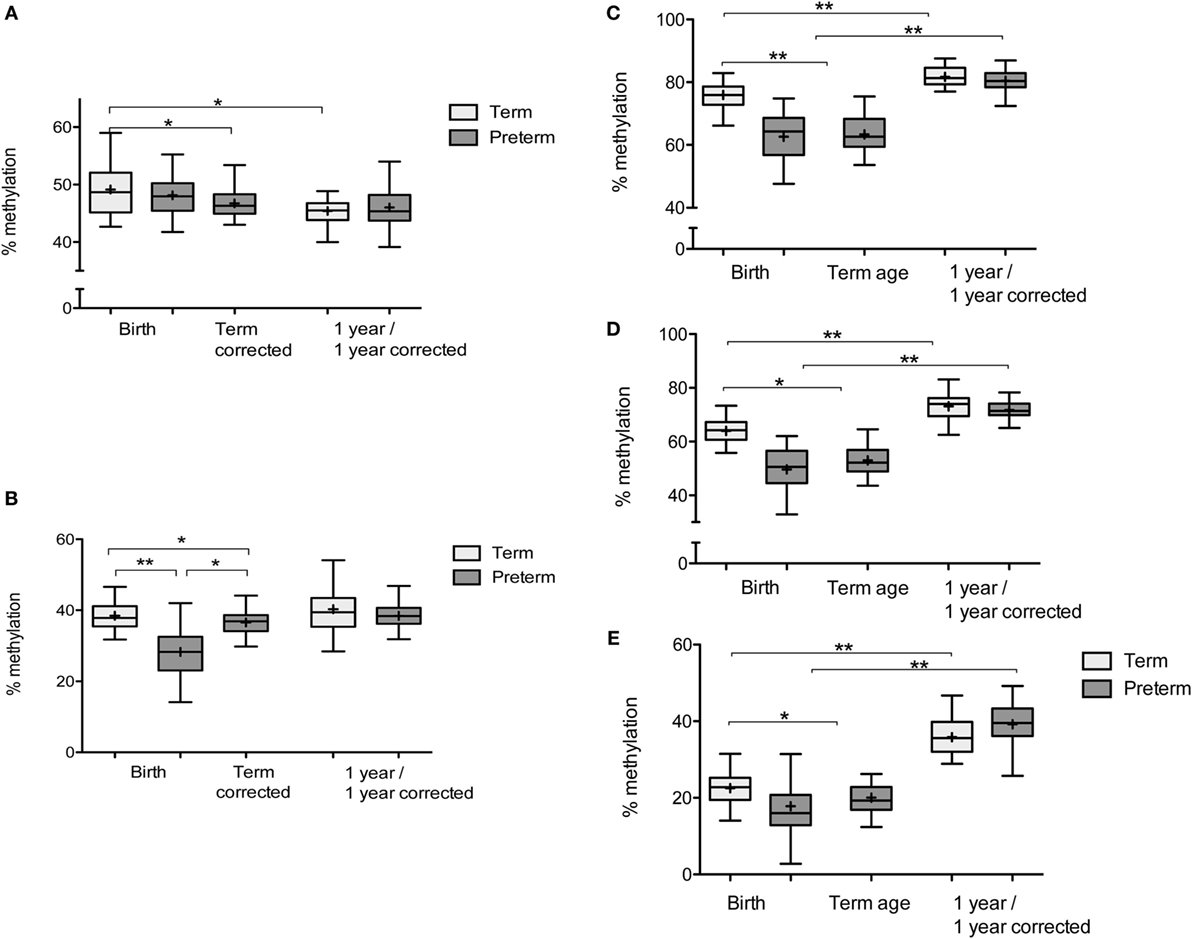
Figure 2. Percentage DNA methylation at IGF2/H19 and FKBP5: box and whisker plots: Tukey with line at median (+indicates mean). (A) % DNA methylation at H19ICR: term infants: n = 28 at birth and 31 at 1 year. Preterm infants: n = 23 at birth, 31 at term corrected, and 32 at 1-year corrected. (B) % DNA methylation at IGF2DMR2: term infants: n = 25 at birth and 23 at 1 year. Preterm infants: n = 22 at birth, 32 at term corrected, and 28 at 1-year corrected. (C) DNA methylation at FKBP5 CpG1: term infants: n = 32 at birth and 31 at 1 year. Preterm infants: n = 27 at birth, 37 at term-corrected age, and 32 at 1-year corrected. (D) DNA methylation at FKBP5 CpG2: term infants: n = 28 at birth and 30 at 1 year. Preterm infants: n = 25 at birth, 33 at term-corrected age, and 32 at 1-year corrected. (E) DNA methylation at FKBP5 CpG3: term infants: n = 30 at birth and 30 at 1 year. Preterm infants: n = 27 at birth, 36 at term-corrected age, and 36 at 1-year corrected (n denotes the number of measurements obtained). *p < 0.05, **p < 0.001.
FKBP5 SNP Genotyping
FKBP5 rs1360780 genotyping was performed on a Roche LightCycler 480 System using a TaqMan SNP Genotyping Assay (Applied Biosystems). Thermal cycling conditions were 95°C for 10 min, 45× (95°C – 15 s, 60°C – 1 min, 50°C – 30 s). Genotypes were called using endpoint genotyping LightCycler 480 Software version 1.5. Genotypes were in Hardy–Weinberg equilibrium (p = 0.17). Participants were divided into protective–genotype (CC) and risk-allele carriers (CT or TT).
Covariates
Covariates that could confound the association or be in the causal pathway were added into the model in a hierarchical manner: maternal smoking, male gender, and social deprivation for FKBP5 and IGF2/H19. Additionally, breast milk at 3 months for IGF2/H19 at 1 year and risk-allele carriage for FKBP5 at all time points. Social deprivation was coded as deprivation category (DEPCAT) scores based on the mother’s postcode at booking and obtained from the “Carstairs scores for Scottish postcode sectors from the 2001 Census.” In this system, scoring is based on the material affluence/deprivation of the area in which a person lives. Postcode sectors are allocated a DEPCAT score, derived from four sets of information: overcrowding, male unemployment, car ownership, and the proportion of people in households in social class 4 or 5 and scores range from 1 to 7 where 7 indicates the worst social deprivation. Maternal smoking was categorized as current smoker, never smoked, former (stopped pre-pregnancy), or former (stopped during pregnancy). Breast milk at 3 months indicates whether or not the infant was receiving any breast milk at 3 months corrected age.
Statistics
Weight, length, OFC, skin fold thickness, and weight gain were adjusted for age and gender by converting to SD scores (SDS) (z-scores) using LMSgrowth, a Microsoft Excel Add-in to access growth references that define the UK-WHO growth charts (23). Demographic, clinical characteristics, and risk-allele carriage between preterm and term infants were compared using independent samples t-testing and chi-square analyses, as appropriate. Multivariate linear regression was used to assess variation in body composition and to test the hypothesis that preterm birth is associated with altered DNA methylation. Outcome variables were percentage DNA methylation at birth, term-corrected age, and 1 year. Unstandardized regression (β) coefficients from these models indicate the change in percentage methylation associated with prematurity and a one-unit change in the other predictors. Paired samples t-testing was used where appropriate. One-way ANOVA with Dunnett’s post hoc t testing was used to test the effect of maternal smoking on DNA methylation at FKBP5. Statistical significance for all analyses was set at p < 0.05 (two-tailed).
Results
Preterm and Term Infant Demographics
Characteristics of the cohort are shown in Table 1. Birth weight SDS was lower in preterm infants p < 0.001, and there were more males in the preterm group, p = 0.006. There was no significant difference between the frequency of FKBP5 risk-allele carriage between the groups (p = 0.43). Maternal age, body mass index (BMI), DEPCAT scores, folic acid in first trimester, and maternal smoking were different between the two groups.
Growth and Body Composition
Weight and OFC was reduced in preterm infants at all time points compared to term infants (Figures 1A–C). Preterm infants were shorter than term infants at term-corrected age and 3 months corrected age, but not at 1-year corrected (Figures 1A–C). Preterm infants had a higher percentage body fat at term-corrected age when compared with term infants at birth (Figure 1D) (mean difference 5.5%, 95% CI [8.0, 3.0], p < 0.001) and this remained significant following adjustment for gender (β = 5.7, 95% CI [3.1, 8.3], p < 0.001). The difference in percentage body fat did not persist at 3 months/3 months corrected, including when adjusted for gender and breast milk intake (β = −0.3, 95% CI [−2.8, 2.2], p = 0.82) (Figure 1D). At 1-year corrected, preterm infants had lower triceps skin fold thickness SDS than term infants, adjusted for breast milk exposure at 3 months (β = −0.4, 95% CI [−1.3, −0.3], p = 0.008), but there were no differences in subscapular skin fold thickness (Figure 1E).
Prematurity and DNA Methylation
Mean DNA methylation at IGF2DMR2 was lower in preterm infants at birth compared to term infants at birth and this remained significant in adjusted analyses (β = −11.2, 95% CI [−15.2, −7.3], p < 0.001) (Figure 2B). There was no difference in mean DNA methylation at the H19ICR between preterm and term infants at birth after adjusted analysis (β = −1.3, 95% CI [−3.8, 1.2], p = 0.3) (Figure 2A). In preterm infants at term-corrected age, DNA methylation was reduced at IGF2DMR2 and H19ICR compared to infants born at term, and this remained significant in adjusted analyses (β = −2.8, 95% CI [−5.0, −0.6], p = 0.01 and β = −2.3, 95% CI [−4.6, −0.1], p = 0.049, respectively) (Figures 2A,B). However, the significance was attenuated when social deprivation was added into the model for IGF2DMR2 (β = −2.1, 95% CI [−4.3, 0.05], p = 0.055). At 1 year of age, there was no difference in mean DNA methylation in adjusted analyses at either IGF2DMR2 (β = −0.3, 95% CI [−4.0, 3.3], p = 0.86) or H19ICR (β = 0.8, 95% CI [−1.5, 3.0], p = 0.49) (Figures 2A,B).
The presence of the FKBP5 risk allele was associated with higher DNA methylation at FKBP5 CpG3 at birth (β = 4.0, 95% CI [0.3, 7.6], p = 0.03) and term-corrected age (β = 2.5, 95% CI [0.4, 4.5], p = 0.02) across all infants. DNA methylation at CpG1, CpG2, and CpG3 was lower in preterm infants at birth and at term-corrected age when compared to term infants at birth, including after adjusted analysis (Table 2). There was an increase in DNA methylation at all three CpGs in both groups during the first year (Figures 2C–E) and, by 1 year of age, there were no persistent differences in mean methylation at any CpG between preterm and term infants (Table 2). The presence/absence of the FKBP5 risk allele as a covariate did not alter the findings at 1 year and there was no moderation of the relationship between prematurity and DNA methylation by the presence of the risk allele at any time.
Body Composition and DNA Methylation
IGF2DMR2 methylation was highly significantly related to birthweight SDS in preterm (R = 0.7, p < 0.001), but not term infants. DNA methylation across FKBP5 was also positively associated with birthweight SDS (β = 0.3, 95% CI [0.3, 5.4], p = 0.03) when adjusted for prematurity, but only in the preterm infants when analyzed separately (R = 0.4, p = 0.04). There were no other significant relationships between DNA methylation at IGF2DMR2, H19ICR, or FKBP5 and weight or measures of body composition in either preterm or term infants at any other time point (Table 3).
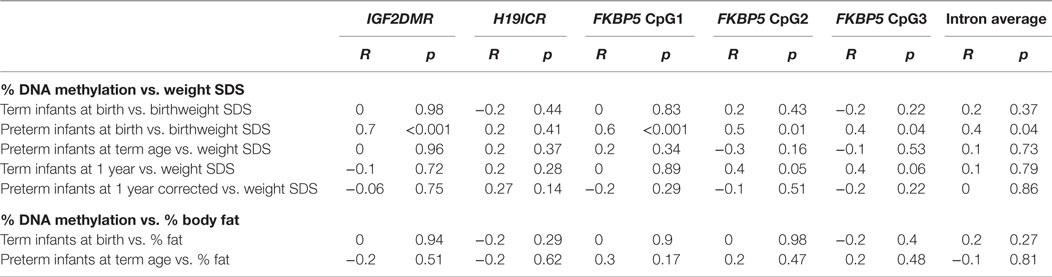
Table 3. Correlations between percentage DNA methylation at IGF2DMR2, H19ICR, and FKBP5 with weight SDS and percentage body fat.
Social Deprivation, Maternal Smoking, and DNA Methylation
Social deprivation was an independent contributor toward reducing DNA methylation at IGF2DMR2 in preterm infants at birth (β = −1.6, 95% CI [−2.8, −0.3], p = 0.02) and at term-corrected age (β = −0.9, 95% CI [−1.5, −0.2], p = 0.02) although this did not persist at 1-year corrected age (p = 0.07). Social deprivation was also independently associated with a reduction in DNA methylation at FKBP5 CpG2 in preterm infants at term-corrected age (β = −1.5, 95% CI [−2.9, −0.02], p = 0.047). Finally, maternal smoking was independently associated with a marked reduction in DNA methylation in preterm infants at FKBP5 CpG1 (β = −2.6, 95% CI [−4.4, −0.7], p = 0.008) and CpG2 (β = −2.1, 95% CI [−4.1, −0.03], p = 0.047) at birth, compared to term infants. Additionally, maternal smoking was associated with a reduction in DNA methylation at birth in both groups at these loci (Table 4).
Discussion
Preterm infants demonstrated a growth trajectory comparable to that reported in previous studies, such that they were lighter than term infants during the first year of life (24, 25). Although preterm infants had increased percentage fat mass at term-corrected age, there was no persisting difference at 3 months. Previous studies have reported increased and/or altered fat distribution in preterm infants at term-corrected age (26–28), with some reporting that these differences had resolved by 3 months (28). Despite this apparent resolution of excess adiposity in early life, preterm infants do show abnormalities of body composition in young adulthood, with higher adiposity, ectopic lipid deposition, and increased intra-abdominal fat (29).
DNA methylation at IGF2DMR2 and FKBP5 intron 7 was markedly lower in preterm infants at birth in comparison to term-born infants, and this was still the case at term-corrected age. Although we did not test the functional consequences of these alterations in DNA methylation, reduced IGF2DMR2 DNA methylation would be predicted to reduce IGF2 expression with potential implications for early growth. Reduced methylation of FKBP5 intron 7 CpGs is associated with higher induction of FKBP5 by GR activation, leading to increased GR resistance (20); however, whether this might play a role in the HPA axis dysregulation seen in preterm infants (9) is unclear. Social deprivation was an independent predictor of reduced methylation at IGF2DMR2 at birth and term-corrected age. Inter-related factors such as smoking that accompany socioeconomic deprivation can impact on DNA methylation in adults (30), including at FKBP5 (31). Recent studies in children and adults exposed to cigarette smoke in utero have reported alterations in global and site-specific DNA methylation (32–35), and our results showing that maternal smoking was independently associated with a marked reduction in DNA methylation at FKBP5 at birth suggest that this extends to effects on FKBP5.
There were no persistent differences in DNA methylation at 1 year of age at the DMRs of IGF2 and at FKBP5. Our data support an epigenome-wide association study (EWAS), which demonstrated that although there were many differences in DNA methylation between preterm and term babies at birth, these had largely resolved by 18 years of age. However, DNA methylation differences did persist at a subset of CpGs (12). This EWAS did not identify changes at IGF2 or FKBP5, which may reflect that it was small (n = 12 per group) and studied DNA methylation changes in blood spots, rather than buccal DNA. Our findings are also in agreement with longitudinal EWASs, which show that DNA methylation undergoes developmental changes during childhood (36). Notably, for FKBP5, DNA methylation increased between birth and 1 year in both term and preterm infants, and DNA methylation at CpG3 was still significantly lower at 1 year of age in comparison to levels described in adulthood (20), suggesting that there are ongoing changes in DNA methylation at this locus through childhood. Since studies clearly show that DNA methylation changes through infancy and childhood, it may be that the lower levels of DNA methylation in preterm infants at birth is a normal finding for infants at this gestation. For obvious reasons, we are unable to compare DNA methylation in saliva in infants of comparable gestation, who remained in utero. There were some persistent differences between term infants and preterm infants at term-corrected age, and we suggest that this may reflect differences between the intrauterine maturation in term-born infants and factors acting during the extra-uterine period to which preterm infants are exposed.
Alternative/additional explanations for the differences in DNA methylation between preterm and term infants in very early life could include the altered nutritional state of infants born preterm (37, 38); and/or glucocorticoid overexposure, since preterm infants experience both in utero exposure to synthetic glucocorticoids and significant early postnatal “stress.” In vivo and in vitro studies have shown that glucocorticoid exposure leads to stable DNA demethylation at these specific sites within the FKBP5 locus (20, 39), and several studies have now shown that exposure to trauma during childhood associates with allele-specific demethylation at FKBP5 in adulthood (20, 40). Although childhood abuse impacts on DNA methylation in carriers of the risk allele (20), we found no additional effects of the presence or absence of the risk allele. This is in agreement with a lack of effect of the risk allele on DNA methylation patterns following severe parental trauma (40), supporting the concept that these effects are specific to the timing of exposure (20), and it may be that the stresses around preterm birth occur outside this “vulnerable” period. Finally, it is also possible that differences in cell subtype populations in the saliva from preterm and term infants may impact on DNA methylation (41).
In summary, we found changes in DNA methylation at key regions of IGF2/H19 and FKBP5 in this cohort of preterm infants who did not achieve the reference for either growth or body composition by the time they reached 1 year of age. Further, we identified a number of contributing factors including maternal smoking and social deprivation. We found no persisting differences at 1 year of age. Whether further differences will emerge over longer follow-up is unknown; alterations in DNA methylation at key DMRs controlling the expression of IGF2 have been reported in adults exposed to altered nutrition or prematurity (16, 17, 42); however, these were not longitudinal studies, so that any differences may have arisen postnatally. Additionally, these studies may have been complicated by the phenomenon of reverse causation, where the development of disease leads to changes in DNA methylation rather than vice versa (41). Further longitudinal studies are required to understand any potential long-term effects of early differences in DNA methylation in the perinatal period on the health of individuals born preterm.
Ethics Statement
Infants were recruited within the first week of life from the Simpson Centre for Reproductive Health, Edinburgh, UK, with informed written parental consent. Ethical approval was obtained from the South East Scotland Research Ethics Committee (Reference 11/AL/0329). NHS management approval was obtained (Lothian R&D Project number 2011/R/NE/03). Perinatal samples were collected under the Edinburgh Reproductive Tissue BioBank (ERTBB) (West of Scotland Research Ethics Service Reference 09/S0704/3). All parents gave written informed consent and all studies were performed in accordance with the declaration of Helsinki. Infant samples were collected under the framework of the ERTBB following an amendment to ethical approval (Reference AM07/1).
Author Contributions
CP and AD conceived the study. CP, GM, JS, and AD designed the study. CP, JC, BK, RS, NP, TW, RR, EB, and AD performed the study and analyses. CP and AD wrote the initial manuscript draft and all authors revised it critically for intellectual content. All authors gave final approval of the version to be published.
Conflict of Interest Statement
The authors declare that the research was conducted in the absence of any commercial or financial relationships that could be construed as a potential conflict of interest.
Acknowledgments
Our thanks go to Karen French for technical assistance, to the staff of the Wellcome Trust Children’s Clinical Research Facility, Edinburgh for their assistance with clinical studies, and to Susann Sauer for excellent technical assistance with FKBP5 methylation analysis. Finally, our thanks go to the families who took part in this study.
Funding
Funding for the study was provided by a Scottish Senior Clinical Fellowship (SCD/09 to AD) and the MRC (G0501904 to AD). The purchase of the PEAPOD was supported by an equipment grant from Diabetes UK. FKBP5 methylation analysis was supported by a European Research Council starting grant (grant# 281338, GxE molmech) within the FP7 framework to EB. NP was supported by a fellowship from the Canadian Institute of Health Research. We also acknowledge the support of the British Heart Foundation and the Edinburgh Reproductive Tissue BioBank.
References
1. Risnes KR, Vatten LJ, Baker JL, Jameson K, Sovio U, Kajantie E, et al. Birthweight and mortality in adulthood: a systematic review and meta-analysis. Int J Epidemiol (2011) 40(3):647–61. doi:10.1093/ije/dyq267
2. Gluckman PD, Hanson MA. Living with the past: evolution, development, and patterns of disease. Science (2004) 305(5691):1733–6. doi:10.1126/science.1095292
3. Parkinson JRC, Hyde MJ, Gale C, Santhakumaran S, Modi N. Preterm birth and the metabolic syndrome in adult life: a systematic review and meta-analysis. Pediatrics (2013) 131(4):e1240–63. doi:10.1542/peds.2012-2177
4. Bayman E, Drake AJ, Piyasena C. Prematurity and programming of cardiovascular disease risk: a future challenge for public health? Arch Dis Child Fetal Neonatal Ed (2014) 99(6):F510–4. doi:10.1136/archdischild-2014-306742
5. Tinnion R, Gillone J, Cheetham T, Embleton N. Preterm birth and subsequent insulin sensitivity: a systematic review. Arch Dis Child (2014) 99(4):362–8. doi:10.1136/archdischild-2013-304615
6. Johnson S, Hollis C, Kochhar P, Hennessy E, Wolke D, Marlow N. Psychiatric disorders in extremely preterm children: longitudinal finding at age 11 years in the EPICure study. J Am Acad Child Adolesc Psychiatry (2010) 49(5):453–63.e.1. doi:10.1016/j.jaac.2010.02.002
7. Blencowe H, Cousens S, Chou D, Oestergaard M, Say L, Moller A-B, et al. Born too soon: the global epidemiology of 15 million preterm births. Reprod Health (2013) 10(Suppl 1):S2. doi:10.1186/1742-4755-10-S1-S2
8. Embleton NE, Pang N, Cooke RJ. Postnatal malnutrition and growth retardation: an inevitable consequence of current recommendations in preterm infants? Pediatrics (2001) 107(2):270–3. doi:10.1542/peds.107.2.270
9. Grunau RE, Haley DW, Whitfield MF, Weinberg J, Yu W, Thiessen P. Altered basal cortisol levels at 3, 6, 8 and 18 months in infants born at extremely low gestational age. J Pediatr (2007) 150(2):151–6. doi:10.1016/j.jpeds.2006.10.053
10. Brummelte S, Grunau RE, Zaidman-Zait A, Weinberg J, Nordstokke D, Cepeda IL. Cortisol levels in relation to maternal interaction and child internalizing behavior in preterm and full-term children at 18 months corrected age. Dev Psychobiol (2011) 53:184–95. doi:10.1002/dev.20511
11. Godfrey KM, Lillycrop KA, Burdge GC, Gluckman PD, Hanson MA. Epigenetic mechanisms and the mismatch concept of the developmental origins of health and disease. Pediatr Res (2007) 61(5 Pt 2):5R–10R. doi:10.1203/pdr.0b013e318045bedb
12. Cruickshank M, Oshlack A, Theda C, Davis P, Martino D, Sheehan P, et al. Analysis of epigenetic changes in survivors of preterm birth reveals the effect of gestational age and evidence for a long term legacy. Genome Med (2013) 5(10):96. doi:10.1186/gm500
13. Parets SE, Conneely KN, Kilaru V, Fortunato SJ, Syed TA, Saade G, et al. Fetal DNA methylation associates with early spontaneous preterm birth and gestational age. PLoS One (2013) 8(6):e67489. doi:10.1371/journal.pone.0067489
14. Sparrow S, Manning JR, Cartier J, Anblagan D, Bastin ME, Piyasena C, et al. Epigenomic profiling of preterm infants reveals DNA methylation differences at sites associated with neural function. Transl Psychiatry (2016) 6:e716. doi:10.1038/tp.2015.210
15. Ollikainen M, Smith KR, Joo EJ-H, Ng HK, Andronikos R, Novakovic B, et al. DNA methylation analysis of multiple tissues from newborn twins reveals both genetic and intrauterine components to variation in the human neonatal epigenome. Hum Mol Genet (2010) 19(21):4176–88. doi:10.1093/hmg/ddq336
16. Heijmans BT, Tobi EW, Stein AD, Putter H, Blauw GJ, Susser ES, et al. Persistent epigenetic differences associated with prenatal exposure to famine in humans. Proc Natl Acad Sci U S A (2008) 105(44):17046–9. doi:10.1073/pnas.0806560105
17. Drake AJ, McPherson RC, Godfrey KM, Cooper C, Lillycrop KA, Hanson MA, et al. An unbalanced maternal diet in pregnancy associates with offspring epigenetic changes in genes controlling glucocorticoid action and foetal growth. Clin Endocrinol (2012) 77(6):808–15. doi:10.1111/j.1365-2265.2012.04453.x
18. Paquette AG, Lester BM, Lesseur C, Armstrong DA, Guerin DJ, Appleton AA, et al. Placental epigenetic patterning of glucocorticoid response genes is associated with infant neurodevelopment. Epigenomics (2015) 7(5):767–79. doi:10.2217/epi.15.28
19. Zannas AS, Binder EB. Gene-environment interactions at the FKBP5 locus: sensitive periods, mechanisms and pleiotropism. Genes Brain Behav (2014) 13(1):25–37. doi:10.1111/gbb.12104
20. Klengel T, Mehta D, Anacker C, Rex-Haffner M, Pruessner JC, Pariante CM, et al. Allele-specific FKBP5 DNA demethylation mediates gene-childhood trauma interactions. Nat Neurosci (2013) 16(1):33–41. doi:10.1038/nn.3275
21. Paquette AG, Lester BM, Koestler DC, Lesseur C, Armstrong DA, Marsit CJ. Placental FKBP5 genetic and epigenetic variation is associated with infant neurobehavioral outcomes in the RICHS cohort. PLoS One (2014) 9(8):e104913. doi:10.1371/journal.pone.0104913
22. Dupont JM, Tost J, Jammes H, Gut IG. De novo quantitative bisulphite sequencing using the pyrosequencing technology. Anal Biochem (2004) 333(1):119–27. doi:10.1016/j.ab.2004.05.007
23. Pan H, Cole TJ. LMSgrowth, a Microsoft Excel Add-In to Access Growth References Based on the LMS Method. Version 2.77. (2012). Available from: http://www.healthforallchildren.co.uk
24. Hack M, Schluchter M, Cartar L, Rahman M, Cuttler L, Borawski E. Growth of very low birth weight infants to age 20 years. Pediatrics (2003) 112(1):e30–8. doi:10.1542/peds.112.1.e30
25. Cole TJ, Statnikov Y, Santhakumaran S, Pan H, Modi N; Neonatal Data Analysis Unit and the Preterm Growth Investigator Group, et al. Birth weight and longitudinal growth in infants born below 32 weeks’ gestation: a UK population study. Arch Dis Child Fetal Neonatal Ed (2014) 99(1):F34–40. doi:10.1136/archdischild-2012-303536
26. Uthaya S, Thomas EL, Hamilton G, Dore CJ, Bell J, Modi N. Altered adiposity after extremely preterm birth. Pediatr Res (2005) 57:211–5. doi:10.1203/01.PDR.0000148284.58934.1C
27. Roggero P, Gianni ML, Amato O, Orsi A, Piemontese P, Morlacchi L, et al. Is term newborn body composition being achieved postnatally in preterm infants? Early Hum Dev (2009) 85(6):349–52. doi:10.1016/j.earlhumdev.2008.12.011
28. Ramel SE, Gray HL, Ode KL, Younge N, Georgieff MK, Demerath EW. Body composition changes in preterm infants following hospital discharge: comparison with term infants. J Pediatr Gastroenterol Nutr (2011) 53(3):333–8. doi:10.1097/MPG.0b013e3182243aa7
29. Thomas EL, Parkinson JR, Hyde MJ, Yap IKS, Holmes E, Dore CJ, et al. Aberrant adiposity and ectopic lipid deposition characterize the adult phenotype of the preterm infant. Pediatr Res (2011) 70(5):507–12. doi:10.1038/pr.2011.732
30. Elliott HR, Tillin T, McArdle WL, Ho K, Duggirala A, Frayling TM, et al. Differences in smoking associated DNA methylation patterns in South Asians and Europeans. Clin Epigenetics (2014) 6(1):4. doi:10.1186/1868-7083-6-4
31. Dogan MV, Lei MK, Beach SR, Brody GH, Philibert RA. Alcohol and tobacco consumption alter hypothalamic pituitary adrenal axis DNA methylation. Psychoneuroendocrinology (2016) 66:176–84. doi:10.1016/j.psyneuen.2016.01.018
32. Flom JD, Ferris JS, Liao Y, Tehranifar P, Richards CB, Cho YH, et al. Prenatal smoke exposure and genomic DNA methylation in a multiethnic birth cohort. Cancer Epidemiol Biomarkers Prev (2011) 20(12):2518–23. doi:10.1158/1055-9965.epi-11-0553
33. Murphy SK, Adigun A, Huang Z, Overcash F, Wang F, Jirtle RL, et al. Gender-specific methylation differences in relation to prenatal exposure to cigarette smoke. Gene (2012) 494(1):36–43. doi:10.1016/j.gene.2011.11.062
34. Novakovic B, Ryan J, Pereira N, Boughton B, Craig JM, Saffery R. Postnatal stability, tissue, and time specific effects of AHRR methylation change in response to maternal smoking in pregnancy. Epigenetics (2014) 9(3):377–86. doi:10.4161/epi.27248
35. Joubert BR, Felix JF, Yousefi P, Bakulski KM, Just AC, Breton C, et al. DNA methylation in newborns and maternal smoking in pregnancy: genome-wide consortium meta-analysis. Am J Hum Genet (2016) 98(4):680–96. doi:10.1016/j.ajhg.2016.02.019
36. Simpkin AJ, Hemani G, Suderman M, Gaunt TR, Lyttleton O, McArdle WL, et al. Prenatal and early life influences on epigenetic age in children: a study of mother-offspring pairs from two cohort studies. Hum Mol Genet (2016) 25(1):191–201. doi:10.1093/hmg/ddv456
37. Waterland RA, Lin J-R, Smith CA, Jirtle RL. Post-weaning diet affects genomic imprinting at the insulin-like growth factor 2 (Igf2) locus. Hum Mol Genet (2006) 15(5):705–16. doi:10.1093/hmg/ddi484
38. Rijlaarsdam J, Cecil CA, Walton E, Mesirow MS, Relton CL, Gaunt TR, et al. Prenatal unhealthy diet, insulin-like growth factor 2 gene (IGF2) methylation, and attention deficit hyperactivity disorder symptoms in youth with early-onset conduct problems. J Child Psychol Psychiatry (2016). doi:10.1111/jcpp.12589
39. Lee RS, Tamashiro KLK, Yang X, Purcell RH, Harvey A, Willour VL, et al. Chronic corticosterone exposure increases expression and decreases deoxyribonucleic acid methylation of Fkbp5 in mice. Endocrinology (2010) 151(9):4332–43. doi:10.1210/en.2010-0225
40. Yehuda R, Daskalakis NP, Bierer LM, Bader HN, Klengel T, Holsboer F, et al. Holocaust exposure induced intergenerational effects on FKBP5 methylation. Biol Psychiatry (2015) 80(5):372–80. doi:10.1016/j.biopsych.2015.08.005
41. Birney E, Smith GD, Greally JM. Epigenome-wide association studies and the interpretation of disease -omics. PLoS Genet (2016) 12(6):e1006105. doi:10.1371/journal.pgen.1006105
Keywords: prematurity, DNA methylation, IGF2, FKBP5, glucocorticoids
Citation: Piyasena C, Cartier J, Provençal N, Wiechmann T, Khulan B, Sunderesan R, Menon G, Seckl JR, Reynolds RM, Binder EB and Drake AJ (2016) Dynamic Changes in DNA Methylation Occur during the First Year of Life in Preterm Infants. Front. Endocrinol. 7:158. doi: 10.3389/fendo.2016.00158
Received: 17 October 2016; Accepted: 29 November 2016;
Published: 15 December 2016
Edited by:
Susan Ozanne, University of Cambridge, UKReviewed by:
Kazuhiro Takahashi, Tohoku University, JapanDaniel Vaiman, French Institute of Health and Medical Research, France
Copyright: © 2016 Piyasena, Cartier, Provençal, Wiechmann, Khulan, Sunderesan, Menon, Seckl, Reynolds, Binder and Drake. This is an open-access article distributed under the terms of the Creative Commons Attribution License (CC BY). The use, distribution or reproduction in other forums is permitted, provided the original author(s) or licensor are credited and that the original publication in this journal is cited, in accordance with accepted academic practice. No use, distribution or reproduction is permitted which does not comply with these terms.
*Correspondence: Amanda J. Drake, bWFuZHkuZHJha2VAZWQuYWMudWs=