- 1AG Integrative Sensory Physiology, Institute for Animal Physiology, Justus-Liebig-Universität Gießen, Gießen, Germany
- 2Institute for General and Applied Zoology, Justus-Liebig-Universität Gießen, Gießen, Germany
The defense glands in the dorsal prothorax are an important autapomorphic trait of stick insects (Phasmatodea). Here, we study the functional anatomy and neuronal innervation of the defense glands in Anisomorpha paromalus (Westwood, 1859) (Pseudophasmatinae), a species which sprays its defense secretions when disturbed or attacked. We use a neuroanatomical approach to identify the nerves innervating the gland muscles and the motoneurons with axons in the different nerves. The defense gland is innervated by nerves originating from two segments, the subesophageal ganglion (SOG), and the prothoracic ganglion. Axonal tracing confirms the gland innervation via the anterior subesophageal nerve, and two intersegmental nerves, the posterior subesophageal nerve, and the anterior prothoracic nerve. Axonal tracing of individual nerves reveals eight identified neuron types in the subesophageal or prothoracic ganglion. The strongest innervating nerve of the gland is the anterior subesophageal nerve, which also supplies dorsal longitudinal thorax muscles (neck muscles) by separate nerve branches. Tracing of individual nerve branches reveals different sets of motoneurons innervating the defense gland (one ipsilateral and one contralateral subesophageal neuron) or the neck muscle (ventral median neurons). The ipsilateral and contralateral subesophageal neurons have no homologs in related taxa like locusts and crickets, and thus evolved within stick insects with the differentiation of the defense glands. The overall innervation pattern suggests that the longitudinal gland muscles derived from dorsal longitudinal neck muscles. In sum, the innervating nerves for dorsal longitudinal muscles are conserved in stick insects, while the neuronal control system was specialized with conserved motoneurons for the persisting neck muscles, and evolutionarily novel subesophageal and prothoracic motoneurons innervating the defense gland.
Introduction
In stick insects (Phasmatodea), paired exocrine defense glands are found in the prothorax (Bedford, 1978; Chow, 2008; Bradler, 2009). The gland opening is located at the anterio-dorsal pronotum. The defense glands are an autapomorphic character for the Phasmatodea (Grimaldi and Engel, 2005; Bradler, 2009). Even in the Indian walking stick Carausius morosus which apparently does not produce defensive secretions (Bässler, 1983; Carlberg, 1985a), the glands are found in the prothorax albeit in relatively small size (Marquardt, 1940; Stolz et al., 2015). In species displaying chemical defense, the paired defense glands produce the defense fluid from a secretory epithelium lining the inner gland muscle layers (Happ et al., 1966; Strong, 1975; Eisner et al., 1997). The defense secretions have been studied for the biochemical components in detail in different species of stick insects (e.g., Meinwald et al., 1962; Smith et al., 1979; Chow and Lin, 1986; Ho and Chow, 1993; Bouchard et al., 1997; Eisner et al., 1997; Dossey et al., 2006, 2008; Schmeda-Hirschmann, 2006; summarized in Dettner, 2015). In Anisomorpha species, the principle component of the defense spray is anisomorphal, a monoterpene dialdehyde (Meinwald et al., 1962) which occurs in three diastereomers in Anisomorpha buprestoides (Dossey et al., 2006).
In addition, the importance of chemical defense secretion for the survival of stick insects has been shown in behavioral assays as an effective mechanism against predators for several species (Eisner, 1965; Carlberg, 1981, 1985b,c, 1986a, 1987; Bouchard et al., 1997; Dossey et al., 2012; but see Nentwig, 1990). The defense glands can be employed in defense against predators like birds or rodents by secreting or spraying a repellent or irritating fluid, or by releasing an odor (Eisner, 1965; Bedford, 1978; Carlberg, 1986a; Eisner et al., 2005). Secretion release usually covers parts of the body surface with the fluid, while spraying release occurs over a distance at potential predators (Carlberg, 1986b; Eisner et al., 1997, 2005; van de Kamp et al., 2015).
Aimed spraying is arguably the most complex among these chemical defense behaviors. Behavioral experiments have shown that A. buprestoides can direct the spray from either both glands, or only a single gland, depending on the direction of contact (Eisner, 1965). Further, they can spray in an aimed direction at birds prior to any contact with the insect body (Eisner, 1965). In general, different sensory stimuli are integrated for fluid ejection including vision and touch. The chemical defense must thus be under a neuronal control which goes beyond local, reflex-like circuits in the prothorax. A previous comparative study has revealed a complex innervation pattern of the glands by three peripheral nerves from the subesophageal and prothoracic ganglion in four species including C. morosus (Stolz et al., 2015). The most prominent nerve supplying the defense glands, the Nervus anterior, originates in the subesophageal ganglion (SOG), and also supplies adjacent thoracic longitudinal muscles (Stolz et al., 2015). In addition, the intersegmental nerve complex innervates the glands by the posterior subesophageal nerve and the anterior prothoracic nerve in most species studied (Stolz et al., 2015). Between these distantly related groups (Goldberg et al., 2015), the peripheral nerve pattern is conserved. Notably, in Peruphasma schultei only the anterior subesophageal nerve innervates the defense glands, but not the intersegmental nerve complex (Stolz et al., 2015). Few neuron types located in the SOG and the prothoracic ganglion have axons in the Nervus anterior SOG (Stolz et al., 2015).
Here, we study the neuronal innervation of the defense glands in further detail in the species Anisomorpha paromalus, which is closely related to A. buprestoides (Bradler, 2009). The aim of this study is (1) to document the innervation pattern of the defense gland for a species from a genus which has a strong spraying defense behavior from prominent defense glands (Eisner, 1965), (2) to identify the particular neurons which innervate the defense gland muscles in nerves which also target adjacent muscles (Stolz et al., 2015), and (3) to compare the innervation in A. paromalus to the closely related P. schultei (both Pseudophasmatinae; Goldberg et al., 2015) for the functional specialization on the N. ant. SOG innervation. We use axonal tracing of individual nerve branches from the Nervus anterior SOG to differentiate the neuronal innervation of the defense gland and of the dorsal longitudinal muscles (or neck muscles, Honegger et al., 1984). Comparison of these motoneurons in stick insects to homologous neurons in related species of cockroaches (Davis, 1983) and locusts (Altman and Kien, 1979; Honegger et al., 1984) reveals neurons which have evolved with the development of the defense glands. This comparison of identified neurons (Goodman et al., 1979; Hoyle, 1983; Arbas et al., 1991; Kutsch and Breidbach, 1994) allows to identify the evolutionary novel nerve cells in stick insects which underlie chemical defense.
Materials and Methods
Animals
Anisomorpha paromalus (Westwood, 1859) (Pseudophasmatinae) were reared in a crowded lab culture of females and males at the Institute for Animal Physiology, Justus-Liebig-University, Gießen. Animals were kept under a 12:12 light-dark regime. They were fed with Ligustrum leaves ad libitum. Only adult individuals were used in this study.
Scanning Electron Microscopy
The gland opening was documented by scanning electron microscopy from specimens stored in 70% ethanol (Carl Roth, Karlsruhe, Germany). Thoraces were isolated from the rest of the body and cut in the middle with scissors. They were dehydrated in a graded ethanol series (Carl Roth, Karlsruhe, Germany). The preparations were critical point-dried (BAL-TEC, Balzers, Liechtenstein) and sputter-coated with gold (SCD 004 SputterCoater, Balzers), and viewed and documented with a Phillips XL 20 SEM.
Dissection Procedures
Prior to preparation of the glands and the nervous system, insects were cold-anesthetized for 10 min at 4°C. The dissection procedures followed those established for other species (Stolz et al., 2015), cutting the insects open with scissors on the dorsal side of the thorax and head capsule, and flattening them out in glass dishes which were filled with Sylgard (Sylgard 184, Suter Kunststoffe AG, Fraunbrunnen, Switzerland) with insect pins. The esophagus and gut were removed with scissors, and the ganglia and peripheral nerves were thus exposed for neuroanatomical investigations from the dorsal side. The preparations were covered with Carausius saline with pH = 7.4 (0.00684 mol/l NaCl; 0.01744 mol/l KCl; 0.00748 mol/l CaCl2 × 2 H2O; 0.01844 mol/l MgCl2 × 6 H2O; 0.00198 mol/l Tris; Weidler and Diecke, 1969; Bässler, 1977). This dissection procedure was also followed in preparations for axonal tracing (see below).
Neuroanatomy and Axonal Tracing
Gland Innervation Pattern
To document the peripheral nerves innervating the defense gland, these structures were stained in situ. The preparations were shortly incubated for 20–40 s with Janus Green B solution (Sigma-Aldrich, St. Louis, Missouri; used 0.02% in Carausius saline). Janus Green B stains the fine peripheral nerves and other tissues. The Janus Green B solution was used at storage temperature of 4°C, and quickly washed out after incubation using Carausius saline.
Axonal Tracing Experiments
To reveal the gland innervation by specific nerves and the somata located in the central nervous system of neurons with axons in these nerves, axonal tracing was used. Again, the tracing procedures were carried out in situ following the dissection described above (for details see Stolz et al., 2015). Neurobiotin was used as tracing solution (5%, dissolved in Aqua dest.; Vector Laboratories, Burlingame, CA, USA). The different nerves taking up the tracing solution were cut with iridectomy scissors (see schematics in Figures 7, 8). Preparations were incubated at 4°C for 4 days in a moist chamber. Ganglia were then taken out and fixed in 4% paraformaldehyde (Sigma Chemicals, St. Louis, Missouri, USA) dissolved in phosphate buffer (0.04 mol/l Na2HPO4; 0.00574 mol/l NaH2PO4 × 2 H2O) for 1 h. They were subsequently rinsed in phosphate buffer and stored in PBST buffer (0.1369 mol/l NaCl, 0.0027 mol/l KCl, 0.01 mol/l Na2HPO4, 0.00176 mol/l KH2PO4, all substances from Merck, Darmstadt, Germany; and 0.1% Triton X-100, Roth, Karlsruhe, Germany; with pH = 7.2). The uptake of neurobiotin in neurites and somata was visualized as described previously (Stolz et al., 2015) using the Avidin-Biotin kit (Vectastain ABC Kit PK-6100; Vector Laboratories, Burlington CA) and DAB solution (Vectastain DAB kit SK-4100; Vector Laboratories) according to manufacturers' instructions.
Histology of the Defense Glands
After axonal tracing, the defensive glands were dehydrated in a graded ethanol series (30% ethanol to 96% ethanol) followed by two changes of 99.9% 2-propanol, each step for several hours at room temperature. The tissues were then embedded in Histosec® (Merck, Darmstadt, Germany) and 10 μm sections were made with a Leitz rotary microtome and mounted on glass slides (SuperFrost™, Carl Roth, Karlsruhe, Germany). Sections were deparaffined with Roti®-Histol (Carl Roth, Karlsruhe, Germany) and rehydrated in a descending ethanol series.
For histological staining to analyze the gland muscles, the sections were also incubated in methanol for 5 min at room temperature and then stained in double concentrated Giemsa solution (Stock solution from Carl Roth, Karlsruhe, Germany) for 1 h. Excess staining solution was washed off with demineralized water, and the slides were next differentiated with 1% (v/v) acetic acid for 3 s and washed with demineralized water. In addition, gland sections were also analyzed without counter-staining. These sections were mounted directly after deparaffination (see below).
Microscopy and Documentation
In Situ Preparations of Peripheral Nerves with Vital Staining
The stained preparations covered with Carausius saline were viewed with a Leica dissection microscope and drawn with help of a Leica drawing mirror. The drawings were scanned and digitally redrawn using CorelDraw version 11 (Corel, Ottawa, Canada).
Preparations with Axonal Tracing
Glands were viewed in phosphate buffer with a Leica dissection microscope and documented by photographs from a Nikon Digital Sight DS-5M camera (1,280 × 960 pixel) attached to the microscope. Ganglia with filled nerves were dehydrated in a graded ethanol series (Carl Roth, Karlsruhe, Germany), cleared in methyl salicylate (Merck, Darmstadt, Germany). They were viewed with an Olympus BH-2 microscope, and documented by photographs from a Leica DFC 7000 T digital camera (1,920 × 1,440 pixel) connected to the microscope, using the Leica Application Suite V4.9.
Histological Sections
Histological sections were dehydrated in 100% xylol (Carl Roth) and mounted in Entellan® (Merck, Darmstadt, Germany). Photomicrographs were taken with a CV12 camera (Olympus K.K., Shinjuku, Japan) mounted on a BX50 microscope (Olympus K.K., Shinjuku, Japan). Series of single pictures were stitched together with the program AutoStitch v2.2 (Brown and Lowe, 2007).
Terminology
The terminology of peripheral nerves follows Marquardt (1940) and Honegger et al. (1984). The terminology for thoracic muscles follows Friedrich and Beutel (2008) and Wipfler et al. (2015) for the neopteran muscle groundplan in the thorax. For comparison to Orthoptera, we refer to the muscle descriptions and muscle terminology by Honegger et al. (1984).
Morphometric Analysis and Statistics
Ganglion areas and neuron soma areas were measured from digitized photographs of ganglia using the freeware program ImageJ (https://imagej.nih.gov/ij/). Since animals show a sexual dimorphism in size, we compared the data between sexes for SOG area, absolute soma size for two identified subesophageal neurons (ipsilateral neuron ILN and contralateral neuron CLN, see results), and the relative soma size for ILN and CLN. The relative soma size was calculated by dividing the absolute soma size by the ganglion area of the respective ganglion. Statistical analysis used the software GraphPad Prism 4 (GraphPad, San Diego, CA). Data were tested for normal distribution using the Kolmogorov-Smirnov (KS) normality test. Since not all data were normally distributed, we further used the Mann-Whitney test to compare data from female and male individuals.
Results
Defense Glands and Innervation Pattern
Anisomorpha paromalus shows a notable dimorphism in size between sexes with females being much larger than males (Figure 1a). In both sexes, the gland opening is located below a cuticular ridge at the anterio-dorsal edge of the pronotum (Figure 1b). This external opening of the ejaculatory duct was very small and surrounded by membranes (Figure 1c).
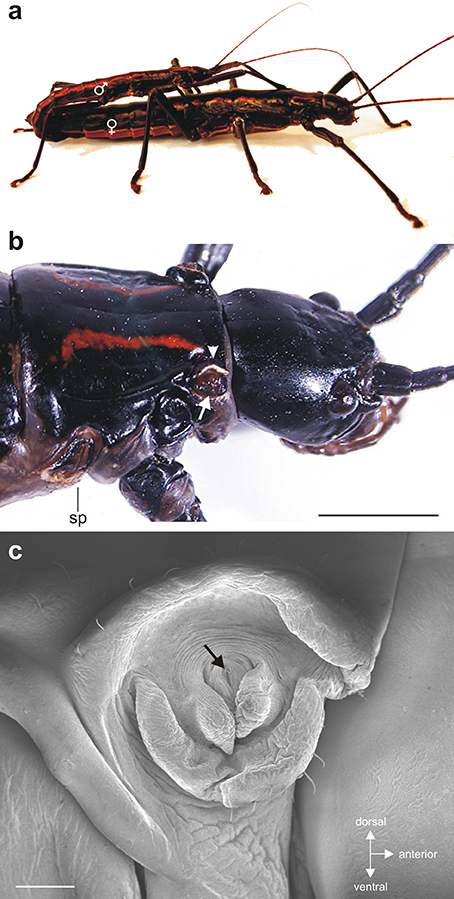
Figure 1. (a) Couple of Anisomorpha paromalus with male on top and female below. (b) The gland opening (white arrow) is located below a cuticular ridge (arrowhead) at the anterio-dorsal edge of the pronotum. (c) Scanning electron micrograph of the small release opening (black arrow) surrounded by membranous structures. Scales: (b) = 4 mm; (c) = 250 μm.
In situ preparations showed the paired defense glands in the dorsal prothorax (Figures 2ai,bi). In both sexes, the long glands span through the prothorax. For both sexes, the most obvious innervating nerve to the glands originates from the SOG (Figures 2aii,bii). This nerve is the subesophageal Nervus anterior (N. ant. SOG) which originates on each side of the SOG, runs under a cuticular apodeme on each side of the head and enters the prothorax toward the gland (Figures 2aii,bii). During the distance between the SOG and the gland, no further innervation targets occur. Close to the defense gland, the nerve splits into two nerve branches which contact the proximal gland surface. These two branches run on the dorsal and ventral side of the gland (for overview, see Figures 2, 3; for details also Figures 5a,c). There are four dorsal longitudinal muscles in the prothorax of A. paromalus (Figure 4), and their overall organization resembles that reported for C. morosus (Jeziorski, 1918; Marquardt, 1940). Based on their position and anterior attachment points at an apodeme behind the head capsule, the two anterior muscles are Idlm1 (the median muscle) and Idlm2 (the lateral muscle) (Friedrich and Beutel, 2008). In C. morosus, the more posterior muscles are termed 3a and 3b (Jeziorski, 1918) or D3 and D4 (Marquardt, 1940). These muscles are more difficult to homologize, since in C. morosus some dorsal longitudinal muscles are absent in the prothorax (Idlm3, Idlm6; Jeziorski, 1918; Leubner et al., 2016). By their positions, the two posterior longitudinal muscles could represent Idlm5 and Idlm6 (Friedrich and Beutel, 2008), or two sets of fibers from Idlm5. Importantly, the major anterior dorsal longitudinal muscles are still present in A. paromalus, and locate next to the defense gland (Figures 4, 5).
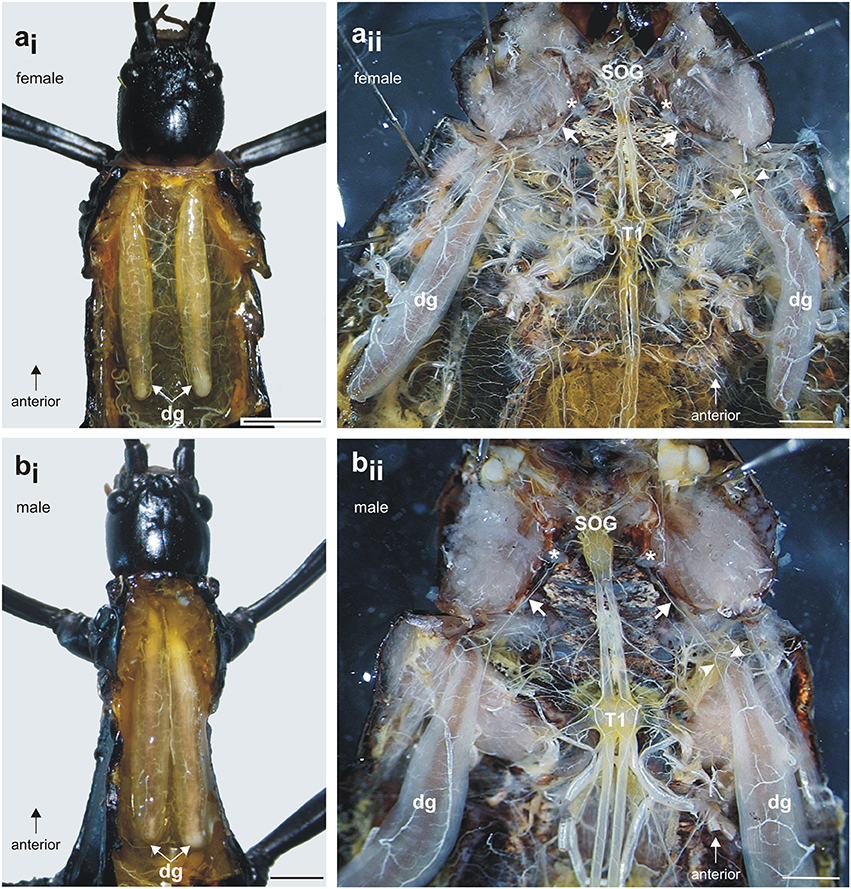
Figure 2. Defense glands in A. paromalus (a) female and (b) male individuals. (ai, bi) Paired defense glands lay dorsally in the prothorax. (aii, bii) The innervation by a prominent nerve from the subesophageal ganglion (SOG) is similar in both sexes: the nerve (white arrow) runs under a cuticular apodeme (asterisk) toward the anterior defense gland where it splits into two nerve branches which contact the gland surface (white arrowheads). Scales: female = 4 mm, male = 2 mm. dg, defense glands; T1, prothoracic ganglion; SOG, subesophageal ganglion.
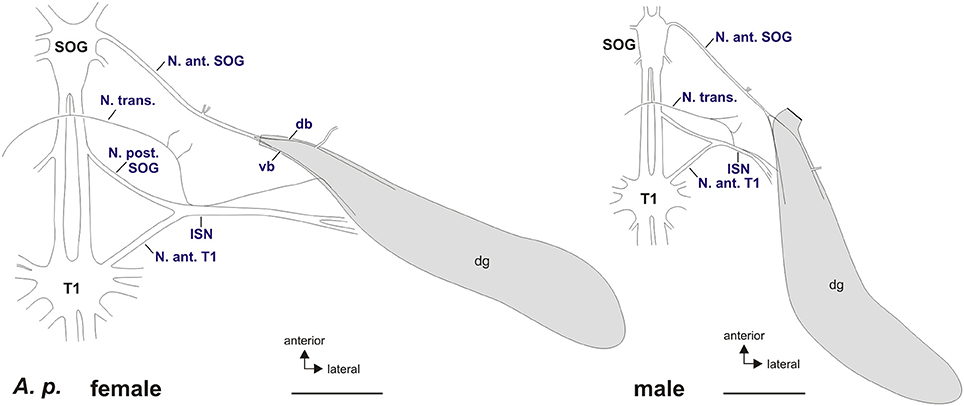
Figure 3. Schematic of peripheral nerves contacting the defense glands in A. paromalus female (left) and male (right). The defense glands are innervated by the Nervus anterior of the subesophageal ganglion (N. ant. SOG) and the intersegmental nerve complex (ISN) formed by the Nervus transversus (N. trans.), Nervus posterior of the subesophageal ganglion (N. post. SOG), and the Nervus anterior of the prothoracic ganglion (N. ant. T1) via a small nerve branch. The N. anterior SOG splits into a dorsal branch (db) and a ventral branch (vb) which contact the gland muscles. Scales = 2 mm. db, dorsal branch of N. anterior SOG; dg, defense glands; ISN, intersegmental nerve complex; N. ant. SOG, Nervus anterior of the subesophageal ganglion; N. ant. T1, Nervus anterior of the prothoracic ganglion; N. post. SOG, Nervus posterior of the subesophageal ganglion; N. trans., Nervus transversus; SOG, subesophageal ganglion; T1, prothoracic ganglion; vb, ventral branch of N. anterior SOG.
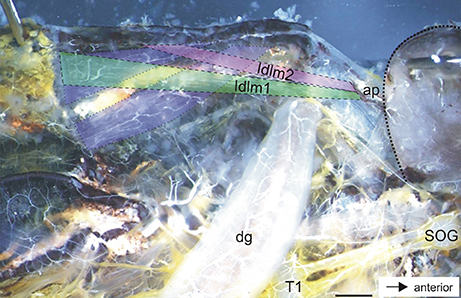
Figure 4. Dorsal longitudinal neck muscles in the prothorax of A. paromalus. Dorsal longitudinal muscles are indicated by hatched lines and color coded for Idlm1 (green), Idlm2 (magenta), and two posterior muscles (blue). Dotted line indicates the outline of the head capsule. Scale = 1.5 mm. ap, apodeme; dg, defense gland; SOG, subesophageal ganglion; T1, prothoracic ganglion.
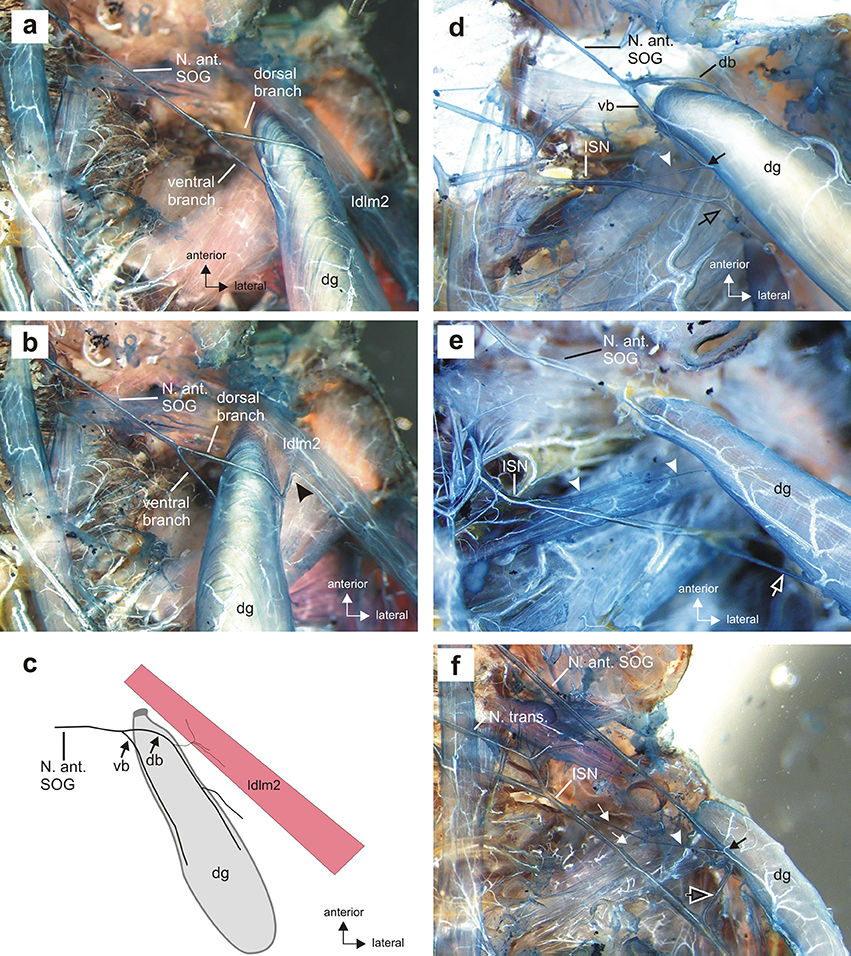
Figure 5. Details of the gland innervation in situ. (a) Innervation of the defense gland (dg) by two branches of the N. anterior SOG, the dorsal and ventral branch, in dorsal view. (b) The dorsal branch innervates both the defense gland by the larger dorsal branch contacting the gland muscle and a dorsal longitudinal muscle (Idlm2) in the prothorax by a short side branch (black arrowhead), apparent with defense gland turned medially. Longitudinal neck muscle Idlm1 was removed during preparation. (c) Schematic of the shared innervation of dorsal longitudinal muscle (Idlm2) by the dorsal branch of the N. anterior SOG; the ventral branch exclusively innervates the defense gland. (d) The intersegmental nerve complex (ISN) usually contacts the defense gland by a fine nerve branch (white arrowhead). The ventral nerve branch (vb) and the nerve branch from the ISN connect at the gland surface (black arrow). The ISN extends laterally of the defense gland toward a longitudinal muscle, Idlm1 (black open arrow). (e) A second contact from the ISN can occur further distally on the gland surface (white open arrow). White arrowheads indicate the fine branch in more anterior position. (f) Two fine branches of the ISN (white arrows) can merge before contacting the gland as a single fiber (white arrowhead), with a shared contact zone with the N. anterior SOG (black arrow) on the gland surface. Note the ISN extending laterally toward the longitudinal muscle Idlm1 (black open arrow). db, dorsal branch of Nervus anterior SOG; dg, defense gland; dlm, dorsal longitudinal muscle; ISN, intersegmental nerve complex; N. ant. SOG, Nervus anterior of the subesophageal ganglion; N. transv., Nervus transversus; vb, ventral branch of N. anterior SOG.
The innervation by N. ant. SOG is not exclusively on the gland, as a further nerve branch splits off from the dorsal branch which innervates a dorsal longitudinal neck muscle (Figures 5b,c). The muscle innervated together with the defense gland by N. ant. SOG (Figures 5b,c) is homologous to muscle Idlm2 in the neopteran groundplan (Friedrich and Beutel, 2008), and to the closely aligned muscles 50/51 in Orthoptera by its attachment sites and position (Altman and Kien, 1979; Honegger et al., 1984).
A further gland innervation originates from the intersegmental nerve complex, which is formed by three distinct nerves as they run toward the periphery: the Nervus posterior of the SOG (N. post. SOG), the Nervus anterior of the prothoracic ganglion (N. ant. T1), and the Nervus transversus (N. trans.) (Figure 3). From the nerve complex, a thin nerve branch splits off and contacts the defense gland. This nerve branch usually contacts the ventral branch of N. ant. SOG at the gland (Figure 5d). Some variability was found in the innervation by the intersegmental nerve complex, as an additional second branch from the intersegmental nerve complex could occur (Figure 5e) or two branches left the intersegmental nerve complex but fused into one branch which ultimately contacted the defense gland (Figure 5f). This overall innervation pattern is identical to the species studied before (Stolz et al., 2015; Stolz, 2017).
Axonal tracing of different nerves was used to confirm the individual nerves innervating the gland muscles, in particular in the intersegmental nerve complex (Figures 6a,e, Table 1). The two branches of N. ant. SOG form a fine network of arborizations on the gland surface (Figure 6b). While the ventral and dorsal branch at the anterior gland run over the gland surface (Figures 5a, 6b), histological sections of the middle of the gland showed that the two branches run between the outer longitudinal muscle layer and the inner ring muscle layer (Figure 6c). The longitudinal muscles are asymmetrically organized, with thicker longitudinal muscles at the ventral and dorsal side, and very thin longitudinal muscles at the medial and lateral side (Figure 6c). The secretory epithelium lines the inside of the gland (Figure 6). Sections revealed fine neuronal arborizations mainly in the outer longitudinal muscles (Figure 6d). A similarly clear innervation of the ring muscle fibers was not detectable.
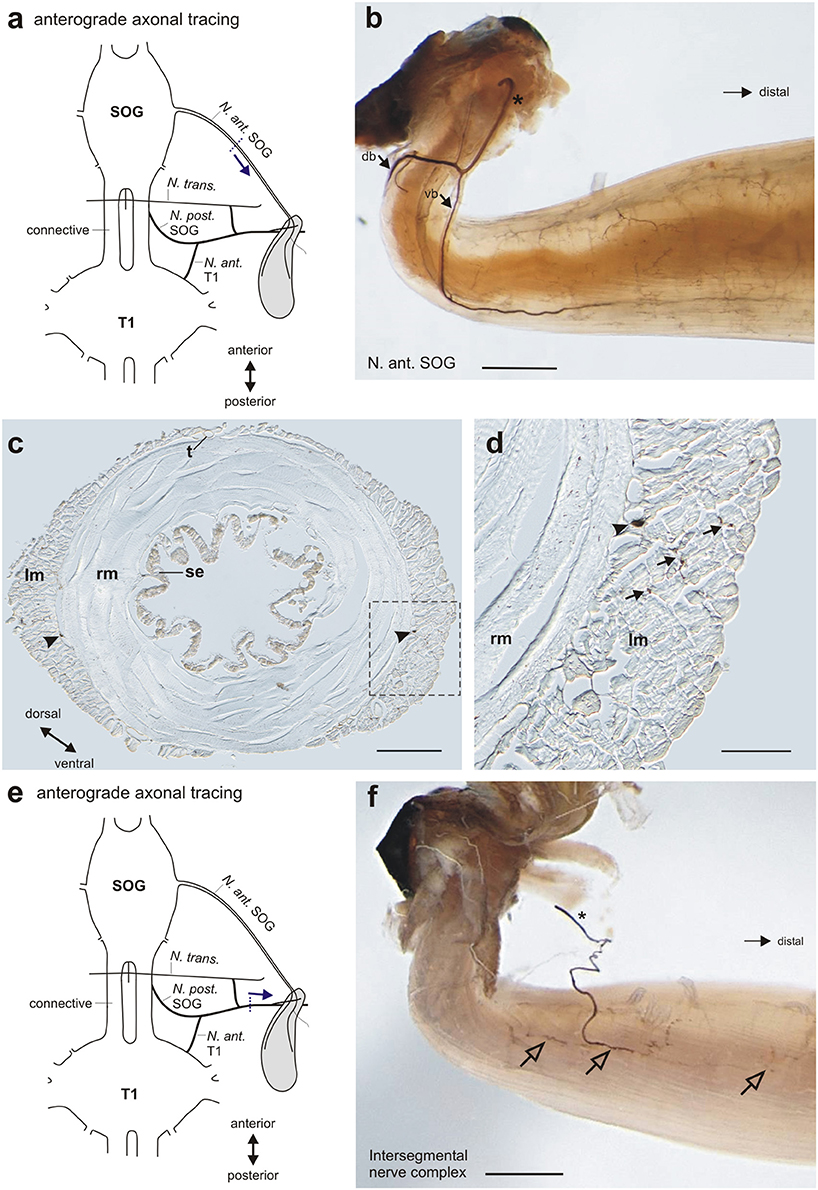
Figure 6. Neural innervation of the defense gland shown by axonal tracing. (a) Schematic for the anterograde tracing of N. ant. SOG toward the defense gland. Dotted line indicates cut site of the nerve, arrow indicates the direction of axonal tracing. (b) Staining of nerve branches (dorsal branch db, ventral branch vb) and fine nerve arborization on the gland muscle after tracing of N. ant. SOG. Cut end of N. ant. SOG is indicated by asterisk. (c) Histological section at the middle of the gland shows the two nerve branches (arrowheads) between the outer longitudinal muscles (lm) and inner ring muscles (rm). Note the asymmetric organization of the outer, longitudinal muscle layer. Boxed area is shown in magnification in (d). (d) Detail of muscle layers shows the stained nerve branch (arrowhead) at the border between longitudinal muscles (lm) and inner ring muscles (rm), and adjacent nerve endings in the outer longitudinal muscles (arrows). (e) Schematic for the anterograde tracing of the intersegmental nerve complex toward the defense gland. Dotted line indicates cut site of the nerve, arrow indicates the direction of axonal tracing. (f) Staining of the nerve branch from the intersegmental nerve complex (indicated by asterisk) and nerve arborizations on the gland muscle (open arrows) after tracing of the intersegmental nerve complex. Scales: (b,f) = 1 mm; (c) = 200 μm; (d) = 150 μm. db, dorsal branch of Nervus anterior SOG; lm, longitudinal muscles; N. ant. SOG, Nervus anterior SOG; N. ant. T1, Nervus anterior T1; N. post SOG, Nervus posterior SOG; N. trans., Nervus transversus; rm, ring muscles; SOG, subesophageal ganglion; se, secretory epithelium; t, trachea; T1, prothoracic ganglion; vb, ventral branch of Nervus anterior SOG.
In the intersegmental nerve complex, the fine nerve branch was also confirmed by anterograde axonal tracing toward the gland (Figure 6f). The nerve terminals occurred also on the gland surface but have a smaller innervation area. The different nerves of the intersegmental nerve complex were tested by individual tracing experiments. The obtained stainings showed an innervation by N. ant. SOG and N. post. T1, but not by N. transversus (Table 1). This overall innervation pattern is consistent with the innervation described for few other species (Stolz et al., 2015).
Identified Neurons Innervating the Defense Glands
We used retrograde axonal tracing on the N. ant. SOG and its nerve branches as well as the nerve branch of the intersegmental nerve complex to reveal the neurons with axons supplying the defense gland.
Tracing the complete N. ant. SOG (Figure 7a) stained three identified neuron types in the SOG (Figure 7bi) and one in the prothoracic ganglion (T1) (Figure 7bii). The neurons in the SOG have been termed the ipsilateral neuron (ILN) which has the largest soma of the neuron types discussed here, the contralateral neuron (CLN), and the ventral medial neurons (VMN) with the smallest somata located at the ganglions ventral midline (Figure 7bi) (see also Stolz et al., 2015). The number of VMNs can vary from 2 to 4 neurons but is in most preparations 3 VMNs. The neuron in the prothoracic ganglion has been termed the prothoracic intersegmental neuron (PIN) (Stolz et al., 2015). No difference in the neuron sets were found between the sexes.
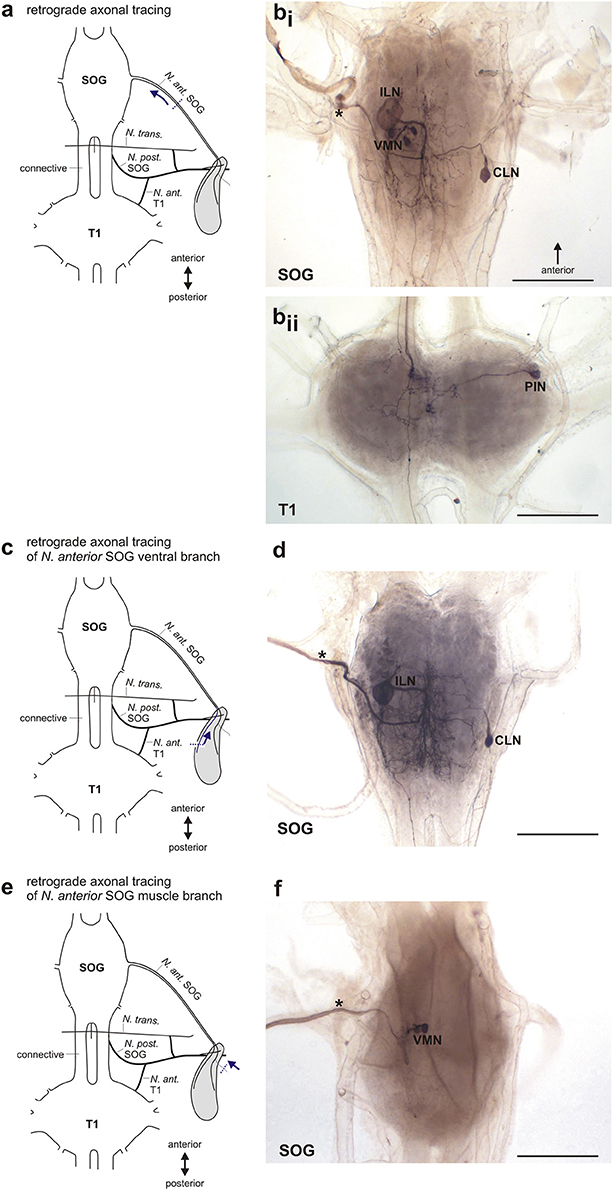
Figure 7. Retrograde axonal tracing of the N. anterior SOG. (a) Schematic for the retrograde tracing of N. ant. SOG toward the central nervous system. Dotted line indicates cut site of the nerve, arrow indicates the direction of axonal tracing. (b) Stained neuron types after retrograde tracing of N. ant. SOG in the subesophageal ganglion (bi) and prothoracic ganglion (bii). Traced nerve N. ant. SOG is indicated by asterisk. (c) Schematic for the retrograde tracing of the ventral branch of N. ant. SOG toward the central nervous system. Dotted line indicates cut site of the nerve, arrow indicates the direction of axonal tracing. (d) Stained neuron types after retrograde tracing of the ventral branch of N. ant. SOG (indicated by asterisk). (e) Schematic for the retrograde tracing of the nerve branch of N. ant. SOG innervating dorsal longitudinal muscle Idlm2 toward the central nervous system. Dotted line indicates cut site of the nerve, arrow indicates the direction of axonal tracing. (f) Stained neuron types after retrograde tracing of the nerve branch of N. ant. SOG innervating dorsal longitudinal muscle Idlm2 (indicated by asterisk). Scales = 500 μm. CLN, contralateral neuron; ILN, ipsilateral neuron; N. ant. SOG, Nervus anterior SOG; N. ant. T1, Nervus anterior T1; N. post. SOG, Nervus posterior SOG; N. trans., Nervus transversus; PIN, prothoracic intersegmental neuron; SOG, subesophageal ganglion; T1, prothoracic ganglion; VMN, ventral medial neurons.
We next used selective filling of N. ant. SOG nerve branches to distinguish between neurons innervating the defense glands, the longitudinal neck muscle Idlm2, or both. First, the ventral or dorsal nerve branches of N. ant SOG were carefully separated from the anterior gland surface (Figure 7c). For the ventral branch of N. ant. SOG (n = 15), the tracing revealed the subesophageal ILN and CLN (Figure 7d) as well as the prothoracic PIN (not shown). Among these neurons, the PIN was only stained in 50% of the preparations. This relatively rare staining was most likely due to the extended distance from tracing of N. ant. SOG close to the head capsule (Figure 7a) to tracing the ventral branch at the defense gland (Figure 7c). The subesophageal VMNs were never stained by tracing the ventral nerve branch of N. ant. SOG which innervates the defense gland. For tracing of the dorsal nerve branch of N. ant SOG innervating the defense gland (n = 7), the ILN and CLN were also reliably stained (not shown). In two of these preparations of the dorsal branch, the VMNs were also stained. Tracing the short nerve branch innervating the longitudinal neck muscle Idlm2 (Figure 7e; n = 8) stained the VMNs (Figure 7f), but no other neuron type. These selective backfills suggest that the ILN and CLN as well as the PIN innervate the defense gland, while the VMNs innervate the dorsal neck muscle Idlm2. The joint staining of ILN, CLN, and the VMNs in a subset of dorsal nerve branch fills was likely an artifact for the VMNs, due to the close proximity of the dorsal branch on the gland to the branch innervating the neck muscle. Based on the tracings of the ventral branch of the N. ant. SOG and the majority of the dorsal branch of the N. ant. SOG, the gland innervation by VMNs can be excluded. These findings provide evidence that distinct sets of neurons with axons in the N. ant. SOG innervate the defense gland (ILN, CLN, PIN) and the longitudinal neck muscle (VMNs).
Retrograde tracing of the nerve branch from the intersegmental nerve complex (Figure 8a) also revealed different neuron types in the SOG and the T1, by the N. post. SOG and the N. ant. T1. These experiments generally gave lower staining rates for the SOG, probably due to the thin nerve branch. In the SOG, a single contralateral neuron was stained (Figure 8bi). In the prothoracic ganglion, a dorsal unpaired median neuron (DUM), one contralateral neuron, and one median neuron with a curved neurite were stained (Figure 8bii). Since the nerve branch from the intersegmental nerve complex contacts only the defense gland, the neurons revealed by tracing of this nerve branch can be assumed to innervate the gland.
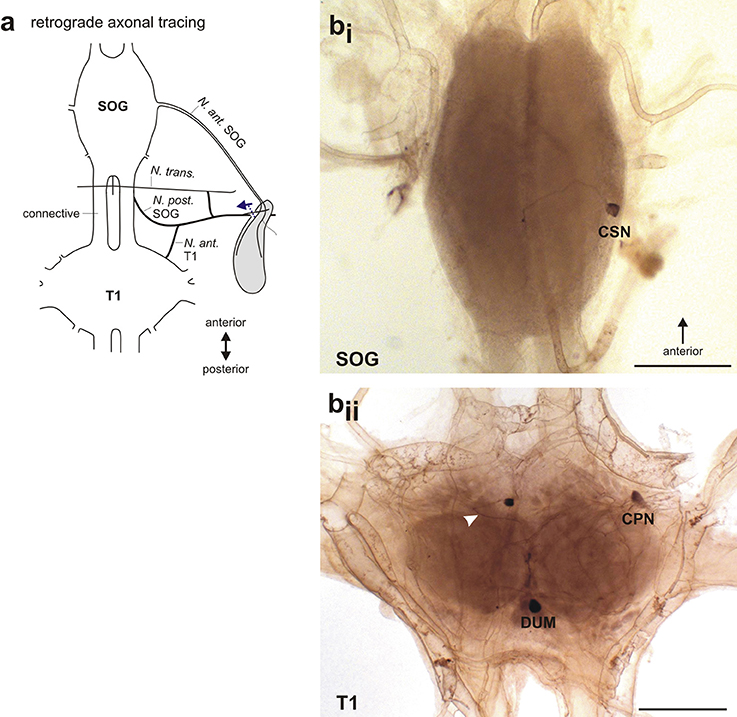
Figure 8. Retrograde axonal tracing of the intersegmental nerve complex. (a) Schematic for the retrograde tracing of the intersegmental nerve complex toward the central nervous system. Dotted line indicates cut site of the nerve, arrow indicates the direction of axonal tracing. (b) Stained neuron types after retrograde tracing of the intersegmental nerve complex in (bi) the subesophageal ganglion with one contralateral subesophageal neuron (CSN), and (bii) the prothoracic ganglion with a dorsal medial unpaired neuron (DUM), a contralateral prothoracic neuron (CPN), and a neuron located at the midline with a bending neurite (white arrowhead). Scale = 500 μm. CPN, contralateral prothoracic neuron; CSN, contralateral subesophageal neuron; DUM, dorsal unpaired median neuron; N. ant. SOG, Nervus anterior SOG; N. ant. T1, Nervus anterior T1; N. post. SOG, Nervus posterior SOG; N. trans., Nervus transversus; SOG, subesophageal ganglion; T1, prothoracic ganglion.
Since we noted differences in the soma size for the ILN between individuals (Figures 9ai,aii; see Supplementary Data), we measured the SOG sizes and somata sizes for ILN and CLN. Because the animals and also the subesophageal ganglia differ in sizes between sexes, we compared data from females and males. Since not all data tested showed normal distribution (p = 0.0011–0.1; KS distance = 0.1070–0.3480), we subsequently used the Mann-Whitney test for comparing data between the sexes. The absolute area of the SOG was significantly larger in females than in males (Figure 9b, females: n = 15, males: n = 13; one-tailed Mann-Whitney test: p < 0.0001, Mann-Whitney U = 9.000). For the ILN and CLN somata, we compared also absolute somata areas. The somata were significantly larger in females for both ILN somata (Figure 9c; females: n = 15, males: n = 13; one-tailed Mann-Whitney test: p = 0.0362, Mann-Whitney U = 58.00) and CLN somata (Figure 9d; females: n = 10, males: n = 9; one-tailed Mann-Whitney test: p = 0.0175, Mann-Whitney U = 19.00). To account for the size differences between sexes, we calculated relative somata sizes in relation to the ganglion area. Differences in relative somata sizes were not significant between sexes for the ILN (Figure 9e; females n = 15, males = 13; one-tailed Mann-Whitney test: p = 0.4816, Mann-Whitney U = 96.00) and the CLN (Figure 9f; females: n = 10, males: n = 9; one-tailed Mann-Whitney test: p = 0.0912; Mann-Whitney U = 28). We further tested for a correlation between ILN and CLN somata area and ganglion area. We found a statistically significant correlation between ILN somata area and SOG area for female A. paromalus (n = 15; one-tailed Spearman rank test: p = 0.0099, Spearman r = −0.5929), and between CLN somata area and SOG area for male A. paromalus (n = 9; one-tailed Spearman rank test: p = 0.0484, Spearman r = 0.600). However, no statistically significant correlation was found for male ILN (n = 13; one-tailed Spearman rank test: p = 0.0872, Spearman r = 0.4011) and for female CLN (n = 10; one-tailed Spearman rank test: p = 0.3161, Spearman r = −0.1758).
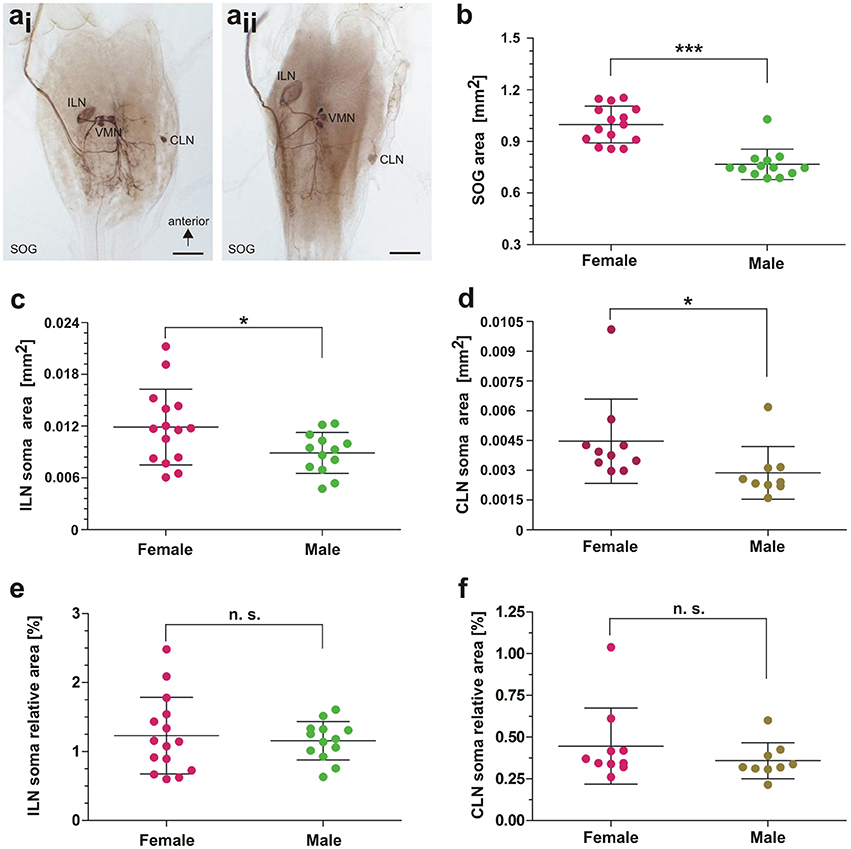
Figure 9. Morphometrical analysis of neuronal elements. (a) Examples of ILN size differences between two male individuals. (b) Comparison of subesophageal ganglion area in females and males; Mann-Whitney test, p < 0.0001. (c) Comparison of ILN soma area in females and males; Mann-Whitney test, p < 0.05. (d) Comparison of CLN soma area in females and males; Mann-Whitney test, p < 0.05. (e) Comparison in ILN soma relative area in females and males; Mann-Whitney test, p > 0.05. (f) Comparison in CLN soma relative area in females and males; Mann-Whitney test, p > 0.05. Scales = 500 μm. For details, see main text. CLN, contralateral neuron; ILN, ipsilateral neuron; SOG, subesophageal ganglion; VMN, ventral median neurons. Statistics: *0.05 > p > 0.01; ***p < 0.001.
Discussion
Innervation Pattern of the Defense Gland in Anisomorpha paromalus
The defense gland of A. paromalus is innervated by three distinct nerves, via the N. ant. SOG and the intersegmental nerve complex (N. post. SOG and N. ant. T1). The most prominent nerve is the N. ant. SOG, while the intersegmental nerve complex forms a fine nerve branch to the gland. This pattern is also found in other stick insects from different lineages (Timematidae: Timema chumash, Timema petita, Lonchodinae: C. morosus, Necrosciinae: Sipyloidea sipylus; Lanceocercata: Extatosoma tiaratum; Stolz et al., 2015; Stolz, 2017). The nerve pattern is thus identical across species irrespective of the particular mode of chemical defense, or even the lack of defensive secretions (in C. morosus; Carlberg, 1985a). Only in P. schultei (Pseudophasmatinae) is the innervation exclusively from the N. ant. SOG (Stolz et al., 2015). Anisomorpha species are very closely related to P. schultei (Goldberg et al., 2015), but the defense gland innervation is not specialized exclusively to the N. ant. SOG. Therefore, the innervation is only simplified in P. schultei. Other species including A. paromalus have an innervation by three distinct nerves (Stolz et al., 2015).
The peripheral nerve pattern is conserved also with respect to related taxa of Orthoptera (Honegger et al., 1984), Embioptera (Rähle, 1970), and Blattodea (Davis, 1983), where the nerves N. ant. SOG, N. post. SOG, and N. ant. T1 also innervate dorsal longitudinal (“neck”) muscles. The transverse nerve as part of the intersegmental nerve complex does not innervate the defense gland in stick insects (present study; Stolz et al., 2015). In Orthoptera, it supplies one to two smaller dorso-ventral muscles (Honegger et al., 1984).
Identified Neurons Innervating the Defense Gland and Evolutionary Novelties at the Cellular Level in a Conserved Innervation Pattern
Here, we conducted selective retrograde backfills of N. ant. SOG nerve branches from the defense gland or the Idlm2 neck muscle to identify the neurons which innervate gland muscles or thoracic neck muscles.
Tracing of the complete N. an. SOG reveals the ILN, CLN, VMNs, and PIN in the central nervous system (Figure 7b), as in other stick insects (Stolz et al., 2015). In A. paromalus, tracing the nerve branch of N. ant. SOG innervating the Idlm2 stains exclusively the VMNs. This neuron type is evolutionary conserved and innervates anterior dorsal longitudinal muscles in orthopteroid insects (Davis, 1983; Honegger et al., 1984), which we confirm also for stick insects (Figure 7f).
Tracing of the nerve branches on the defense gland reveals a subset of these neurons with ILN, CLN, and PIN. These neuron types are not present when tracing the homologous nerve 6 in Orthoptera (Altman and Kien, 1979; Honegger et al., 1984) or the homologous tergal nerve in cockroaches (Davis, 1983). The selective backfills thus show that the defense gland and Idlm2 are supplied by distinct neurons. The ILN, CLN, and PIN are therefore evolutionary novel types of neurons, which evolved in Phasmatodea with the development of the defense gland (Stolz, 2017). Notably, in the most basal phasmatodean lineage Timema only the VMNs are present. The ILN varies in soma size between different species of stick insects, with a larger soma in species with spraying release of defense secretion (see Stolz et al., 2015). The prominent ILN is thus likely the main motoneuron to elicit the gland discharge. The ILN soma size in A. paromalus also supports this correlation. Comparisons of the ILN and CLN soma sizes showed no statistically consistent correlation to the ganglion sizes for females and males, as female CLN soma area and male ILN soma area showed no significant correlation to the ganglion area. Only the homogenous relative soma areas show that differences in ILN and CLN soma sizes between sexes likely depend on differences in the size of the SOG and hence body size (Figures 9e,f). In orthopteran insects, large muscles with a high number of fibers are also innervated by relatively large motoneurons (Burrows and Hoyle, 1972; Wolf, 2014). Size differences of the ILN and CLN motoneurons might thus also be influenced by the size of the defense gland or the muscle layers. Currently, we also investigate the neuropil areas and tracts with neurite projections from the ILN/CLN or VMNs in the stick insect SOG from selective backfill experiments to analyze the possible functional separation of these neuronal systems in the central nervous system (Stolz, Strauß, et al., in prep.).
The innervation of the defense gland by the intersegmental nerve complex is less prominent than the innervation by the N. ant. SOG, consisting of a rather fine nerve branch. Despite the small size (compared to the N. ant. SOG), it also contains neurites from four neuron types. Since this traced nerve branch from the intersegmental nerve complex contacts only the defense gland (Figure 8a), these neurons all innervate the defense gland. They include a known neuromodulatory neuron, the DUM neuron (e.g., Hoyle, 1978; Duch et al., 1999; Bräunig and Pflüger, 2001), and further motoneurons. The DUM neuron supplying the defense gland via N. ant. T1 was also found in S. sipylus (Stolz et al., 2015). It is also found to innervate longitudinal neck muscles in locusts in Nerve 1 (homologous to N. ant. T1; Honegger et al., 1984; Kutsch and Heckmann, 1995a) and in cockroaches in the homologous tergal nerve (Davis, 1983). Such DUM or DUM-like neurons innervating dorsal longitudinal muscles via the intersegmental nerve complexes in different ganglia are strongly conserved in insects (Heckmann and Kutsch, 1990, 1995). For the N. ant. T1, a single contralateral neuron or few contralateral neurons in the prothoracic ganglion, which innervate the longitudinal muscle Idlm1, also occur in locusts (Honegger et al., 1984; Kutsch and Heckmann, 1995a). In contrast to the N. ant. SOG, the intersegmental nerve complex reveals not only a conserved nerve, but also conserved neuron types to innervate the muscles in the defense gland. In several species of insects, larger sets of motoneurons innervate muscles via the intersegmental nerve complex (Storrer et al., 1986; Heckmann and Kutsch, 1995; Kutsch and Heckmann, 1995b; Goldammer et al., 2012). Few of such contralateral and median neurons might have been recruited during the evolution of the gland, resulting in a specialized system innervated by single representatives of several identified neuron types.
Gland Muscle Innervation and Muscle Homologies
The stick insect defense gland is mainly innervated by the prominent N. ant. SOG, but also by the intersegmental nerve complex. The innervation by nerves and neurons can be used to gain insights into the evolutionary origin of the longitudinal muscles in the defense gland (Table 2). In locusts, the closely aligned muscle pair 50/51 (Idlm2) is the only longitudinal neck muscle supplied by the N. ant. SOG, as shown by retrograde axonal tracing of peripheral nerves (Honegger et al., 1984). In crickets, an occasional additional innervation of muscle 65 (Idlm3) by the VMNs was interpreted as a likely staining artifact (Honegger et al., 1984). This innervation pattern suggests that the longitudinal gland muscle derives, at least partly, from the longitudinal neck muscle Idlm2. This is further supported by the shared innervation of the defense gland and Idlm2 by nerve branches from the N. ant. SOG reported here (Figures 6b,c). The intersegmental nerve complex of Orthoptera innervates the dorsal longitudinal muscle Idlm1 (Honegger et al., 1984), which is close to Idlm2 (Friedrich and Beutel, 2008; for Timema, see Stolz, 2017). In A. paromalus and other stick insects, the intersegmental nerves not only innervate the defense gland, but also Idlm1 (Figures 5d,f; Stolz, 2017). This substantiates that also Idlm1 has contributed to the longitudinal musculature of the defense gland. The more posterior dorsal longitudinal muscle with unclear homology to the neopteran groundplan (Friedrich and Beutel, 2008) are apparently not involved in the gland muscle set.
Both the N. ant. SOG and the intersegmental nerve thus innervate dorsal longitudinal muscles and gland muscle (Figures 5, 6, Table 2). The shared innervation pattern of longitudinal muscles also found in Orthoptera suggests that both muscles, Idlm1 and Idlm2, have contributed to the longitudinal muscle fibers in the stick insect defense gland. Since the muscles persist in Phasmatodea, it is likely that sets of muscle fibers of both Idlm1 and Idlm2 contributed to forming the defense gland.
Histological sections from glands after axonal tracing confirm the innervation of longitudinal muscle fibers (Figures 6c,d). In frontal sections of the gland, the transversal fibers innervating ring muscles are more difficult to reveal. Innervation of the ring muscle might also be finer, but the position of the two main N. ant. SOG branches between the two muscle layers in the middle and distal gland indicates that both muscle layers are supplied by these branches. This would allow a simultaneous activation of muscle layers for fluid ejection.
Conclusion and Outlook
In sum, the defense gland in A. paromalus is innervated by a complex set of peripheral nerves (N. ant. SOG, N. post. SOG. N., ant. T1). The innervation pattern is identical to other stick insect species, including C. morosus, a species with relatively small defense glands which apparently does not produce defensive secretions. The nerves involved suggest that the defense gland muscles, at least in parts, derive from the longitudinal neck muscles Idlm1 and Idlm2. Selective backfills of nerve branches from N. ant. SOG to the gland or the dorsal longitudinal muscles reveal a functional specialization for both these systems with different motoneurons innervating the gland (ILN, CLN, PIN) and the dorsal longitudinal muscles (VMNs). The ILN, CLN, and PIN are evolutionary novel motoneurons. This suggests that they evolved with the evolutionary development of the defense gland. A similar pattern was shown for two neuron types from the intersegmental nerves. These neuroanatomical findings show that the conserved innervation pattern has allowed the evolution of the gland control by specialization of few additional motoneurons. It remains to be investigated further how the different nerves and neuron types independently or together activate and modulate the gland musculature, and how the muscle layers coordinate during contraction and fluid ejection.
Author Contributions
Carried out experiments: JS, C-RvB, YvB. Carried out preparations: JS, KS. Analyzed data: JS, C-RvB, YvB, KS. Provided reagents and lab facilities: RL-H, TT. Assembled figures: JS. Drafted and wrote manuscript: JS. Edited manuscript: JS, C-RvB, YvB, KS, TT, RL-H. All authors read the manuscript and agreed with the publication.
Conflict of Interest Statement
The authors declare that the research was conducted in the absence of any commercial or financial relationships that could be construed as a potential conflict of interest.
The reviewer FL and handling Editor declared their shared affiliation.
Acknowledgments
We thank Dr. Michael Gebhardt, Freising, for providing the Anisomorpha individuals for this study. We thank Julia Nothacker for providing additional Anisomorpha individuals. We thank Dr. Martin Hardt and Sabine Agel of the Imaging Unit at Justus-Liebig-Universität Gießen for excellent help with SEM. We thank two reviewers for very constructive and helpful comments which improved this manuscript.
Supplementary Material
The Supplementary Material for this article can be found online at: https://www.frontiersin.org/articles/10.3389/fevo.2017.00151/full#supplementary-material
References
Altman, J. S., and Kien, J. (1979). Suboesophageal neurons involved in head movements and feeding in locusts. Proc. R. Soc. Lond. B. 205, 209–227. doi: 10.1098/rspb.1979.0061
Arbas, E. A., Meinertzhagen, I. A., and Shaw, S. R. (1991). Evolution in the nervous system. Annu. Rev. Neurosci. 14, 9–38. doi: 10.1146/annurev.ne.14.030191.000301
Bässler, U. (1977). Sense organs in the femur of the stick insect and their relevance to the control of position of the femur-tibia-joint. J. Comp. Physiol. 121, 99–113. doi: 10.1007/BF00614183
Bedford, G. O. (1978). Biology and ecology of the Phasmatodea. Ann. Rev. Entomol. 23, 25–149. doi: 10.1007/978-3-642-68813-3
Bouchard, P., Hsiung, C.-C., and Yaylayan, V. A. (1997). Chemical analysis of defense secretions of Siyploidea sipylus and their potential use as repellents against rats. J. Chem. Ecol. 23, 2049–2057. doi: 10.1023/B:JOEC.0000006488.58081.66
Bradler, S. (2009). Die Phylogenie der Stab- und Gespenstschrecken (Insecta: Phasmatodea). Species Phyl. Evol. 2, 1–139. doi: 10.17875/gup2009-710
Bräunig, P., and Pflüger, H. J. (2001). The unpaired median neurons of insects. Adv. Insect Physiol. 28, 185–266. doi: 10.1016/S0065-2806(01)28011-4
Brown, M., and Lowe, D. G. (2007). Automatic panoramic image stitching using invariant features. Int. J. Comput. Vision 74:59. doi: 10.1007/s11263-006-0002-3
Burrows, M., and Hoyle, G. (1972). Neural mechanisms underlying behavior in the locust Schistocerca gregaria. III. Topography of limb motorneurons in the metathoracic ganglion. J. Neurobiol. 4, 167–186. doi: 10.1002/neu.480040207
Carlberg, U. (1981). An analysis of the secondary defence reactions in stick insects (Phasmida). Biol. Zentralblatt 100, 295–303.
Carlberg, U. (1985a). Secondary defence in Carausius morosus (de Sinety) (Insecta: Phasmida). Zool. Anz. 215, 373–384.
Carlberg, U. (1985b). Chemical defense in Extatosoma tiaratum (Macleay) (Insecta, Phasmida). Zool. Anz. 214, 185–192.
Carlberg, U. (1985c). Chemical defense in Anisomorpha buprestoides (Houttuyn in Stoll) (Insecta, Phasmida). Zool. Anz. 215, 177–188.
Carlberg, U. (1986b). Chemical defense in Sipyloidea sipylus (Westwood) (Insecta, Phasmida). Zool. Anz. 217, 31–38.
Chow, Y. S. (2008). “Walkingstick defensive behavior and regeneration of appendages,” in Encyclopedia of Entomology, 2nd Edn., ed J. L. Capinera (Springer: Netherlands), 4133–4135.
Chow, Y. S., and Lin, Y. M. (1986). Actinidine, a defensive secretion of stick insect, Megacrania alpheus Westwood (Orthoptera: Phasmatidae). J. Entomol. Sci. 21, 97–101. doi: 10.18474/0749-8004-21.2.97
Davis, N. T. (1983). Serial homologies of the motor neurons of the dorsal intersegmental muscles of the cockroach, Periplaneta americana (L.). J. Morphol. 176, 197–210. doi: 10.1002/jmor.1051760208
Dettner, K. (2015). “Toxins, defensive compounds and drugs from insects,” in Insect Molecular Biology and Ecology, ed K. H. Hoffmann (Boca Raton FL: CRC Press), 39–93.
Dossey, A. T., Walse, S. S., and Edison, A. S. (2008). Developmental and geographical variation in the chemical defense of the walkingstick Anisomorpha buprestoides. J. Chem. Ecol. 34, 584–590. doi: 10.1007/s10886-008-9457-8
Dossey, A. T., Walse, S. S., Rocca, J. R., and Edison, A. S. (2006). Single insect NMR: a new tool to probe chemical biodiversity. ACS Chem. Biol. 1, 511–514. doi: 10.1021/cb600318u
Dossey, A. T., Whitaker, J. M., Dancel, M. C., Vander Meer, R. K., Bernier, U. R., Gottardo, M., et al. (2012). Defensive spirotekals from Asceles glaber (Ohasmatoidea): absolute configuration and effects on ants and mosquitoes. J. Chem. Ecol. 38, 1105–1115. doi: 10.1007/s10886-012-0183-x
Duch, C., Mentel, T., and Pflüger, H.-J. (1999). Distribution and activation of different types of octopaminergic DUM neurons in the locust. J. Comp. Neurol. 403, 119–134. doi: 10.1002/(SICI)1096-9861(19990105)403:1<119::AID-CNE9>3.0.CO;2-F
Eisner, T. (1965). Defensive spray of a phasmid insect. Science 148, 966–968. doi: 10.1126/science.148.3672.966
Eisner, T., Eisner, M., and Siegler, M. (2005). Secret Weapons. Defenses of Insects, Spiders, Scorpions, and Other Many-Legged Creatures. Cambridge, MA: Belknap.
Eisner, T., Morgan, R. C., Attygalle, A. B., Smedley, S. R., Herath, K. B., and Meinwald, J. (1997). Defensive production of quinoline by a phasmid insect (Oreophoetes peruana). J. Exp. Biol. 200, 2493–2500.
Friedrich, F., and Beutel, R. G. (2008). The thorax of Zorotypus (Hexapoda, Zoraptera) and a new nomenclature for the musculature of Neoptera. Arthropod. Structure Dev. 37, 29–54. doi: 10.1016/j.asd.2007.04.003
Goldammer, J., Büschges, A., and Schmidt, J. (2012). Motoneurons, DUM cells, and sensory neurons in an insect thoracic ganglion: a tracing study in the stick insect Carausius morosus. J. Comp. Neurol. 520, 230–257. doi: 10.1002/cne.22676
Goldberg, J., Bresseel, J., Constant, J., Kneubühler, B., Leubner, F., Michalik, P., et al. (2015). Extreme convergence in egg-laying strategy across insect orders. Sci. Rep. 5:7825. doi: 10.1038/srep07825
Goodman, C. S., Pearson, K. G., and Heitler, W. J. (1979). Variability of identified neurons in grasshoppers. Comp. Biochem. Physiol. A 64, 455–462. doi: 10.1016/0300-9629(79)90571-1
Grimaldi, D. G., and Engel, M. (2005). Evolution of the Insects. Cambridge: Cambridge University Press.
Happ, G. M., Strandberg, J. D., and Happ, C. M. (1966). The terpene-producing glands of a phasmid insect. Cell Morphol. Histochem. J. Morphol. 119, 143–160. doi: 10.1002/jmor.1051190204
Heckmann, R., and Kutsch, W. (1990). “Common neural “Bauplan” in Tracheata? Innervation of the dorsal longitudinal muscles,” in Brain – Perception – Recognition, eds N. Elsner and G. Roth (Stuttgart: Thieme Verlag), 39.
Heckmann, R., and Kutsch, W. (1995). Motor supply of the dorsal longitudinal muscles II: comparison of motoneurone sets in Tracheata. Zoomorphology 115, 197–211. doi: 10.1007/BF00393800
Ho, H.-Y., and Chow, Y. S. (1993). Chemical identification of defensive secretion of stick insect, Megacrania tsudai Shiraki. J. Chem. Ecol. 19, 39–46. doi: 10.1007/BF00987469
Honegger, H.-W., Altman, J. S., Kein, J., Müller-Tautz, R., and Pollerberger, E. (1984). A comparative study of neck muscle motor neurons in a cricket and a locust. J. Comp. Neurol. 230, 517–535. doi: 10.1002/cne.902300404
Hoyle, G. (1978). The dorsal, unpaired, median neurones of the locust metathoracic ganglion. J. Neurobiol. 9, 43–57. doi: 10.1002/neu.480090105
Hoyle, G. (1983). “On the way to neuroethology: The identified neuron approach,” in Neuroethology and Behavioral Physiology. Roots and Growing Points, eds F. Huber and H. Markl (Heidelberg: Springer), 9–25.
Kutsch, W., and Breidbach, O. (1994). Homologous structures in the nervous systems of Arthropoda. Adv. Insect Physiol. 24, 1–113. doi: 10.1016/S0065-2806(08)60082-X
Kutsch, W., and Heckmann, R. (1995a). Motor supply of the dorsal longitudinal muscles, I: homonomy and ontogeny of the motoneurones in locusts (Insecta, Caelifera). Zoomorphology 115, 179–195. doi: 10.1007/BF00403172
Kutsch, W., and Heckmann, R. (1995b). “Homologous structures, exemplified by motoneurons of Mandibulata,” in The Nervous System of Invertebrates: An Evolutionary and Comparative Approach, eds O. Breidbach and W. Kutsch (Basel: Birkhäuser), 221–248.
Leubner, F., Hörnschemeyer, T., and Bradler, S. (2016). The thorax of the cave cricket Troglophilus neglectus: anatomical adaptations in an ancient wingless insect lineage (Orthoptera: Rhaphidophoridae). BMC Evol. Biol. 16:39. doi: 10.1186/s12862-016-0612-5
Marquardt, F. (1940). Beiträge zur Anatomie der Muskulatur und der peripheren Nerven von Carausius (Dixippus) morosus. BR. Zool. Jahrb. Anat. Ontog. 66, 63–128.
Meinwald, J., Chadha, M. S., Hurst, J. J., and Eisner, T. (1962). Defense mechanisms of arthropods. 9. Anisomorphal, the secretion of a phasmid insect. Tetrahedron Lett. 3, 29–33. doi: 10.1016/S0040-4039(00)62038-5
Nentwig, W. (1990). Stick insects (Phasmida) as prey of spiders: size, palatability and defence mechanisms in feeding tests. Oecologia 82, 446–449. doi: 10.1007/BF00319784
Rähle, W. (1970). Untersuchungen an Kopf und Prothorax von Embia ramburi Rimsky-Korsakow 1906 (Embioptera, Embiidae). Zool. Jahrb. Anat. Onto. 87, 248–330.
Schmeda-Hirschmann, G. (2006). 4-Methyl-1-hepten-3-one, the defensive compound from Agathemera elegans (Philippi) (Phasmatidae) Insecta. Z. Naturforschung 61, 592–594. doi: 10.1515/znc-2006-7-820
Smith, R. M., Brophy, J. J., Cavill, G. W. K., and Davies, N. W. (1979). Iridodials and nepetalactone in the defensive secretion of the coconut stick insects, Graeffea crouani. J. Chem. Ecol. 5, 727–735. doi: 10.1007/BF00986557
Stolz, K. (2017). Functional morphology and neuronal innervation of the prothoracic defense gland in Timema. Acta Zool. doi: 10.1111/azo.12216. [Epub ahead of print].
Stolz, K., von Bredow, C.-R., von Bredow, Y. M., Lakes-Harlan, R., Trenczek, T. E., and Strauß, J. (2015). Neurons of self-defence: neuronal innervation of the exocrine defence glands in stick insects. Front. Zool. 12:29. doi: 10.1186/s12983-015-0122-0
Storrer, J., Bässler, U., and Mayer, S. (1986). Motoneurone im Meso- und Metathorakalganglion der Stabheuschrecke Carausius morosus. Zool. Jahrb. Abt. Allg. Zool. Physiol. Tiere 90, 359–374.
Strong, L. (1975). Defence glands in the giant spiny phasmid Extatosoma tiaratum. J. Entomol. 50, 65–72. doi: 10.1111/j.1365-3032.1975.tb00093.x
van de Kamp, T., dos Santos Rolo, T., Baumbach, T., and Greven, H. (2015). X-ray radiography of a spraying insect (Phasmatodea). Entomol. Heute 27, 37–44.
Weidler, D. J., and Diecke, F. P. J. (1969). The role of cations in conduction in the central nervous system of the herbivorous insect Carausius morosus. Z. Vergl. Physiol. 64, 372–399. doi: 10.1007/BF00340433
Wipfler, B., Klug, R., Ge, S. Q., Bai, M., Göbbels, J., Yang, X. K., et al. (2015). The thorax of Mantophasmatodea, the morphology of flightlessness, and the evolution of the neopteran insects. Cladistics 31, 50–70. doi: 10.1111/cla.12068
Keywords: stick insect, neuroanatomy, defense gland, innervation, motoneurons
Citation: Strauß J, von Bredow C-R, von Bredow YM, Stolz K, Trenczek TE and Lakes-Harlan R (2017) Multiple Identified Neurons and Peripheral Nerves Innervating the Prothoracic Defense Glands in Stick Insects Reveal Evolutionary Conserved and Novel Elements of a Chemical Defense System. Front. Ecol. Evol. 5:151. doi: 10.3389/fevo.2017.00151
Received: 30 September 2017; Accepted: 14 November 2017;
Published: 30 November 2017.
Edited by:
Sven Bradler, University of Göttingen, GermanyReviewed by:
Fanny Leubner, University of Göttingen, GermanyThomas Van De Kamp, Karlsruhe Institute of Technology, Germany
Copyright © 2017 Strauß, von Bredow, von Bredow, Stolz, Trenczek and Lakes-Harlan. This is an open-access article distributed under the terms of the Creative Commons Attribution License (CC BY). The use, distribution or reproduction in other forums is permitted, provided the original author(s) or licensor are credited and that the original publication in this journal is cited, in accordance with accepted academic practice. No use, distribution or reproduction is permitted which does not comply with these terms.
*Correspondence: Johannes Strauß, am9oYW5uZXMuc3RyYXVzc0BwaHlzem9vbC5iaW8udW5pLWdpZXNzZW4uZGU=
Reinhard Lakes-Harlan, cmVpbmhhcmQubGFrZXMtaGFybGFuQHBoeXN6b29sLmJpby51bmktZ2llc3Nlbi5kZQ==
†These authors have contributed equally to this work.