- 1Wellman Center for Photomedicine, Massachusetts General Hospital, Harvard Medical School, Boston, MA, United States
- 2School of Human Sciences, London Metropolitan University, London, United Kingdom
Topical and transdermal delivery has historically offered an attractive and non-invasive route for administration of medicines. However, human skin is known to be a remarkably good barrier to the permeation of substances. The majority of dermatological drug products have been reported to only deliver a portion of the total dose applied, often resulting in low drug bio-availability at the site of action inside the skin. This insufficient formulation performance, coupled with the fact that percutaneous delivery is heavily influenced by the innate physicochemical properties of the active, pose limitations on effective treatment and prevention of diseases by using solely topical formulations. Generally, it is known that the rate and the extent of drug delivery to and through the skin is highly dependent on the formulation components. This work highlights the importance of the vehicle for the design of efficacious skin products, discusses current limitations in dermal delivery and explores recent advances for overcoming these challenges. Novel materials with penetration enhancing properties and innovative formulation strategies are also explored, together with future perspectives and outlooks. The emphasis here is on studies focused on passive skin transport because of clinical limitations associated with disrupting the skin barrier by physical methods. This information is believed to aid in the design and optimization of dermatological drug products for topical and transdermal delivery of actives.
1 Introduction
The therapeutic efficacy of dermatological drug products is characterized by the rate and extent that the active reaches the desired site of action. For topical formulations, this may be located in specific areas inside the viable epidermis and/or the dermis. Additionally, intra-follicular drug disposition is of special significance for the targeted treatment of conditions related to skin appendages, such as hair growth disorders or sebaceous gland-associated disorders. In contrast, for transdermal delivery systems, the active must permeate across the tissue and reach the blood circulation for systemic effects (Lane et al., 2011; Patzelt and Lademann, 2020).
Topical and transdermal drug delivery systems have been approved for clinical use for many years. The last decades have seen a considerable growth of skin formulation development, with the global dermatological drug market projected to reach approximately USD 40 billion by 2030. Yet, to date, only a limited number of drugs are considered as suitable for administration via the percutaneous route, mainly owing to the selective permeability of the skin barrier. Drugs of non-optimal physicochemical properties and potency (e.g., macro-molecules) remain excluded from conventional dermatological drug development, thereby limiting the medical applications of the field (Prausnitz et al., 2004).
Recent technological advances in drug delivery systems have shown promising results in terms of addressing the deficiencies and limitations of conventional means for dermatological drug administration. This review focuses on the utility of film-forming systems (FFS) and ionic liquid (IL) -based systems, as innovative passive permeation enhancement strategies for targeted delivery into and through the skin, and highlights the importance of appropriate excipient selection for the design of efficacious skin products. The selection of studies was based on permeation data using porcine or human skin, since results from these membranes are known to correlate with human in vivo data (Barbero and Frasch, 2009; Huong et al., 2009; Hopf et al., 2020). The use of nano-delivery systems, such as nano-emulsions, nano-fibers and nano-carriers, also included in the passive enhancement category, has been discussed elsewhere in the literature (Prow et al., 2011; Cui et al., 2020; Almeida et al., 2022; Tapfumaneyi et al., 2022) and was not be the focus of the current work. Physical enhancement involves a wide range of invasive methods to disrupt the skin barrier, including the use of microneedles or application of electrical energy. Detailed reviews on novel methods of such delivery have been produced elsewhere (Cross and Roberts, 2004; Larran˜eta et al., 2016; Benson et al., 2019).
2 Skin barrier and percutaneous absorption
The human skin is a large and complex multilayered structure. It comprises approximately 10% of the total body mass of an average person and covers a surface area of 1.5–2 m2. It is the interface between the body and the external environment, and its primary role is to act as a protective barrier against the ingress of toxins and microbes, as well as the egress of endogenous water. This barrier function is determined by the thin (10–20 µm) outermost layer of the skin, namely the stratum corneum (SC), which also represents the non-viable part of the tissue (Hadgraft, 2001, 2004; Hadgraft and Lane, 2011). The SC consists of tightly connected terminally differentiated protein-enriched cells (keratinocytes) embedded in an inter-cellular densely packed lipid lamellae, in a structural arrangement analogous to a ‘brick and mortar wall’, where the bricks correspond to the corneocytes and the mortar to the lipid matrix (Michaels et al., 1975). The molecular architecture of the SC, including the composition and organization of the lipid domain as well as metabolic responses and cellular signalling underlying barrier repair and homeostasis have been thoroughly discussed in the literature (Proksch et al., 2008; Elias, 2012; Menon et al., 2012; Boncheva, 2014; van Smeden et al., 2014; Schmitt and Neubert, 2018, 2020; Starr et al., 2022). This pattern of SC organization defines the barrier properties and determines the penetration pathways for a molecule to cross the membrane. Transport across the SC occurs via passive diffusion through three routes of permeation: the inter-cellular (i.e., via the lipid lamellae), intra-cellular (i.e., in a straight line through both corneocytes and lipid matrix) and/or via skin appendages (trans-appendageal) (Figure 1).The inter-cellular route via the continuous lipid domain is generally accepted as the predominant permeation pathway. This represents a tortuous route of diffusion that has been estimated to be ∼ 500 μm, i.e., more than 20 times longer than just the simple thickness of the SC (Hadgraft, 2004). The inter-cellular diffusion of molecules has been confirmed in many visualization studies over the years (Elias and Friend, 1975; Bodde´ et al., 1989; Bodde´ et al., 1991; Neelissen et al., 2000; Talreja et al., 2001). Additionally, the trans-appendageal route, via shunt pathways formed by hair follicles and sweat glands, is known to play an important role in percutaneous absorption (Feschuk et al., 2022). Scheuplein (1967) proposed that shunt diffusion is predominant in the early stages shortly after topical application of formulations, and it is eventually overtaken at longer times before steady-state absorption is established by general partition and diffusion through the intact SC. Over the years, several studies have investigated the distribution of topically applied chemicals in hair follicles, demonstrating the considerable contribution of this pathway (Grams et al., 2004; Frum et al., 2007; Otberg et al., 2008; Farah et al., 2020; Mangion et al., 2022). The intra-cellular pathway requires the permeant to travel perpendicular to the skin surface, passing into and out of the protein-rich corneocytes and the inter-cellular lipids sequentially. The corneocytes are filled with water and microfibrillar keratin, surrounded by a cornified envelope comprised of a densely crosslinked layer of proteins (van Smeden et al., 2014). The partitioning of molecules into these regions is generally assumed to be hindered, and the contribution of this route has been largely discounted. It is worth noting, however, that diffusion through corneocytes has been reported by various experimental studies using human skin ex vivo, demonstrating that the trans-cellular route may have a significant contribution for the absorption of several actives (Yu et al., 2003; Kushner et al., 2007; Barbero and Frasch, 2017).
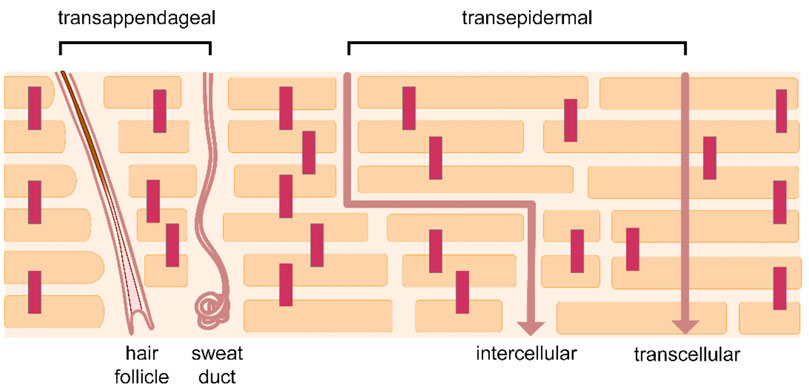
FIGURE 1. Skin permeation pathways. Reprinted from Rahma and Lane (2022), according to the terms and conditions of the Creative Commons Attribution 4.0 International License (CC BY 4.0).
The process of topical and transdermal delivery begins with the partitioning of the active from the vehicle to the SC. After penetrating to the SC, the drug must diffuse through the membrane until it reaches the end of the SC, where it must partition to the water-rich viable epidermis. The hydrophilic nature of this region can be a rate limiting barrier for the permeation of highly lipophilic drugs in deeper layers (Hadgraft and Lane, 2011; Lane et al., 2011). Naturally, these processes are determined by the innate physicochemical properties of the solute, i.e., moderate lipophilicity will enable partitioning/solubility to the various layers of the skin (optimal range of logP (o/w) between 1 and 3); relatively low molecular mass (<500 Da) and low melting point will promote diffusivity through the permeation pathways. The degree of ionization, and the presence of hydrogen bonding groups on the permeant are additional important parameters that may affect the actives’ ability to move in lipophilic or hydrophilic environments (Barry, 2001; Hadgraft, 2004). Clearly, the number of compounds that possess these characteristics is limited, and therefore innovative formulation strategies are required to extend the range of compounds that can be used for percutaneous absorption.
3 Strategies to enhance percutaneous drug delivery
3.1 Thermodynamic activity and supersaturation
The chemical potential gradient is known to be the driving force for diffusion, and the manipulation of the thermodynamic activity of the drug in the vehicle has been used as a strategy to increase drug penetration to the skin for many years. The thermodynamic activity of a solute is defined by its degree of saturation i.e., the concentration of the solute in the vehicle as a fraction of the maximum solubility in that same vehicle. Typically, maximum thermodynamic activity, equal to one, will be reached when the drug saturates the formulation. Greater thermodynamic activity values can be achieved by abrupt changes of drug concentration in the vehicle that force the solute to exceed its saturation solubility and reach a supersaturated state. A major problem with this strategy is that supersaturated solutions are inherently thermodynamically unstable due to eventual recrystallization. Ideally, the supersaturated states should be achieved in situ upon application, for example following the depletion of volatile formulation components. This can be accomplished by the use of appropriate combinations of volatile and non- or less volatile solvent systems. In this scenario, a large part of the applied formulation will be rapidly lost by evaporation, thereby leading to increased drug concentrations in the residual phase on the skin. This approach was initially reported many decades ago, by Coldman et al. (1969), who examined the in vitro percutaneous absorption of fluocinolide and fluocinolone acetonide from binary solvent systems consisting of the volatile isopropanol and either propylene glycol or isopropyl myristate at various ratios. Maximum drug permeation was found from formulations with high amounts of volatile solvent in the system, and this effect was attributed to the high thermodynamic potential of the drug at supersaturated state in the remaining solvent.
A relatively recent application of this strategy involved the development of polymeric film-forming systems (FFS) as innovative formulations to achieve enhanced and sustained percutaneous delivery (Reid et al., 2009). Generally, FFS contain volatile solvent(s) that, upon application to the skin, evaporate leaving the drug in a residual film of excipients on the skin surface. A major challenge for an efficient FFS is to sustain the delivery of actives after the initial transient release of the compound. After the evaporation of the volatile solvent, the residual phase composition should contain sufficient drug in a molecularly dispersed state within the residual film to ensure the capability of the drug for sustained diffusion (Frederiksen et al., 2016). Appropriate polymer selection is critical for preventing crystallization of the active from a supersaturated state, as well as for achieving optimal rate of delivery, as demonstrated in a recent study by Edwards et al. (2017). These researchers investigated the impact of two different polymethacrylate copolymers, Eudragit RS and Eudragit E, on the flux of both methylphenidate and methylphenidate hydrochloride, across a silicone membrane in vitro. Overall, drug delivery was reported to be linearly proportional to the degree of drug saturation in the polymeric film formulation, estimated by the drug solubility in the polymer. Additionally, drug delivery was dependent on the degree of anti-nucleant action of the selected polymer. One of the first FFS to be approved for the treatment of skin disease was the anti-fungal single dose Lamisil® Once (Kienzler et al., 2007). This formulation consisted of ethanol as the volatile solvent, terbinafine hydrochloride as the active pharmaceutical ingredient, and acrylates/octylacrylamide copolymer as the preparation’s polymeric backbone. Upon application on the skin, the rapid evaporation of the solvent resulted in the formation of a drug-containing residual film that promoted prolonged delivery of the active to the SC. In vivo human dermatopharmacokinetic studies showed that the FFS system had a greater residence time on the skin and promoted greater drug penetration to the SC compared to a cream vehicle of the same drug concentration (1%) when applied as a single dose. Later experimental studies investigated the impact of additional excipients into the FFS on the duration and the extent of drug delivery to the skin ex vivo. Garvie-Cook et al. (2015) examined the skin distribution of a lipophilic drug, betamethasone 17-valerate, from various FFS to porcine skin ex vivo by the tape-stripping technique. Higher drug uptake was reported from hydrophobic FFS than from those containing a more hydrophilic polymer. Drug was observed to be rapidly taken up into the SC from which it subsequently diffused into the lower, viable skin layers over a sustained period. The authors proposed that hydrogen bond interactions between the drug and the hydrophobic acrylate polymers may allow for the formation of drug ‘reservoirs’ in both the residual polymeric film on the skin surface, as well as within the SC, from which sustained delivery can be maintained. In the presence of a lipophilic excipient (medium-chain triglyceride) into the hydrophobic FFS, the films were reported to adopt a two-phase film structure (soft lipid-enriched inclusions within a solid polymeric surrounding), leading to a higher drug diffusivity. More recently, Kapoor et al. (2022) conducted ex vivo penetration experiments in pig ear skin to examine the skin delivery of Tazarotene from novel FFS at various drug concentrations. The total amounts of drug permeated for the FFS containing 0.1% Tazarotene were subsequently compared with those for commercially available Tazorac® cream (0.1%). The FFS consisted of a lipophilic polyamide-3 polymer and ethanol, and 50 µL of formulation were applied to an area of 0.5 cm2 (diameter 8 mm) over 24 h. Horizontal sections (10 µm thickness) of the epidermis and dermis were sliced and the amounts of drug that penetrated to these sections were determined according to a previously reported model (Herbig et al., 2015). Overall, the FFS was found to promote ten times greater amounts of drug to the upper dermis compared to the cream formulation. The corresponding amounts of drug that penetrated to the epidermis were not reported by the authors; however, the superior performance of FFS with regards to the rate and extent of drug delivery was evident.
Drug loaded FFS have shown great potential for enhanced and sustained drug delivery into the skin. The increased residence time on the skin coupled with high mechanical resistance may contribute to the replacement of multiple daily applications of conventional semi-solid topical products with a single administration, thereby promoting improved patient compliance. In addition to enhanced drug delivery, the good wipe-off resistance and cosmetic attractiveness are features that may play a role in dose consistency for dermal drug administration. Although research on FFS has been going on for several years, only a handful of drugs are currently formulated in such delivery systems and are available for clinical use (Pünnel and Lunter, 2021). Clearly, the applications and capabilities of polymeric FFS are far from being exhausted.
3.2 Ionic liquids and deep eutectic solvent systems as penetration enhancers
3.2.1 Vehicle selection
The use of ionic liquids (ILs) and deep eutectic solvent systems (DESs) as excipients in dermatological drug delivery systems for permeation enhancement has received exceptional interest in recent years, especially with regard to transdermal transport of macro-molecules (Berton and Shamshina, 2021; Liu et al., 2022). ILs are defined as molten salts with low melting points, typically below 100°C, with many ILs being liquid at ambient temperature (room-temperature ILs (RT-ILs)) (Hayes et al., 2015). ILs are comprised solely by ions, namely a relatively large and asymmetric organic cation and an organic or inorganic anion, normally mixed in a 1:1 molar ratio. DESs are eutectic mixtures of two or more distinct components, hydrogen bond donors and acceptors, which when combined at a certain ratio experience deep melting point depression, typically below room temperature, due to strong hydrogen-bonding. DESs are often regarded as a class of ILs because they share many of the same genera characteristics, such as high thermal stability, low volatility and low vapor pressure (Wolbert et al., 2019; Hansen et al., 2020). Most importantly, the structure of ILs and DESs gives them the remarkable advantage of pharmaceutical tunability, i.e., their physicochemical and biological properties, including hydrophobicity and hydrogen-bonding ability, can be modified or chosen a priori by precise selection of the system constituents and their stoichiometric ratios (Tanner et al., 2019).
In an early study, Moniruzzaman et al., 2010b, Moniruzzaman et al., 2010c examined the topical delivery of acyclovir, a drug with low solubility in water, and commonly used organic solvents from various systems, including a novel IL-based microemulsion. Microemulsions are thermodynamically stable isotropic mixtures of at least three components, i.e., two immiscible solvents and a surfactant (Heuschkel et al., 2008). The presence of an imidazolium-based IL [C1mim][(MeO)2PO2] (dimethylimidazolium dimethyl phosphate), in the formulation was reported to significantly increase drug delivery to and through porcine skin ex vivo for the IL-in-oil (IL/o) micro-emulsions, compared with conventional water-in-oil (w/o) micro-emulsions of similar composition. The IL or water phase accounted for 4% (w/w), for both the IL/o and w/o preparations respectively. The IL/o micro-emulsion was additionally found to outperform a commercial acyclovir cream of the same drug concentration (5 mg/mL), in terms of promoting drug permeation across the skin. In a similar work, Islam et al. (2020) used choline-based ILs (choline formate, choline lactate, or choline propionate) to prepare various acyclovir-containing IL/o micro-emulsions. Additionally, IL/o formulations contained choline oleate, an IL with long hydrophobic tail (C18) with surfactant-like activity. This type of solvents are known as surface active ionic liquids (SAILs), and they have been reported to be able to enhance drug penetration through the skin (Ali et al., 2021, Ali et al., 2022). Overall, IL-based micro-emulsions were reported to result in a significantly increased penetration of acyclovir compared to either w/o micro-emulsions or neat formulation components across Yucatan minipig skin ex vivo. In a later study, the same research group used a similar experimental methodology to investigate the impact of different SAILs on transdermal delivery of insulin. In this study, Islam et al. (2021) prepared a series of insulin-containing IL/o micro-emulsions (1 mg/mL), coprised of choline propionate IL as a non-aqueous polar internal phase and isopropyl myristate (IPM) as a continuous oil phase. Different SAILs were added as surfactants, either choline stearate, choline oleate, or choline linoleate ([Chl][C18:0] [Chl][C18:1], and [Chl][C18:2], respectively) at 7.5 (w/w). Transdermal penetration of insulin at 48 h for the formulations containing the SAIL [Chl][C18:2] was found to be significantly higher than that of all other formulations examined. These researchers proposed that SAILs had a fluidization effect on the SC lipid bilayers, and they highlighted the significant role of these excipients on topical and transdermal delivery.
The penetration enhancing properties reported for several ILs and DESs were investigated in a mechanistic study by Qi et al. (2020). These researchers utilized attenuated-total-reflectance Fourier transform infrared (ATR-FTIR) technique to examine the influence of 31 neat ammonium-based ILs and DESs on the structure of isolated porcine SC after 24 h of incubation. The effects of these materials on the barrier were compared with those from 44 commonly used chemical penetration enhancers (CPEs). The impact of the tested solvents on SC lipids were assessed by the area change of -CH2 symmetric stretching peak (2,850 cm−1), while influences on the keratin secondary structure were determined by the peak area change of the α helix peak (-C=O; 1,650 cm−1). The degree of changes estimated in the lipids and protein structures were used to assess penetration enhancement efficacy and irritation potential, respectively. All solvents were subsequently ranked by an ‘enhancement performance score’, calculated based on their potency and safety. Most ILs/DESs were found to exert a disordering effect on the skin barrier, by both lipid fluidization and extraction, while a protein stabilization effect, indicating potential reduced irritation, was also reported. Overall, the ILs/DESs were reported to have a higher Performance Score (1.1) than the conventional chemical penetration enhancers (0.4). In the same work, the Hansen solubility parameter values of solvents were estimated and served as a measure of the affinity of the tested compounds with the skin membrane. Generally, a linear correlation between the saturation solubility and the solubility parameter values has been demonstrated in a variety of experimental studies for various compounds in neat or multiple-component vehicles (Lane et al., 2012; Iliopoulos et al., 2019, Iliopoulos et al., 2020a). A higher percentage of the total ILs/DDEs examined (26%) compared to the CPEs (4%) were found to have solubility parameter values similar to those of the skin, thereby indicating increased solubility and partitioning inside the membrane for these materials.
One of the top performers identified among the ILs/DESs was the choline and geranic acid (CAGE)-based system (Performance Score 2.6) (Qi et al., 2020). This material has recently attracted significant interest due to its reported extraordinary potential to promote transdermal delivery of large hydrophilic molecules, such as dextrans of various molecular weights up to 150 kDa, across pig skin ex vivo (Qi and Mitragotri, 2019). From a physicochemical point of view, the structure of this solvent has caused discussion among the research community in terms of its correct classification and nomenclature, since it contains characteristics to be both IL and DES: it comprises ionic species, cholinium and geranate, as well as neutral species of geranic acid (Banerjee et al., 2018; Rogers and Gurau, 2018). In an interesting study, Banerjee et al. (2017) reported that CAGE promoted percutaneous transport of insulin in pig skin ex vivo under infinite dose conditions (300 µL). These researchers utilized confocal microscopy to visualize the fluorescein isothiocyanate (FITC)-insulin distribution across the skin 24 h post-application of various vehicles. The vehicles used were CAGE 1:2, pure phosphate buffered saline (PBS), 50:50 (v/v) PBS:diethylene glycol monoethyl ether (DGME), and 50:50 (v/v) PBS:ethanol. The concentration of insulin in the solutions was 1 mg/mL. Confocal images showed that FITC–insulin penetration into the dermis layer was evident for the CAGE samples, but not for the other vehicles. Subsequent permeation studies showed FITC-insulin penetration into porcine skin ex vivo, with significant permeation into the dermis at 24 h (5.5 µg/cm2) and 48 h (17.2 µg/cm2). Transdermal insulin penetration was additionally confirmed in vivo, where topical CAGE-insulin treatment in rats was found to result in 40% drop of blood glucose levels at 4 h, which remained relatively sustained for 12 h. A later study of the same research group examined FITC-insulin permeation under similar experimental conditions, using a wider range of vehicles: four different ratios of CAGE constituents of, i.e., 1:1, 1:2, 1:4 and 2:1, as well as neat geranic acid, choline bicarbonate and PBS. Overall, the CAGE 1:2 vehicle was able to deliver insulin across porcine skin, while the CAGE 1:4 was also found to promote insulin penetration to a lesser extent (Tanner et al., 2018). Clearly, further experimental work is required before these systems can be used clinically, especially from a toxicity point of view. To date, little is known about possible safety considerations associated with topically applied ILs (Monti et al., 2017; Hwang et al., 2018; Navti et al., 2022). A comprehensive investigation on potential IL-induced dermal irritation, with a particular focus on diseased skin is essential; however, the ability of ILs and DESs excipients to promote delivery of macro-molecules by passive diffusion can revolutionize the field of skin delivery by creating a great array of new applications and medicinal treatments.
3.2.2 Manipulation of the active
Modification of the physicochemical properties of drug molecules as a strategy to favor their ability to partition and diffuse through the membranes is not a new concept. However, such modification typically comes at the cost of drug structure alterations (e.g., prodrug formations), which may involve multi-step synthesis and additional considerations associated with safety evaluation and efficacy testing (Ettmayer et al., 2004). A simple way to increase the aqueous solubility of active pharmaceutical ingredients (APIs) while maintaining the drug structure is by conversion to salts. When the salt contains both an organic cation and anion, the structure could possess a residual degree of hydrophobicity. Salts of this type have been proposed to behave as ion pairs and they can be transported through hydrophobic membranes (Valenta et al., 2000). In the last decades, ion-pairing has been examined as a strategy to modify the properties of an active, especially in respect to its solubility, and as a result enhance skin uptake. Over the years, several drugs paired with various counter ions have been reported to demonstrate enhanced skin permeability compared to the corresponding non-charged molecules Cristofoli et al., 2021, Cristofoli et al., 2022.
A more recent approach based on the ionic pairing strategy involves the conversion of a drug to IL (Active Pharmaceutical Ingredient (API)-IL system), by pairing an ionizable drug molecule with an appropriate room temperature (RT)-IL-forming counterion (Moshikur and Goto, 2021). The inherent tunability of API-IL systems enables the customization of hydrophilicity or hydrophobicity of drug molecules compared to their solid or crystalline forms, for optimal skin permeability. For example, poorly soluble drugs, such as acyclovir and sulfasalazine, have been shown to exhibit a 450- and 4,000- fold increase in aqueous solubility, respectively, when converted to a cholinium-based IL (Shadid et al., 2015; Shamshina et al., 2017). An example of API-IL formation that is more applicable to skin delivery was reported by Miwa et al. (2016). These researchers prepared an API-IL by mixing etodolac with lidocaine (1:1, mol/mol) and this transformation resulted in significant increases of the saturation solubility in water as well as in organic solvents (propylene glycol, diethyl sebacate and octanol) for both compounds. Additionally, the permeation of the API-IL across porcine skin ex vivo was investigated in comparison with the individual drug molecules. Transdermal patches containing either API-IL (4.4%), etodolac (2.4%) or lidocaine (2%) were prepared and applied to the skin for 24 h. Overall, the API-IL was reported to result in a 9.3-fold increase of etodolac permeation across the skin compared with the conventional etodolac patch. Interestingly, a decrease of lidocaine permeation was found for the API-IL patch, underlying the significance of the IL constituents for targeted penetration enhancement. More recently, Janus et al. (2020) prepared various ibuprofen ILs with L-valine alkyl esters (ValOR), where R ranged in the alkyl-chain length from an ethyl to a hexyl group, and examined their ability to cross porcine skin ex vivo. Franz-type diffusion studies were conducted, where the donor chamber was filled with the solution of a tested compound in ethanol 70% (v/v) at equal concentrations, 0.01 g/mL. The skin absorption of each derivative was compared with the permeation of ibuprofen free acid. Overall, all API-ILs resulted in greater percentages of applied ibuprofen that permeated across the skin, ranging from 7.3% to 13.6%, compared with ibuprofen free acid (6.1%). The L-valine propyl ([ValOPr][IBU]) was found to have the best performance among the API-IL systems. The significant potential of API-ILs in drug delivery has been proposed in the recent years (Shamshina et al., 2013, Shamshina et al., 2015; Moshikur and Goto, 2021). A rational design of API-ILs enables precise selection of drug physicochemical and biomedical properties for enhanced and targeted delivery. Additionally, the unique characteristics of API-IL systems can address the solubility and polymorphism challenges associated with solid or crystalline forms of drugs. Although thorough pharmacokinetic and pharmacodynamic investigations of API-ILs are still to be conducted, it is evident that this class of materials has a lot to offer in dermatological drug delivery in the future. Table 1 shows examples of various applications of IL-assisted dermatological drug formulation strategies.
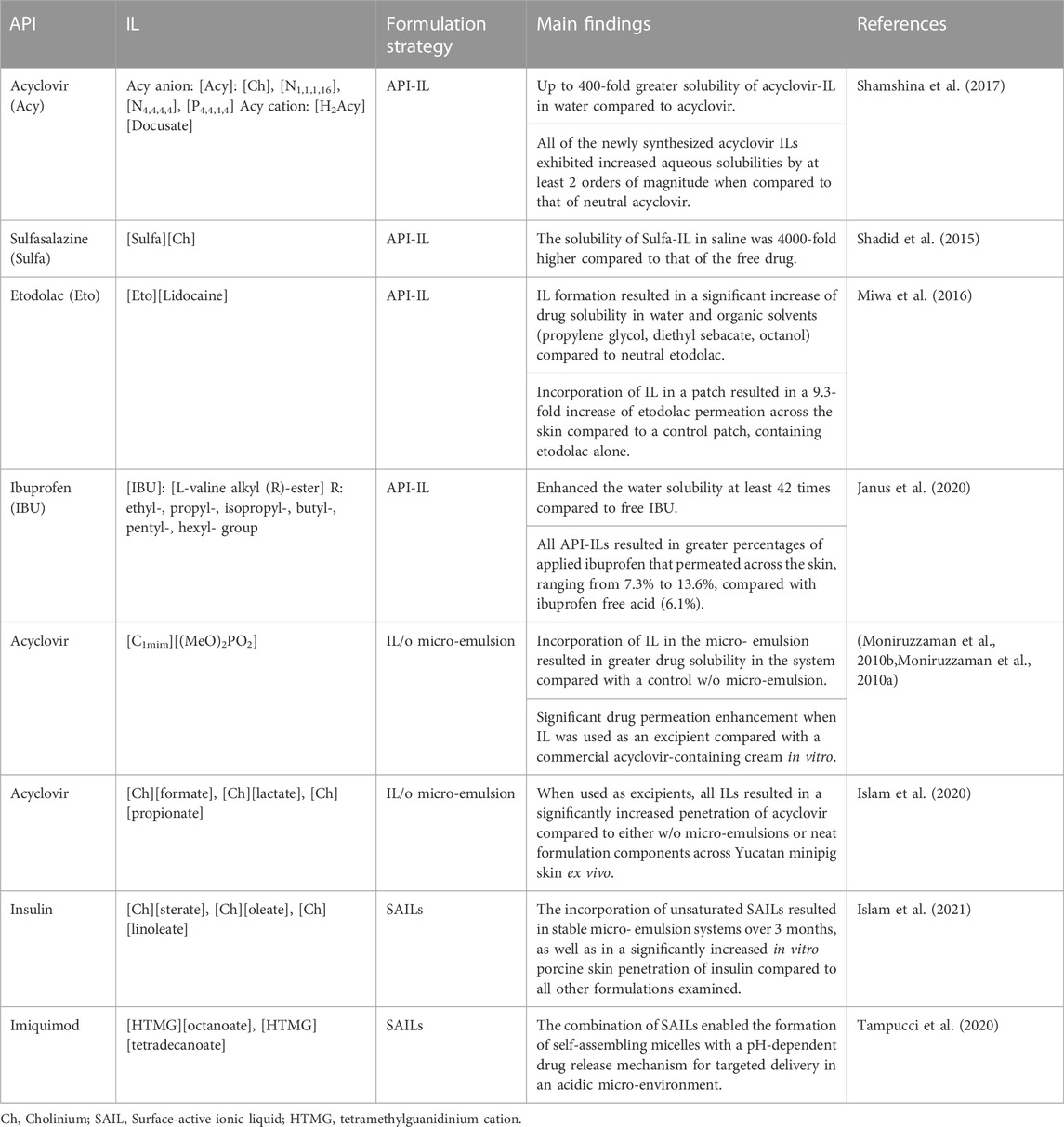
TABLE 1. IL-based formulation strategies for the development of topical and transdermal formulations.
4 Conclusions
The present review has highlighted the great potential for innovative uses of commonly used excipients, as well as the development of novel materials for efficient and sustained delivery of actives. Exciting research advances in the field promise new opportunities for dermatological drug product development. These advances in formulations will likely also be accompanied by increased use of more advanced chemical analysis techniques, including spectroscopy and microscopy methods, to evaluate both their compositional dynamics as well as interactions with skin. Complex formulations, especially FFS-, IL-and DES-based systems, not only undergo complex changes over time, but also impart major micro- and macro-structural changes within skin. These simultaneous dynamics must be adequately captured and studied to visualize and understand the behavior of these formulations to make future breakthroughs in topical drug delivery. Infrared (IR), Raman, coherent Raman, and atomic force microscopy (AFM) methods have the potential to play large roles in upcoming ex vivo and in vivo studies (Gre´goire et al., 2020; Pena et al., 2020). We envisage that in this incessant domain of pharmaceutical development, more emphasis will be given to the use of pharmaceutical excipients that originate from sustainable, eco-friendly and renewable sources. The continuous scientific progress allied to patient/customer needs will dictate how we foresee the development of new skin formulations which will not only enhance human dermatopharmacokinetics, but also comply with future green-manufacturing guidelines. Although some of the aforementioned new formulation approaches partly aim at tackling the current, and upcoming, world shortages, preliminary development which mainly focus on continual and viable resources will take uppermost importance in future research.
Author contributions
FI wrote the first draft of the manuscript. All authors contributed to manuscript revision, read, and approved the submitted version.
Conflict of interest
The laboratory of CLE has received sponsored research funding for pharmacokinetics research from LEO Pharma and Pfizer.
The remaining authors declare that the research was conducted in the absence of any commercial or financial relationships that could be construed as a potential conflict of interest.
Publisher’s note
All claims expressed in this article are solely those of the authors and do not necessarily represent those of their affiliated organizations, or those of the publisher, the editors and the reviewers. Any product that may be evaluated in this article, or claim that may be made by its manufacturer, is not guaranteed or endorsed by the publisher.
References
Ali, M. K., Moshikur, R. M., and Goto, M. (2021). Surface-active ionic liquids for medical and pharmaceutical applications. Singapore: Springer Singapore, 165–186. doi:10.1007/978-981-16-4365-1_9
Ali, M., Moshikur, R. M., Goto, M., Moniruzzaman, M., et al. (2022). Recent developments in ionic liquid-assisted topical and transdermal drug delivery. Pharm. Res. 39, 2335–2351. doi:10.41007/s11095-022-03322-x
Almeida, C., Filipe, P., Rosado, C., and Pereira-Leite, C. (2022). Nanodelivery strategies for skin diseases with barrier impairment: Focusing on ceramides and glucocorticoids. Nanomaterials 12, 275. doi:10.3390/nano12020275
Banerjee, A., Ibsen, K., Brown, T., Chen, R., Agatemor, C., and Mitragotri, S. (2018). Reply to rogers and gurau: Definitions of ionic liquids and deep eutectic solvents. Proc. Natl. Acad. Sci. U. S. A. 115, E11000. doi:10.1073/pnas.1815526115
Banerjee, A., Ibsen, K., Iwao, Y., Zakrewsky, M., and Mitragotri, S. (2017). Transdermal protein delivery using choline and geranate (CAGE) deep eutectic solvent. Adv. Healthc. Mat. 6, 1601411. doi:10.1002/adhm.201601411
Barbero, A. M., and Frasch, H. F. (2017). Effect of stratum corneum heterogeneity, anisotropy, asymmetry and follicular pathway on transdermal penetration. J. Control. Release 260, 234–246. doi:10.1016/j.jconrel.2017.05.034
Barbero, A. M., and Frasch, H. F. (2009). Pig and Guinea pig skin as surrogates for human in vitro penetration studies: A quantitative review. Toxicol. Vitro 23, 1–13. doi:10.1016/j.tiv.2008.10.008
Barry, B. (2001). Novel mechanisms and devices to enable successful transdermal drug delivery. Eur. J. Pharm. Sci. 14, 101–114. doi:10.1016/S0928-0987(01)00167-1
Benson, H. A., Grice, J. E., Mohammed, Y., Namjoshi, S., and Roberts, M. S. (2019). Topical and transdermal drug delivery: From simple potions to smart technologies. Curr. Drug Deliv. 16, 444–460. doi:10.2174/1567201816666190201143457
Berton, P., and Shamshina, J. L. (2021). “Ionic liquids for transdermal drug delivery: Choline geranate system as a case study,” in Application of ionic liquids in drug delivery. Editors M. Goto, and M. Moniruzzaman (Singapore: Springer Singapore), 35–50. doi:10.1007/978-981-16-4365-1_3
Bodde´, H., Kruithof, M., Brussee, J., and Koerten, H. (1989). Visualisation of normal and enhanced hgcl2 transport through human skin in vitro. Int. J. Pharm. 53, 13–24. doi:10.1016/0378-5173(89)90356-6
Bodde´, H., van den Brink, I., Koerten, H., and de Haan, F. (1991). Visualization of in vitro percutaneous penetration of mercuric chloride; transport through intercellular space versus cellular uptake through desmosomes. J. Control. Release 15, 227–236. doi:10.1016/0168-3659(91)90114-S
Boncheva, M. (2014). The physical chemistry of the stratum corneum lipids. Int. J. Cosmet. Sci. 36, 505–515. doi:10.1111/ics.12162
Coldman, M., Poulsen, B., and Higuchi, T. (1969). Enhancement of percutaneous absorption by the use of volatile: Nonvolatile systems as vehicles. J. Pharm. Sci. 58, 1098–1102. doi:10.1002/jps.2600580912
Cristofoli, M., Hadgraft, J., Lane, M. E., and Sil, B. C. (2022). A preliminary investigation into the use of amino acids as potential ion pairs for diclofenac transdermal delivery. Int. J. Pharm. 623, 121906. doi:10.1016/j.ijpharm.2022.121906
Cristofoli, M., Kung, C.-P., Hadgraft, J., Lane, M. E., and Sil, B. C. (2021). Ion pairs for transdermal and dermal drug delivery: A review. Pharmaceutics 13, 909. doi:10.3390/pharmaceutics13060909
Cross, S., and Roberts, M. (2004). Physical enhancement of transdermal drug application: Is delivery technology keeping up with pharmaceutical development? Curr. Drug Deliv. 1, 81–92. doi:10.2174/1567201043480045
Cui, M., Wiraja, C., Chew, S. W. T., and Xu, C. (2020). Nanodelivery systems for topical management of skin disorders. Mol. Pharm. 18, 491–505. doi:10.1021/acs.molpharmaceut.0c00154
Edwards, A., Qi, S., Liu, F., Brown, M., and McAuley, W. (2017). Rationalising polymer selection for supersaturated film forming systems produced by an aerosol spray for the transdermal delivery of methylphenidate. Eur. J. Pharm. Biopharm. 114, 164–174. doi:10.1016/j.ejpb.2017.01.013
Elias, P. M., and Friend, D. S. (1975). The permeability barrier in mammalian epidermis. J. Cell. Biol. 65, 180–191. doi:10.1083/jcb.65.1.180
Elias, P. M. (2012). Structure and function of the stratum corneum extracellular matrix. J. Invest. Dermatol. 132, 2131–2133. doi:10.1038/jid.2012.246
Ettmayer, P., Amidon, G. L., Clement, B., and Testa, B. (2004). Lessons learned from marketed and investigational prodrugs. J. Med. Chem. 47, 2393–2404. doi:10.1021/jm0303812
Farah, H., Brown, M., and McAuley, W. (2020). Enhanced follicular delivery of finasteride to human scalp skin using heat and chemical penetration enhancers. Pharm. Res. 37, 112. doi:10.1007/s11095-020-02822-y
Feschuk, A. M., Kashetsky, N., Chiang, C., Burli, A., Burdick, H., and Maibach, H. I. (2022). Regional variation in percutaneous absorption in in vitro human models: A systematic review. J. Toxicol. Environ. Health. B Crit. Rev. 25, 97–112. doi:10.1080/10937404.2022.2032517
Frederiksen, K., Guy, R. H., and Petersson, K. (2016). The potential of polymeric film-forming systems as sustained delivery platforms for topical drugs. Expert Opin. Drug Deliv. 13, 349–360. doi:10.1517/17425247.2016.1124412
Frum, Y., Bonner, M. C., Eccleston, G. M., and Meidan, V. M. (2007). The influence of drug partition coefficient on follicular penetration: In vitro human skin studies. Eur. J. Pharm. Sci. 30, 280–287. doi:10.1016/j.ejps.2006.11.014
Garvie-Cook, H., Frederiksen, K., Petersson, K., Guy, R. H., and Gordeev, S. N. (2015). Biophysical elucidation of the mechanism of enhanced drug release and topical delivery from polymeric film-forming systems. J. Control. Release 212, 103–112. doi:10.1016/j.jconrel.2015.06.015
Grams, Y. Y., Whitehead, L., Cornwell, P., and Bouwstra, J. A. (2004). Time and depth resolved visualisation of the diffusion of a lipophilic dye into the hair follicle of fresh unfixed human scalp skin. J. Control. Release 98, 367–378. doi:10.1016/j.jconrel.2004.05.010
Gre´goire, S., Luengo, G. S., Hallegot, P., Pena, A.-M., Chen, X., Bornschlo¨gl, T., et al. (2020). Imaging and quantifying drug delivery in skin–part 1: Autoradiography and mass spectrometry imaging. Adv. Drug Deliv. Rev. 153, 137–146. doi:10.1016/j.addr.2019.11.004
Hadgraft, J., and Lane, M. E. (2011). Skin: The ultimate interface. Phys. Chem. Chem. Phys. 13, 5215–5222. doi:10.1039/c0cp02943b
Hadgraft, J. (2004). Skin deep. Eur. J. Pharm. Biopharm. 58, 291–299. doi:10.1016/j.ejpb.2004.03.002
Hadgraft, J. (2001). Skin, the final frontier. Int. J. Pharm. 224, 1–18. doi:10.1016/S0378-5173(01)00731-1
Hansen, B. B., Spittle, S., Chen, B., Poe, D., Zhang, Y., Klein, J. M., et al. (2020). Deep eutectic solvents: A review of fundamentals and applications. Chem. Rev. 121, 1232–1285. doi:10.1021/acs.chemrev.0c00385
Hayes, R., Warr, G. G., and Atkin, R. (2015). Structure and nanostructure in ionic liquids. Chem. Rev. 115, 6357–6426. doi:10.1021/cr500411q
Herbig, M. E., Houdek, P., Gorissen, S., Zorn-Kruppa, M., Wladykowski, E., Volksdorf, T., et al. (2015). A custom tailored model to investigate skin penetration in porcine skin and its comparison with human skin. Eur. J. Pharm. Biopharm.Biological Barriers 95, 99–109. doi:10.1016/j.ejpb.2015.03.030
Heuschkel, S., Goebel, A., and Neubert, R. H. (2008). Microemulsions—Modern colloidal carrier for dermal and transdermal drug delivery. J. Pharm. Sci. 97, 603–631. doi:10.1002/jps.20995
Hopf, N., Champmartin, C., Schenk, L., Berthet, A., Chedik, L., Du Plessis, J., et al. (2020). Reflections on the oecd guidelines for in vitro skin absorption studies. Regul. Toxicol. Pharmacol. 117, 104752. doi:10.1016/j.yrtph.2020.104752
Huong, S. P., Bun, H., Fourneron, J.-D., Reynier, J.-P., and Andrieu, V. (2009). Use of various models for in vitro percutaneous absorption studies of ultraviolet filters. Skin. Res. Technol. 15, 253–261. doi:10.1111/j.1600-0846.2009.00368.x
Hwang, J., Park, H., Choi, D. W., Nam, K. T., and Lim, K.-M. (2018). Investigation of dermal toxicity of ionic liquids in monolayer-cultured skin cells and 3d reconstructed human skin models. Toxicol. Vitro 46, 194–202. doi:10.1016/j.tiv.2017.09.025
Iliopoulos, F., Hossain, A. S. M. M. A., Sil, B. C., Moore, D. J., Lucas, R. A., and Lane, M. E. (2020a). Topical delivery of 3-O-ethyl l-ascorbic acid from complex solvent systems. Sci. Pharm. 88, 19. doi:10.3390/scipharm88020019
Iliopoulos, F., Sil, B. C., Monjur Al Hossain, A., Moore, D. J., Lucas, R. A., and Lane, M. E. (2020b). Topical delivery of niacinamide: Influence of neat solvents. Int. J. Pharm. 579, 119137. doi:10.1016/j.ijpharm.2020.119137
Iliopoulos, F., Sil, B. C., Moore, D. J., Lucas, R. A., and Lane, M. E. (2019). 3-O-ethyl-l-ascorbic acid: Characterisation and investigation of single solvent systems for delivery to the skin. Int. J. Pharm. X 1, 100025. doi:10.1016/j.ijpx.2019.100025
Islam, M. R., Chowdhury, M. R., Wakabayashi, R., Kamiya, N., Moniruzzaman, M., and Goto, M. (2020). Ionic liquid-in-oil microemulsions prepared with biocompatible choline carboxylic acids for improving the transdermal delivery of a sparingly soluble drug. Pharmaceutics 12, 392. doi:10.3390/pharmaceutics12040392
Islam, M. R., Uddin, S., Chowdhury, M. R., Wakabayashi, R., Moniruzzaman, M., and Goto, M. (2021). Insulin transdermal delivery system for diabetes treatment using a biocompatible ionic liquid-based microemulsion. ACS Appl. Mat. Interfaces 13, 42461–42472. doi:10.1021/acsami.1c11533
Janus, E., Ossowicz, P., Klebeko, J., Nowak, A., Duchnik, W., Kucharski, L., et al. (2020). Enhancement of ibuprofen solubility and skin permeation by conjugation with l-valine alkyl esters. RSC Adv. 10, 7570–7584. doi:10.1039/D0RA00100G
Kapoor, K., Gra¨fe, N., and Herbig, M. E. (2022). Topical film-forming solid solutions for enhanced dermal delivery of the retinoid tazarotene. J. Pharm. Sci. 111, 2779–2787. doi:10.1016/j.xphs.2022.04.006
Kienzler, J.-L., Queille-Roussel, C., Mugglestone, C., Ortonne, J., and Larnier, C. (2007). Stratum corneum pharmacokinetics of the anti-fungal drug, terbinafine, in a novel topical formulation, for single-dose application in dermatophytoses. Curr. Med. Res. Opin. 23, 1293–1302. doi:10.1185/030079907X199664
Kushner, J., Kim, D., So, P. T., Blankschtein, D., and Langer, R. S. (2007). Dual-channel two-photon microscopy study of transdermal transport in skin treated with low-frequency ultrasound and a chemical enhancer. J. Invest. Dermatol. 127, 2832–2846. doi:10.1038/sj.jid.5700908
Lane, M. E., Hadgraft, J., Oliveira, G., Vieira, R., Mohammed, D., and Hirata, K. (2012). Rational formulation design. Int. J. Cosmet. Sci. 34, 496–501. doi:10.1111/j.1468-2494.2012.00747.x
Lane, M. E., Santos, P., Watkinson, A. C., and Hadgraft, J. (2011). “Passive skin permeation enhancement,” in Topical and transdermal drug delivery: Principles and practice. Editors H. A. E. Benson, and A. C. Watkinson (Hoboken, New Jersey: John Wiley & Sons), 23–42. doi:10.1002/9781118140505.ch2
Larran˜eta, E., McCrudden, M. T., Courtenay, A. J., and Donnelly, R. F. (2016). Microneedles: A new frontier in nanomedicine delivery. Pharm. Res. 33, 1055–1073. doi:10.1007/s11095-016-1885-5
Liu, C., Chen, B., Shi, W., Huang, W., and Qian, H. (2022). Ionic liquids for enhanced drug delivery: Recent progress and prevailing challenges. Mol. Pharm. 19, 1033–1046. doi:10.1021/acs.molpharmaceut.1c00960
Mangion, S. E., Sandiford, L., Mohammed, Y., Roberts, M. S., and Holmes, A. M. (2022). Multi-modal imaging to assess the follicular delivery of zinc pyrithione. Pharmaceutics 14, 1076. doi:10.3390/pharmaceutics14051076
Menon, G. K., Cleary, G. W., and Lane, M. E. (2012). The structure and function of the stratum corneum. Int. J. Pharm. 435, 3–9. doi:10.1016/j.ijpharm.2012.06.005
Michaels, A. S., Chandrasekaran, S. K., and Shaw, J. E. (1975). Drug permeation through human skin: Theory and in vitro experimental measurement. AIChE J. 21, 985–996. doi:10.1002/aic.690210522
Miwa, Y., Hamamoto, H., and Ishida, T. (2016). Lidocaine self-sacrificially improves the skin permeation of the acidic and poorly water-soluble drug etodolac via its transformation into an ionic liquid. Eur. J. Pharm. Biopharm. 102, 92–100. doi:10.1016/j.ejpb.2016.03.003
Moniruzzaman, M., Kamiya, N., and Goto, M. (2010a). Ionic liquid based microemulsion with pharmaceutically accepted components: Formulation and potential applications. J. Colloid Interface Sci. 352, 136–142. doi:10.1016/j.jcis.2010.08.035
Moniruzzaman, M., Tahara, Y., Tamura, M., Kamiya, N., and Goto, M. (2010b). Ionic liquid-assisted transdermal delivery of sparingly soluble drugs. Chem. Commun. 46, 1452–1454. doi:10.1039/B907462G
Moniruzzaman, M., Tamura, M., Tahara, Y., Kamiya, N., and Goto, M. (2010c). Ionic liquid-in-oil microemulsion as a potential carrier of sparingly soluble drug: Characterization and cytotoxicity evaluation. Int. J. Pharm. 400, 243–250. doi:10.1016/j.ijpharm.2010.08.034
Monti, D., Egiziano, E., Burgalassi, S., Chetoni, P., Chiappe, C., Sanzone, A., et al. (2017). Ionic liquids as potential enhancers for transdermal drug delivery. Int. J. Pharm. 516, 45–51. doi:10.1016/j.ijpharm.2016.11.020
Moshikur, R. M., and Goto, M. (2021). “Ionic liquids as active pharmaceutical ingredients (APIs),” in Application of ionic liquids in drug delivery. Editors M. Goto, and M. Moniruzzaman (Singapore: Springer Singapore), 13–33. doi:10.1007/978-981-16-4365-1_2
Navti, P. D., Pandey, A., Nikam, A. N., Padya, B. S., Kalthur, G., Koteshwara, K. B., et al. (2022). Ionic liquids assisted topical drug delivery for permeation enhancement: Formulation strategies, biomedical applications, and toxicological perspective. AAPS PharmSciTech 23, 161–221. doi:10.1208/s12249-022-02313-w
Neelissen, J. A., Arth, C., Wolff, M., Schrijvers, A. H., Junginger, H. E., and Bodde, H. E. (2000). Visualization of percutaneous 3H-estradiol and 3H-norethindrone acetate transport across human epidermis as a function of time. Acta Derm. Venereol. Suppl. 80, 36–43. doi:10.1080/000155500750042844
Otberg, N., Patzelt, A., Rasulev, U., Hagemeister, T., Linscheid, M., Sinkgraven, R., et al. (2008). The role of hair follicles in the percutaneous absorption of caffeine. Br. J. Clin. Pharmacol. 65, 488–492. doi:10.1111/j.1365-2125.2007.03065.x
Patzelt, A., and Lademann, J. (2020). Recent advances in follicular drug delivery of nanoparticles. Expert Opin. Drug Deliv. 17, 49–60. doi:10.1080/17425247.2020.1700226
Pena, A.-M., Chen, X., Pence, I. J., Bornschlo¨ gl, T., Jeong, S., Gre´goire, S., et al. (2020). Imaging and quantifying drug delivery in skin–part 2: Fluorescence and vibrational spectroscopic imaging methods. Adv. Drug Deliv. Rev. 153, 147–168. doi:10.1016/j.addr.2020.03.003
Prausnitz, M. R., Mitragotri, S., and Langer, R. (2004). Current status and future potential of transdermal drug delivery. Nat. Rev. Drug Discov. 3, 115–124. doi:10.1038/nrd1304
Proksch, E., Brandner, J. M., and Jensen, J.-M. (2008). The skin: An indispensable barrier. Exp. Dermatol. 17, 1063–1072. doi:10.1111/j.1600-0625.2008.00786.x
Prow, T. W., Grice, J. E., Lin, L. L., Faye, R., Butler, M., Becker, W., et al. (2011). Nanoparticles and microparticles for skin drug delivery. Adv. Drug Deliv. Rev.Nanodrug Part. Nanoformulations Drug Deliv. 63, 470–491. doi:10.1016/j.addr.2011.01.012
Pünnel, L. C., and Lunter, D. J. (2021). Film-forming systems for dermal drug delivery. Pharmaceutics 13, 932. doi:10.3390/pharmaceutics13070932
Qi, Q. M., Duffy, M., Curreri, A. M., Balkaran, J. P. R., Tanner, E. E. L., and Mitragotri, S. (2020). Comparison of ionic liquids and chemical permeation enhancers for transdermal drug delivery. Adv. Funct. Mat. 30, 2004257. doi:10.1002/adfm.202004257
Qi, Q. M., and Mitragotri, S. (2019). Mechanistic study of transdermal delivery of macromolecules assisted by ionic liquids. J. Control. Release 311-312, 162–169. doi:10.1016/j.jconrel.2019.08.029
Rahma, A., and Lane, M. E. (2022). Skin barrier function in infants: Update and outlook. Pharmaceutics 14, 433. doi:10.3390/pharmaceutics14020433
Reid, M. L., Jones, S. A., and Brown, M. B. (2009). Transient drug supersaturation kinetics of beclomethasone dipropionate in rapidly drying films. Int. J. Pharm. 371, 114–119. doi:10.1016/j.ijpharm.2008.12.025
Rogers, R. D., and Gurau, G. (2018). Is ”choline and geranate” an ionic liquid or deep eutectic solvent system? Proc. Natl. Acad. Sci. U. S. A. 115, E10999. doi:10.1073/pnas.1814976115
Scheuplein, R. J. (1967). Mechanism of percutaneous absorption: Ii. transient diffusion and the relative importance of various routes of skin penetration. J. Invest. Dermatol. 48, 79–88. doi:10.1038/jid.1967.11
Schmitt, T., and Neubert, R. H. (2018). State of the art in stratum corneum research: The biophysical properties of ceramides. Chem. Phys. Lipids 216, 91–103. doi:10.1016/j.chemphyslip.2018.09.017
Schmitt, T., and Neubert, R. H. (2020). State of the art in stratum corneum research. Part ii: Hypothetical stratum corneum lipid matrix models. Skin. Pharmacol. Physiol. 33, 213–230. doi:10.1159/000509019
Shadid, M., Gurau, G., Shamshina, J. L., Chuang, B.-C., Hailu, S., Guan, E., et al. (2015). Sulfasalazine in ionic liquid form with improved solubility and exposure. Med. Chem. Commun. 6, 1837–1841. doi:10.1039/C5MD00290G
Shamshina, J. L., Barber, P. S., and Rogers, R. D. (2013). Ionic liquids in drug delivery. Expert Opin. Drug Deliv. 10, 1367–1381. doi:10.1517/17425247.2013.808185
Shamshina, J. L., Cojocaru, O. A., Kelley, S. P., Bica, K., Wallace, S. P., Gurau, G., et al. (2017). Acyclovir as an ionic liquid cation or anion can improve aqueous solubility. Acs Omega 2, 3483–3493. doi:10.1021/acsomega.7b00554
Shamshina, J. L., Kelley, S. P., Gurau, G., and Rogers, R. D. (2015). Chemistry: Develop ionic liquid drugs. Nature 528, 188–189. doi:10.1038/528188a
Starr, N. J., Khan, M. H., Edney, M. K., Trindade, G. F., Kern, S., Pirkl, A., et al. (2022). Elucidating the molecular landscape of the stratum corneum. Proc. Natl. Acad. Sci. U. S. A. 119, e2114380119. doi:10.1073/pnas.2114380119
Talreja, P. S., Kasting, G. B., Kleene, N. K., Pickens, W. L., and Wang, T.-F. (2001). Visualization of the lipid barrier and measurement of lipid pathlength in human stratum corneum. AAPS PharmSci 3, E13–E56. doi:10.1208/ps030213
Tampucci, S., Guazzelli, L., Burgalassi, S., Carpi, S., Chetoni, P., Mezzetta, A., et al. (2020). ph-responsive nanostructures based on surface active fatty acid-protic ionic liquids for imiquimod delivery in skin cancer topical therapy. Pharmaceutics 12, 1078. doi:10.3390/pharmaceutics12111078
Tanner, E. E., Ibsen, K. N., and Mitragotri, S. (2018). Transdermal insulin delivery using choline-based ionic liquids (CAGE). J. Control. Release 286, 137–144. doi:10.1016/j.jconrel.2018.07.029
Tanner, E. E. L., Curreri, A. M., Balkaran, J. P. R., Selig-Wober, N. C., Yang, A. B., Kendig, C., et al. (2019). Design principles of ionic liquids for transdermal drug delivery. Adv. Mat. 31, 1901103. doi:10.1002/adma.201901103
Tapfumaneyi, P., Imran, M., Mohammed, Y. H., and Roberts, M. (2022). Recent advances and future prospective of topical and transdermal delivery systems. Front. Drug Deliv. 25. doi:10.3389/fddev.2022.957732
Valenta, C., Siman, U., Kratzel, M., and Hadgraft, J. (2000). The dermal delivery of lignocaine: Influence of ion pairing. Int. J. Pharm. 197, 77–85. doi:10.1016/S0378-5173(99)00453-6
van Smeden, J., Janssens, M., Gooris, G., and Bouwstra, J. (2014). The important role of stratum corneum lipids for the cutaneous barrier function. Biochim. Biophys. Acta 1841, 295–313. doi:10.1016/j.bbalip.2013.11.006
Wolbert, F., Brandenbusch, C., and Sadowski, G. (2019). Selecting excipients forming therapeutic deep eutectic systems—A mechanistic approach. Mol. Pharm. 16, 3091–3099. doi:10.1021/acs.molpharmaceut.9b00336
Keywords: transdermal, chemical penetration enhancer, formulation design, skin, topical, film-forming systems, deep eutectic systems, ionic liquids
Citation: Iliopoulos F, Sil BC and Evans CL (2022) The role of excipients in promoting topical and transdermal delivery: Current limitations and future perspectives. Front. Drug. Deliv. 2:1049848. doi: 10.3389/fddev.2022.1049848
Received: 21 September 2022; Accepted: 28 November 2022;
Published: 20 December 2022.
Edited by:
Tarl Wayne Prow, University of York, United KingdomReviewed by:
Silvia Tampucci, University of Pisa, ItalyWilliam McAuley, University of Hertfordshire, United Kingdom
Copyright © 2022 Iliopoulos, Sil and Evans. This is an open-access article distributed under the terms of the Creative Commons Attribution License (CC BY). The use, distribution or reproduction in other forums is permitted, provided the original author(s) and the copyright owner(s) are credited and that the original publication in this journal is cited, in accordance with accepted academic practice. No use, distribution or reproduction is permitted which does not comply with these terms.
*Correspondence: Fotis Iliopoulos, filiopoulos@mgh.harvard.edu; Conor L. Evans, evans.conor@mgh.harvard.edu