- 1Faculty of Pharmacy and Pharmaceutical Sciences, Josai University, Sakado, Japan
- 2Farnex Incorporated, Yokohama Joint Research Center, Yokohama, Japan
- 3Faculty of Pharmaceutical Sciences, Josai International University, Togane, Japan
Non-lamellar liquid crystal (NLLC) structures have gained increasing attention for the controlled release of entrapped drugs. In the present study, an in situ NLLC structure-forming depot formulation through contact with water was developed using a ternary mixture system of soya phosphatidyl choline (SPC), 1, 2-dioleoyl-sn-glycero-3-phosphoglycerol sodium salt (DOPG), and sorbitan trioleate (Span 85), and the long-term release of an entrapped model drug, leuprolide acetate (LA), was investigated using evaluation of in vitro release and in vivo blood concentration–time profiles. Polarized images and small angle X-ray scattering analysis were used to confirm the presence of NLLC structures by contacting the prepared formulation with water. In addition, LA release and blood concentration–time profiles were investigated using in vitro and in vivo experiments, respectively. In situ NLLC constructed formulations by contacting water were achieved using a ternary mixture of SPC, DOPG, and Span 85. In particular, negative curvature was increased with an increase in the amount of Span 85 in the formulation, and an Fd3m structure was obtained with a sustained release of LA. A maintained blood concentration of LA over 21 days was confirmed by subcutaneous (s.c.) administration of the formulation. No retained administered formulation at the injection site was confirmed 28 days after administration without any signs of irritation, inflammation, or other apparent toxicity confirmed by visual observation. This result may be helpful for the development of a lipid-based formulation of peptides and proteins with sustained drug release.
Introduction
Self-injectable formulation that enables extend-drug release such as depot formulation has been increasing attention because it can improve patients’ quality of life and reduces the risk of recurrence or deterioration of symptoms. In addition, it would be helpful to reduce the burden on healthcare professionals (Lim et al., 2022). Polymer-based depot formulations such as poly (lactic-co-glycolic acid), PLGA, has been already used, and various PLGA-based long-acting drug products have been approved by the U.S. Food and Drug Administration (FDA) for therapeutic delivery. However, PLGA-based formulations are difficult due to their complex manufacturing process, and most of these formulations exhibit initial burst release followed by slow and incomplete release (Lim et al., 2022).
Lipid formulations, such as liposomes and lipid nanoparticles, have gained increasing attention as vehicles for controlled drug delivery (Seo et al., 2023). Recently, non-lamellar liquid crystal (NLLC) technology has emerged as a novel formulation with sustained release properties (van ’t Hag et al., 2017). NLLCs composed of amphiphilic lipids can be classified into discontinuous cubic phases, such as primitive (Im3m), diamond (Pn3m), and gyroid phases (Ia3d), inversed hexagonal phase (H2), and discontinuous micellar cubic phase (Fd3m) based on their assembly shape (Muszynski et al., 2018). The critical packing parameter (CPP) is used to explain the shape of the amphiphiles, which determines the type of their construction form. The CPP is calculated by V/al, where V is the hydrophobic chain volume, a is the cross-sectional area of the hydrophilic head group, and l is the cross-sectional area of the hydrophobic chain length in the molecule. The drug release rate of the loaded drug is affected by the constructed structure of the NLLC. In particular, Fd3m, which has a closely packed inverse micelles structure, displays slower drug release compared with other NLLC structures. In situ systems that form NLLC structures by contacting water in body fluids may be a preferable formulation for drug delivery with microneedles and needle-free injectors.
Amphiphilic lipids form an NLLC structure, with glycerol monooleate and glycerol dioleate, mono- and di-oleic ester compounds with glycerol as a hydrophilic group having been used (Nielsen et al., 1998). Because these NLLC forming lipids have CPP >1, higher negative curvature of the membrane is induced by the addition, and many strategies have been reported for engineering structures that are a compositional mix of various lipids with different CPPs (Martiel et al., 2014). Changes in the constructed structure and diameter of aqueous channels in the structure are methods to control drug release.
In our previous study, a novel NLLC-forming lipid, mono-O-(5, 9, 13-trimethyl-4-tetradecenyl) glycerol ester, MGE, was used on contact with body fluids (Okada et al., 2021). The formulation consisting of MGE, 1, 2-dioleoyl-sn-glycero-3-phosphoglycerol sodium salt, DOPG, showed that the blood concentration of LA was maintained for 21 days or more after administration. However, a higher injection force was required for injection. As the injection force is a very important parameter for self-injectable formulations (Okada et al., 2021), the injection force should be considered when developing a self-injectable formulation.
Recently, non-ionic surfactants of sorbitan fatty acid esters, such as sorbitan monooleate, have been used as alternative lipids to fabricate NLLC structures (Baez-Santos et al., 2016). Sorbian fatty acid esters are cheap nonionic surfactants, commonly used as food emulsifiers, in cosmetic and pharmaceutical products. In particular, sorbitan trioleate (Span 85) has a large lipophilic moiety and is used as an excellent emulsifier. Liposomes composed of a phospholipid bilayer (CPP = 1) are traditionally used as drug delivery systems, and recently liposomal formulations have been utilized in a depot-injectable formulations (Ki et al., 2014; Rahnfeld and Luciani, 2020).
Controlled drug release by adding Span 85 to phospholipid-based formulations to form NLLCs by inducing higher negative curvature may be an effective procedure. Therefore, in the present study, phospholipid-based gel formulations that turn into an NLLC structures by contacting water in body fluids, including soya phosphatidyl choline (SPC), 1,2-dioleoyl-sn-glycero-3-phosphoglycerol sodium salt (DOPG), and Span 85 were prepared, and leuprolide acetate (LA) was entrapped as a model drug with a middle-sized molecular weight. In addition, injectability force was also investigated to show the usefulness of a self-injectable formulation.
2 Materials and methods
2.1 Materials
LA was purchased from Shin Nippon Yakugyo K.K. (Tokyo, Japan). SPC was purchased from Funakoshi Co., Ltd. (Tokyo, Japan). Span 85 was purchased from Kanto Chemical Co., Ltd. (Tokyo, Japan). DOPG was purchased from NOF Corporation (Tokyo, Japan). Other reagents and solvents were of special grade, and further purification was not conducted.
2.2 Animal experiments
Male Wistar rats (body weight 200 ± 20 g, 8 weeks old) were purchased from Sankyo Lab Service Co. Rats were kept in a room regulated at 25°C ± 2°C with a 12-h light/dark cycle (on, off time: 9:00–21:00). The rats were also allowed to consume water and feed ad libitum. Feed was purchased from MF Oriental Yeast Industry Co. Experimental animals were handled in accordance with the Josai University Experimental Animal Regulations after obtaining approval from the Josai University Ethics Committee (JU22005).
2.3 Preparation of formulations
Different proportions of SPC/DOPG/Span 85 were prepared at room temperature. The percentage compositions of the prepared formulations are shown in Table 1. The formulations were prepared by direct mixing of SPC/DOPG/Span 85. SPC was previously dissolved in 50 µL of ethanol The preparation procedure was as follows: SPC was completely dissolved in ethanol, then SPC/ethanol solution, Span 85, DOPG were weighed (ex. 50 μL of ethanol was added to 85 mg of SCP to obtain SPC/ethanol solution, and then 5 mg of DOPG and 10 mg of Span 85 were mixed with SPC/ethanol solution). LA was added at 3.75 mg per 100 µL of the obtaining lipid solution, and the mixed formulation was stirred for 1 h in a vial at room temperature. The obtained lipid solution containing LA was used for all evaluations in the present study. The phase behavior was confirmed using a polarized microscope.
2.4 Polarized microscope observation
A microscope (VHX-5000, Keyence Corp., Osaka, Japan) was used for observation of polarized images of the prepared formulation. The observation was performed 5 min after applying 10 µL of each prepared formulation onto a slide glass with a high-viscosity dispenser (M10, M&S Instruments Inc., Osaka, Japan), followed by dropping an equal volume of phosphate-buffered saline (PBS, pH 7.4) onto the formulation. Immediately after applying PBS, the samples were covered with a cover glass and then observed at room temperature.
2.5 Small angle X-ray scattering (SAXS) analysis
A small angle X-ray diffraction instrument (Nano-Viewer, Rigaku Co., Ltd., Akishima, Tokyo, Japan) was used to confirm the structure. Measurements were performed at 30 kV and 40 mA (CuK α radiation, λ = 1.5418 Å). The focal length of the camera was set to 700 mm. Preformulations were immersed in 3 mL of PBS for 4 h, and measurements were performed at Kanazawa University (Kanazawa, Ishikawa, Japan). The obtained pattern was analyzed as follows (Okada et al., 2020). Briefly, the obtained X-ray pattern was analyzed by a Rigaku NANO-Solver program. All operation was conducted by a qualified researcher at Kanazawa University (Kanazawa, Ishikawa, Japan). The crystalline interplanar spacing parameter, d, was determined using the Bragg equation.
2.6 In vitro LA release from the formulations
LA release experiments from the prepared formulations were conducted using the dialysis method. Each formulation (100 μL) was placed into dialysis tubing (Pur-A-LyzerTM Mini 12,000 dialysis kit 25, molecular cut-off 12,000, Sigma Aldrich, St. Louis, MO, United States). Each formulation (100 μL) was placed in a dialysis tube (Pur-A-LyzerTM Mini 12,000 dialysis kit 25, molecular cut-off 12,000, Sigma Aldrich, St. Louis, MO, United States). The dialysis tube loaded with fromulation was immersed in 20 mL of PBS in a centrifuge tube (25 mL, centrifuge tube Mini MINI-2362-025, AGC Technoglass Co., Ltd., Shizuoka, Japan). The release experiment was conducted over 7 days, and during the experiment the centrifuge tube was set in a water bath at 37°C ± 0.02°C. The solution outside the dialysis membrane, receiver solution, was stirred with a stir bar during the experiment. Periodically, 500 µL of PBS was sampled from the solution outside the dialysis membrane, receiver compartment, and the same amount of fresh PBS was added to the receiver compartment to maintain a constant volume. Before each sampling, the receiver solution was agitated using a pipet for 10 s. The cumulative percentage of LA released was calculated by the loaded LA in the formulation.
2.7 Evaluation of injectability force of the prepared formulation
Injectability force of the prepared formulation was tested by a texture analyzer equipped with a 10 kg load cell (EZ Test, Shimadzu Corporation, Kyoto, Japan). Compression model was used for the analysis with a 1 mL syringes connected with a 23G needle. The formulation was filled into the syringe and the test was conducted at a speed of 1 mm/s. The maximum force (N) was determined when loaded force showed a constant value over the time. All measurement were carried out in triplicate.
2.8 In vivo experiments
Rats were cannulated in the jugular vein 1 day before the experiment. The prepared formulation was injected subcutaneously through a 23G needle in the dorsal region. The injection site was shaved prior to injection. Blood samples (100 μL) were taken periodically through the cannula until 28 days after injection. The obtained blood samples were centrifuged (21,500 × g, 5 min, 4°C) to obtain plasma. Plasma samples were stored at −80°C until assayed. LA solution was dissolved in saline containing 5% dimethyl sulfoxide at a concentration of 37.5 mg/mL, and the prepared formulation was s.c. injected at a dose of 18.75 mg/kg. The area under the curve over 21 days after administration (AUC21days) was calculated using the trapezoidal method.
2.9 LA determination
The samples obtained from the in vitro release experiment were mixed with acetonitrile at a ratio of 1:1 (v/v) by vortexing for 5 min, and the resulting solution was used as the measured sample. Plasma samples obtained in the in vivo experiment were mixed with acetonitrile at a 1:1 (v/v) ratio by vortexing for 5 min and then centrifuged (21,500 × g, 5 min, 4°C). The upper layer of the resulting solution was used as the measurement sample.
A liquid chromatography–tandem mass spectrometry (LC-MS/MS) system was used for the measurement of LA detection. The system was consisted by a system controller (CBM- 20A; Shimadzu Corporation), pump (LC- 20 AD; Shimadzu Corporation), auto-sampler (SIL-20 AC; Shimadzu Corporation), column oven (CTO-20 AC; Shimadzu Corporation), detector (3,200 QTRAP; AB Sciex, Tokyo, Japan), and analysis software (Analyst® version 1.4.2; Shimadzu Corporation). The column and the guard column were Shodex® ODP2 HP-2B 2.0 mm × 50 mm and ODP2 HPG-2A 2.0 mm × 10 mm, respectively (Showa Denko, Tokyo, Japan). The column temperature was set to 40°C, and the flow rat of mobile phase [isocratic mode, a mixture of 0.1% formic acid in water and acetonitrile (7:3)] was set to 0.2 mL/min. LA ionization was conducted by electrospray ionization, and the measured molecular weight of LA was set to m/z 605.30 for the precursor ion and m/z 249.00 for the production. The ion spray voltage was 5,000 V, the nebulizer gas pressure was 80 psi, the drying gas flow rate was 10 L/min, and the drying gas temperature was 600°C. The lower limit of quantification of this assay was 1.0 ng/mL. As an internal standard of betamethasone valerate was used for the LA assay. A standard curve was prepared with spiked plasma for the detection of blood concentration and without spiked plasma for the detection of LA release with standard LA over the range of 1.0 to 1.0 × 105 ng/mL.
2.10 Statistical analysis
JMP® Pro software (ver. 16.0.0, SAS Institute Inc., Cary, NC, United States) was used for statistical analyses. One-way ANOVA and Tukey’s honestly significant difference post hoc analysis was used to determine statistical significance (p < 0.05). More than three replicates, except for SAXS analysis, were conducted and all data were expressed as mean with standard deviation.
3 Results
3.1 Polarized microscope observation results of prepared formulations
Polarized microscope observation was used for formulations consisting of SPC/DOPG/Span 85 at different proportions. Non-polarized images were observed for all formulations before contacting water (data not shown). Non-polarized images were obtained for formulations containing more than 50% Span 85, even after contacting water (F25:25:50 and F12.5:25:62.5). In addition, higher viscosity, which made it difficult to pass through a 23 G needle, was confirmed with increasing DOPG content (>50%) in the formulation (F25:50:25 and F12.5:75:12.5). Figure 1 shows a polarized image of the prepared formulations after swelling with water. All formulations for which polarization images obtained were injectable.
3.2 SAXS analysis
SAXS analysis was investigated for formulations that showed polarization images. Figure 2 shows the SAXS analysis results of the prepared formulations after contacting water. SAXS peaks were confirmed for all formulations. The spacing ratio of F85:5:10 was √2:2:√6:√8, whereas F70:5:25, F55:5:40, and F75:15:10 were 1:√3:2:√7, and the other formulations had 1:√3:2:√7. According to the diffraction patterns, the observed formulations had primitive cubic (Im3m), inverted hexagonal (H2), and reversed discontinuous micellar cubic (Fd3m) structures, respectively. The constructed NLLC structure changed from Im3m to H2 or Fd3m according to increases in DOPG or Span 85 contents in the formulations. Interplanar spacing calculated from the Bragg equation is shown in Table 2. A larger interplanar spacing was confirmed with F55:5:40, which had H2 structure, and the interplanar spacing was also changed by increasing Span 85 content in the formulation among the same structures.
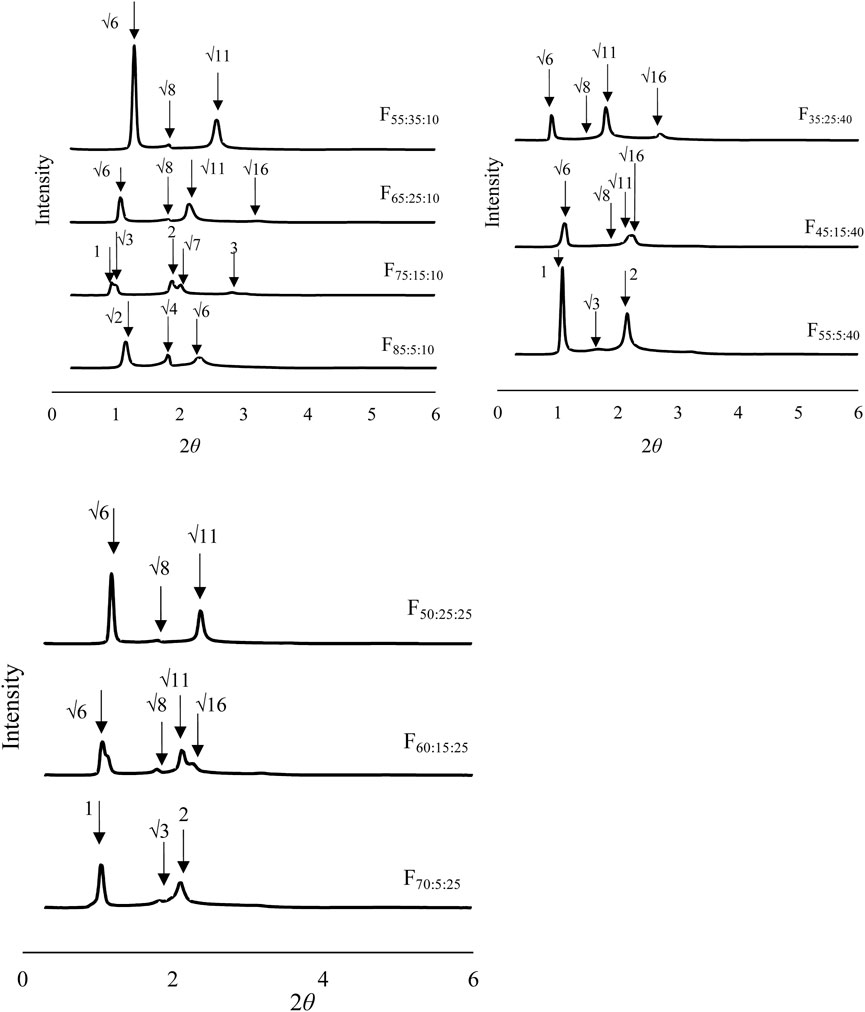
FIGURE 2. Structural analysis of the formulations after contacting water using small angle X-ray scattering analysis.
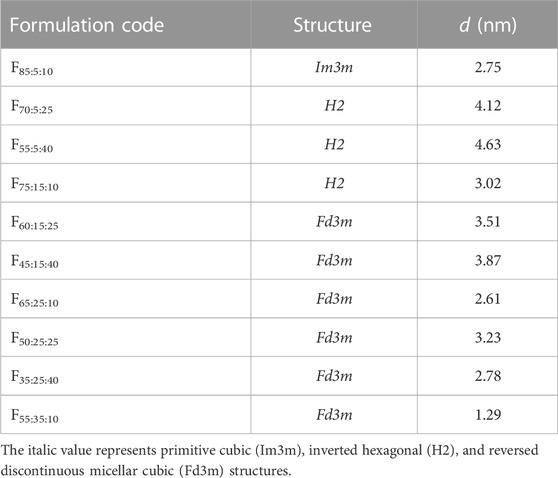
TABLE 2. Observed structures of formulations after swelling with water and interplanar spacing (d) calculated using the Bragg equation.
3.3 In vitro release experiment
Figure 3 shows the cumulative amount of LA released over 7 days, Q, from the prepared formulations. LA released from the formulations constructed of NLLC structures after contacting water was investigated. All formulations exhibited release behavior with more than 50% of LA remaining in the formulation after 7 days. Almost the same Q7d value was observed in formulations containing 10% Span 85, F85:5:10, F75:15:10, F65:25:10, and F55:35:10, independent of increasing DOPG or decreasing SPC contents in the formulations (Figure 3A). On the other hand, LA release was decreased with an increase in DOPG content at 40% Span 85; 5% DOPG content formulation, F55:5:40, had Q7d of 44%, 15% DOPG content formulation, F45:15:40, had Q7d of 33%, and 25% DOPG content formulation, F35:25:40, had Q7d of 23% (Figure 3B). With 25% Span 85 containing formulations, F70:5:25 showed a higher Q7d value compared with other formulations F60:15:25 and F50:25:25, and these F60:15:25 and F50:25:25 formulations showed almost the same Q7d value although they had different DOPG or decreasing SPC contents (Figure 3C).
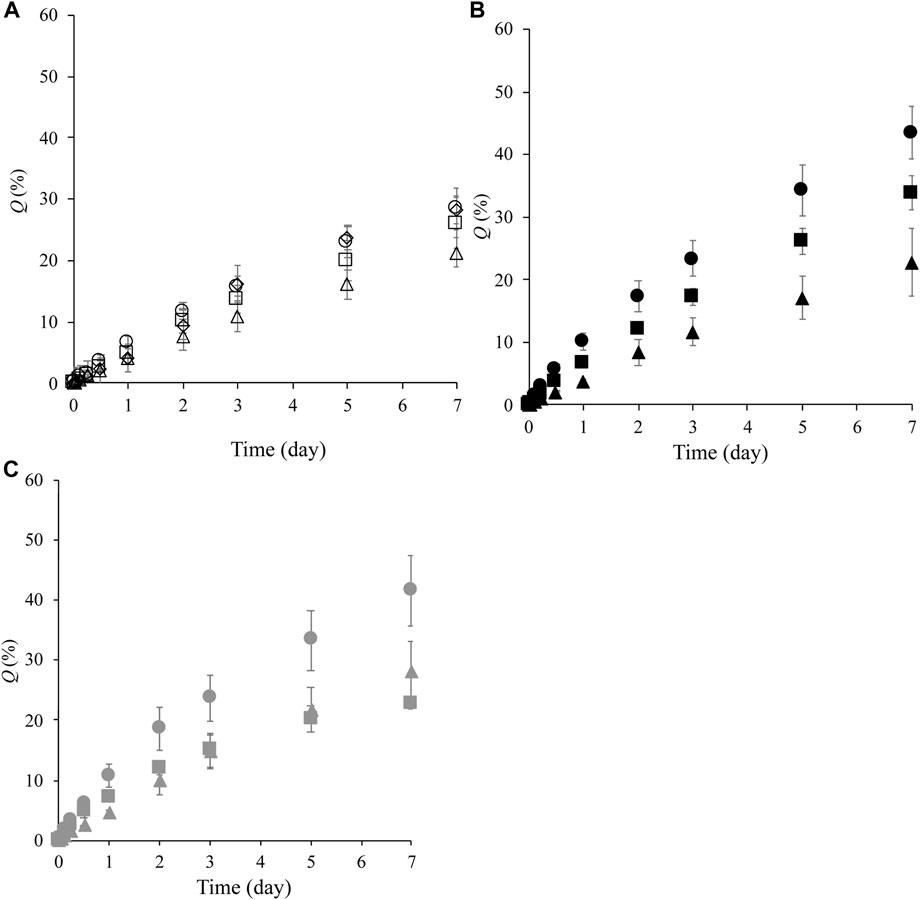
FIGURE 3. LA release profile from formulations over 7 days. (A): release profile from F85:5:10 (○), F75:15:10 ( ), F65:25:10 (△), and F55:35:10 (◇), (B): release profile from F55:5:40 (●), F45:15:40 (■), and F35:25:40 (▲), (C): release profile from F70:5:25 (●), F60:15:25 (■), and F50:25:25 (▲). Each value shows the mean ± S.D. (n = 3).
3.4 In vivo experiment
Figure 4 shows the blood concentration–time profile of LA after s.c. administration of the formulations F65:25:10, F55:35:10, F55:5:40, and LA solution. The formulations F65:25:10 and F55:35:10, which had the lowest and highest LA release levels, respectively, were selected for in vivo experiments. In addition, F55:5:40 was also selected to evaluate the effect of Span 85 content in the formation on the blood concentration–time profile was investigated compared with F55:35:10, although almost the same Q7d values between them were obtained in in vitro release experiments.
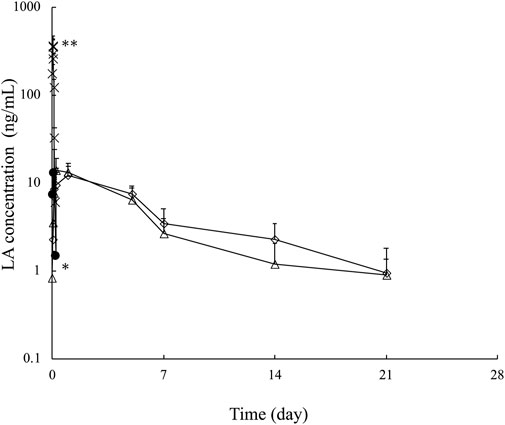
FIGURE 4. Plasma LA concentration–time profile over 21 days after subcutaneous injection of LA solution ( ), F65:25:10 (△), F55:35:10 (◇), and F55:5:40 (●). Each point shows the mean ± S.E. (n = 3–5). **p < 0.001 LA solution vs. the other formulations at 0.04 h, *p < 0.05 F55:5:40 vs. F65:25:10 and F55:35:10 at 1 day.
When LA solution was given by s.c. administration, a higher LA concentration was confirmed, although the concentration reached the lower limitation of detection (1 ng/mL) 8 h after administration. On the other hand, the tested formulations exhibited a maintained LA concentration compared with its solution. However, when F55:5:40 was given by s.c. administration, LA was detected until 24 h after administration for 21 days. The other formulations with F65:25:10 and F55:35:10 maintained LA concentrations. These formulations showed different release properties in in vitro release experiments; however, the same LA release profiles were confirmed after s.c. administration. Further LA detection 21 days after s.c. administration was not performed due to the lower limit of quantification of LA in blood. The calculated AUC21days was 0.61 ± 0.08 μg・h/mL for LA solution, 0.84 ± 0.12 μg•h/mL for F55:5:40, 1.47 ± 0.44 μg•h/mL for F65:25:10, and 2.2 ± 0.42 μg•h/mL for F55:35:10. Increased AUC21days was confirmed by F55:5:40, F65:25:10, and F55:35:10 compared with LA solution. However, the improved AUC21days effect was slight for F55:5:40 compared to F65:25:10 and F55:35:10.
3.5 Injectability force of the prepared formulation
Figure 5 shows the injectability force of F65:25:10, F55:35:10, and F55:5:40. Water was selected as a control. The average force of the prepared formulation was higher than control, and their average forces were 7.38 N, 7.37 N, and 7.07 N for F65:25:10, F55:35:10, and F55:5:40, respectively. A slightly decreased maximum force was observed in F55:5:40 compared with the other prepared formulations.
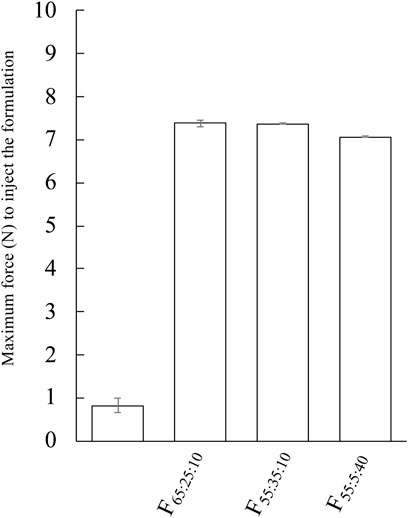
FIGURE 5. Evaluation of injectability force of F65:25:10, F55:35:10, and F55:5:40. The injectability force of the formulation was performed with 23G. Each value shows the mean ± S.D. (n = 3).
4 Discussion
Injectable depot formulations are of increasing interest because they offer a reduction in the number of required injections and maintain therapeutic efficacy by stabilizing blood concentrations. These advantages help in reducing unwanted side effects. In addition, depot formulations have been used for many different medical controls (antipsychotic, substance abuse, and hormonal therapy drugs) (Chaudhary et al., 2019). Polymers and lipids are the major excipients used in depot formulations. PLGA, a commonly used polymer in depot formulations, has different molecular weights and copolymer compositions, providing a wide range of drug release control. However, PLGA formulations are difficult due to their complex manufacturing process, and lipid-based formulations have gained attention instead of PLGA formulation for delivering drugs for long-term-period, in addition to PLGA formulation. Oil-based (Wilkinson et al., 2022) and nanodispersion-based (Altamura et al., 2003) formulations, such as liposomes and emulsions, have been used as lipid-based depot formulations (Lim et al., 2022). Recently, in situ lipid-based crystal-forming system have been assessed (Mei et al., 2018; Li et al., 2019; Pineda-Hernandez et al., 2020). NLLC structures can be formed spontaneously by contacting aqueous fluid, and it may be possible to control the drug release of entrapped drugs from the constructed structure. Drug release controlled by a constructed non-lamellar structure and its interplanar distance have been reported (Clogston and Caffrey, 2005). In addition, entrapped drug stability in the constructed non-lamellar structure has also been reported (Boge et al., 2016). However, the normal viscosity of the formulations is increased by forming an NLLC. When microneedle systems and non-needle-free injection systems as well as conventional needles were used as administration devices into the skin, formulations with a lower viscosity are preferable. Thus, in the present study, in situ crystal-forming systems with a simple composition were investigated with amphiphilic components to provide sustained release.
Many researchers have reported drug release from NLLC nanoparticles, and NLLCs can slowly release entrapped drugs. Drugs can diffuse in the lamella phase along a planner bilayer, whereas in a cubic phase, they can diffuse three-dimensionally. On the other hand, a hexagonal phase has one-dimensional water parallel with the water cylinder, and hydrophilic drugs exist in the polar core of the inversed micellar cube. Therefore, in general, the release rate of drugs exhibit the following order; diffusion in the lamellar structure (Dlamellar) > Dcubic phase > Dhexagonal phase > Dmicellar cubic (Muszynski et al., 2018). In the present study, higher Q7d values seen with F70:5:25 and F55:5:40, which exhibited H2 structures, were confirmed compared with formulations with an Fd3m structure (F60:15:25, F45:15:40, F65:25:10, F50:25:25, F35:25:40, and F55:35:10). Although this result corresponded with other reports, almost the same Q7d value was confirmed in even H2 constructing formulations such as F75:15:10 compared with Fd3m constructing formulations. Electrostatic interactions between the charged drug and the polar group in the lipid component may also achieve drug release control (Lynch et al., 2003; Negrini et al., 2014). Therefore, in the present study, formulations containing higher DOPG were listed (Table 1), but no experiments were performed with those with more than 50% DOPG because of their high viscosity.
The F75:15:10 formulation had a higher proportion of DOPG, which has a positive charge at pH 7.4, compared with F70:5:25 and F55:5:40. Thus, negatively charged LA was thought to have a slower release rate. In addition, the F85:5:10 formulation showed a relatively lower release rate compared with the other formulations. The release rate depended on the constructed structure in addition to the size of the aqueous channel (Muszynski et al., 2018; Zhai et al., 2022) because, in the case of hydrophilic drugs, they must diffuse through an aqueous channel and cross the lipid membrane. Therefore, a smaller aqueous channel in F85:5:10 may be the reason for the slower LA release rate.
Generally, when the rate-limiting step for drug absorption with aqueous solubility is the rate of drug release; the release rate can be considered to be related to the blood concentration–time profile. However, no blood concentration differences were confirmed between F55:35:10 and F65:25:10, although these have different release profiles of LA. On the other hand, the blood concentration of LA after s.c. administration of F55:5:40, which had a faster LA release rate, displayed the lower limit of detection within 8 h. Figure 5 shows the observation results of the remaining formulation in the body 21 days after administration. The observation was performed by surgically incising around the administration site. Phospholipids and Span 85 were degraded by lipases and non-enzymatic hydrolytic processes (He et al., 2023). Biodegradation of the formulation causes erosion, and subsequent formulation reduction until sufficient excretion is reached. Therefore, the biodegradation process may also affect the drug release rate from the formulation, especially in formulations with sustained drug release. In the present study, retention of the formulation was confirmed 7 days and 28 days after administration. When the F55:5:40 formulation was administered, no retained formulation was observed (Figure 6A). On the other hand, the F55:35:10 and F65:25:10 formulations were observed 7 days after administration (Figures 6B, C, respectively), but these formulations were not clearly observed 28 days after administration (Figures 6D, E, respectively). Since blood concentration of LA was not detected 21 days after administration, only formulation excipients would remain at the injection site. No signs of irritation, inflammation, or any other apparent toxicity were observed grossly at the injection site. In addition, body weight loss was not observed in the present study 28 days after administration compared with just before administration.
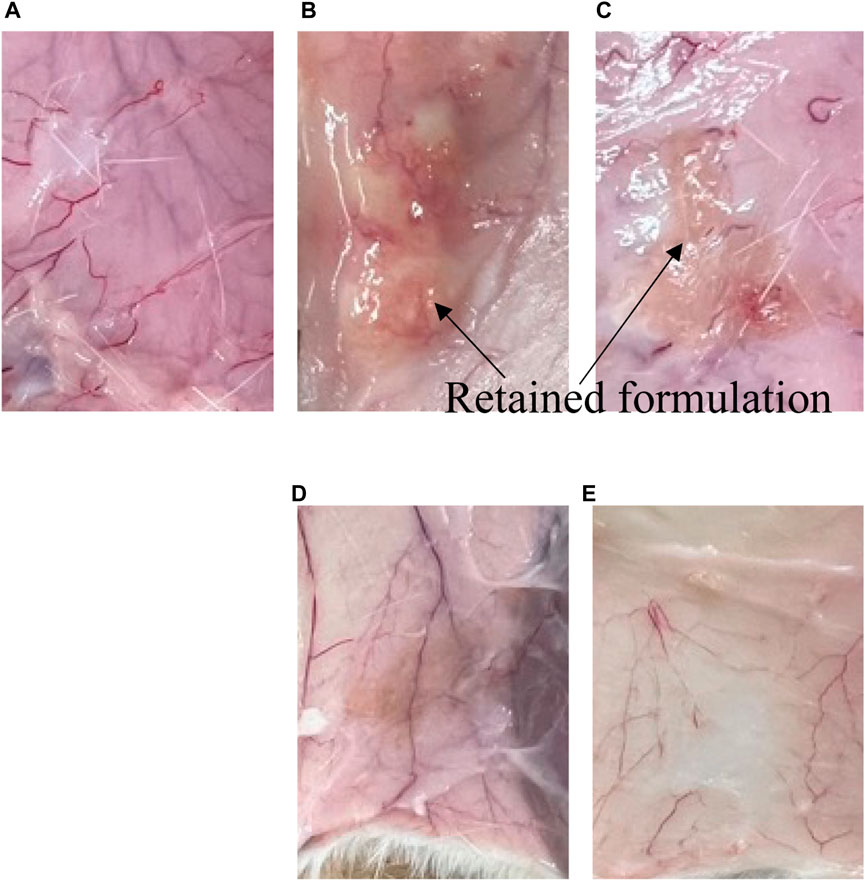
FIGURE 6. Remaining formulation at the injection site at 7 and 28 days after s.c. administration. (A–C) show 7 days after administration of (A) F55:5:40, (B) F55:35:10, and (C) F65:25:10, respectively. (D,E) show 28 days after administration of F55:35:10 and F65:25:10, respectively.
Controlled drug release may be attributed to the time required for water penetration into the core of the administrated formulation. In in vitro release conditions, drug release can be achieved without enzymatic degradation. In addition, in in vivo conditions, the transition time to construct an NLLC structure might be delayed in the body because of limited interstitial fluid volume. When the F55:5:40 formulation was administered, the absence of retention was confirmed 7 days after administration. On the other hand, the F55:35:10 and F65:25:10 formulations, which showed almost the same Q7d value, had a similar blood concentration–time profile. Therefore, it might be possible that the spread of components of the NLLC formulation at the administration site, which affects the construction of the NLLC structure, may be involved as a factor. This might be related to the lack of significant differences in blood concentration–time profiles between the F55:35:10 and F65:25:10 formulations.
When F55:35:10 and F65:25:10 formulations were administered, AUC21days values were 2.2 ± 0.42 μg•h/mL and 1.47 ± 0.44 μg•h/mL, respectively. Compared with the AUC21days after s.c. administration of LA solution, increased AUC21days was confirmed in both formulations. Ki et al. (2014) have reported AUC value after s.c. administration of a commercial product of PLGA formulation. The calculated AUC was 3.7 μg•h/mL when the dose of 12.5 mg/kg was administered to rats (approximately 300 g body weight). As these AUC values were corrected for administrated LA dosage, the commercial product of PLGA formulation showed about 3 times higher AUC/dose value compared with that of F55:35:10. In the present study, Span 85 mixed with phospholipids was used to provide sustained release of LA by constructing NLLC formulations. Furthermore, the average maximum force requirement of these formulations was less than 10 N when 29G needle was used. Patki et al. (2021) reported on self-injection formulations with sustained release and concluded that formulations with an average maximum force of 10 N or less are very easy to inject. F55:35:10 and F65:25:10 showed that around 7 N when the formulation was injected with 29G needle. In our previous report with NLLC forming lipid of MGE, which has CPP>1 (Okada et al., 2021), a maintained the blood concentration of LA over 21 days or more after administration. However, there was a problem for injectability because they needed a higher expulsion force (higher than 10 N, when the formulation was tested according to the experiment method in 2.7). On the other hand, in the present study, phospholipid formulation showed a maintained concentration of LA until 21 days with a good injectability. Molecular interaction is generally related to viscosity (Pichot et al., 2013), so the difference in injectability properties of the prepared formulation would be related to additives and components in the formulation.
Examination of tail chain length on the effect of the constructed NLLC structure revealed that an increase in CPP value induced phase transition from lamellar or cubic to reverse hexagonal structures (van ’t Hag et al., 2017; Ruela et al., 2016). Because Span 85 has a large lipophilic moiety similar to medium- and long-chain triacylglycerol, thus phase transition to increasing negative interfacial curvature may occur by adding it to a formulation. However, AUC/dose value in our prepared formulation was lower than that in the commercial product of PLGA formulation, suggesting that further formulation development to improve bioavailability should be necessary.
5 Conclusion
In the present study, in situ NLLC constructed formulations by contacting water were developed using a ternary mixture of SPC, DOPG, and Span 85. In particular, the negative curvature was increased with an increase in the amount of Span 85 in the formulation, and an Fd3m structure was obtained with a sustained release of LA. A maintained blood concentration of LA over 21 day was confirmed by s.c. administration of the formulation. However, the elimination of components of the formulation by enzymatic degradation might need to be considered together with in vitro release experiments for the evaluation of depot formulations with a sustained release. In the present study, evaluation of systemic and local toxicity after s.c. administration was not performed.
This lipid-based formulation can be prepared as a depot formulation with sustained release of the encapsulated drug more simply than polymer-based formulations. Further investigations, such as the detailed mechanism of self-assembly of the NLLC structure by analyzing lipid-lipid and lipid-drug interactions, as well as rheological studies to enable injection, will be useful in the selection of lipid compositions capable of forming the NLLC structures in situ. Although bioavailability equivalent to commercial PLGA products would be required to indicate the usefulness of the phospholipid and Span 85-based formulation, these investigations will be helpful for developing injectable depot formulations with controllable release of drugs such as peptides and proteins.
Data availability statement
The raw data supporting the conclusion of this article will be made available by the authors, without undue reservation.
Ethics statement
The animal study was approved by Josai University Experimental Animal Regulations after obtaining approval from the Josai University Ethics Committee. The study was conducted in accordance with the local legislation and institutional requirements.
Author contributions
RN: Formal Analysis, Investigation, Writing–original draft. AO: Data curation, Formal Analysis, Methodology, Writing–original draft. HT: Project administration, Supervision, Writing–original draft, Writing–review and editing. IN: Data curation, Formal Analysis, Methodology, Writing–original draft. KI: Data curation, Formal Analysis, Methodology, Writing–original draft. SI: Formal Analysis, Project administration, Validation, Writing–original draft. IH: Conceptualization, Data curation, Writing–review and editing. KS: Conceptualization, Data curation, Writing–review and editing.
Funding
The author(s) declare that no financial support was received for the research, authorship, and/or publication of this article.
Conflict of interest
Author IH was employed by Farnex Inc.
The remaining authors declare that the research was conducted in the absence of any commercial or financial relationships that could be construed as a potential conflict of interest.
Publisher’s note
All claims expressed in this article are solely those of the authors and do not necessarily represent those of their affiliated organizations, or those of the publisher, the editors and the reviewers. Any product that may be evaluated in this article, or claim that may be made by its manufacturer, is not guaranteed or endorsed by the publisher.
References
Altamura, A. C., Sassella, F., Santini, A., Montresor, C., Fumagalli, S., and Mundo, E. (2003). Intramuscular preparations of antipsychotics: uses and relevance in clinical practice. Drugs 63, 493–512. doi:10.2165/00003495-200363050-00004
Baez-Santos, Y. M., Otte, A., Mun, E. A., Soh, B. K., Song, C. G., Lee, Y. N., et al. (2016). Formulation and characterization of a liquid crystalline hexagonal mesophase region of phosphatidylcholine, sorbitan monooleate, and tocopherol acetate for sustained delivery of leuprolide acetate. Int. J. Pharm. 514, 314–321. doi:10.1016/j.ijpharm.2016.06.138
Boge, L., Bysell, H., Ringstad, L., Wennman, D., Umerska, A., Cassisa, V., et al. (2016). Lipid-based liquid crystals as carriers for antimicrobial peptides: phase behavior and antimicrobial effect. Langmuir 32, 4217–4228. doi:10.1021/acs.langmuir.6b00338
Chaudhary, K., Patel, M. M., and Mehta, P. J. (2019). Long-acting injectables: current perspectives and future promise. Crit. Rev. Ther. Drug Carr. Syst. 36, 137–181. doi:10.1615/CritRevTherDrugCarrierSyst.2018025649
Clogston, J., and Caffrey, M. (2005). Controlling release from the lipidic cubic phase. Amino acids, peptides, proteins and nucleic acids. J. Control Release 107, 97–111. doi:10.1016/j.jconrel.2005.05.015
He, Q., Chen, Y., Wang, Z., He, H., and Yu, P. (2023). Cellular uptake, metabolism and sensing of long-chain fatty acids. Front. Biosci. (Landmark Ed. 28, 10. doi:10.31083/j.fbl2801010
Ki, M. H., Lim, J. L., Ko, J. Y., Park, S. H., Kim, J. E., Cho, H. J., et al. (2014). A new injectable liquid crystal system for one month delivery of leuprolide. J. Control Release 185, 62–70. doi:10.1016/j.jconrel.2014.04.034
Li, Y., Angelova, A., Liu, J., Garamus, V. M., Li, N., Drechsler, M., et al. (2019). In situ phase transition of microemulsions for parenteral injection yielding lyotropic liquid crystalline carriers of the antitumor drug bufalin. Colloids Surf. B Biointerfaces 173, 217–225. doi:10.1016/j.colsurfb.2018.09.023
Lim, Y. W., Tan, W. S., Ho, K. L., Mariatulqabtiah, A. R., Abu Kasim, N. H., Abd Rahman, N., et al. (2022). Challenges and complications of poly(lactic-co-glycolic acid)-based long-acting drug product development. Pharmaceutics 14, 614. doi:10.3390/pharmaceutics14030614
Lynch, M. L., Ofori-Boateng, A., Hippe, A., Kochvar, K., and Spicer, P. T. (2003). Enhanced loading of water-soluble actives into bicontinuous cubic phase liquid crystals using cationic surfactants. J. Colloid Interface Sci. 260, 404–413. doi:10.1016/s0021-9797(02)00016-4
Martiel, I., Sagalowicz, L., and Mezzenga, R. (2014). Phospholipid-based nonlamellar mesophases for delivery systems: bridging the gap between empirical and rational design. Adv. Colloid Interface Sci. 209, 127–143. doi:10.1016/j.cis.2014.03.005
Mei, L., Xie, Y., Huang, Y., Wang, B., Chen, J., Quan, G., et al. (2018). Injectable in situ forming gel based on lyotropic liquid crystal for persistent postoperative analgesia. Acta Biomater. 67, 99–110. doi:10.1016/j.actbio.2017.11.057
Muszynski, A., Zaleska-Radziwill, M., and Doskocz, N. (2018). Factors affecting accumulibacter population structure in full- and laboratory-scale biological reactors with nutrients removal. Water Sci. Technol. 77, 2794–2802. doi:10.2166/wst.2018.267
Negrini, R., Sanchez-Ferrer, A., and Mezzenga, R. (2014). Influence of electrostatic interactions on the release of charged molecules from lipid cubic phases. Langmuir 30, 4280–4288. doi:10.1021/la5008439
Nielsen, L. S., Schubert, L., and Hansen, J. (1998). Bioadhesive drug delivery systems. I. Characterisation of mucoadhesive properties of systems based on glyceryl mono-oleate and glyceryl monolinoleate. Eur. J. Pharm. Sci. 6, 231–239. doi:10.1016/s0928-0987(97)10004-5
Okada, A., Todo, H., Hijikuro, I., Itakura, S., and Sugibayashi, K. (2020). Controlled release of a model hydrophilic high molecular weight compound from injectable non-lamellar liquid crystal formulations containing different types of phospholipids. Int. J. Pharm. 577, 118944. doi:10.1016/j.ijpharm.2019.118944
Okada, A., Todo, H., Itakura, S., Hijikuro, I., and Sugibayashi, K. (2021). A lipid-based depot formulation with a novel non-lamellar liquid crystal forming lipid. Pharm. Res. 38, 503–513. doi:10.1007/s11095-021-03014-y
Patki, M., Palekar, S., Reznik, S., and Patel, K. (2021). Self-injectable extended release formulation of remdesivir (SelfExRem): A potential formulation alternative for covid-19 treatment. Int. J. Pharm. 597, 120329. doi:10.1016/j.ijpharm.2021.120329
Pichot, R., Watson, R. L., and Norton, I. T. (2013). Phospholipids at the interface: current trends and challenges. Int. J. Mol. Sci. 14, 11767–11794. doi:10.3390/ijms140611767
Pineda-Hernandez, M. T., Perez-Urizar, J. T., and Ganem-Rondero, A. (2020). Thermo-reversible in situ forming implant with nanostructured lipid carriers (NLC) as a delivery system for the administration of estradiol valerate. Drug Deliv. Transl. Res. 10, 1393–1402. doi:10.1007/s13346-019-00704-4
Rahnfeld, L., and Luciani, P. (2020). Injectable lipid-based depot formulations: where do we stand? Pharmaceutics 12, 567. doi:10.3390/pharmaceutics12060567
Ruela, A. L., Carvalho, F. C., and Pereira, G. R. (2016). Exploring the phase behavior of monoolein/oleic acid/water systems for enhanced donezepil administration for alzheimer disease treatment. J. Pharm. Sci. 105, 71–77. doi:10.1016/j.xphs.2015.10.016
Seo, Y., Lim, H., Park, H., Yu, J., An, J., Yoo, H. Y., et al. (2023). Recent progress of lipid nanoparticles-based lipophilic drug delivery: focus on surface modifications. Pharmaceutics 15, 772. doi:10.3390/pharmaceutics15030772
van 't Hag, L., Gras, S. L., Conn, C. E., and Drummond, C. J. (2017). Lyotropic liquid crystal engineering moving beyond binary compositional space - ordered nanostructured amphiphile self-assembly materials by design. Chem. Soc. Rev. 46, 2705–2731. doi:10.1039/c6cs00663a
Wilkinson, J., Ajulo, D., Tamburrini, V., Gall, G. L., Kimpe, K., Holm, R., et al. (2022). Lipid based intramuscular long-acting injectables: current state of the art. Eur. J. Pharm. Sci. 178, 106253. doi:10.1016/j.ejps.2022.106253
Keywords: lipid-based depot formulation, in situ forming system, sustained release, non-lamellar liquid crystal, long-acting drug delivery system
Citation: Todo H, Niki R, Okada A, Narita I, Inamura K, Ito A, Itakura S, Hijikuro I and Sugibayashi K (2023) Development of a depot formulation with an in situ non-lamellar liquid crystal-forming system with phospholipids. Front. Drug Deliv. 3:1270584. doi: 10.3389/fddev.2023.1270584
Received: 01 August 2023; Accepted: 22 September 2023;
Published: 19 October 2023.
Edited by:
Dolores R. Serrano Lopez, Complutense University of Madrid, SpainReviewed by:
Jyothsna Manikkath, Manipal University, IndiaAna Isabel Fraguas, Complutense University, Spain
Copyright © 2023 Todo, Niki, Okada, Narita, Inamura, Ito, Itakura, Hijikuro and Sugibayashi. This is an open-access article distributed under the terms of the Creative Commons Attribution License (CC BY). The use, distribution or reproduction in other forums is permitted, provided the original author(s) and the copyright owner(s) are credited and that the original publication in this journal is cited, in accordance with accepted academic practice. No use, distribution or reproduction is permitted which does not comply with these terms.
*Correspondence: Hiroaki Todo, aHQtdG9kb0Bqb3NhaS5hYy5qcA==