- Translational Stroke Research Laboratory, Faculty of Health and Medicine, School of Biomedical Sciences and Pharmacy, Hunter Medical Research Institute and The University of Newcastle, Callaghan, NSW, Australia
Stroke is predominantly a senescent disease, yet most preclinical studies investigate treatment in young animals. We recently demonstrated that short-duration hypothermia-treatment completely prevented the dramatic intracranial pressure (ICP) rise seen post-stroke in young rats. Here, our aim was to investigate whether a similar ICP rise occurs in aged rats and to determine whether short-duration hypothermia is an effective treatment in aged animals. Experimental middle cerebral artery occlusion (MCAo-3 h occlusion) was performed on male Wistar rats aged 19–20 months. At 1 h after stroke-onset, rats were randomized to 2.5 h hypothermia-treatment (32.5°C) or normothermia (37°C). ICP was monitored at baseline, for 3.5 h post-occlusion, and at 24 h post-stroke. Infarct and edema volumes were calculated from histology. Baseline pre-stroke ICP was 11.2 ± 3.3 mmHg across all animals. Twenty-four hours post-stroke, ICP was significantly higher in normothermic animals compared to hypothermia-treated animals (27.4 ± 18.2 mmHg vs. 8.0 ± 5.0 mmHg, p = 0.03). Infarct and edema volumes were not significantly different between groups. These data demonstrate ICP may also increase 24 h post-stroke in aged rats, and that short-duration hypothermia treatment has a profound and sustained preventative effect. These findings may have important implications for the use of hypothermia in clinical trials of aged stroke patients.
Introduction
Age is the most important independent non-modifiable risk factor for ischemic stroke, and with an aging population, the burden of stroke is expected to increase dramatically. The difficulty, expense and mortality rate in older animals has resulted in very few aged animal stroke studies being conducted, despite the STAIR criteria suggesting the use of aged animals following initial evaluations in young and healthy animals (Fisher et al., 2009). Such testing is a key part of the strategy for successful translation from bench to bedside (O’Collins et al., 2006). Previous investigations by our group have demonstrated that early treatment with short-duration hypothermia has a preventative effect on intracranial pressure (ICP) elevation, 24 h post-stroke in young rats (Murtha et al., 2014a, 2015). In this short report we aimed to: (1) Determine whether ICP elevation occurred post-stroke in aged rats, and (2) Determine whether a short duration of hypothermia treatment prevented this ICP elevation post-stroke in aged rats.
Materials and Methods
Animals
Experiments were performed on male outbred Wistar rats, aged 19–20 months (n = 16; 623–952 g). This study was carried out in accordance with the Australian Code for the Care and Use of Animals for Scientific Purposes (National Health and Medical Research Council, 2013) and was approved by the University of Newcastle’s Animal Care and Ethics Committee (A.2011.112).
Experimental Protocol
Experimental protocols were conducted according to our established techniques in young and aged animals (McLeod et al., 2011, 2013, 2015; Murtha et al., 2014a,b, 2015; Beard et al., 2015). Following anesthetic induction, a right femoral arterial catheter was inserted for arterial blood pressure (BP) monitoring. A laser Doppler probe and fiber-optic pressure catheter were inserted for monitoring of tissue perfusion and ICP, respectively, and experimental stroke was performed (3 h intraluminal middle cerebral artery occlusion-MCAo). Following 1 h of MCAo, rats were randomly assigned to 2.5 h of hypothermia-treatment (32.5°C) or normothermia (37°C). Animals were then recovered from anesthesia. At 24 h following stroke, neurological deficit scores were measured followed by 1 h of ICP and BP monitoring. Brains were removed for histology.
Anesthesia and Physiological Monitoring
Rats were anesthetized in isoflurane (5% induction, 1.5–2.0% maintenance) in 60:40% N2:O2 and spontaneously breathed through a low dead-space nose cone. Core temperature was maintained and monitored via a thermocouple rectal probe and warming plate. Surgical incision sites were injected with subcutaneous Bupivicaine (2 mg/kg 0.05%; Pfizer, Sydney, NSW, Australia). A single subcutaneous injection of Atropine (0.05 mg/kg) was administered to reduce respiratory secretions during surgery. Arterial blood pressure (BP), cerebral perfusion pressure (CPP), respiratory rate (RR), heart rate (HR) and oxygen saturation (SpO2) were continuously monitored throughout surgery, as previously described (Murtha et al., 2014a, 2015). Prior to recovery, animals were injected with 0.5 mL subcutaneous saline to aide in rehydration. Rats were returned to cages placed half over a heat mat with free access to food and water. All physiological monitoring equipment was reconnected and a left femoral artery catheter inserted for monitoring at 24 h post-stroke.
Intracranial Pressure and Laser Doppler Measurement
Cranial surgery was performed according to previously described methods (Murtha et al., 2012). Briefly, the ICP probe (SAMBA Sensors, Gothenburg, Sweden) was inserted epidurally into a hollow, saline-filled, polyether ether ketone (PEEK) screw placed 2 mm lateral and 2 mm posterior from Bregma in the left parietal bone. The laser Doppler probe (Moor Instruments, UK) was inserted into a second hollow PEEK screw placed 5 mm lateral and 2 mm posterior from Bregma in the right parietal bone. The screws were secured with dental cement and an airtight seal was created around each probe using a caulking material (Silagum, Gunz Dental, Sydney, NSW, Australia). Laser Doppler flow (LDF) and ICP was measured at baseline and throughout stroke surgery. Both probes were removed prior to recovery, and the screws sealed with Silagum. The ICP probe was reinserted and sealed in place for 24 h ICP monitoring.
Experimental Stroke and Hypothermia-Treatment
Stroke surgery was performed using a silicon-tipped monofilament (3.0 mm long × 0.4 mm wide tip) inserted to the base of the right middle cerebral artery via the external carotid artery, as previously described (Spratt et al., 2006). Following 3 h of MCAo, the thread was retracted to the base of the external carotid artery. Occlusion and reperfusion of the middle cerebral artery was confirmed by a respective drop and increase in LDF. One hour after occlusion, animals were randomized to 2.5 h hypothermia-treatment or normothermia under anesthesia. Hypothermia animals were cooled to 32.5°C by evaporative cooling using 70% ethanol spray, a fan at the scapula region of the back, and the reduction of the heat mat temperature to 32.5°C. Normothermia animals were kept at 37°C. Animals were recovered immediately following hypothermia/normothermia in a cage placed half over a heat mat, to allow self-thermoregulation. Neurological deficit testing was performed prior to 24 h physiological monitoring. The forelimb flexion, lateral push and torso twist tests were performed by an assessor blinded to treatment allocation. A total neurological deficit score was given out of 6 where a higher score equated to a larger deficit (Murtha et al., 2014a).
Histological Analysis
Following the 24 h ICP monitoring, rats were transcardially perfused with saline followed by 4% paraformaldehyde in 0.2 M phosphate buffer. Brains were fixed in-skull in neutral-buffered formalin before processing for hematoxylin and eosin staining. Images were scanned on a high resolution digital scanner (Aperio, Vista, CA, USA) before hemisphere and infarct volumes were traced by an investigator blind to treatment-allocation. Infarct volume was corrected for edema using the formula: corrected infarct volume (mm3) = infarct volume × (contralateral volume/ipsilateral volume). Edema was calculated by infarct volume minus corrected infarct volume (Murtha et al., 2015).
Exclusion Criteria and Statistical Analysis
Subarachnoid hemorrhage, equipment malfunction, and lack of infarct were pre-specified exclusion criteria. A sample size calculation (alpha 0.05, power 0.8) for change in ICP from baseline to 24 h (ΔICP) was performed based on our previously published ICP data in young Wistar rats (Murtha et al., 2015; n = 3/group). However, we used a minimum sample size of n = 5 to allow for outlier effects and a potentially smaller effect size. Two-tailed Student’s t-tests were used to compare differences between the treatment groups at particular time points (unpaired) or between time points within treatment groups (paired). Mann-Whitney U-tests were performed to compare neurological deficit scores between treatment groups. Significance was accepted at p < 0.05. Data are presented as mean ± standard deviation (SD). Neurological deficit scores are presented as median (25th–75th interquartile range).
Results
Two animals were excluded prior to surgical intervention: facial tumor (16 months) and unexpected death (19 months). Three animals were excluded following surgical intervention: equipment malfunction (prior to randomization); lack of infarct (normothermia); the presence of blood at the carotid canal extending into the subarachnoid space (normothermia). This hemorrhage was likely due to calcification of the carotid canal causing friction on occluding thread insertion and consequent rupture of the arterial wall. Eleven animals were included in this study.
Mean temperature was 32.7 ± 0.7°C during hypothermia treatment and 37.1 ± 0.4°C during the equivalent normothermia period; Figure 1A. Physiological variables are presented in Table 1. ICPs were 10.4 ± 3.6 mmHg and 11.8 ± 3.1 mmHg at baseline (non-significant), and 27.4 ± 18.2 mmHg and 8.0 ± 5.0 mmHg at 24 h (p = 0.03) in the normothermic and hypothermia-treated groups, respectively. ΔICP was significantly higher in the normothermic group vs. the hypothermia-treated group (17.0 ± 15.8 mmHg vs. −3.8 ± 4.4 mmHg, p = 0.01); Figure 1B.
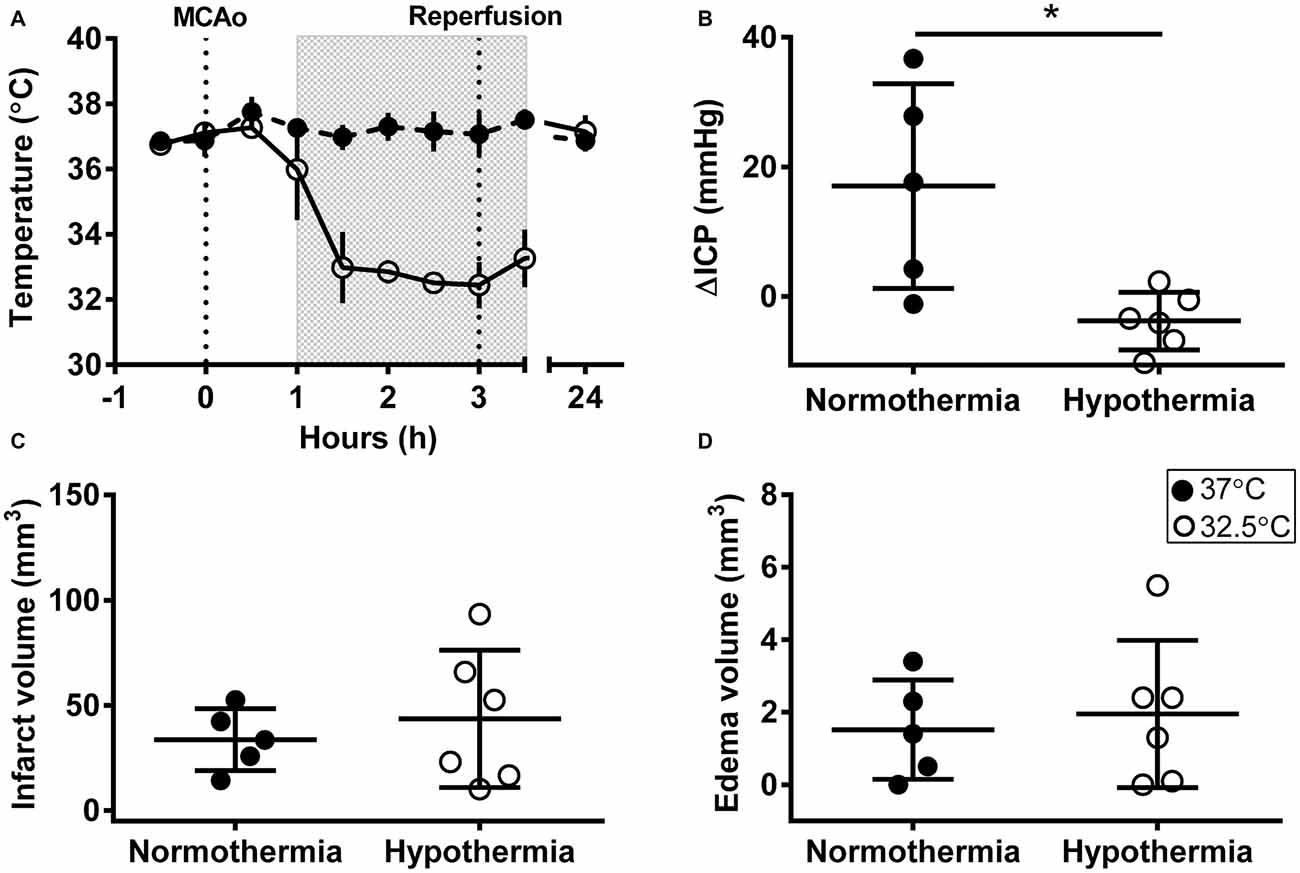
Figure 1. Intracranial pressure (ICP) elevation after experimental stroke was prevented by early, short-duration hypothermia treatment. (A) Rectal temperature during middle cerebral artery occlusion (MCAo), and at 24 h post-MCAo, shaded box indicates hypothermia-treatment. (B) Change in ICP (ΔICP) from baseline to 24 h post-stroke. (C) Infarct volume (corrected for edema) calculated from histological analysis. (D) Edema volume calculated from infarct volume minus corrected infarct volume. Individual data ± SD. *p < 0.05. Normothermia (37°C; closed circles; n = 5); hypothermia-treated (32.5°C; open circles; shaded box; n = 6).
There was no significant difference in infarct volume between normothermic, 33.7 ± 14.7 mm3, and hypothermia-treated groups, 43.6 ± 32.6 mm3, p = 0.55; Figure 1C. Representative images are presented in Figure 2. Edema volume was small in both groups, 1.5 ± 1.4 mm3 and 2.0 ± 2.0 mm3, in the normothermic and hypothermia-treated groups, respectively; p = 0.70; Figure 1D. No significant difference in neurological deficit scores were seen between the normothermia group [median score 2.0 (0.5–2.5)] and the hypothermia-treated group [median score 2.0 (1.0–3.0)], p = 0.61.
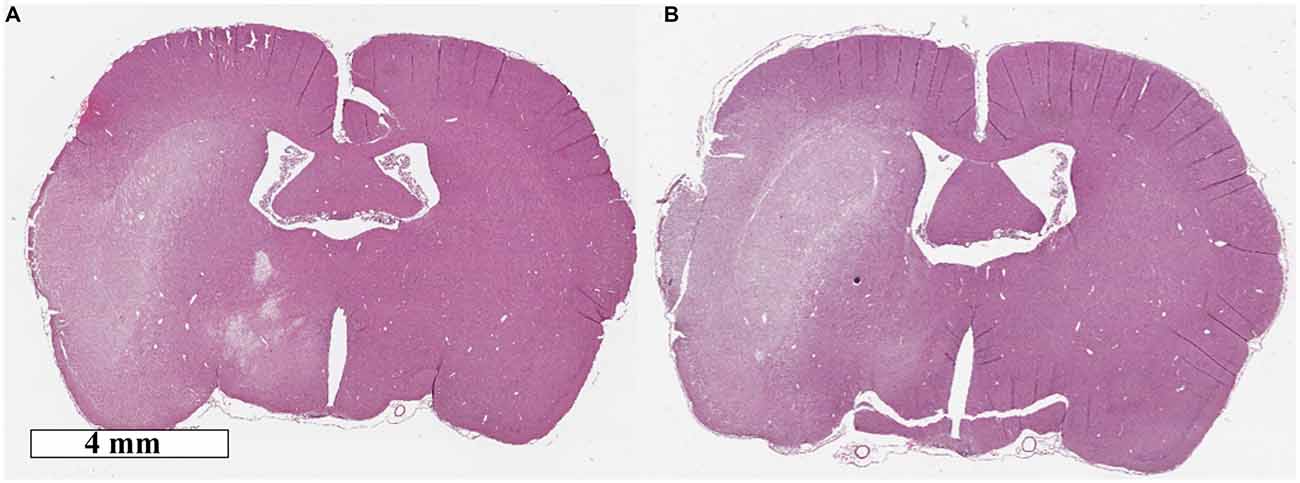
Figure 2. Representative hematoxolin and eosin images of (A) normothermia and (B) hypothermia-treated animals.
Discussion
In this study, we have shown that the newly discovered phenomenon of ICP elevation at 24 h following minor stroke is also found in most aged animals. Second, the dramatic preventative effect of short-duration hypothermia on ICP elevation is also seen in aged animals. It introduces the possibility that: (1) aged patients may have a similar ICP response following ischemic stroke, and (2) short-duration cooling may prevent ICP elevation in aged stroke patients.
Normal human physiological ICP has been shown to change with age (Dunn, 2002), however our knowledge of ICP changes in the aged stroke population is limited. A study by Schwab et al. (1996) demonstrated in a small cohort of large hemispheric stroke patients that maximum ICP values were considerably higher in patients who subsequently died, than in surviving patients (42 vs. 28 mmHg). They found no correlation between patient age and initial ICP values, however correlation between age and maximum ICP values was not reported. It is important to note that these data applied to patients with large infarct and cerebral edema volumes with a mean ICP device insertion of 2.8 ± 1.5 days post stroke-onset. Conversely, our studies in young and aged rats demonstrated ICP elevation in animals with minor infarct and minimal edema volumes, with a peak around 24 h post stroke-onset (Murtha et al., 2014a, 2015). It is likely that two different ICP elevation mechanisms are at play here. Kotwica et al. (1991) noted that in animals with small-medium strokes ICP peaked 24 h after stroke, however, in animals with large volumes of infarct and edema, a second ICP peak was observed after 3 to 4 days. We believe the initial peak in animals with minor stroke is an edema-independent mechanism of ICP elevation. Given the invasiveness of current monitoring equipment, ICP is only measured in patients with large infarct and edema volumes, thus it is possible that initial 24 h peaks are missed in those with smaller strokes. Interestingly, in the current study, two of the five aged animals in the normothermia group had no biologically significant elevation in ICP. This is in contrast to the young animals in which ICP elevation has been near universal (only three of 30 cumulative young animals had an ICP elevation < 10 mmHg; Murtha et al., 2014a, 2015). Even if ICP elevation occurs only in a proportion of aged patients, we believe the concept of ICP elevation following minor stroke warrants further investigation.
Short-duration hypothermia, administered 1 h after stroke in aged animals, completely prevented ICP elevation at 24 h. This study supports the findings from our previous studies of a profound and sustained ICP prevention with short-duration hypothermia in young animals (Murtha et al., 2014a, 2015). Therapeutic hypothermia has long been known to have a neuroprotective effect following global ischemic brain injury—post cardiac arrest (Bernard et al., 2002), and in neonatal hypoxia ischemia (Shankaran et al., 2005). It has also been used as an effective treatment in reducing ICP following traumatic brain injury and hepatic encephalopathy (Jalan et al., 1999; Tokutomi et al., 2003, 2009). Clinical application and feasibility in stroke patients, however, have been hampered by the logistic challenges of long duration cooling in humans (24–48 h) and concerns about rebound ICP complications during rewarming (Schwab et al., 1998, 2001). Few studies have investigated hypothermia after experimental stroke in aged animals, and those that have (long duration gaseous hypothermia, 24–48 h) demonstrated temporary neuroprotection (Florian et al., 2008; Joseph et al., 2012; Sandu et al., 2016; Vintilescu et al., 2016). ICP, and thus rebound elevations during rewarming, however, were not measured in these studies. The majority of experimental animal stroke studies have been performed in young animals and have shown clear neuroprotective benefit with short duration hypothermia (<6 h; van der Worp et al., 2007; Dumitrascu et al., 2016). Furthermore, when a short duration of mild or moderate hypothermia is administered to young or aged rats many hours before ICP begins to rise, there is a sustained preventative effect, without rebound ICP elevation (Murtha et al., 2014a, 2015). Further experimental studies are clearly needed prior to clinical translation, i.e., dosage, time-window and large animal studies. However, if a short period of cooling by 2–5°C is all that is required for sustained ICP prevention, then clinical translation may lead to a quicker, easier and potentially safer treatment than the current long-duration hypothermia protocols.
ICP was chosen as the primary outcome for the current study, rather than infarct volume, to replicate our previous studies in young animals. Our sample size calculation was therefore based on the ICP data from our young animal studies, and was not powered to show differences in infarct volume. The random distribution of variable infarct volumes in a small study may account for the lack of significance for hypothermic neuroprotection. Our previous work has shown that inadvertent occlusion of the anterior choroidal artery is a major source of the variability seen in the filament MCAo model (McLeod et al., 2013). In particular it may account for the dichotomous infarct volume distribution often seen, and which was apparent in the hypothermia group in the current study. Importantly, ICP elevation was completely prevented by hypothermia-treatment even in the animals with larger infarct volumes.
In conclusion, this study has confirmed that the edema-independent ICP rise following minor stroke that was seen in young animals, also occurs in aged animals. Furthermore, we have confirmed that this ICP elevation is prevented by early short-duration hypothermia treatment. Larger scale studies will be required to determine whether this effect on ICP results in smaller infarcts and better neurological recovery. However, these findings, coupled with our recent data showing effects of ICP elevation on collateral blood flow (Beard et al., 2015) indicate that this newly described pathophysiological phenomenon may be of significant potential clinical importance in aged patients.
Author Contributions
LAM, DDM, JTB and NJS were involved in the conception and design of the experiments. LAM performed the surgical and experimental components of the study, analyzed the data, including statistical analysis, and drafted the manuscript. Histological analysis and neurological testing was performed by DP. LAM, DDM, DJB and NJS discussed and interpreted the results and reviewed and edited the manuscript.
Funding
This work was supported by a NHMRC Project Grant, APP1033461, and research support from the Hunter Medical Research Institute from funds donated by the Greater Building Society. LAM was supported by an Emlyn and Jennie Thomas Postgraduate Scholarship. NJS received a NHMRC career development fellowship, APP1035465.
Conflict of Interest Statement
The authors declare that the research was conducted in the absence of any commercial or financial relationships that could be construed as a potential conflict of interest.
Acknowledgments
We would like to thank Dr. Sarah McCann for assistance with pilot studies.
Abbreviations
BP, mean arterial blood pressure; BPM, breaths (RR) or beats (HR) per minute; CPP, cerebral perfusion pressure; HR, heart rate; ICP, intracranial pressure; LDF, laser Doppler flow; MCAo, middle cerebral artery occlusion; RR, respiratory rate; SD, standard deviation; SpO2, oxygen saturation; Temp., temperature.
References
Beard, D. J., McLeod, D. D., Logan, C. L., Murtha, L. A., Imtiaz, M. S., van Helden, D. F., et al. (2015). Intracranial pressure elevation reduces flow through collateral vessels and the penetrating arterioles they supply. A possible explanation for ‘collateral failure’ and infarct expansion after ischemic stroke. J. Cereb. Blood Flow Metab. 35, 861–872. doi: 10.1038/jcbfm.2015.2
Bernard, S. A., Gray, T. W., Buist, M. D., Jones, B. M., Silvester, W., Gutteridge, G., et al. (2002). Treatment of comatose survivors of out-of-hospital cardiac arrest with induced hypothermia. N. Engl. J. Med. 346, 557–563. doi: 10.1056/nejmoa003289
Dumitrascu, O. M., Lamb, J., and Lyden, P. D. (2016). Still cooling after all these years: meta-analysis of pre-clinical trials of therapeutic hypothermia for acute ischemic stroke. J. Cereb. Blood Flow Metab. doi: 10.1177/0271678x16645112 [Epub ahead of print].
Dunn, L. T. (2002). Raised intracranial pressure. J. Neurol. Neurosurg. Psychiatry 73, i23–i27. doi: 10.1136/jnnp.73.suppl_1.i23
Fisher, M., Feuerstein, G., Howells, D. W., Hurn, P. D., Kent, T. A., Savitz, S. I., et al. (2009). Update of the stroke therapy academic industry roundtable preclinical recommendations. Stroke 40, 2244–2250. doi: 10.1161/STROKEAHA.108.541128
Florian, B., Vintilescu, R., Balseanu, A. T., Buga, A. M., Grisk, O., Walker, L. C., et al. (2008). Long-term hypothermia reduces infarct volume in aged rats after focal ischemia. Neurosci. Lett. 438, 180–185. doi: 10.1016/j.neulet.2008.04.020
Jalan, R., O Damink, S. W., Deutz, N. E., Lee, A., and Hayes, P. C. (1999). Moderate hypothermia for uncontrolled intracranial hypertension in acute liver failure. Lancet 354, 1164–1168. doi: 10.1016/s0140-6736(98)12440-6
Joseph, C., Buga, A. M., Vintilescu, R., Balseanu, A. T., Moldovan, M., Junker, H., et al. (2012). Prolonged gaseous hypothermia prevents the upregulation of phagocytosis-specific protein annexin 1 and causes low-amplitude EEG activity in the aged rat brain after cerebral ischemia. J. Cereb. Blood Flow Metab. 32, 1632–1642. doi: 10.1038/jcbfm.2012.65
Kotwica, Z., Hårdemark, H. G., and Persson, L. (1991). Intracranial pressure changes following middle cerebral artery occlusion in rats. Res. Exp. Med. (Berl) 191, 99–104. doi: 10.1007/bf02576664
McLeod, D. D., Beard, D. J., Parsons, M. W., Levi, C. R., Calford, M. B., and Spratt, N. J. (2013). Inadvertent occlusion of the anterior choroidal artery explains infarct variability in the middle cerebral artery thread occlusion stroke model. PLoS One 8:e75779. doi: 10.1371/journal.pone.0075779
McLeod, D. D., Parsons, M. W., Hood, R., Hiles, B., Allen, J., McCann, S. K., et al. (2015). Perfusion computed tomography thresholds defining ischemic penumbra and infarct core: studies in a rat stroke model. Int. J. Stroke 10, 553–559. doi: 10.1111/ijs.12147
McLeod, D. D., Parsons, M. W., Levi, C. R., Beautement, S., Buxton, D., Roworth, B., et al. (2011). Establishing a rodent stroke perfusion computed tomography model. Int. J. Stroke 6, 284–289. doi: 10.1111/j.1747-4949.2010.00564.x
Murtha, L. A., McLeod, D. D., McCann, S. K., Pepperall, D., Chung, S., Levi, C. R., et al. (2014a). Short-duration hypothermia after ischemic stroke prevents delayed intracranial pressure rise. Int. J. Stroke 9, 553–559. doi: 10.1111/ijs.12181
Murtha, L. A., Yang, Q., Parsons, M. W., Levi, C. R., Beard, D. J., Spratt, N. J., et al. (2014b). Cerebrospinal fluid is drained primarily via the spinal canal and olfactory route in young and aged spontaneously hypertensive rats. Fluids Barriers CNS 11:12. doi: 10.1186/2045-8118-11-12
Murtha, L. A., McLeod, D. D., Pepperall, D., McCann, S. K., Beard, D. J., Tomkins, A. J., et al. (2015). Intracranial pressure elevation after ischemic stroke in rats: cerebral edema is not the only cause and short-duration mild hypothermia is a highly effective preventive therapy. J. Cereb. Blood Flow Metab. 35, 592–600. doi: 10.1038/jcbfm.2014.230
Murtha, L., McLeod, D., and Spratt, N. (2012). Epidural intracranial pressure measurement in rats using a fiber-optic pressure transducer. J. Vis. Exp. 62:e3689. doi: 10.3791/3689
O’Collins, V. E., Macleod, M. R., Donnan, G. A., Horky, L. L., van der Worp, B. H., and Howells, D. W. (2006). 1,026 experimental treatments in acute stroke. Ann. Neurol. 59, 467–477. doi: 10.1002/ana.20741
Sandu, R. E., Buga, A. M., Balseanu, A. T., Moldovan, M., and Popa-Wagner, A. (2016). Twenty-four hours hypothermia has temporary efficacy in reducing brain infarction and inflammation in aged rats. Neurobiol. Aging 38, 127–140. doi: 10.1016/j.neurobiolaging.2015.11.006
Schwab, S., Aschoff, A., Spranger, M., Albert, F., and Hacke, W. (1996). The value of intracranial pressure monitoring in acute hemispheric stroke. Neurology 47, 393–398. doi: 10.1212/wnl.47.2.393
Schwab, S., Georgiadis, D., Berrouschot, J., Schellinger, P. D., Graffagnino, C., and Mayer, S. A. (2001). Feasibility and safety of moderate hypothermia after massive hemispheric infarction. Stroke 32, 2033–2035. doi: 10.1161/hs0901.095394
Schwab, S., Steiner, T., Aschoff, A., Schwarz, S., Steiner, H. H., Jansen, O., et al. (1998). Early hemicraniectomy in patients with complete middle cerebral artery infarction. Stroke 29, 1888–1893. doi: 10.1161/01.str.29.9.1888
Shankaran, S., Laptook, A. R., Ehrenkranz, R. A., Tyson, J. E., McDonald, S. A., Donovan, E. F., et al. (2005). Whole-body hypothermia for neonates with hypoxic-ischemic encephalopathy. N. Engl. J. Med. 353, 1574–1584. doi: 10.1056/NEJMcps050929
Spratt, N. J., Fernandez, J., Chen, M., Rewell, S., Cox, S., van Raay, L., et al. (2006). Modification of the method of thread manufacture improves stroke induction rate and reduces mortality after thread-occlusion of the middle cerebral artery in young or aged rats. J. Neurosci. Methods 155, 285–290. doi: 10.1016/j.jneumeth.2006.01.020
Tokutomi, T., Miyagi, T., Takeuchi, Y., Karukaya, T., Katsuki, H., and Shigemori, M. (2009). Effect of 35°C hypothermia on intracranial pressure and clinical outcome in patients with severe traumatic brain injury. J. Trauma 66, 166–173. doi: 10.1097/TA.0b013e318157dbec
Tokutomi, T., Morimoto, K., Miyagi, T., Yamaguchi, S., Ishikawa, K., and Shigemori, M. (2003). Optimal temperature for the management of severe traumatic brain injury: effect of hypothermia on intracranial pressure, systemic and intracranial hemodynamics and metabolism. Neurosurgery 52, 102–111; discussion 111–112. doi: 10.1097/00006123-200301000-00013
van der Worp, H. B., Sena, E. S., Donnan, G. A., Howells, D. W., and Macleod, M. R. (2007). Hypothermia in animal models of acute ischaemic stroke: a systematic review and meta-analysis. Brain 130, 3063–3074. doi: 10.1093/brain/awm083
Keywords: ischemic stroke, intracranial pressure, therapeutic hypothermia, aged rats, middle cerebral artery occlusion, cerebral edema
Citation: Murtha LA, Beard DJ, Bourke JT, Pepperall D, McLeod DD and Spratt NJ (2016) Intracranial Pressure Elevation 24 h after Ischemic Stroke in Aged Rats Is Prevented by Early, Short Hypothermia Treatment. Front. Aging Neurosci. 8:124. doi: 10.3389/fnagi.2016.00124
Received: 23 March 2016; Accepted: 13 May 2016;
Published: 27 May 2016.
Edited by:
Aurel Popa-Wagner, University of Medicine Rostock, GermanyReviewed by:
Raluca Elena Sandu, University of Medicine and Pharmacy Craiova, RomaniaBruno Meloni, Sir Charles Gairdner Hospital, Australia
Copyright © 2016 Murtha, Beard, Bourke, Pepperall, McLeod and Spratt. This is an open-access article distributed under the terms of the Creative Commons Attribution License (CC BY). The use, distribution and reproduction in other forums is permitted, provided the original author(s) or licensor are credited and that the original publication in this journal is cited, in accordance with accepted academic practice. No use, distribution or reproduction is permitted which does not comply with these terms.
*Correspondence: Neil J. Spratt, neil.spratt@newcastle.edu.au