- División de Neurociencias, Instituto de Fisiología Celular, Universidad Nacional Autónoma de México, México City, Federal District, México
Suprathreshold corticostriatal responses recorded from medium spiny neurons (MSNs) from the direct and indirect pathways of the basal ganglia are different. Their differences readily distinguish D1- and D2-type receptor expressing MSNs in both bacterial artificial chromosome-transgenic mice and their control littermates as well as in rats: indirect pathway neurons are more excitable than direct pathway neurons revealing autoregenerative spikes underlying their spike trains, whereas direct pathway neurons exhibit more prolonged plateau potentials and spike trains. SFK 81297, a selective agonist for D1-class receptors enhanced corticostriatal responses in direct pathway neurons, while quinelorane, a selective agonist for D2-class receptors reduced orthodromic and autoregenerative responses in indirect pathway neurons thus making both neuron classes similarly excitable. Because dopaminergic postsynaptic actions target CaV1 (L) class voltage-gated calcium channels in MSNs, we hypothesized that these channels are involved and can explain a part of the dopaminergic actions on corticostriatal integration. Both 2.5 μM nicardipine and 400 nM calciseptine, selective CaV1 channel blockers, reduced corticostriatal responses in both D1- and D2-receptor expressing neurons, respectively. A previous blockade of CaV1 channels occluded the actions of dopamine agonists in both neuronal classes. In contrast, a CaV1 (L) channel activator, 2.5 μM Bay K 8644, enhanced corticostriatal responses in neurons from both pathways. It is concluded that CaV1 intrinsic currents mediate a part of the dopaminergic modulation during orthodromic synaptic integration of cortical inputs in both classes of MSNs.
Introduction
Striatal dopamine (DA) is involved in initiation of learned procedures (Schultz, 2007). Localization of different DA receptors in medium spiny neurons (MSNs) belonging to direct and indirect pathways of the basal ganglia (Gerfen, 2000) has led to the idea that balanced activity in these two pathways is regulated by opposite actions of DA in each of them: the “two pathways hypothesis” (Albin et al., 1989; Mink, 2003; Redgrave et al., 2010). Both voltage- and current-clamp data in single cells have partially confirmed these assumptions: D1-class receptor (D1R) activation facilitates firing in MSNs of the direct pathway by enhancing CaV1 (L) calcium current, whereas D2-class receptor (D2R) activation represses firing in MSNs of the indirect pathway by decreasing the same current (Surmeier et al., 1995; Hernández-López et al., 1997, 2000). Supposedly, facilitating the direct pathway and preventing activity in the indirect pathway are DA roles when promoting movement (Bateup et al., 2010; Kravitz et al., 2010). These DA receptors use different signaling cascades to target CaV1 (L) calcium channels (Surmeier et al., 1995; Hernández-López et al., 1997, 2000).
Nonetheless, although clear differences in DA actions can be recorded during direct stimulation experiments (Surmeier et al., 1995, 2007; Galarraga et al., 1997; Hernández-López et al., 1997, 2000; Salgado et al., 2005), these differences have not been observed during more physiological corticostriatal synaptic responses (Flores-Barrera et al., 2010), when neurons are embedded in their rich polysynaptic network where indirect effects can ensue (Albin et al., 1989; Mink, 2003; Flores-Barrera et al., 2009; Bateup et al., 2010; Kravitz et al., 2010; Redgrave et al., 2010). Two questions need to be answered: First, if indirect pathway D2-expressing neurons are more excitable than direct pathway neurons (Day et al., 2006; Flores-Barrera et al., 2010) then, it is important to know whether activation of D1-class receptors is strong enough to make direct pathway neurons more excitable, perhaps as excitable as indirect pathway neurons. Secondly, it is also important to know whether D2-class receptor activation is strong enough to decrease significantly the corticostriatal response of indirect pathway neurons. In other words, to test the robustness of dopaminergic actions on corticostriatal responses it is necessary to observe whether DA actions do in fact produce a balance in excitability as the “two pathway hypothesis” postulates. If this is so, it is necessary to know how much margin is available to the system in order to achieve the supposed balance. To answer these questions, we used selective agonists and antagonists of D1- and D2-class receptors, bacterial artificial chromosome (BAC) transgenic mice to identify the recorded neurons, and evoked suprathreshold synaptic responses in which both polysynaptic and intrinsic currents are involved (Flores-Barrera et al., 2009, 2010).
Because both DA receptors and CaV1 channels are found in dendrites of MSNs where most cortical inputs arrive (Freund et al., 1984; Carter and Sabatini, 2004; Kreitzer and Malenka, 2005; Day et al., 2006), and because DA modulation particularly targets CaV1 channels in MSNs (Surmeier et al., 1995; Hernández-López et al., 1997, 2000), the present study focuses in the involvement of this intrinsic current on corticostriatal synaptic integration and its role during dopaminergic modulation. However, the present work was not intended to exclude other possible contributors. It was found that dopaminergic actions expected by the two pathways hypothesis are robust and readily observed upon corticostriatal responses, provided the recorded cells from both pathways are identified and selective pharmacological tools are employed.
Materials and Methods
All experiments were carried out in accordance with the National Institutes of Health Guide for Care and Use of Laboratory Animals and were approved by the Institutional Animal Care Committee of the Universidad Nacional Autónoma de México. BAC-transgenic mice for D1- and D2-receptors expressing MSNs (D1- and D2-MSNs) of 60- to 90-day-old mice were anesthetized, decapitated, and their brains removed and submerged in an iced saline solution containing (in mM): 120 NaCl, 3 KCl, 25 NaHCO3, 2 CaCl2, 1 MgCl2, and 11 glucose (33–35°C, 300 mOsm/l with glucose, pH 7.4, after bubbling with 95% O2 plus 5% CO2). Briefly, and as previously described in detail, 350 μm thick parasagittal slices were cut (Flores-Barrera et al., 2010) on a vibratome and left to equilibrate for at least 1 h at room temperature in the same saline. Thereafter, slices were transferred to a recording chamber and continuously superfused with the same saline at 33–35°C. Intracellular recordings were performed from spiny neurons of the dorsal neostriatum using sharp electrodes filled with potassium-acetate 3 M and 1% biocytin (d.c. resistance 80–120 MΩ). Records were obtained with an active bridge electrometer (Neuro Data, Cygnus Technology, Inc., Delaware Water Gap, PA, USA), digitized, and saved for off-line analysis with a personal computer. After recordings, neurons were injected with biocytin for its anatomical identification. Biocytin injected neurons were identified as D1- or D2-type receptors expressing MSNs. D1- and D2-type dopaminergic agonists: SKF 81297 and qinelorane, as well as antagonists: SCH 23390 and sulpìride were used. CaV1 (L) type calcium agonist Bay K 8644 and L-type calcium channel blockers, nicardipine (Sigma, St. Louis, MO, USA), and calciseptine (Alomone Labs, Jerusalem, Israel) were dissolved and applied to the bath saline. Most experiments were paired, so that recordings in the presence and absence of bath-applied drugs were compared in the same neuron with non-parametric statistics (Systat v.7., SPSS Inc., Chicago, IL, USA): When D1 and D2-MSNs parameters were compared Mann–Whitney’s U test was employed. Statistical significance was set at P < 0.05.
Results
Dopaminergic Modulation of Corticostriatal Responses in MSNs from Direct and Indirect Pathways
Medium spiny neurons were identified as belonging to the direct or indirect pathways by double labeling the biocytin-filled and green fluorescent protein positive (GFP+) recorded cells. Figure 1A shows a suprathreshold corticostriatal response (red) from a BAC D1-receptor expressing neuron. Photomicrograph at right shows the double labeled recorded neuron (superimposed eGFP-green and biocytin-red). Figure 1B shows a suprathreshold corticostriatal response (green) from a BAC D2-receptor expressing neuron. Photomicrograph at right shows the double labeled neuron. Corticostriatal responses in D1-receptor expressing MSN (D1-MSN) show action potentials of increasing amplitude and a slowly decaying plateau potentials, whereas D2-receptor expressing MSN (D2-MSN) shows a larger but briefer depolarization with firing of inactivating action potentials followed by a quasi exponential decay. Inset in Figure 1A shows superimposition of both recordings to emphasize their differences (Flores-Barrera et al., 2010): area under D1-MSNs response is 10,568 ± 523 mV·ms and area under D2-MSNs response, without including the autoregenerative response, is 8,204 ± 697 mV·ms, respectively (n = 36; P < 0.001).
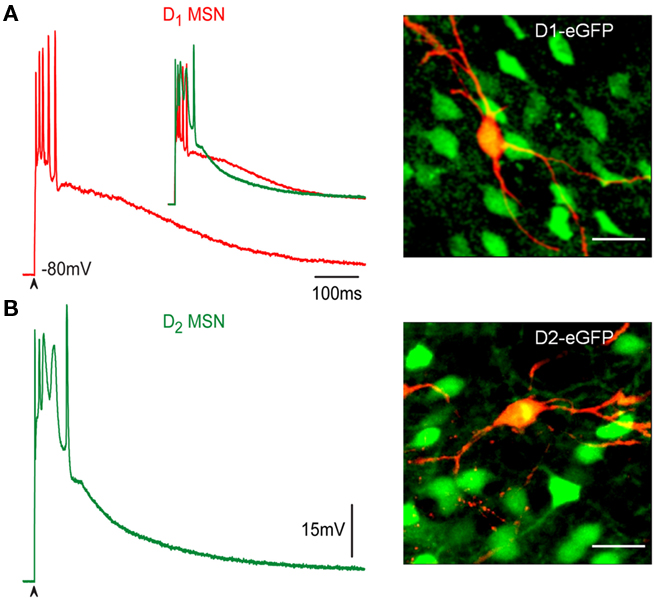
Figure 1. Corticostriatal response in D1- and D2-MSNs. (A,B) Suprathreshold corticostriatal responses in direct (red) and indirect (green) pathway MSNs are different (inset in A, superimposed records) and can serve to identify the neurons during physiological experiments: intrinsic autoregenerative response underlies spikes in D2-MSNs, a more sustained plateau potential is characteristic of D1-MSNs. At right, superimposition of biocytin-filled recorded cells in red with green fluorescent protein positive (GFP+) neurons from BAC mice. Recorded cells are double labeled (yellow). Calibration: 20 μm.
Corticostriatal responses evoked with increasing stimulation strength were tested in D1-MSNs with 1 μM SKF 81297, a selective D1-class receptor agonist (Figures 2A–C, black traces: control; red traces: plus SKF 81297). In all direct pathway neurons recorded, D1-receptor activation enhanced the response, augmenting the depolarization, and the number of action potentials fired. Surprisingly, responses exhibited inactivation of action potentials during synaptic depolarization, a phenomenon more commonly seen in D2-MSNs but rarely seen in D1-MSNs in control conditions (Flores-Barrera et al., 2010). That is, D1-receptor activation could render direct pathway neurons as excitable as indirect pathway neurons. Action of SKF 81297 was completely blocked by the D1-receptor antagonist 1 μM SCH 23390 (n = 3; not shown). Percentages of depolarization enhancement induced by D1-agonist in the maximal corticostriatal response were 29 ± 8% (n = 8; P < 0.03). Figure 2D shows subtractions from the records above. Note that D1-receptor activation enhances the corticostriatal response both by prolonging and increasing the plateau potential in a gradual manner and, most importantly, by disclosing an initial underlying autoregenerative response at the initial depolarization during the highest stimulus strength (Figure 2D, arrow).
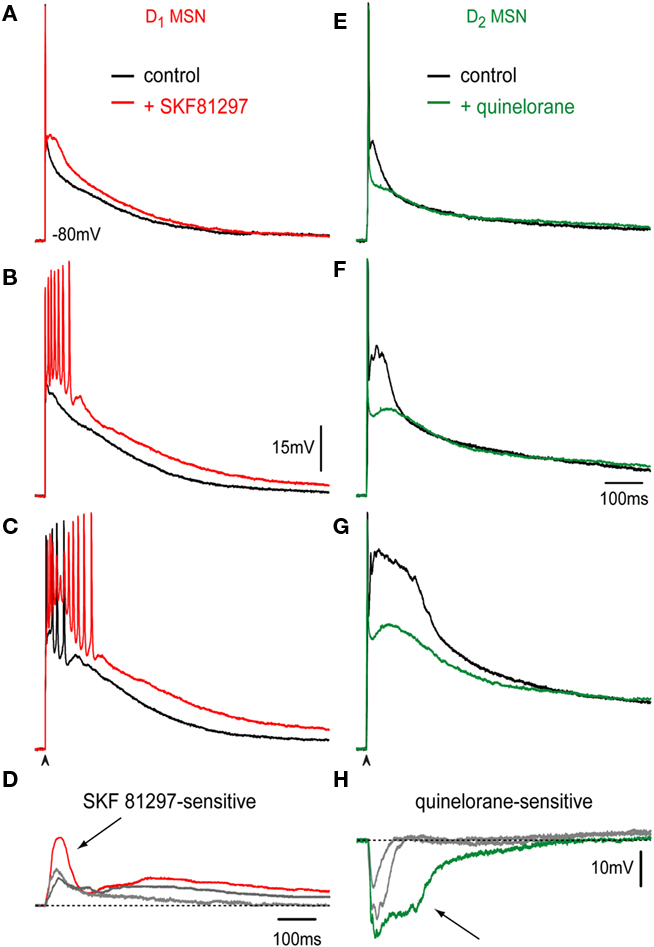
Figure 2. Dopaminergic modulation of corticostriatal responses in D1- and D2-MSNs. (A–C) Orthodromic corticostriatal responses to a single stimulus of increasing stimulation intensity in a D1-MSN. Controls: black and after addition of 1 μM of the D1R selective agonist SKF 81297 (red); superimposed in each frame. Note increasing firing and depolarization due to D1R activation including action potential inactivation (C). (E–G) Orthodromic corticostriatal responses to a single stimulus of increasing stimulation intensity in a D2-MSN. Controls: black and after addition of 1 μM of the D2R selective agonist quinelorane (green); superimposed in each frame. Underlying autoregenerative response is shown instead of the action potential train. Note a decrease in synaptically evoked depolarizations due to D2R activation in each frame. (D,H) Subtraction of superimposed recordings above: D1R action facilitated both initial and sustained plateau depolarizations. Note a possible autoregenerative response (arrow, D). D2R action decreased depolarizations at all stimulus intensities, including autoregenerative responses (arrow, H).
Corticostriatal responses evoked with increasing stimulation strength were tested in D2-MSNs with 1 μM quinelorane, a selective D2-class receptor agonist (Figures 2E–G, black traces: control; green traces: plus quinelorane). In all indirect pathway neurons D2-receptor activation reduced the response, decreasing the depolarization. In these traces, it was preferred to illustrate the autoregenerative response that commonly underlies the train of action potentials in indirect pathway neurons (Flores-Barrera et al., 2010) to show that D2R action decreases that response. Action of quinelorane was completely blocked by the D2-receptor antagonist 1 μM sulpiride (n = 3; not shown). Percentages of depolarization reduction induced by D2-agonist in the maximal corticostriatal response without including the autoregenerative response were 23 ± 6% (n = 10; P < 0.03). That is, D2-receptor activation significantly decreased the synaptic response of indirect pathway neurons. Figure 2H shows subtractions from the records above. Note that D2-receptor activation decreases the corticostriatal response including the autoregenerative response (Figure 2H, arrow; Bargas et al., 1991; Flores-Barrera et al., 2010).
Taking all the results together it appears that DA actions can balance the excitability of direct and indirect pathway neurons of the basal ganglia by both enhancing the excitability of direct and repressing the excitability of indirect pathway neurons (Galarraga et al., 1997; Carter and Sabatini, 2004; Liu et al., 2004; Day et al., 2008) as the “two pathways hypothesis” requires. Areas under synaptic responses for each cell type after DA receptors activation and with respect to their own controls are 9,124 ± 609 mV·ms vs. 11,810 ± 1,264 mV·ms for D1-MSNs (n = 8, P < 0.03) and 8,950 ± 1,006 mV·ms vs. 7,173 ± 687 mV·ms for D2-MSNs (n = 10; P < 0.03).
CaV1 Calcium Current Contribution During Corticostriatal Responses
Both DA receptors and CaV1 (L) calcium channels are found in dendrites of MSNs where cortical inputs arrive to generate corticostriatal responses (Freund et al., 1984; Carter and Sabatini, 2004; Kreitzer and Malenka, 2005; Day et al., 2006). CaV1 channels are activated during corticostriatal responses in non-identified MSNs (Galarraga et al., 1997; Adermark and Lovinger, 2007; Flores-Barrera et al., 2009). These channels are also a main target for D1- and D2-receptors signaling during direct somatic stimulation (Hernández-López et al., 1997, 2000). Finally, CaV1 channels have also been shown to participate in the generation of down- to up-state voltage transitions in MSNs (Vergara et al., 2003). Therefore, it is logical to infer that they may be involved in the evoked corticostriatal responses in both direct and indirect MSNs, however, this last point has not been proved.
Figures 3A,B show that the CaV1 channel blocker, 400 nM calciseptine, reduces corticostriatal responses in both D1- and D2-MSNs. Superimposed records in control (black) and in the presence of calciseptine (blue) confirm that intrinsic calcium currents are activated by corticostriatal transmission in both classes of projection neurons. Blockade of CaV1 channels in D1-MSNs decreased the corticostriatal response by 29 ± 2% (n = 7; P < 0.05), while it decreased the response by 29 ± 2% (n = 8; P < 0.007) in D2-MSNs. Similar reductions were observed after 2.5 μM nicardipine (see Figure 4). Note two rising components in D1-MSNs: initial and late, and only an initial component plus a prolonged decay in D2-MSNs. Subtractions of superimposed records disclose the calciseptine-sensitive component (Figures 3C,D; Flores-Barrera et al., 2010). Areas under responses for each cell type after blockers of CaV1 channels with respect to their own controls are 11,646 ± 750 mV·ms vs. 8,212 ± 462 mV·ms for D1-MSNs (n = 7; P < 0.03) and 7,876 ± 864 mV·ms vs. 5,611 ± 675 mV·ms for D2-MSNs (n = 8; P < 0.007). Inset in Figure 3B shows in other indirect pathway neuron, a reduction in the orthodromic autoregenerative response after 2.5 μM nicardipine.
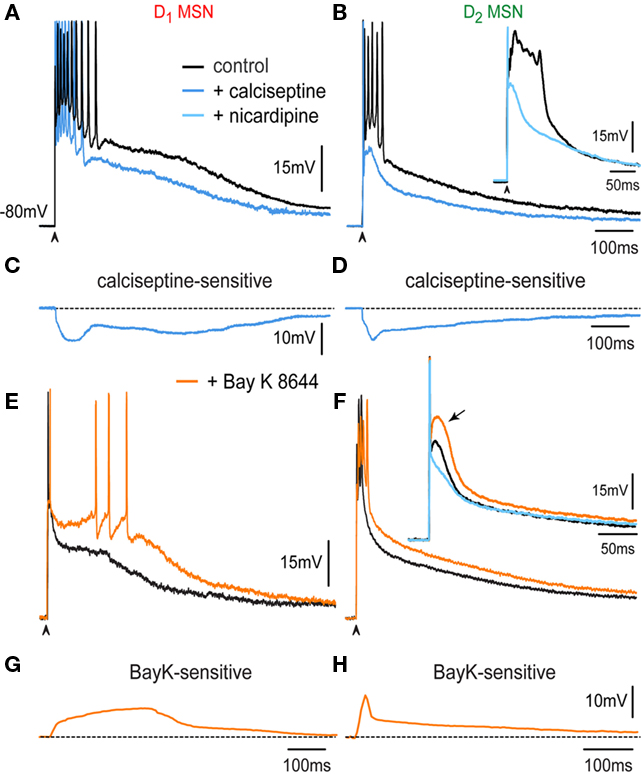
Figure 3. Contribution of CaV1 calcium current during the corticostriatal response in D1- and D2-MSNs. (A) Superimposed records: in control (black) and in the presence of 400 nM calciseptine (blue) in a D1-MSN. (B) Superimposed records: in control (black) and in the presence of 400 nM calciseptine (blue) in a D2-MSN. Note briefer train of action potentials in D2-MSNs as compared to D1-MSNs (cf. A,B). Inset: shows the autoregenerative response that underlies the train of action potentials in D2-MSNs (cf. Figure 2), before (black trace) and during 2.5 μM nicardipine (light blue). (C,D) Subtractions of superimposed records above disclose the calciseptine-sensitive component in D1- and D2-MSNs. Note initial and late rising components in D1-MSNs, and only the initial followed by continuous decay in D2-MSNs. (E) Superimposed records: in control (black) and in the presence of 2.5 μM Bay K 8644 (orange) in a D1-MSN. (F) Superimposed records: in control (black) and in the presence of 2.5 μM Bay K 8644 (orange) in a D2-MSN. CaV1 agonist prolonged the plateau potential and induced the firing of more action potentials in D1-MSNs, whereas it depolarized the response of D2-MSNs along its decay. Inset in (F) shows that Bay K 8644 helps in eliciting an autoregenerative response in D2-MSNs. (G,H) Subtraction of Bay K 8644 actions: Note an increasing and sustained depolarization in D1-MSNs, and a decaying depolarization after the initial peak in D2-MSNs.
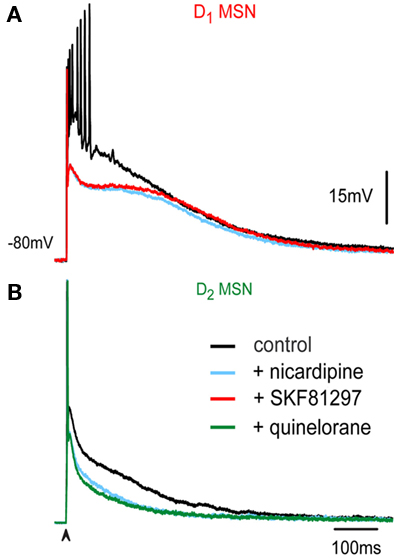
Figure 4. Dopaminergic modulation is decreased when CaV1 are blocked. (A) Superimposed records in a D1-MSN and (B) in a D2-MSN in control (black records) and in the presence of 2.5 μM nicardipine (light blue records). Addition of D1R-agonist SKF 81297 (red recording) in a D1-MSN (A) and of a D2R-agonist quinelorane (green recordings) in a D2-MSN (B). Note lack of actions of DA agonists once CaV1 channels are blocked.
We next tested the effects of the CaV1 (L) calcium agonist Bay K 8644 (2.5–5 μM) on the corticostriatal response. Superimposed records: in control (black) and in the presence of 2.5 μM Bay K 8644 (orange) in a D1-MSN (Figure 3E) and in a D2-MSN (Figure 3F) show that the CaV1 agonist prolongs the plateau potential and induces the firing of more action potentials in D1-MSNs, whereas it depolarized the response of D2-MSNs along its entire decay. Bay K 8644 helps in eliciting an autoregenerative response in D2-MSNs (inset in Figure 3F). Bay K 8644 actions show increasing and sustained depolarizations in D1-MSNs (Figure 3G), and a decaying depolarization after the initial peak in D2-MSNs (Figure 3H). Inset in Figure 3F shows in another indirect pathway neuron, after 2.5 μM nicardipine in the presence of Bay K 8644 a reduction in orthodromic autoregenerative response.
Because the actions of calcium blockers was so similar in both neuronal classes, the different roles that CaV1 channels accomplish in each response, as disclosed by Bay K 8644, suggests that other synaptic or intrinsic currents are in charge of CaV1 channels regulation, perhaps at dendritic sites.
Areas under synaptic responses for each cell type after CaV1 agonist Bay K 8644 with respect to their own controls are 11,821 ± 1,324 mV·ms vs. 15,306 ± 1,600 mV·ms for D1-MSNs (n = 4; P < 0.05) and 7,919 ± 2,178 mV·ms vs. 11,308 ± 2,600 mV·ms for D2-MSNs (n = 4; P < 0.05).
Dopaminergic Modulation is Occluded when CaV1 Channels are Blocked
Figure 4A shows superimposed corticostriatal responses in a representative D1-MSN in control (black), in the presence of 2.5 μM nicardipine (light blue) and after application of the D1-class receptor agonist 1 μM SKF 81297 (red). Note absence of action of D1-agonist after blockade of CaV1 channels. Figure 4B shows superimposed corticostriatal responses in a representative D2-MSN in control (black), in the presence of 2.5 μM nicardipine (light blue) and after application of the D2-class receptor agonist 1 μM quinelorane (green). Note absence of action of D2-agonist after blockade of CaV1 channels. These experiments suggest that CaV1 channels are a major target of DA modulation during corticostriatal integration.
Discussion
Corticostriatal responses of D1- and D2-MSNs constitute an “electrophysiological footprint” that readily distinguishes between direct and indirect pathway neurons (Flores-Barrera et al., 2010) because dendritic excitability, and therefore, corticostriatal integration is different in D1- and D2-MSNs when all synaptic and intrinsic currents are in place (Day et al., 2008; Flores-Barrera et al., 2010). The present work demonstrated: (1) A selective dopaminergic D1-class receptor mediated increase in firing and depolarization of the corticostriatal response in D1-MSNs in such a way as to approach the level of excitability found in D2-MSNs. (2) A selective dopaminergic D2-class receptor mediated a significant decrease in firing and depolarization of the corticostriatal response in D2-MSNs. (3) CaV1 (L) calcium currents contribute to corticostriatal integration in both D1- and D2-MSNs; including the autoregenerative response underlying the train of spikes characteristic of D2-MSNs. (4) Blockade of CaV1 channels occluded the actions of selective DA receptor agonists in their respective responsive neurons from the direct and indirect pathways. (5) By acting in both pathways simultaneously DA may balance the excitability of the neurons from both basal ganglia pathways to make D2-MSNs less excitable than D1-MSNs, if necessary, thus reversing their responsiveness to cortical commands. These findings show that there is enough margin to tune up the excitability of the neurons from both pathways, upon demand. This tuning capability is very probably lost during Parkinsonism (Flores-Barrera et al., 2010).
The present work did not exclude, however, the possible participation of other calcium channels (Carter and Sabatini, 2004; Salgado et al., 2005; Higley and Sabatini, 2010) in corticostriatal integration and only underlines the importance of CaV1 channels.
CaV1 channels are positioned near cortical glutamatergic synapses in MSNs dendrites (Freund et al., 1984; Carter and Sabatini, 2004; Higley and Sabatini, 2010), a strategic location to participate in corticostriatal integration. However, in spite of voltage- and current-clamp recordings during direct stimulation experiments in single cells agree with the assumptions of the “two pathways hypothesis,” that are, first, D1-class receptor activation facilitates firing in D1-MSNs during direct somatic stimulation of single cells (Hernández-López et al., 1997) and enhances CaV1 calcium current in isolated neurons (Surmeier et al., 1995), and secondly, D2-class receptor activation represses firing in MSNs of the indirect pathway by decreasing the same current (Hernández-López et al., 1997, 2000; Day et al., 2008), the fact is that in trying to find these differences during striatal network activity, in vivo, the expectations of the two pathways hypothesis become very variable and even contradictory (Liang et al., 2008; Kravitz et al., 2010).
It is argued that an undisputed electrophysiological evidence of the two pathways hypothesis is lacking during more physiological conditions because indirect circuit influences distort or increase the variability of the responses (e.g., Kravitz et al., 2010). Therefore, in this work we wanted to observe whether dopaminergic actions expected by the “two pathways hypothesis” is robust enough to be preserved during corticostriatal suprathreshold dendritic integration which is known to activate polysynaptic pathways as well as several intrinsic inward and outward currents (Flores-Barrera et al., 2009, 2010). This implies to test DA actions during more physiological responses.
The present experimental work demonstrates the main postulate of the “two pathways hypothesis,” that is, that the excitability of neurons from both basal ganglia pathways can reach a dynamic balance due to both D1- and D2-class receptors activation which enhance and decrease, respectively, the corticostriatal responses of direct and indirect pathway neurons. DA actions have a wide range of operation since D1-MSNs can become more excitable than D2-MSNs if necessary. Therefore, the present data support DA role as that of balancing both basal ganglia pathways. Targeting of CaV1 channels was somehow expected since the gating of voltage-dependent and ligand-gated (ionotropic) ion channels in the dendritic membrane, as well as long-term synaptic plasticity, depend on calcium entry through these channels (Snyder, et al., 2000; Hallett et al., 2006; Adermark and Lovinger, 2007).
Variable results and randomness in firing found in vivo more physiological situations (Kostal et al., 2007) are attributed to a population or vectorial neural coding (Wu et al., 2002), evidence of which, has been obtained for the striatal microcircuit (Carrillo-Reid et al., 2008, 2009). Thus, although the present work supports potent and robust DA postsynaptic actions in a more physiological situation, several groups have also reported potent presynaptic actions on terminals making contact with each class of projection neuron (Flores-Hernández et al., 1997; Guzmán et al., 2003; Bamford et al., 2004; Tecuapetla et al., 2007) and following determined connections rules (Taverna et al., 2008), suggesting the possibility of a concomitant dynamic regulation of synaptic weights as well as dopaminergic regulation of striatal interneurons (Tepper et al., 2004).
Conclusion
Dopamine plays a fundamental role in normal basal ganglia function, the deficits arising from reductions in the sustained stimulation of DA receptors show a rather wide involvement in behavioral processes (Schultz, 2007). After DA depletion, a reduction in the corticostriatal response was reported in substance P expressing MSNs whereas an enhancement in the response was observed in enkephalin expressing MSNs, partially explained by a decrease of synaptic GABAergic connections among MSNs (Flores-Barrera et al., 2010). In contrast, the present study shows that the dopaminergic D1R activation increased the corticostriatal response in D1-MSN up to the point of action potential inactivation as that observed in D2-MSNs whereas D2R activation depressed the corticostriatal response in D2-MSNs and decreases duration and amplitude of calcium autoregenerative responses recorded in these neurons. Therefore, dopaminergic DA receptors actions tend to equalize the excitability of MSNs from both pathways.
Because modulation of CaV1 channels is lost in the absence of DA, the changes observed in the corticostriatal response after DA depletion: a decrease in the depolarizing plateau of SP+ expressing MSNs, and a greater intrinsic excitability of ENK+ expressing MSNs can be readily explained. The end result is that both classes of MSNs lose their ability to balance their activity during Parkinsonism (Flores-Barrera et al., 2010), being, perhaps, a main cause of movement impairment.
It is concluded that strong cortical stimulation can overcome all these indirect network effects so that the present results support the DA role expected by the “two pathways hypothesis.”
Conflict of Interest Statement
The authors declare that the research was conducted in the absence of any commercial or finantcial relationships that could be construed as a potential conflict of interest.
Acknowledgments
We thank Antonio Laville and Gabriela X Ayala for technical support and advice. This work was supported by grants from a Project Program grant IMPULSA 03 from UNAM, by Consejo Nacional de Ciencia y Tecnología (Mexico) Grant 98004, and by grants from Direccion General de Asuntos del Personal Académico. Universidad Nacional Autónoma de México: IN205610 and IN206010 to José Bargas and Elvira Galarraga.
References
Adermark, L., and Lovinger, D. M. (2007). Combined activation of L-type Ca2+ channels and synaptic transmission is sufficient to induce striatal long-term depression. J. Neurosci. 27, 6781–6787.
Albin, R. L., Young, A. B., and Penney, J. B. (1989). The functional anatomy of basal ganglia disorders. Trends Neurosci. 12, 366–375.
Bamford, N. S., Zhang, H., Schmitz, Y., Wu, N. P., Cepeda, C., Levine, M. S., Schmauss, C., Zakharenko, S. S., Zablow, L., and Sulzer, D. (2004). Heterosynaptic dopamine neurotransmission selects sets of corticostriatal terminals. Neuron 42, 653–663.
Bargas, J., Galarraga, E., and Aceves, J. (1991). Dendritic activity on neostriatal neurons as inferred from somatic intracellular recordings. Brain Res. 539, 159–163.
Bateup, H. S., Santini, E., Shen, W., Birnbaum, S., Valjent, E., Surmeier, D. J., Fisone, G., Nestler, E. J., and Greengard, P. (2010). Distinct subclasses of medium spiny neurons differentially regulate striatal motor behaviors. Proc. Natl. Acad. Sci. U.S.A. 107, 14845–14850.
Carrillo-Reid, L., Tecuapetla, F., Tapia, D., Hernandez-Cruz, A., Galarraga, E., Drucker-Colin, R., and Bargas, J. (2008). Encoding network states by striatal cell assemblies. J. Neurophysiol. 99, 1435–1450.
Carrillo-Reid, L., Tecuapetla, F., Vautrelle, N., Hernandez, A., Vergara, R., Galarraga, E., and Bargas, J. (2009). Muscarinic enhancement of persistent sodium current synchronizes striatal medium spiny neurons. J. Neurophysiol. 102, 682–690.
Carter, A. G., and Sabatini, B. L. (2004). State-dependent calcium signaling in dendritic spines of striatal medium spiny neurons. Neuron 44, 483–493.
Day, M., Wang, Z., Ding, J., An, X., Ingham, C. A., Shering, A. F., Wokosin, D., Ilijic, E., Sun, Z., Sampson, A. R., Mugnaini, E., Deutch, A. Y., Sesack, S. R., Arbuthnott, G. W., and Surmeier, D. J. (2006). Selective elimination of glutamatergic synapses on striatopallidal neurons in Parkinson disease models. Nat. Neurosci. 9, 251–259.
Day, M., Wokosin, D., Plotkin, J. L., Tian, X., and Surmeier, D. J. (2008). Differential excitability and modulation of striatal medium spiny neuron dendrites. J. Neurosci. 28, 11603–11614.
Flores-Barrera, E., Laville, A., Plata, V., Tapia, D., Bargas, J., and Galarraga, E. (2009). Inhibitory contribution to suprathreshold corticostriatal responses: an experimental and modeling study. Cell. Mol. Neurobiol. 29, 719–731.
Flores-Barrera, E., Vizcarra-Chacón, B. J., Tapia, D., Bargas, J., and Galarraga, E. (2010). Different corticostriatal integration in spiny projection neurons from direct and indirect pathways. Front. Syst. Neurosci. 4:15. doi: 10.3389/fnsys.2010.00015
Flores-Hernández, J., Galarraga, E., and Bargas, J. (1997). Dopamine selects glutamatergic excitatory inputs to the neostriatum. Synapse 25, 185–195.
Freund, T. F., Powell, J. F., and Smith, A. D. (1984). Tyrosine hydroxylase-immunoreactive boutons in synaptic contact with identified striatonigral neurons, with particular reference to dendritic spines. Neuroscience 13, 1189–1215.
Galarraga, E., Hernández-López, S., Reyes, A., Barral, J., and Bargas, J. (1997). Dopamine facilitates EPSPs through an L-type Ca2+-conductance. Neuroreport 8, 2183–2186.
Gerfen, C. R. (2000). Molecular effects of dopamine on striatal-projection pathways. Trends Neurosci. 23, S64–S70.
Guzmán, J. N., Hernandez, A., Galarraga, E., Tapia, D., Laville, A., Vergara, R., Aceves, J., and Bargas, J. (2003). Dopaminergic modulation of axon collaterals interconnecting spiny neurons of the rat striatum. J. Neurosci. 23, 8931–8940.
Hallett, P. J., Spoelgen, R., Hyman, B. T., Standaert, D. G., and Dunah, A. W. (2006). Dopamine D1 activation potentiates striatal NMDA receptors by tyrosine phosphorylation-dependent subunit trafficking. J. Neurosci. 26, 4690–4700.
Hernández-López, S., Bargas, J., Surmeier, D. J., Reyes, A., and Galarraga, E. (1997). D1 receptor activation enhances evoked discharge in neostriatal medium spiny neurons by modulating an L-type Ca2+-conductance. J. Neurosci. 17, 3334–3342.
Hernández-López, S., Tkatch, T., Pérez-Garci, E., Galarraga, E., Bargas, J., Hamm, H., and Surmeier, D. J. (2000). D2 dopamine receptors in striatal medium spiny neurons reduce L-type Ca2+ currents and excitability through a novel PLCB1/IP3/calcineurin signaling cascade. J. Neurosci. 20, 8987–8995.
Higley, M. J., and Sabatini, B. L. (2010). Competitive regulation of synaptic Ca2+ influx by D2 dopamine and A2A adenosine receptors. Nat. Neurosci. 13, 958–967.
Kostal, L., Lansky, P., and Rospars, J. P. (2007). Neuronal coding and spiking randomness. Eur. J. Neurosci. 26, 2693–2701.
Kravitz, A. V., Freeze, B. S., Parker, P. R., Kay, K., Thwin, M. T., Deisseroth, K., and Kreitzer, A. C. (2010). Regulation of parkinsonian motor behaviours by optogenetic control of basal ganglia circuitry. Nature 466, 622–626.
Kreitzer, A. C., and Malenka, R. C. (2005). Dopamine modulation of state dependent endocannabinoid release and long-term depression in the striatum. J. Neurosci. 25, 10537–10545.
Liang, L., DeLong, M. R., and Papa, S. M. (2008). Inversion of dopamine responses in striatal medium spiny neurons and involuntary movements. J. Neurosci. 28, 7537–7547.
Liu, J. C., DeFazio, R. A., Espinosa-Jeffrey, A., Cepeda, C., de Vellis, J., and Levine, M. S. (2004). Calcium modulates dopamine potentiation of N-methyl-d-aspartate responses: electrophysiological and imaging evidence. J. Neurosci. Res. 76, 315–322.
Mink, J. W. (2003). The basal ganglia and involuntary movements: impaired inhibition of competing motor patterns. Arch. Neurol. 60, 1365–1368.
Redgrave, P., Rodriguez, M., Smith, Y., Rodriguez-Oroz, M. C., Lehericy, S., Bergman, H., Agid, Y, Delong, M. R., and Obeso, J. A. (2010). Goal-directed and habitual control in the basal ganglia: implications for Parkinson’s disease. Nat. Rev. Neurosci. 11, 760–772.
Salgado, H., Tecuapetla, F., Perez-Rosello, T., Perez-Burgos, A., Perez-Garci, E., Galarraga, E., and Bargas, J. (2005). A reconfiguration of CaV2 Ca2+ channels current and its dopaminergic D2 modulation in developing neostriatal neurons. J. Neurophysiol. 94, 3771–3787.
Schultz, W. (2007). Multiple dopamine functions at different time courses. Annu. Rev. Neurosci. 30, 259–288.
Snyder, G. L., Allen, P. B., Fienberg, A. A., Valle, C. G., Huganir, R. L., Nairn, A. C., and Greengard, P. (2000). Regulation of phosphorylation of the GluR1 AMPA receptor in the neostriatum by dopamine and psychostimulants in vivo. J. Neurosci. 20, 4480–4488.
Surmeier, D. J., Bargas, J., Hemmings, H. C. Jr., Nairn, A. C., and Greengard P. (1995). Modulation of calcium currents by a D1 dopaminergic protein kinase/phosphatase cascade in rat neostriatal neurons. Neuron 14, 385–397.
Surmeier, D. J., Ding, J., Day, M., Wang, Z., and Shen, W. (2007). D1 and D2 dopamine-receptor modulation of striatal glutamatergic signaling in striatal medium spiny neurons. Trends Neurosci. 30, 228–235.
Taverna, S., Ilijic, E., and Surmeier, D. J. (2008). Recurrent collateral connections of striatal medium spiny neurons are disrupted in models of Parkinson’s disease. J. Neurosci. 28, 5504–5512.
Tecuapetla, F., Carrillo-Reid, L., Bargas, J., and Galarraga, E. (2007). Dopaminergic modulation of short term synaptic plasticity at striatal inhibitory synapses. Proc. Natl. Acad. Sci. U.S.A. 104, 10258–10263.
Tepper, J. M., Koós, T., and Wilson, C. J. (2004). GABAergic microcircuits in the neostriatum. Trends Neurosci. 27, 662–669.
Vergara, R., Rick, C., Hernández-López, S., Laville, J. A., Guzmán, J. N., Galarraga, E., Surmeier, D. J., and Bargas, J. (2003). Membrane potential oscillations of striatal medium spiny neurons in a corticostriatal slice. J. Physiol. 553, 169–182.
Keywords: CaV1 channels, strionigral pathway, striopallidal pathway, medium spiny neurons, dopamine receptors, corticostriatal pathway
Citation: Flores-Barrera E, Vizcarra-Chacón BJ, Bargas J, Tapia D and Galarraga E (2011) Dopaminergic modulation of corticostriatal responses in medium spiny projection neurons from direct and indirect pathways. Front. Syst. Neurosci. 5:15. doi: 10.3389/fnsys.2011.00015
Received: 17 December 2010;
Paper pending published: 06 March 2011;
Accepted: 15 March 2011;
Published online: 29 March 2011.
Edited by:
James M. Tepper, Rutgers, The State University of New Jersey, USAReviewed by:
Michael S. Levine, Brain Research Institute, USAJeffery R. Wickens, Okinawa Institute of Science and Technology, Japan
Copyright: © 2011 Flores-Barrera, Vizcarra-Chacón, Bargas, Tapia and Galarraga. This is an open-access article subject to an exclusive license agreement between the authors and Frontiers Media SA, which permits unrestricted use, distribution, and reproduction in any medium, provided the original authors and source are credited.
*Correspondence: Elvira Galarraga, División de Neurociencias, Instituto de Fisiología Celular, Universidad Nacional Autónoma de México, PO Box: 70-253, México City, Federal District 04510, México.e-mail:egalarra@ifc.unam.mx