- 1Department of Organisms and Ecosystems Research, National Institute of Biology, Ljubljana, Slovenia
- 2University of Ljubljana, Ljubljana, Slovenia
- 3Jovan Hadži Institute of Biology, Research Centre of the Slovenian Academy of Sciences and Arts, Ljubljana, Slovenia
- 4Department of Entomology, National Museum of Natural History, Smithsonian Institution, Washington, DC, United States
- 5Centre for Behavioural Ecology and Evolution, College of Life Sciences, Hubei University, Wuhan, Hubei, China
- 6Department of Biotechnology and Systems Biology, National Institute of Biology, Ljubljana, Slovenia
Historical biogeography is an integrative scientific field critical for testing evolutionary hypotheses pertinent to organismal distributions, but despite recent theoretical and analytical advances, biogeographic reconstructions continue to struggle with accuracy and rigor. Most modern studies include the three elements needed for historical biogeographic inference, namely a time-calibrated phylogeny, contemporary taxonomic distributions, and estimations of organismal dispersal probabilities. The latter, we argue, are particularly vague, and historical biogeography would greatly benefit from dispersal probability estimations that are better informed and biologically meaningful. To achieve that goal, next-generation biogeography should ideally consider: a) dispersal-related traits; b) ecology; c) geological histories; and d) geographical factors. We briefly recap the three case studies on spiders that have pioneered this approach. Due to their old age and mega-diversity—considering both phylogenetic and life style diversity—arachnids are an ideal animal lineage for modern biogeographic research. There is no reason, however, that the concept should not be applied to all life. Further modifications of the proposed concept and, particularly, methodological implementation are needed to render this biogeographic framework widely useful.
Introduction
Lying at the interface of numerous disciplines, such as evolutionary biology, paleontology, geology, geography, climatology, ecology, and phylogenetics, biogeography is critical for understanding both past and future organismal distributions. Before the end of the previous century, historical biogeography was descriptive. The cladistic era brought to it a hypothetico-deductive agenda, with area cladograms assuming three potential mechanisms responsible for observed biogeographic distributions, i.e. vicariance, dispersal, and extinction (Morrone and Crisci, 1995; Ronquist and Sanmartín, 2011; Sanmartín, 2012). Of these, vicariance was usually the preferred explanation of patterns (de Queiroz, 2005). Since then, a new paradigm has been embraced that established long-distance dispersal as another testable explanation of biogeographical patterns (Cowie and Holland, 2006; Gillespie et al., 2012). Along with the development of statistical approaches to ancestral area estimation, the field of historical biogeography has gained a new momentum (Ronquist and Sanmartín, 2011; Matzke, 2014; Yu et al., 2015). These tools have allowed for modern, comprehensive reconstructions of organismal biogeographic histories that are critical for testing evolutionary hypotheses.
Limitations in ancestral area reconstruction do persist, however, preventing modern biogeography from improving its rigor. As pointed out by Wiens and Donoghue (2004), historical biogeography has largely developed its own agenda devoid of details of organismal ecology. Eighteen years since their paper, this gap has not narrowed substantially. In our view, the most serious limitation is the arbitrary estimation of dispersal probabilities that should consider dispersal-related traits of organisms, their ecology, as well as the relevant geological histories and geographical factors. Here, we emphasize the need for an integrative biological concept intended to overcome this perceived gap and aid our understanding of past and present patterns of biodiversity. The proposed approach, termed next-generation biogeography, is to integrate pertinent data from phylogenetics, geography, taxonomy, evolution, natural history, and ecology—all these are often used or considered separately—into a historic biogeographic framework that can be used for studying any organisms, anywhere.
Next-generation biogeography
Elements and factors for a next-generation biogeographic reconstruction
Most historical biogeographic studies already contain the following three elements (Figure 1): 1. A time calibrated phylogeny; 2. Contemporary taxonomic distributions binned into logical biogeographical units relevant to the question; and 3. Organismal dispersal probabilities. Algorithms combine these elements for ancestral area estimation using various biogeographic models (Matzke, 2014; Yu et al., 2015). We argue that existing biogeographical research is particularly weak in establishing biologically meaningful, and repeatable, dispersal probabilities. The left panel in Figure 1 lists the factors that in our view should be considered when estimating dispersal probabilities:
a. Dispersal-related traits; These include behaviors and physiologies that affect dispersal (Canestrelli et al., 2016; Arnold et al., 2017), solitary versus group-living (Avilés et al., 2007; Johannesen et al., 2007), sex-specific biologies (Fairbairn et al., 2007; Kuntner and Coddington, 2020), the presence of extended phenotypes such as webs, nests or retreats (Blamires, 2010; Mainwaring et al., 2014; Fisher et al., 2019), or any other life histories of target organisms that may affect modes or effectiveness of dispersal (Stevens et al., 2012).
b. Ecology; Species and lineages face different environmental and biotic conditions and interactions that affect their realized niches at various times in history (Wiens and Donoghue, 2004; Guisan et al., 2006).
c. Geological histories; Within the relevant areas, these refer to plate tectonic movements including geologic histories of islands (Roderick and Gillespie, 1998; Lomolino et al., 2017; Whittaker et al., 2017).
d. Geographical factors; Within the relevant areas, decisive geographical factors may include changes in wind and sea currents (Renner, 2004; Gillespie et al., 2012), climatic oscillations (Bowman et al., 2010), and other events potentially affecting organismal dispersal, such as volcanic eruptions (McDowall, 1996), mountain uplifts (Esquerré et al., 2019), or river formation events (Xu et al., 2018). This category also includes anthropogenic translocations (Wilmshurst et al., 2008).
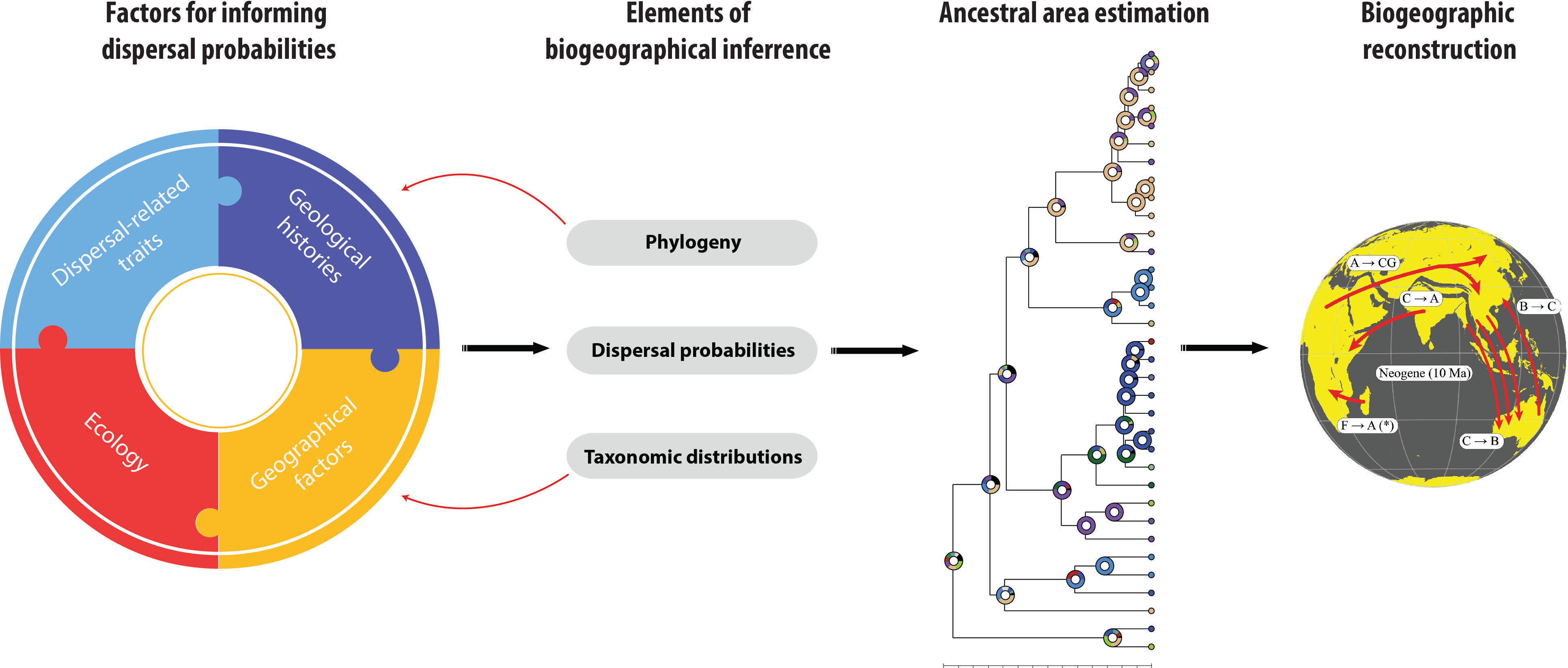
Figure 1 A conceptual biogeographical framework for reconstructing ancestral distributions with better-informed dispersal probabilities. While most conventional biogeographic studies already include the three elements, i.e. a time calibrated phylogeny, contemporary taxonomic distributions, and organismal dispersal probabilities, the latter are particularly arbitrary. The left panel lists the factors that could improve estimations of organismal dispersal probabilities.
The four categories of factors (Figure 1: left panel) can be reciprocally informed by the time-calibrated phylogeny, as well as extant and extinct taxonomic distributions of the taxa in question (Figure 1: red arrows). This means that a hypothesis of species relatedness (phylogeny) and the absolute ages of the evolutionary splits, as well as the lengths of branches (chronogram), should influence the way we deem that dispersal probabilities must be affected in a given geographic, geological, and ecological space and time. Furthermore, the known taxonomic distributions combined with phylogenetic information are informative of the geologies and ecologies of relevant areas, the geographical activities of that time, and dispersal-related traits of extant taxa as well as their hypothetical ancestors, even if extinct. Once all this information is accounted for in dispersal probability estimates, ancestral area estimation will increase in reliability, and, with it, its interpretations: the reconstruction of biogeographic history (Figure 1: panels on right). Consequently, biogeographic hypotheses will undergo more rigorous testing.
A hypothetical example of next-generation biogeographic reconstruction
To illustrate our point, consider a hypothetical example of a lineage whose extant species show sufficient biological variation and inhabit a range of landmasses and habitats. The various types of information needed to estimate dispersal probabilities in this hypothetical clade are: a) Within the dispersal-related traits category, one would quantify this clade’s dispersal ability based on, for example, how effective active aerial dispersal of these organisms is and how it differs among the sexes or life stages; b) Within the ecology category, one would evaluate past distribution patterns of suitable habitat and climate, the presence of competing clades, and the presence of predators and prey; c) Within the geological histories category, distances among landmasses in the relevant time periods should be considered (as reciprocally informed by the chronogram); and finally, d) Within the geographical factors category, one would take into account the emergence of substantial mountain barriers and changes in wind patterns in the relevant time frames (again, as reciprocally informed by the chronogram).
All factors a-d could be quantified on a uniform scale, directly or via appropriate, concretely quantified proxies, and combined into super-informed dispersal probability estimates. One of the most widely used software packages for biogeographic history inference, RASP (Yu et al., 2015), integrates a set of matrices, allowing the user to set time- and area-specific values for various parameters influencing historical biogeography, such as dispersal probabilities. We should note here that current software does not allow for dispersal probabilities to vary across the phylogeny, thus failing to account for known biological differences among taxa within a studied lineage. Matrices that include the proposed super-informed dispersal estimates can currently only vary by time slices. Future software development should ideally allow for dispersal probability variation among the taxa within a given phylogeny.
Case studies on spiders
In practice, inclusion of all listed elements in Figure 1 may not always be feasible, primarily due to lack of data. We demonstrate this below on three case studies on spiders that have pioneered this concept of arriving at better-informed dispersal probabilities in historical biogeography (Turk et al., 2020; Turk et al., 2021a; Magalhaes and Ramírez, 2022).
In the first case study, Turk et al. (2020) reconstructed the geographical origin and sequence of intercontinental colonization of golden orbweavers, the spider family Nephilidae. That study combined a robust phylogeny with geological (plate tectonic) data, specifics of organismal biology (dispersal behaviour) and probabilities of historic dispersal events, with the aim to test two alternative hypotheses about the origin of this spider clade. To arrive at estimates of dispersal probabilities, they used a novel method of evaluating varying dispersal probabilities among geographical areas through time. They measured physical distances between pairs of areas on a geological reconstruction model and used them as a proxy for dispersal probabilities, thus accounting for plate tectonics. The estimated dispersal probabilities were treated as continuous variables. This analysis was strong in allowing for the factor c (geological histories) to influence the estimated dispersal probabilities though time slices. In addition, this study heeded factor a (dispersal-related traits) by accounting for specific dispersal behaviours of the studied organisms. Namely, because these spiders are known to disperse by ballooning, dispersal probabilities were allowed to vary depending on the measured distances between landmasses in specific time slices. At the global scale of this study, Turk et al. failed to identify any factors relating to factor b (ecology) that would influence dispersal probabilities.
In the second case study, Turk et al. (2021a) zoomed into a subset of the family phylogeny, into the biogeography of coin spiders (the genus Herennia) from Asia and Australasia. The studied geographical area is characterized by a complex geological past, complicating biogeographic reconstruction. Turk et al. (2021a) modified the above approach to account for how geological histories affected dispersal probabilities. This study’s strength, as in the one above, was in allowing for the factor c (geological histories) to influence the estimated dispersal probabilities though time slices. A limitation of this study, however, was the undocumented dispersal biology of Herennia. Rather than invoking factors b (ecology) and d (geography) to inform dispersal probabilities, this study used the outcomes of the biogeographic inference to reciprocally evaluate ecological and geographical hypotheses. For example, it was speculated that interspecific competition, an ecological factor (b), has prevented mainland species from increasing the size of their ranges.
The third case study was on crevice weavers (Filistatidae), a species-poor family of cribellate spiders with global representatives (Magalhaes and Ramírez, 2022). These spiders’ diversification started in the Mesozoic and continued to date, with various clades displaying transcontinental distributions that must have been facilitated by either continental drift, or via transoceanic, long-distance dispersal. To arrive at dispersal probabilities, these authors used the plate tectonic model described above in combination with several models of the distances that these spiders can overcome by long distance dispersal. In effect, Magalhaes and Ramírez (2022) combined all the elements in Figure 1, thereby demonstrating both the relevance and the feasibility of the here proposed approach to historical biogeography.
Arachnids as biogeographical model organisms
Not only spiders, but all arachnids prominently feature in biogeographic studies. Given the old age of many arachnid lineages, empirical studies have helped to reconstruct past events as old as the breakups of Pangea and Gondwana (Boyer et al., 2007; Rix and Harvey, 2012; Xu et al., 2015; Clouse et al., 2017; Chousou-Polydouri et al., 2018) and diversification on major land masses (Chamberland et al., 2018; Esposito and Prendini, 2019; Turk et al., 2020; Ledford et al., 2021; Turk et al., 2021b). Arachnids have featured in research of evolutionary consequences of global climatic oscillations (Luo et al., 2020) and, at finer geographical scales, of glaciation events (Xu et al., 2016; Santibáñez-López et al., 2021), tectonic movements (Opatova et al., 2016), formation of rivers and mountain chains (Hedin et al., 2013; Emata and Hedin, 2016; Xu et al., 2018; Schramm et al., 2021), aridification (Abrams et al., 2019), and other biotic/abiotic events (Bond et al., 2020) as well as processes involved with subterranean colonization and diversification (Harms et al., 2018). Island biogeography has extensively utilized arachnids in empirical and synthetic research bearing on the formation of, and diversification on, island archipelagos such as Hawaii (Gillespie, 2002), the Caribbean (Čandek et al., 2019; Pfingstl et al., 2019; Crews and Esposito, 2020; Shapiro et al., 2022), the Indian Ocean islands (Agnarsson and Kuntner, 2012), or the Malay archipelago (Turk et al., 2021a; Silva De Miranda et al., 2022). Arachnological research has pioneered the use of fossil taxa in formal biogeographic analyses (Wood et al., 2013). This list is not exhaustive, merely illustrative of the diversity of biogeographic research in arachnid science.
All the above biogeographic outcomes can be revisited using better-informed dispersal probabilities within the next-generation biogeographic framework such as the one outlined here. Given an extensive fossil record and the diverse biologies of various arachnid lineages—ranging from extremely sedentary burrowers and cryptic leaf litter dwellers to species capable of aerial dispersal over thousands of kilometers (Kuntner, 2022)—, arachnids should continue to be utilized as excellent model organisms in the study of historical biogeography.
Final remarks
In this perspective, we call for more integrative approaches to biogeographic analysis. For a meaningful estimation of dispersal probabilities—an integral element of ancestral area estimation—, studies should consider organism-specific biologies, ecologies, as well as specifics of the geological and geographical histories of the relevant areas. What precisely the relevant areas and time slices are is reciprocally informed by the time-calibrated phylogeny and the taxonomic distributions of the taxa in question. Only when incorporating these elements will dispersal probabilities yield more precise ancestral areas, and thus reconstructed biogeographic histories will allow for more rigorous hypothesis tests.
Three case studies have already applied this concept to reconstructing global and regional biogeographical scenarios in spiders (Turk et al., 2020; Turk et al., 2021a; Magalhaes and Ramírez, 2022), although we freely acknowledge that not all factors in Figure 1 could be accounted for in some of these studies. As pointed above, certain limitations relate to the patchy understanding of relevant bits of organismal ecologies, and geographical factors that may have affected their dispersal. Nonetheless, similarly designed studies should apply our concept as thoroughly as feasible, depending on data availability. With a great diversity of biologies and clade ages, arachnids lend themselves as a very suitable animal group for studying historical biogeography (Kuntner, 2022). We see no reason, however, that the concept should not be applied to any other organismal group.
In conclusion, a new direction is needed in historical biogeography to effectively address some of the greatest contemporary challenges pertaining to natural and anthropogenic spread of organisms including invasive species, pathogens and their vectors, as well as habitat loss, global changes, and loss of biodiversity, or simply to reconstruct past events. Biogeography continues to thrive in the era of phylogenomics (Wen et al., 2013), but should become even more inclusive of thus far neglected, but influential aspects of organismal biology and the history of their environments.
Data availability statement
The original contributions presented in the study are included in the article/supplementary material. Further inquiries can be directed to the corresponding author.
Author contributions
All authors listed have made a substantial, direct, and intellectual contribution to the work and approved it for publication.
Funding
This work was supported by the Slovenian Research Agency (Javna agencija za raziskovalno dejavnost Republike Slovenije) (research core funding P1-0255 and P1-0236, research project J1-9163 and Ph.D. fellowship 1000-17-0618).
Acknowledgments
We have benefited from discussing our ideas with Ingi Agnarsson, Matjaž Bedjanič, Greta Binford, Jason Bond, Ren-Chung Cheng, Klemen Čandek, Matjaž Gregorič, Chris Hamilton, Marshal Hedin, Maja Kajin, Simona Kralj-Fišer, Ivan Magalhaes, David Stanković, Al Vrezec, Hannah Wood, and Kuang-Ping Yu. We thank the two reviewers for their valuable input.
Conflict of interest
The authors declare that the research was conducted in the absence of any commercial or financial relationships that could be construed as a potential conflict of interest.
Publisher’s note
All claims expressed in this article are solely those of the authors and do not necessarily represent those of their affiliated organizations, or those of the publisher, the editors and the reviewers. Any product that may be evaluated in this article, or claim that may be made by its manufacturer, is not guaranteed or endorsed by the publisher.
References
Abrams K. M., Huey J. A., Hillyer M. J., Humphreys W. F., Didham R. K., Harvey M. S. (2019). Too hot to handle: Cenozoic aridification drives multiple independent incursions of Schizomida (Hubbardiidae) into hypogean environments. Mol. Phylogenet. Evol. 139, 1–12. doi: 10.1016/j.ympev.2019.106532
Agnarsson I., Kuntner M. (2012). “The generation of a biodiversity hotspot: Biogeography and phylogeography of the Western Indian ocean islands,” in Current topics in phylogenetics and phylogeography of terrestrial and aquatic systems. (London: IntechOpen). Available at: www.intechopen.com.
Arnold P. A., Cassey P., White C. R. (2017). Functional traits in red flour beetles: the dispersal phenotype is associated with leg length but not body size nor metabolic rate. Funct. Ecol. 31, 653–661. doi: 10.1111/1365-2435.12772
Avilés L., Agnarsson I., Salazar P. A., Purcell J., Iturralde G., Yip E. C., et al. (2007). Altitudinal patterns of spider sociality and the biology of a new midelevation social Anelosimus species in Ecuador. Am. Nat. 170, 783–792. doi: 10.1086/521965
Blamires S. J. (2010). Plasticity in extended phenotypes: orb web architectural responses to variations in prey parameters. J. Exp. Biol. 213, 3207–3212. doi: 10.1242/jeb.045583
Bond J. E., Hamilton C. A., Godwin R. L., Ledford J. M., Starrett J. (2020). Phylogeny, evolution, and biogeography of the north American trapdoor spider family Euctenizidae (Araneae: Mygalomorphae) and the discovery of a new “endangered living fossil” along California’s central coast. Insect Syst. Divers. 4, 1–14. doi: 10.1093/isd/ixaa010
Bowman D. M. J. S., Brown G. K., Braby M. F., Brown J. R., Cook L. G., Crisp M. D., et al. (2010). Biogeography of the Australian monsoon tropics. J. Biogeogr. 37, 201–216. doi: 10.1111/j.1365-2699.2009.02210.x
Boyer S. L., Clouse R. M., Benavides L. R., Sharma P., Schwendinger P. J., Karunarathna I., et al. (2007). Biogeography of the world: A case study from cyphophthalmid Opiliones, a globally distributed group of arachnids. J. Biogeogr. 34, 2070–2085. doi: 10.1111/j.1365-2699.2007.01755.x
Čandek K., Agnarsson I., Binford G. J., Kuntner M. (2019). Biogeography of the Caribbean Cyrtognatha spiders. Sci. Rep. 9, 1–14. doi: 10.1038/s41598-018-36590-y
Canestrelli D., Bisconti R., Carere C. (2016). Bolder takes all? The behavioral dimension of biogeography. Trends Ecol. Evol. 31, 35–43. doi: 10.1016/j.tree.2015.11.004
Chamberland L., Mchugh A., Kechejian S., Binford G. J., Dolman G., Hamilton C. A., et al. (2018). From Gondwana to GAARlandia: Evolutionary history and biogeography of ogre-faced spiders (Deinopis). J. Biogeogr. 45, 2442–2457. doi: 10.1111/jbi.13431
Chousou-Polydouri N., Carmichael A., Szűts T., Saucedo A., Gillespie R., Griswold C., et al. (2018). Giant goblins above the waves at the southern end of the world: The biogeography of the spider family Orsolobidae (Araneae, Dysderoidea). J. Biogeogr. 46, 332–342. doi: 10.1111/jbi.13487
Clouse R. M., Branstetter M. G., Buenavente P., Crowley L. M., Czekanski-Moir J., General D. E. M., et al. (2017). First global molecular phylogeny and biogeographical analysis of two arachnid orders (Schizomida and Uropygi) supports a tropical Pangean origin and mid-Cretaceous diversification. J. Biogeogr. 44, 2660–2672. doi: 10.1111/jbi.13076
Cowie R. H., Holland B. S. (2006). Dispersal is fundamental to biogeography and the evolution of biodiversity on oceanic islands. J. Biogeogr. 33, 193–198. doi: 10.1111/j.1365-2699.2005.01383.x
Crews S. C., Esposito L. A. (2020). Towards a synthesis of the Caribbean biogeography of terrestrial arthropods. BMC Evol. Biol. 20, 12. doi: 10.1186/s12862-019-1576-z
de Queiroz A. (2005). The resurrection of oceanic dispersal in historical biogeography. Trends Ecol. Evol. 20, 68–73. doi: 10.1016/j.tree.2004.11.006
Emata K. N., Hedin M. (2016). From the mountains to the coast and back again: Ancient biogeography in a radiation of short-range endemic harvestmen from California. Mol. Phylogenet. Evol. 98, 233–243. doi: 10.1016/j.ympev.2016.02.002
Esposito L. A., Prendini L. (2019). Island ancestors and New World biogeography: A case study from the scorpions (Buthidae: Centruroidinae). Sci. Rep. 9, 3500. doi: 10.1038/s41598-018-33754-8
Esquerré D., Brennan I. G., Catullo R. A., Torres-Pérez F., Keogh J. S. (2019). How mountains shape biodiversity: The role of the Andes in biogeography, diversification, and reproductive biology in South America’s most species-rich lizard radiation (Squamata: Liolaemidae). Evol. (N. Y). 73, 214–230. doi: 10.1111/evo.13657
Fairbairn D. J., Blanckenhorn W. U., Székely T. (2007). Sex, size and gender roles. Eds. Fairbairn D. J., Blanckenhorn W. U., Székely T. (New York: Oxford University Press). doi: 10.1093/acprof:oso/9780199208784.001.0001
Fisher D. N., Haines J. A., Boutin S., Dantzer B., Lane J. E., Coltman D. W., et al. (2019). Indirect effects on fitness between individuals that have never met via an extended phenotype. Ecol. Lett. 22, 697–706. doi: 10.1111/ele.13230
Gillespie R. G. (2002). Biogeography of spiders on remote oceanic islands of the pacific: Archipelagoes as stepping stones? J. Biogeogr. 29, 655–662. doi: 10.1046/j.1365-2699.2002.00714.x
Gillespie R. G., Baldwin B. G., Waters J. M., Fraser C. I., Nikula R., Roderick G. K. (2012). Long-distance dispersal: A framework for hypothesis testing. Trends Ecol. Evol. 27, 47–56. doi: 10.1016/j.tree.2011.08.009
Guisan A., Lehmann A., Ferrier S., Austin M., Overton J. M. C., Aspinall R., et al. (2006). Making better biogeographical predictions of species’ distributions. J. Appl. Ecol. 43, 386–392. doi: 10.1111/j.1365-2664.2006.01164.x
Harms D., Curran M. K., Klesser R., Finston T. L., Halse S. A. (2018). Speciation patterns in complex subterranean environments: A case study using short-tailed whipscorpions (Schizomida: Hubbardiidae). Biol. J. Linn. Soc. 125, 355–367. doi: 10.1093/biolinnean/bly102
Hedin M., Starrett J., Hayashi C. (2013). Crossing the uncrossable: Novel trans-valley biogeographic patterns revealed in the genetic history of low-dispersal mygalomorph spiders (Antrodiaetidae, Antrodiaetus) from California. Mol. Ecol. 22, 508–526. doi: 10.1111/mec.12130
Johannesen J., Lubin Y., Smith D. R., Bilde T., Schneider J. M. (2007). The age and evolution of sociality in Stegodyphus spiders: A molecular phylogenetic perspective. Proc. R. Soc. B.: Biol. Sci. 274, 231–237. doi: 10.1098/rspb.2006.3699
Kuntner M. (2022). Phylogenomic, biogeographic, and evolutionary research trends in arachnology. Diversity (Basel). 14, 3–7. doi: 10.3390/d14050347
Kuntner M., Coddington J. A. (2020). Sexual size dimorphism: Evolution and perils of extreme phenotypes in spiders. Annu. Rev. Entomol. 65, 57–80. doi: 10.1146/annurev-ento-011019-025032
Ledford J., Derkarabetian S., Ribera C., Starrett J., Bond J. E., Griswold C., et al. (2021). Phylogenomics and biogeography of leptonetid spiders (Araneae: Leptonetidae). Invertebr. Syst. 35, 332–349. doi: 10.1071/IS20065
Lomolino M., Riddle B. R., Whittaker R. J. (2017). Biogeograhy: Biological diversity across space and time (Sunderland: Sinauer Associates).
Luo Y., Goh S. P., Li D., Gonzaga M. O., Santos A. J., Tanikawa A., et al. (2020). Global diversification of Anelosimus spiders driven by long-distance overwater dispersal and Neogene climate oscillations. Syst. Biol. 69, 1122–1136. doi: 10.1093/sysbio/syaa017
Magalhaes I. L. F., Ramírez M. J. (2022). Phylogeny and biogeography of the ancient spider family Filistatidae (Araneae) is consistent both with long-distance dispersal and vicariance following continental drift. Cladistics 38, 538–562. doi: 10.1111/cla.12505
Mainwaring M. C., Hartley I. R., Lambrechts M. M., Deeming D. C. (2014). The design and function of birds’ nests. Ecol. Evol. 4, 3909–3928. doi: 10.1002/ece3.1054
Matzke N. J. (2014). Model selection in historical biogeography reveals that founder-event speciation is a crucial process in island clades. Syst. Biol. 63, 951–970. doi: 10.1093/sysbio/syu056
McDowall R. M. (1996). Volcanism and freshwater fish biogeography in the northeastern north island of New Zealand. J. Biogeogr. 23, 139–148. doi: 10.1046/j.1365-2699.1996.00960.x
Morrone J. J., Crisci J. V. (1995). Historical biogeography: Introduction to methods. Annu. Rev. Ecol. Syst. 26, 373–401. doi: 10.1146/annurev.es.26.110195.002105
Opatova V., Bond J. E., Arnedo M. A. (2016). Uncovering the role of the Western Mediterranean tectonics in shaping the diversity and distribution of the trap-door spider genus Ummidia (Araneae, ctenizidae). J. Biogeogr. 43, 1955–1966. doi: 10.1111/jbi.12838
Pfingstl T., Baumann J., Lienhard A. (2019). The Caribbean enigma: the presence of unusual cryptic diversity in intertidal mites (Arachnida, Acari, Oribatida). Org. Divers. Evol. 19, 609–623. doi: 10.1007/s13127-019-00416-0/
Renner S. (2004). Plant dispersal across the tropical Atlantic by wind and sea currents. Int. J. Plant Sci. 165, 23–33. doi: 10.1086/383334
Rix M. G., Harvey M. S. (2012). Phylogeny and historical biogeography of ancient assassin spiders (Araneae: Archaeidae) in the Australian mesic zone: Evidence for Miocene speciation within Tertiary refugia. Mol. Phylogenet. Evol. 62, 375–396. doi: 10.1016/j.ympev.2011.10.009
Roderick G. K., Gillespie R. G. (1998). Speciation and phylogeography of Hawaiian terrestrial arthropods. Mol. Ecol. 7, 519–531. doi: 10.1046/j.1365-294x.1998.00309.x
Ronquist F., Sanmartín I. (2011). Phylogenetic methods in biogeography. Annu. Rev. Ecol. Evol. Syst. 42, 441–464. doi: 10.1146/annurev-ecolsys-102209-144710
Sanmartín I. (2012). Historical biogeography: Evolution in time and space. Evolution.: Educ. Outreach. 5, 555–568. doi: 10.1007/s12052-012-0421-2
Santibáñez-López C. E., Cushing P. E., Powell A. M., Graham M. R. (2021). Diversification and post-glacial range expansion of giant north American camel spiders in genus Eremocosta (Solifugae: Eremobatidae). Sci. Rep. 11, 22093. doi: 10.1038/s41598-021-01555-1
Schramm F. D., Valdez-Mondragón A., Prendini L. (2021). Volcanism and palaeoclimate change drive diversification of the world’s largest whip spider (Amblypygi). Mol. Ecol. 30, 2872–2890. doi: 10.1111/mec.15924
Shapiro L., Binford G. J., Agnarsson I. (2022). Single-island endemism despite repeated dispersal in Caribbean Micrathena (Araneae: Araneidae): An updated phylogeographic analysis. Diversity (Basel). 14, 128. doi: 10.3390/d14020128
Silva De Miranda G., Giupponi A. P. L., Scharff N., Prendini L. (2022). Phylogeny and biogeography of the pantropical whip spider family Charinidae (Arachnida: Amblypygi). Zool. J. Linn. Soc. 194, 136–180. doi: 10.1093/zoolinnean/zlaa101
Stevens V. M., Trochet A., van Dyck H., Clobert J., Baguette M. (2012). How is dispersal integrated in life histories: A quantitative analysis using butterflies. Ecol. Lett. 15, 74–86. doi: 10.1111/j.1461-0248.2011.01709.x
Turk E., Bond J. E., Cheng R.-C., Čandek K., Hamilton C. A., Gregorič M., et al. (2021a). A natural colonisation of Asia: Phylogenomic and biogeographic history of coin spiders (Araneae: Nephilidae: Herennia). Diversity (Basel). 13, 515. doi: 10.3390/d13110515
Turk E., Čandek K., Kralj-Fišer S., Kuntner M. (2020). Biogeographical history of golden orbweavers: Chronology of a global conquest. J. Biogeogr. 47, 1333–1344. doi: 10.1111/jbi.13838
Turk E., Kralj-Fišer S., Kuntner M. (2021b). Exploring diversification drivers in golden orbweavers. Sci. Rep. 11, 1–11. doi: 10.1038/s41598-021-88555-3
Wen J., Ree R. H., Ickert-Bond S. M., Nie Z., Funk V. (2013). Biogeography: Where do we go from here? Taxon 62, 912–927. doi: 10.12705/625.15
Whittaker R. J., Fernández-Palacios J. M., Matthews T. J., Borregaard M. K., Triantis K. A. (2017). Island biogeography: Taking the long view of nature’s laboratories. Sci. (1979). 357, eaam8326. doi: 10.1126/science.aam8326
Wiens J. J., Donoghue M. J. (2004). Historical biogeography, ecology and species richness. Trends Ecol. Evol. 19, 639–644. doi: 10.1016/j.tree.2004.09.011
Wilmshurst J. M., Anderson A. J., Higham T. F. G., Worthy T. H. (2008). Dating the late prehistoric dispersal of Polynesians to new Zealand using the commensal Pacific rat. Proc. Natl. Acad. Sci. 105, 7676–7680. doi: 10.1073/pnas.0801507105
Wood H. M., Matzke N. J., Gillespie R. G., Griswold C. E. (2013). Treating fossils as terminal taxa in divergence time estimation reveals ancient vicariance patterns in the palpimanoid spiders. Syst. Biol. 62, 264–284. doi: 10.1093/sysbio/sys092
Xu X., Kuntner M., Liu F., Chen J., Li D. (2018). Formation of rivers and mountains drives diversification of primitively segmented spiders in continental East Asia. J. Biogeogr. 45, 2080–2091. doi: 10.1111/jbi.13403
Xu X., Liu F., Cheng R. C., Chen J., Xu X., Zhang Z., et al. (2015). Extant primitively segmented spiders have recently diversified from an ancient lineage. Proc. R. Soc. B.: Biol. Sci. 282, 1–10. doi: 10.1098/rspb.2014.2486
Xu X., Liu F., Chen J., Ono H., Agnarsson I., Li D., et al. (2016). Pre-pleistocene geological events shaping diversification and distribution of primitively segmented spiders on East Asian margins. J. Biogeogr. 43, 1004–1019. doi: 10.1111/jbi.12687
Keywords: next-generation biogeography, ancestral areas, dispersal biology, geological history, vicariance, extinction
Citation: Kuntner M and Turk E (2022) Towards better-informed dispersal probabilities in historical biogeography: Arachnids as a model lineage. Front. Arachn. Sci. 1:1058676. doi: 10.3389/frchs.2022.1058676
Received: 30 September 2022; Accepted: 14 November 2022;
Published: 12 December 2022.
Edited by:
Fadia Sara Ceccarelli, Center for Scientific Research and Higher Education in Ensenada (CICESE), MexicoReviewed by:
Ivan Magalhaes, Museo Argentino de Ciencias Naturales Bernardino Rivadavia, ArgentinaJeremy Wilson, Queensland Museum, Australia
Copyright © 2022 Kuntner and Turk. This is an open-access article distributed under the terms of the Creative Commons Attribution License (CC BY). The use, distribution or reproduction in other forums is permitted, provided the original author(s) and the copyright owner(s) are credited and that the original publication in this journal is cited, in accordance with accepted academic practice. No use, distribution or reproduction is permitted which does not comply with these terms.
*Correspondence: Matjaž Kuntner, bWF0amF6Lmt1bnRuZXJAbmliLnNp