- 1Department of Biochemistry, CINVESTAV, México City, Mexico
- 2Department of Parasitology and Antimicrobial Resistance Research Center, National Institute of Infectious Diseases, Tokyo, Japan
Introduction
Eukaryotic circular RNAs (circRNAs) emerged in a common ancestor of the land-plant Arabidopsis thaliana, the fungi Saccharomyces cerevisiae and Schizosaccharomyces pombe, and the protists Plasmodium falciparum and Dictyostelium discoideum, more than a billion years ago (Wang et al., 2014). Due to their resistance to exonucleases, these molecules are very stable, and modern-day circRNAs are capable of interacting with proteins and other RNAs (Lasda and Parker, 2014), thus regulating multiple cellular mechanisms (Qu et al., 2015) ranging from cell-cell communication (Yu and Kuo, 2019) to gene expression regulation (Garcia-Lerena et al., 2022) and, together with miRNAs and mRNAs, participating in complex regulatory networks (Cao et al., 2020).
Molecular dating and species number analyses suggest that after their marine origin, some Amoebozoans colonized the land ecosystems, and others diversified with land plant radiation (Fiz-Palacios et al., 2013; Fiz-Palacios et al., 2014). Plants and amoebozoans co-evolved and interacted within these new ecosystems generating modern-day enteric Entamoeba species such as Entamoeba histolytica, which causes dysentery in humans, and E. invadens, which invades multiple tissues of reptiles (Loftus et al., 2005; Lorenzi et al., 2010; Ehrenkaufer et al., 2013; Tanaka et al., 2019). Furthermore, the parasitic E. histolytica speciation processes culminated in a very characteristic Sulfur metabolism (Jeelani and Nozaki, 2014; Mi-Ichi and Yoshida, 2019) including sulfate activation localized in mitochondria-related organelles (mitosomes), and sulfolipid metabolism pathways. The latter is crucial for the encystation of the reptilian parasite E. invadens (Jauregui-Wade et al., 2019; Jauregui-Wade et al., 2020), which is the model of choice to study amoebic differentiation so far.
Recently, 12 intronic (flicRNAs), and 748 exonic and exonic-intronic (circRNAs) circular RNAs have been identified in E. histolytica and E. invadens. In the human parasite, flicRNAs and circRNAs are differentially expressed between virulent (HM1-IMSS) and avirulent (Rahman) amoebic strains (Mendoza-Figueroa et al., 2018; López-Luis, 2022). In contrast, the reported E. invadens circRNAs correspond to 20 h encysting-induced cultures (López-Luis, 2022). As expected, in addition to strain- and encystment-specific circular RNAs, numerous circRNAs derived from genes of multiple functions were reported. We reasoned that the comparison of circular RNAs indicative of species-specific Sulfur metabolism with those indicative of previously acquired differentiation mechanisms, and with those indicative of more recently acquired parasitic behavior (virulence) could suggest their episodic origin (or repurposing) and their functional relationships.
circRNAs Are Shared in Different Metabolic and Differentiation Pathways
To evidence their probable origins, previously reported flicRNAs (Mendoza-Figueroa et al., 2018) and circRNAs were classified as follows. The 748 E. histolytica and E. invadens circRNAs previously identified (López-Luis, 2022) were compared to the 1997 genes with introns and annotated as Iron-Sulfur (Fe-S, marked with S) metabolism in the AmoebaDB. From this set, only 162 genes with two or more exons were considered because introns are required for circRNAs biogenesis through back-splicing (Liu and Chen, 2022). The common genes of this set with those whose circRNAs were detected in the virulent (HM1-IMSS; 174) and the avirulent (Rahman; 191) amoebic strains. Also, the aforementioned 162 genes were compared to the 33 genes whose circRNAs remained after curation of the set of genes corresponding to 20 h encystment-specific E. invadens (114 gene products). The curation process included the removal of the cognate RNAs shared with trophozoites (39 gene products remained) and the identification of their E. histolytica orthologs was carried out (thus, 33 gene products). Obviously, E. invadens-specific loci were not included.
A Venn diagram was used to relate the circRNA-producing genes that had at least one corresponding gene identified in the encystment circRNA library (Ei), in the species-specific Sulfur metabolism (S), or expressed in virulent (V) or avirulent (A) E. histolytica strains (Figure 1). Some of these genes also produce flicRNAs (*).
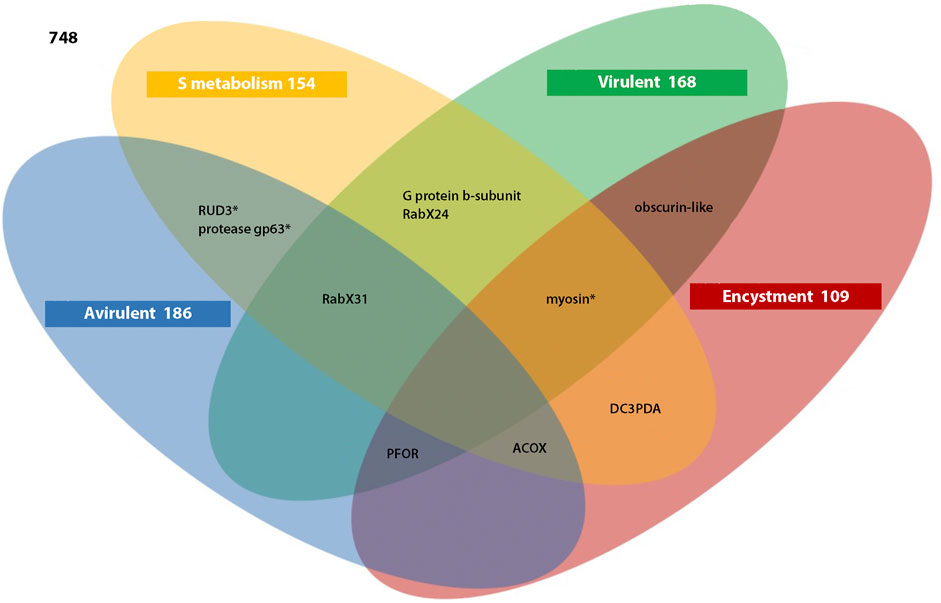
FIGURE 1. Entamoeba histolytica ncRNAs are shared in different biological processes. The 748 circRNAs and flicRNAs (*) dataset was scanned for ncRNAs from genes involved in Sulfur metabolism (162), expressed in the virulent HM1-IMSS strain (174), expressed in the avirulent Rahman strain (191), and expressed in 20 h encystment-induced E. invadens trophozoites. Encystment-specific E. histolytica circRNAs (114) were identified by subtracting circRNAs expressed in trophozoites and E. histolytica orthologs identification. G protein b-subunit, (EHI_000240), RabX24 (EHI_038680), RabX31 (EHI_040310), RUD3 (EHI_014170), pyruvate:ferredoxin oxidoreductase (PFOR, EHI_051060), acyl-CoA oxidase-like (ACOX, EHI_152970), deoxycytidine triphosphate deaminase (DC3PDA, EHI_140240), cell surface protease gp63 (EHI_042870), obscurin-like hypothetical protein (EHI_030890), and myosin (EHI_080740) were related. The genes in each intersection and the remaining figures in each category are shown.
Ten circRNA-producing genes were localized in the intersections of the three categories (two for S-A intersection; two for S-V intersection; and one each for S-E, V-E, S-A-V, S-A-E, S-V-E, and A-V-E intersections). These genes code for cell-signaling (G protein b-subunit, EHI_000240S, V), intracellular vesicle trafficking (Rab GTPases RabX24 EHI_038680S, V; RabX31 EHI_040310S, V, A; Golgi to ER transport RUD3 EHI_014,170 S, A, *), metabolic enzymes (pyruvate:ferredoxin oxidoreductase, PFOR, EHI_051060V, A, Ei; acyl-CoA oxidase-like, ACOX, EHI_152970S, A, Ei; deoxycytidine triphosphate deaminase, DC3PDA, EHI_140240S, Ei), a cell surface protease (gp63, EHI_042870S, A, *), internal membranes organization and calcium storage (obscurin-like, EHI_030890V, Ei), and cell motility (myosin EHI_080740S, V, Ei, *) proteins. The analysis of their relationships is based on their protein functions.
The circRNAs analyzed are shared between encystment and Sulfur metabolism (S-Ei). Possibly they regulate events associated to transport into mitochondria-related organelles, sulfate activation of metabolites, and metabolism of fatty acids, essential for the cyst formation (Mi-ichi et al., 2009; Mi-ichi et al., 2011; Mi-ichi et al., 2015; Mi-Ichi and Yoshida, 2019). In contrast, fewer S-Ei circRNAs are shared to maintain virulent or avirulent traits, 6 and 5, respectively, suggesting a more recent functional regulatory role.
We are aware that our nested search within the available circRNAs identified a small set of molecules to relate. For this reason, our proposal should be taken cautiously. However, most of the circRNAs analyzed here are central to the metabolic/differentiation pathways discussed.
Discussion
It is widely accepted that the first molecules in the origin of life were RNAs whose replication was DNA independent (Frias-Lasserre, 2012). This is additionally supported by the fact that circRNAs are found in the three domains of the tree of life (Villarreal, 2006). These molecules coevolved with their adaptor molecules and were either inherited or laterally transferred to accomplish existing biodiversity.
Assuming that during the evolution of Amoebozoa to E. histolytica speciation, encystment arose prior to S metabolism, and later virulent/avirulent traits emerged enabling the colonization of primate and reptilian hosts, we envisage two possible mechanisms of origin. On the one hand, circRNAs appeared to be shared to regulate different functions in two or more distinct biological processes. For example, S metabolism and non-virulence, or encystment, S metabolism, and virulence. On the other hand, it appears that the most recent functional acquisition of circRNAs involved the modification or repurposing of molecules that already regulated pathways involved in distinct biological roles.
Together, S metabolism and encystment circRNAs are species-specific molecules, possibly with regulatory functions. Since the metabolites that result from sulfate activation can be used during the encystment (Mi-ichi et al., 2009; Mi-ichi et al., 2011; Mi-ichi et al., 2015; Mi-Ichi and Yoshida, 2019), it is expected that the regulation of these two processes could be tightly linked, requiring more gene expression controls exerted in more biochemical pathways. Interestingly, some of these circRNAs are also used in more recently acquired mechanisms employed in host colonization, suggesting that to achieve host invasion, gene re- or multi-purposing might have been the preferred evolutionary routes. A simpler scenario to explain this would be that the sole activity of the proteins and gene expression regulators involved in S-Ei could suffice to successfully colonize a host, however, the fact that fewer circRNAs are shared differentially in V and A can be interpreted as a refinement of pre-existing mechanisms to regulate virulent and avirulent traits of the parasites. Definitely, there are much more genes and functions involved in the regulation of these traits than solely attributing the whole cause to 10 ncRNAs. For instance, all of these genes are present in the nonvirulent human commensal E. dispar however, in the also nonvirulent E. moshkovskii the EHI_030,890 locus has no ortholog. The circRNA produced from this locus is overexpressed in encystment and in the virulent amoeba strain. Provided that this hypothetical protein performs obscurin functions, it can be predicted that E. moshkovskii amoebas could have less efficient Ca++ storage capabilities, impaired internal membrane organization, and probably reduced capping activity as well. It will be exciting to determine the participation of this locus in amoebic virulence.
A comprehensive circRNA-silencing analysis is required to study all genes and functions regulated by the E. histolytica circRNA repertoire and to extend the search for the regulatory roles of circRNAs on amoeba transcription factors, splicing factors, and lysine and arginine methyltransferases that are involved in both nuclear and cytoplasmic functions (Medina-Gomez et al., 2021).
What molecular mechanisms might be in place to adjust particular circRNAs in the regulation of different biological processes? At the molecular level, we predict that a circRNA-miRNA-mRNA regulatory circuitry might be shared between processes, in some cases with opposite effects. For instance, the activity RabX31 circRNA involved in sulfur-metabolism could impact differentially the virulent and avirulent phenotypes. Also, the oxidative decarboxylation of pyruvate to acetyl-CoA and CO2 of PFOR is regulated by its circRNA during encystment and may have differential roles in virulence regulation. We have found evidence that virulent-specific and avirulent-specific circRNAs have complementary sites for Ehi-miRNAs and that might function as miRNA sponges (López-Luis, 2022). A possible scenario could be that an avirulent circRNA could sponge a virulent Ehi-miRNA thus blocking the inhibitory function of such Ehi-miRNA on its avirulent mRNA target; and vice versa.
In conclusion, apparently during the evolution of Entamoeba existing circRNAs might have been repurposed to regulate diverse cellular functions.
Author Contributions
Conceptualization, GG-B. and JV; Data curation, GG-B, JJ-W, TR-L, YS-N and JV; Formal analysis, GG-B, JJ-W, TR-L, YS-N, and JV; Funding acquisition, JV; Investigation, GG-B, JJ-W, TR-L, YS-N, and JV; Methodology, GG-B, JJ-W, TR-L, YS-N, and JV; Project administration, JV; Supervision, JV; Writing—original draft, JV; Writing—review and editing, GG-B and JV. All authors have read and agreed to the published version of the manuscript.
Funding
This research was funded by SEP-CINVESATAV (grant number 56), and by CONACYT México (grant CF-2019-194163), and a Postgraduate Scholarship to GG-B (730187).
Conflict of Interest
The authors declare that the research was conducted in the absence of any commercial or financial relationships that could be construed as a potential conflict of interest.
Publisher’s Note
All claims expressed in this article are solely those of the authors and do not necessarily represent those of their affiliated organizations, or those of the publisher, the editors and the reviewers. Any product that may be evaluated in this article, or claim that may be made by its manufacturer, is not guaranteed or endorsed by the publisher.
References
Cao, M., Yan, X., Su, B., Yang, N., Fu, Q., Xue, T., et al. (2020). Integrated Analysis of circRNA-miRNA-mRNA Regulatory Networks in the Intestine of Sebastes Schlegelii Following Edwardsiella Tarda Challenge. Front. Immunol. 11, 618687. doi:10.3389/fimmu.2020.618687
Ehrenkaufer, G. M., Weedall, G. D., Williams, D., Lorenzi, H. A., Caler, E., Hall, N., et al. (2013). The Genome and Transcriptome of the Enteric Parasite Entamoeba Invadens, a Model for Encystation. Genome Biol. 14 (7), R77. doi:10.1186/gb-2013-14-7-r77
Fiz-Palacios, O., Leander, B. S., and Heger, T. J. (2014). Old Lineages in a New Ecosystem: Diversification of Arcellinid Amoebae (Amoebozoa) and Peatland Mosses. PLoS One 9 (4), e95238. doi:10.1371/journal.pone.0095238
Fiz-Palacios, O., Romeralo, M., Ahmadzadeh, A., Weststrand, S., Ahlberg, P. E., and Baldauf, S. (2013). Did Terrestrial Diversification of Amoebas (Amoebozoa) Occur in Synchrony with Land Plants? PLoS One 8 (9), e74374. doi:10.1371/journal.pone.0074374
Frías-Lasserre, D. (2012). Non Coding RNAs and Viruses in the Framework of the Phylogeny of the Genes, Epigenesis and Heredity. Int. J. Mol. Sci. 13 (1), 477–490. doi:10.3390/ijms13010477
García-Lerena, J. A., González-Blanco, G., Saucedo-Cárdenas, O., and Valdés, J. (2022). Promoter-Bound Full-Length Intronic Circular RNAs-RNA Polymerase II Complexes Regulate Gene Expression in the Human Parasite Entamoeba Histolytica. ncRNA 8 (1), 12. doi:10.3390/ncrna8010012
Jáuregui-Wade, J. M., Cerbón-Solórzano, J., Ávila-García, R., Ayala-Sumuano, J. T., and Valdés, J. (2020). Ceramide Synthase 2 Knockdown Suppresses Trophozoite Growth, Migration, In Vitro Encystment and Excystment of Entamoeba Invadens. Biochem. Biophysical Res. Commun. 524 (1), 135–141. doi:10.1016/j.bbrc.2020.01.093
Jáuregui-Wade, J. M., Valdés, J., Ayala-Sumuano, J. T., Ávila-García, R., and Cerbón-Solorzano, J. (2019). De Novo synthesis of Sphingolipids Plays an Important Role during In Vitro Encystment of Entamoeba Invadens. Biochem. Biophysical Res. Commun. 508 (4), 1031–1037. doi:10.1016/j.bbrc.2018.12.005
Jeelani, G., and Nozaki, T. (2014). Metabolomic Analysis of Entamoeba: Applications and Implications. Curr. Opin. Microbiol. 20, 118–124. doi:10.1016/j.mib.2014.05.016
Lasda, E., and Parker, R. (2014). Circular RNAs: Diversity of Form and Function. RNA 20 (12), 1829–1842. doi:10.1261/rna.047126.114
Liu, C.-X., and Chen, L.-L. (2022). Circular RNAs: Characterization, Cellular Roles, and Applications. Cell 185, 2016–2034. doi:10.1016/j.cell.2022.04.021
Loftus, B., Anderson, I., Davies, R., Alsmark, U. C. M., Samuelson, J., Amedeo, P., et al. (2005). The Genome of the Protist Parasite Entamoeba Histolytica. Nature 433 (7028), 865–868. doi:10.1038/nature03291
López-Luis, M. A. (2022). Silico Identification and Characterization of circRNAs With miRNA-Sponge Potential in Virulent and Non-Virulent Strains of Entamoeba histolytica and Entamoeba invadens. Master in Science. México CDMX: Escuela Nacional de Ciencias Biológicas del Instituto Politécnico Nacional.
Lorenzi, H. A., Puiu, D., Miller, J. R., Brinkac, L. M., Amedeo, P., Hall, N., et al. (2010). New Assembly, Reannotation and Analysis of the Entamoeba Histolytica Genome Reveal New Genomic Features and Protein Content Information. PLoS Negl. Trop. Dis. 4 (6), e716. doi:10.1371/journal.pntd.0000716
Medina-Gómez, C., Bolaños, J., Borbolla-Vázquez, J., Munguía-Robledo, S., Orozco, E., and Rodríguez, M. A. (2021). The Atypical Protein Arginine Methyltrasferase of Entamoeba Histolytica (EhPRMTA) Is Involved in Cell Proliferation, Heat Shock Response and In Vitro Virulence. Exp. Parasitol. 222, 108077. doi:10.1016/j.exppara.2021.108077
Mendoza-Figueroa, M. S., Alfonso-Maqueira, E. E., Vélez, C., Azuara-Liceaga, E. I., Zárate, S., Villegas-Sepúlveda, N., et al. (2018). Postsplicing-Derived Full-Length Intron Circles in the Protozoan Parasite Entamoeba Histolytica. Front. Cell. Infect. Microbiol. 8, 255. doi:10.3389/fcimb.2018.00255
Mi-ichi, F., Makiuchi, T., Furukawa, A., Sato, D., and Nozaki, T. (2011). Sulfate Activation in Mitosomes Plays an Important Role in the Proliferation of Entamoeba Histolytica. PLoS Negl. Trop. Dis. 5 (8), e1263. doi:10.1371/journal.pntd.0001263
Mi-ichi, F., Miyamoto, T., Takao, S., Jeelani, G., Hashimoto, T., Hara, H., et al. (2015). Entamoeba Mitosomes Play an Important Role in Encystation by Association with Cholesteryl Sulfate Synthesis. Proc. Natl. Acad. Sci. U.S.A. 112 (22), E2884–E2890. doi:10.1073/pnas.1423718112
Mi-Ichi, F., and Yoshida, H. (2019). Unique Features of Entamoeba Sulfur Metabolism; Compartmentalization, Physiological Roles of Terminal Products, Evolution and Pharmaceutical Exploitation. Int. J. Mol. Sci. 20 (19), 4679. doi:10.3390/ijms20194679
Mi-ichi, F., Yousuf, M. A., Nakada-Tsukui, K., and Nozaki, T. (2009). Mitosomes in Entamoeba Histolytica Contain a Sulfate Activation Pathway. Proc. Natl. Acad. Sci. U.S.A. 106 (51), 21731–21736. doi:10.1073/pnas.0907106106
Qu, S., Yang, X., Li, X., Wang, J., Gao, Y., Shang, R., et al. (2015). Circular RNA: A New Star of Noncoding RNAs. Cancer Lett. 365 (2), 141–148. doi:10.1016/j.canlet.2015.06.003
Tanaka, M., Makiuchi, T., Komiyama, T., Shiina, T., Osaki, K., and Tachibana, H. (2019). Whole Genome Sequencing of Entamoeba Nuttalli Reveals Mammalian Host-Related Molecular Signatures and a Novel Octapeptide-Repeat Surface Protein. PLoS Negl. Trop. Dis. 13 (12), e0007923. doi:10.1371/journal.pntd.0007923
Villarreal, L. P. (2006). How Viruses Shape the Tree of Life. Future Virol. 1 (5), 587–595. doi:10.2217/17460794.1.5.587
Wang, P. L., Bao, Y., Yee, M.-C., Barrett, S. P., Hogan, G. J., Olsen, M. N., et al. (2014). Circular RNA Is Expressed across the Eukaryotic Tree of Life. PLoS One 9 (6), e90859. doi:10.1371/journal.pone.0090859
Keywords: Entamoeba histolytica, Entamoeba invadens, sulfur metabolism enzymes, encystment, virulence
Citation: González-Blanco G, Jáuregui-Wade JM, Ruiz-Luis TA, Saito-Nakano Y and Valdés J (2022) Old Circular RNAs, New Habits: Repurposing Noncoding RNAs in Parasitic Amebozoa. Front. Syst. Biol. 2:951295. doi: 10.3389/fsysb.2022.951295
Received: 23 May 2022; Accepted: 13 June 2022;
Published: 04 July 2022.
Edited by:
Juan David Ospina-Villa, Colombian Institute of Tropical Medicine (ICMT), ColombiaReviewed by:
Xinguo Chen, National Cancer Institute at Frederick (NIH), United StatesDaniel Guzman-Zapata, CES University, Colombia
Copyright © 2022 González-Blanco, Jáuregui-Wade, Ruiz-Luis, Saito-Nakano and Valdés. This is an open-access article distributed under the terms of the Creative Commons Attribution License (CC BY). The use, distribution or reproduction in other forums is permitted, provided the original author(s) and the copyright owner(s) are credited and that the original publication in this journal is cited, in accordance with accepted academic practice. No use, distribution or reproduction is permitted which does not comply with these terms.
*Correspondence: Jesús Valdés, jvaldes@cinvestav.mx