- Institute for Virology, University Hospital Essen, University Duisburg-Essen, Essen, Germany
The severe acute respiratory syndrome coronavirus 2 (SARS-CoV-2) has caused a global pandemic and public health crisis since the beginning of 2020. First recognized for the induction of severe disease, the virus also causes asymptomatic infections or infections with mild symptoms that can resemble common colds. To provide better understanding of these mild SARS-CoV-2 infections and to monitor the development of symptoms over time, we performed a detailed analysis of self-reported symptoms of SARS-CoV-2 positive and SARS-CoV-2 negative individuals. In an online-based survey, a total of 2117 individuals provided information on symptoms associated with an acute respiratory infection, 1925 of the participants had tested positive for SARS-CoV-2 infection, and 192 had tested negative. The symptoms reported most frequently during the early phases of the pandemic by SARS-CoV-2 infected individuals were tiredness, headache, impairment of smell or taste and dry cough. With the spread of the alpha and delta variants, the frequency of nose symptoms such as blocked or runny nose and sneezing increased to being reported by almost 60% of infected individuals. Interestingly, the spread of the omicron variant brought a sharp decrease in the incidence of impaired sense of smell or taste, which was reported by only 24% in this phase of the pandemic. The constellation of symptoms should be monitored closely in the months ahead, since future SARS-CoV-2 variants are likely to bring about more changes.
Introduction
The severe acute respiratory syndrome coronavirus 2 (SARS-CoV-2), the virus associated with the coronavirus infectious disease 19 (COVID-19), was first detected in Wuhan, China, at the end of 2019 (1), has quickly spread globally and has caused a pandemic and public health crisis since the beginning of 2020. The first infections in Europe occurred in Italy and Germany in late January 2020 (2). By March 2020, numbers of SARS-CoV-2 infection rose rapidly in many countries, and have done so again in multiple waves; as of May 18th 2022, more than 519 million people have been infected by SARS-CoV-2, and more than 6.2 million SARS-CoV-2 related deaths have been reported (3).
From the first cases in China, the typical symptoms of SARS-CoV-2 infection were described as dry cough, fever, and pneumonia (4, 5). These first reports of symptoms associated with SARS-CoV-2 infection/COVID-19 were based on symptoms found in hospitalized patients with severe disease. Notably, in the first reports on COVID-19, disease course was considered mild if patients did not require mechanical ventilation. It soon became obvious though that many SARS-CoV-2 infected individuals experienced asymptomatic or non-severe disease course that resembled common colds and did not require hospitalization (6). Interestingly, it was found that many SARS-CoV-2 infected patients experienced altered or completely lost sense of smell and/or taste, which has since been regarded as a key indicator of SARS-CoV-2 infection as summarized in multiple meta-analyses (7).
The high frequency of lost sense of smell and taste observed in many studies has been convincingly shown to be associated with a virus variant carrying the mutation D614G in the SARS-CoV-2 spike protein that replaced the original Wuhan Hu-1 strain as the prevalent pandemic SARS-CoV-2 variant in early 2020 (8). The variant was shown to be more infectious due to improved structural stability of the spike protein resulting in increased binding affinity to the SARS-CoV-2 receptor angiotensin-converting enzyme 2 (ACE2) (9). The improved binding to ACE2 facilitates effective infection of and replication in the olfactory epithelium, and in particular in sustentacular cells and Bowman’s gland cells as demonstrated both in the mouse model (10) and in tissue samples of deceased COVID-19 patients (11), and is regarded as mechanistically underlying the loss of smell. Similarly, enhanced ACE2 binding leads to higher infection rates of cells in the taste buds that express high levels of ACE2, leading to a disruption of normal cellular turnover from the stem cell layer and thereby to an impaired sense of taste (12). The importance of the D614G mutation for viral fitness is also demonstrated by the fact that so far, all subsequent variants of concern and variants of interest retained this mutation [reviewed in (13)]. Following variants that have spread regionally or globally all exhibited further mutations in the spike protein and in other proteins that gave the variants advantages such as increased transmissibility or infectivity, and also antibody escape to some degree (13). The most successful SARS-CoV-2 variants to date, which spread globally and largely or completely replaced other variants have been at first the G614 variant carrying the hallmark spike protein mutation D614G [Pango lineage name B.1 (14)], the alpha variant [B.1.1.7; defining mutations: Spike: N501Y, A570D, P681H, T716I, S982A, D1118H; other proteins: ORF1ab: T1001I, A1708D, I2230T; ORF8: A27*, R52I, Y73C; N: D3L, S235F; deletions: 11288:9, 21765:6, 21991:3; (15, 16)] the delta variant [B.1.617.2; defining mutations: Spike: T19R, L452R, T478K, P681R, D950N; ORF3a:S26L; M:I82T; ORRF7a: V82A, T120I; N: D63G, R203M, F377Y; (17)] and the currently dominating variant omicron [B.1.1.529; defining mutations: Spike: A67V, T95I, G339D, S371L, S373P, K417N, N440K, G446S, S477N, T478K, E484A, Q493R, G496S, Q498R, N510Y, T547K, D614G, H655Y, N679K, P681H, N764K, D796Y, N856K, Q954H, N969K; ORF1a: K856R, A2710T, T3255I, P3395H, I3758V; ORF1b: P314L, I1566V; E:T9I; M: D3G, Q19E, A63T; N:RG203KR; (18)].
To get a detailed picture of the symptoms of SARS-CoV-2 infection, we created an online questionnaire and starting from April 2020 invited both positive and negative tested individuals to report their symptoms. The symptoms included in the survey comprised general symptoms such as fever, fatigue, headache and joint or muscle pains, and a range of specific eye, nose, throat, respiratory and gastrointestinal symptoms. We were especially interested in the symptoms of infected individuals with a mild disease course, who would not be likely to seek medical care and therefore would not be easily reached in a clinical study. Our results show that over time, the pattern of symptoms reported by SARS-CoV-2 positive individuals changed significantly, with nose symptoms becoming more common since the rise of the alpha and delta variants, and the typical loss of smell and taste becoming far less prevalent with the rise of the omicron variant. Our data show that continued close monitoring of SARS-CoV-2 symptoms in the future is clearly warranted.
Materials and Methods
Ethics Approval
The study was approved by the local Ethics Committee of the Medical Faculty of the University Duisburg-Essen (approval number 20-9233-BO) and was carried out in accordance with the ethical guidelines and regulations. The study participants gave their informed consent to their voluntary participation in this study and to the subsequent use of the data for publication.
Data Collection
Data were collected in an online questionnaire based on LimeSurvey software hosted on the servers of the University of Duisburg-Essen. Participants of the online survey were recruited via public health offices of the city of Hamm (North Rhine-Westphalia, Germany), of the administrative district Soest (North Rhine-Westphalia, Germany), of the administrative district Hochsauerland (North Rhine-Westphalia, Germany) and the SARS-CoV-2 testing center in Lünen, administrative district Unna (North Rhine-Westphalia, Germany), as well as via social media. Participants were invited to complete the survey in case of a positive as well as negative SARS-CoV-2 test result. The data presented in this manuscript were collected between April 6th 2020 and May 17th 2022.
Data Analysis
Data were analyzed using R (version 3.6.3) and RStudio software and fsmb, plyr, splyr, tidyverse, ggplot2 and viridis packages. Only data from individuals who had completed the survey were included in the analysis.
For graphic representation of the survey results of tested individuals, data were sorted by the date of survey completion and the category “fever higher than 38.0°C” was calculated from the questionnaire responses using the tidyverse package, and a heatmap of the survey data was generated using ggplot2 package.
Odds ratios (OR) for individual symptoms were calculated as the conditional maximum likelihood estimate with 90% confidence interval using the function fisher.test of R stats package. OR and frequency of symptoms were visualized using ggplot2.
Statistical significance in symptom frequency in vaccinated and unvaccinated individuals was calculated by Fisher’s exact test using the function fisher.test of the R stats package.
Results
To obtain an overview of the subjective symptoms reported by SARS-CoV-2 infected individuals, especially those with a mild disease course, an online survey was performed. Invitations to the survey were published on social media, and SARS-CoV-2 tested individuals were also directly invited to participate when they received notification of their test results, or by subsequent invitation, by their local public health offices. Participants included individuals who had tested positive or negative for SARS-CoV-2 by PCR. Since the survey was performed in German language, participants from social media are expected to be mainly from Germany, or from neighbouring German-speaking countries.
In total, responses of 2117 participants who had been tested for SARS-CoV-2 infection were included in the survey, 1925 (90.1%) had tested positive for SARS-CoV-2 infection, while 192 (9.1%) of those participants had tested negative. The characteristics of the whole study population are represented in Table 1. The SARS-CoV-2 positive-tested individuals were grouped according to the time of infection, with the time period April 2020 to January 2021 being dominated by the G614 variant SARS-CoV-2 in Germany, the SARS-CoV-2 alpha variant being the dominant circulating virus strain from February 2021 to June 2021, the SARS-CoV-2 delta variant from July 2021 until the first week of January 2022, and the SARS-CoV-2 omicron variant from the second week of January until the end of data collection on May 17th 2022 (19). The number and baseline characteristics of the participants from these four variant phases were comparable, with roughly two third of participants being female and the majority of participants between 18 and 69 years of age, although there was a higher frequency of participants in the 50 – 59 years of age group in the G614 dominated early phase of the pandemic compared to the alpha, delta and omicron dominated phases (Table 1).
Of the SARS-CoV-2 positive-tested individuals, only a minority (G614: 7.6%, alpha: 5.8%, delta: 1.2%, omicron: 5.1%) reported no symptoms, whereas the majority reported mild (G614: 43.5%, alpha: 48.7%, delta: 50.4%, omicron: 55.4%) or moderate symptoms (G614: 30.5%, alpha: 33.9%, delta: 34.6%, omicron: 32.0%). A small number of the participants had been hospitalized (G614: 4.9%, alpha: 2.5%, delta: 0.5%, omicron: 0.5%) or had required intensive care treatment (G614: 1.2%, alpha: 0.0%, delta: 0.0%, omicron: 0.0%) due to the SARS-CoV-2 infection.
The symptoms reported by the tested study population are shown as a heatmap in Figure 1, and a direct comparison of the frequencies of symptoms in the different phases of the pandemic is facilitated by the bar graphs in Figure 2. Clearly, a majority in both SARS-CoV-2 positive and negative individuals reported general symptoms such as tiredness, lethargy and headaches. The frequency of fever higher than 38.0°C decreased from 35.7% of respondents in the G614-dominated phase to 20.7% in the alpha-dominated phase, 17.9% in the delta-dominated and 16.6% in the omicron-dominated phase. On the other hand, during the G614-dominated phase, 52.5% of survey participants reported headache as a symptom, and that increased further to 64.5% for the alpha-, 67.4% for the delta-, and 65.7% for the omicron-dominated phase. Also vertigo saw an increase from 20.7% for G614 to 29.3% and 27.3% for alpha and delta, respectively, but went down to 24.1% for omicron. Lethargy, muscle pain and loss of appetite were reported less frequently in the omicron-dominated phase.
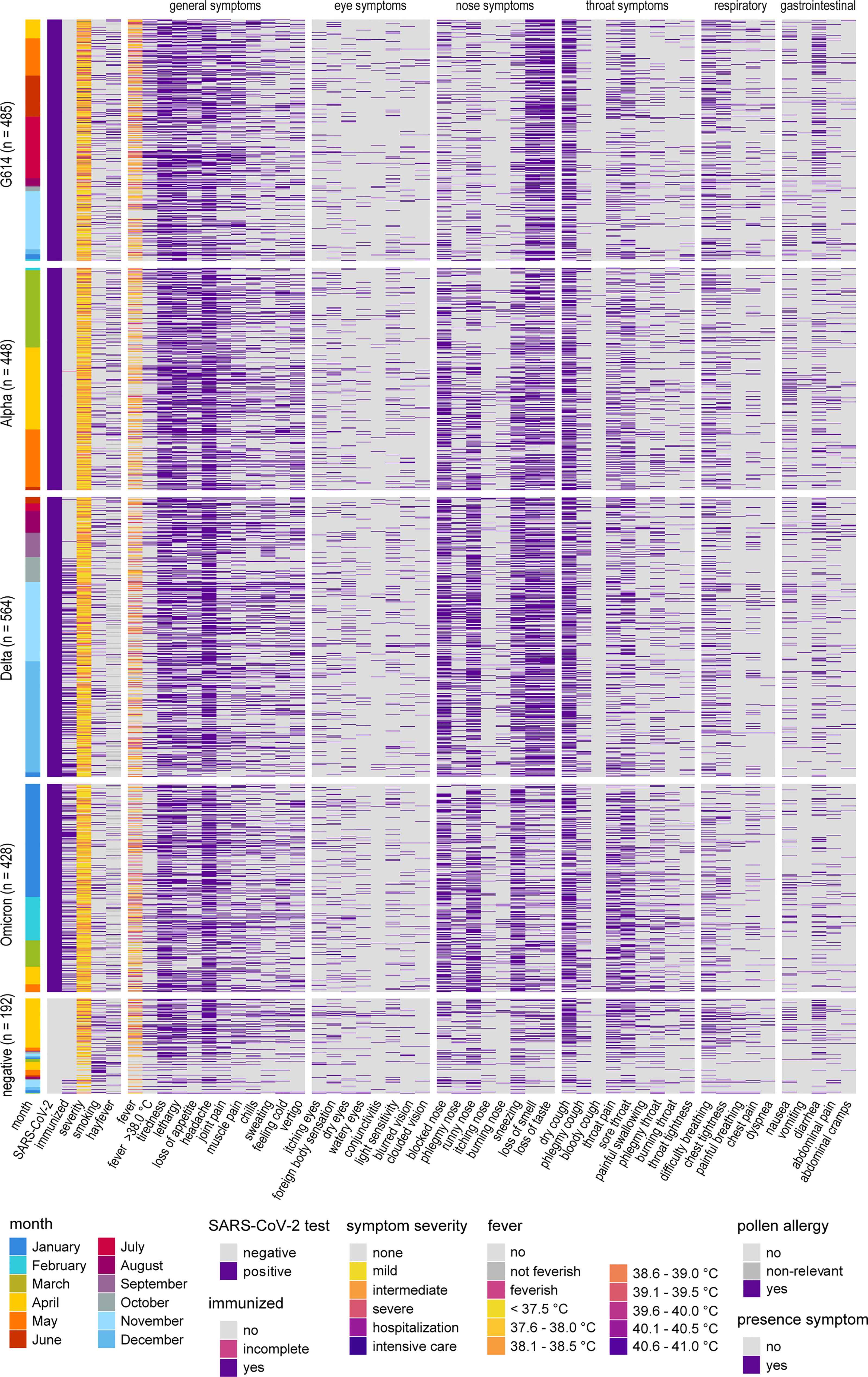
Figure 1 Heatmap of symptoms reported by SARS-CoV-2 positive and negative tested individuals. Symptoms reported by SARS-CoV-2 positive (4 top sections) and SARS-CoV-2 negative individuals (bottom). Subjects were sorted according to SARS-CoV-2 test outcome and date of questionnaire completion; groups of positive subjects are displayed according to periods of dominance of SARS-CoV-2 G614 and the alpha, delta and omicron variants. “Month” indicates the date of completion of the questionnaire. “feverish/not feverish”: subjective judgement, participant did not measure their body temperature.
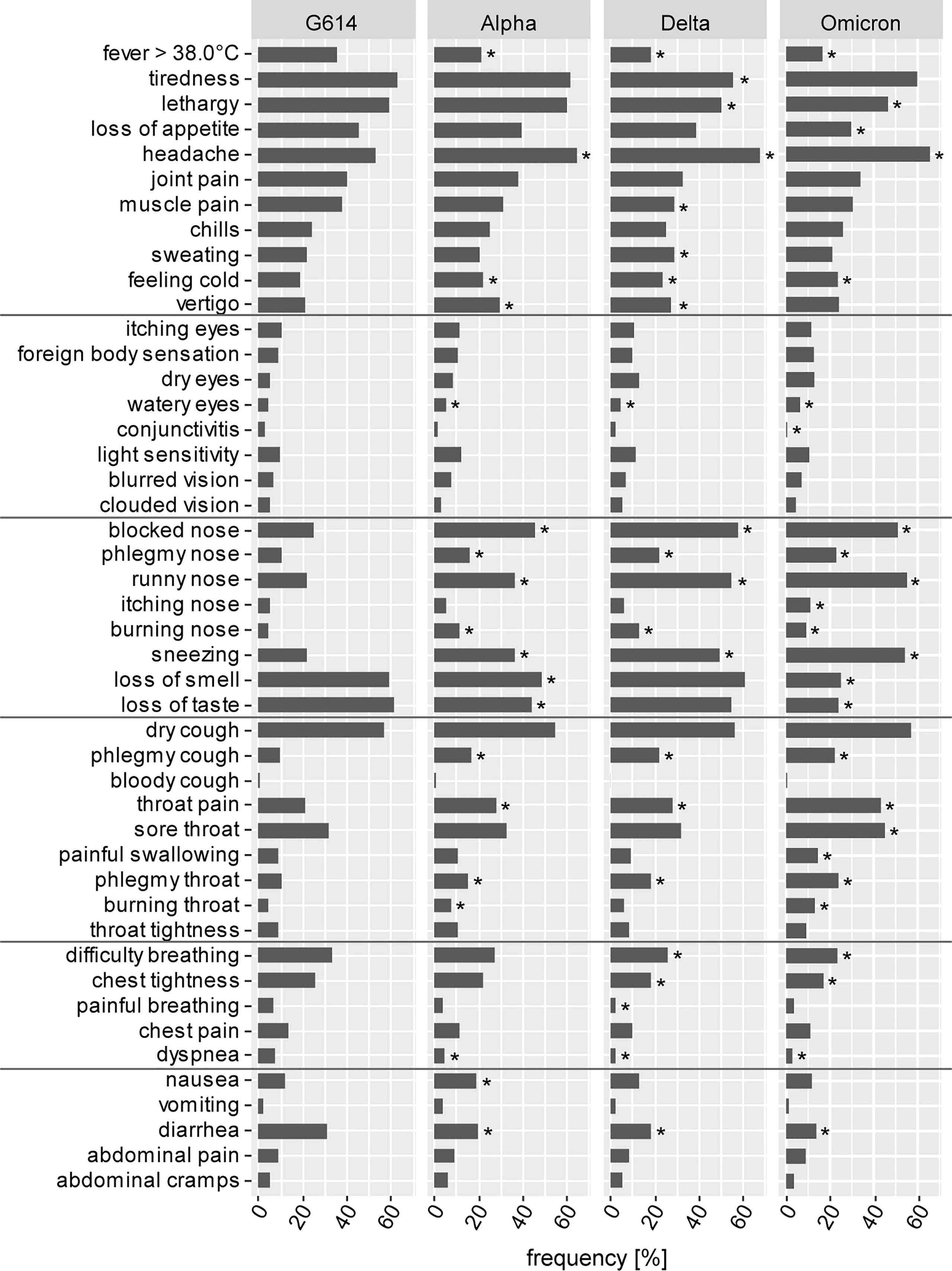
Figure 2 Frequencies of symptoms. Frequencies for each symptom were calculated for SARS-CoV-2 positive individuals from G614, alpha variant, delta variant and omicron variant dominated phases of the SARS-CoV-2 pandemic. Statistically significant differences compared to the frequencies in the G614 phase are indicated by * (p < 0.05, Fisher’s exact test).
The presence of eye symptoms has been low throughout all phases of the pandemic and there have not been considerable changes over the time of data collection. Interestingly, most nose symptoms were reported only by a minority of participants during the early phase of the SARS-CoV-2 pandemic, but the frequency of respondents reporting a blocked or runny nose or sneezing has increased considerably since the spread of the alpha, delta and omicron variant: while only 24.4% and 21.8% reported a blocked or runny nose for G614 infections, this increased to 45.1% and 36.2% for alpha variant infections, 57.6% and 54.8% for delta variant infections and 50.9% and 55.1% for omicron variant infections. The proportion of sneezing increased from 21.8% for G614 to 36.1% for alpha, 49.3% for delta and 54.2% for omicron. Importantly, there have been changes to the symptoms that have been regarded as highly typical of a SARS-CoV-2 infection since early days of the pandemic, which is loss of smell or taste. About two thirds of SARS-CoV-2 positive individuals reported an impaired or lost sense of smell or taste during the G614, alpha and delta dominated phases, however, these frequencies have dropped considerably to only 25% and 23.8%, respectively, for the current omicron variant dominated phase (Figure 2).
Dry cough has been another hallmark symptom of SARS-CoV-2 infection and was reported by almost 60% of SARS-CoV-2 positive individuals, and this frequency remained largely unchanged for the variant-dominated phases. Other throat symptoms had been reported at far lower frequencies during the G614 phase but have increased significantly with the spread of the alpha, delta and omicron variants, most notably throat pain, sore throat and also phlegmy throat and phlegmy cough. Approximately one third of individuals reported difficulty breathing and other respiratory symptoms during the G614-dominated phase of the pandemic (31.3%), and this frequency has also dropped since the spread of the delta and even more so the omicron variant, with the frequency of individuals reporting difficulty breathing now down to 23.4%.
Also gastrointestinal symptoms have been associated with SARS-CoV-2 infections since the beginning, although not reported by a majority of infected individuals. The most often reported gastrointestinal symptom in the G614-dominated early phase of the pandemic was diarrhea (28.2%), which has decreased in frequency in the variant-dominated phases (alpha: 19.4%. delta: 17.9%, omicron: 13.8%).
The changes in symptom frequencies are reflected in the odds ratios for the individual symptoms (Figure 3). Since the distribution of many symptoms was similar in SARS-CoV-2 positive and negative individuals, many odds ratios are close to 1. Clearly, the highest odds ratios were observed for impaired or lost sense of smell in the earlier G614, alpha and delta phases (estimate: 3.8; 90% confidence interval (CI): 2.5 – 6.1 for G614; alpha: 2.7, CI 1.7 – 4.3; delta: 4.5, CI 2.9 – 7.0) and impaired or lost sense of taste (G614: 4.3, CI 2.8 – 6.8; alpha: 2.2, CI 1.4 – 3.5; delta: 3.6, CI 2.4 – 5.7), showing only a very slight decline with the appearance of the alpha and delta variants. For the omicron-dominated phase however, these numbers have dropped considerably to only 1.0 (CI 0.6 – 1.6) and 0.9 (CI 0.6 – 1.5), respectively.
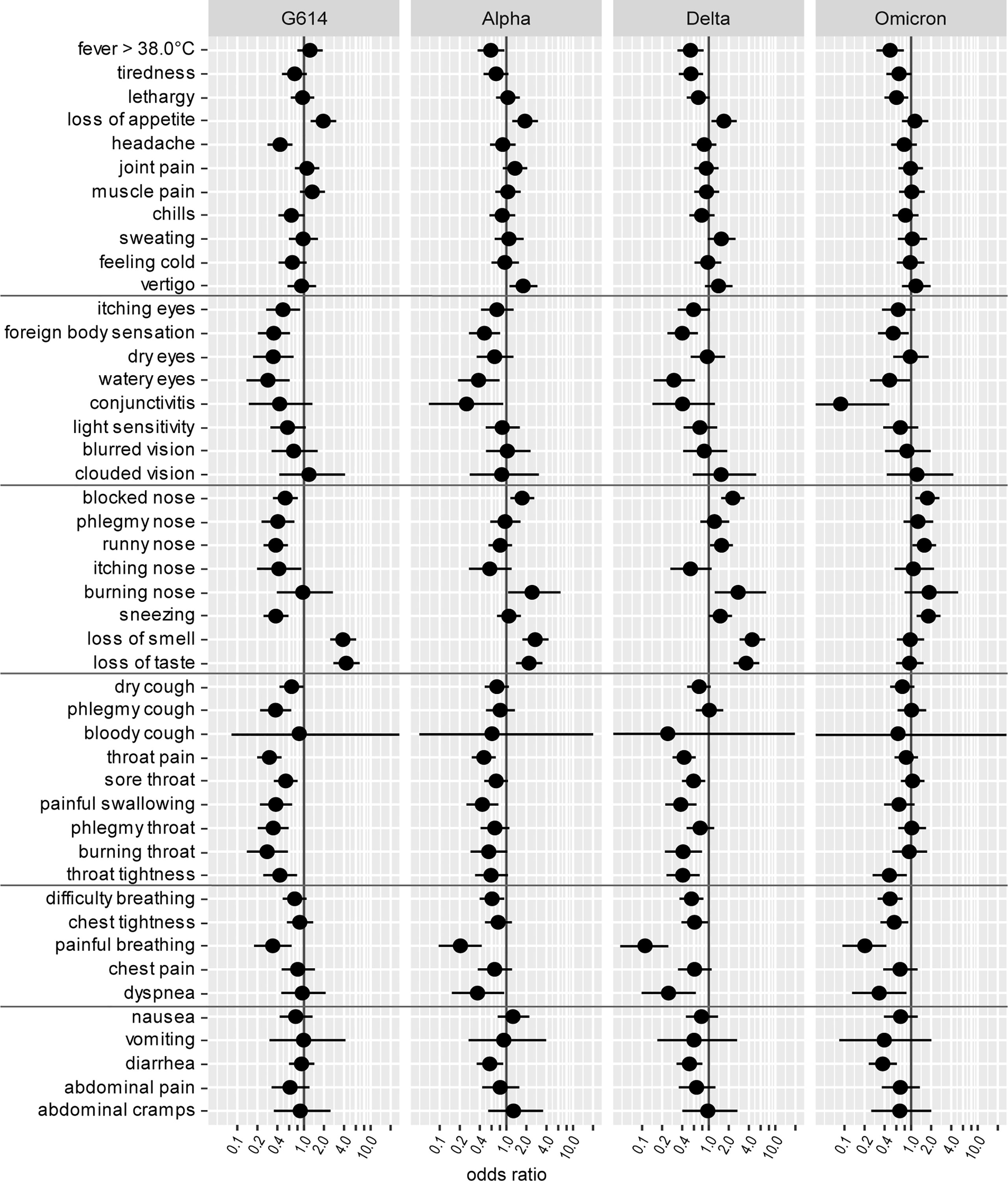
Figure 3 Odds ratios of symptoms. Odds ratios of symptoms and 90% confidence intervals were calculated from frequencies of symptoms in SARS-CoV-2 positive individuals from the G614, alpha variant, delta variant and omicron variant dominated phases of the SARS-CoV-2 pandemic in relation to frequencies of symptoms in symptomatic SARS-CoV-2 negative individuals.
Other notable differences were observed for blocked nose (G614: 0.5, CI: 0.3 – 0.8; alpha: 1.7, CI 1.1 – 2.6; delta: 2.3, CI 1.5 – 3.4; omicron 1.7, CI 1.2 – 2.7), runny nose (G614: 0.4, CI: 0.2 – 0.6; alpha: 0.8, CI 0.5 – 1.2; delta: 1.5, CI 1.0 – 2.3; omicron: 1.6, CI 1.1 – 2.4) and sneezing (G614: 0.4, CI: 0.2 – 0.6; alpha: 1.1, CI 0.7 – 1.6; delta: 1.5, CI 1.0 – 2.2; omicron: 1.8, CI 1.2 – 2.8), all turning from an OR below 1 for G614 to an OR well above 1 for the variants. The increased frequencies of runny nose and sneezing symptoms observed for the variants are not due to a coincidence with hayfever seasons, as the changes can be clearly observed for participants with no reported hayfever or with hayfever that was not relevant at the time of infection (Figure 4).
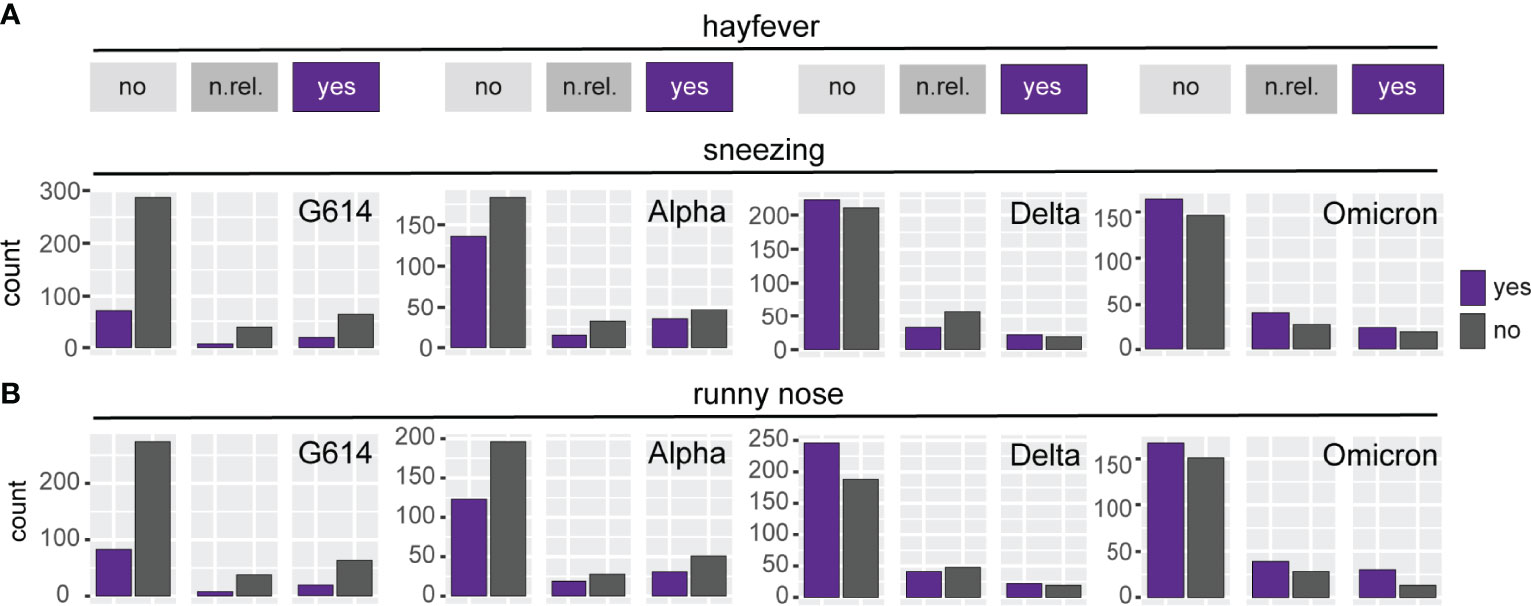
Figure 4 Sneezing and runny nose symptoms stratified by hayfever. Counts of respondents indicating presence or absence of the symptom “sneezing” (A) or “runny nose” (B). Respondents were grouped according to their suffering from hayfever (“yes”), not suffering from hayvever (“no”), or suffering from hayfever but not during the time of SARS-CoV-2 infection (“n.rel.”: not relevant). The increase in frequency of the symptoms “sneezing” and “runny nose” in the variant dominated phases can be clearly observed in individuals who do not suffer from hayfever.
It is important to note that the differences in symptoms reported by participants in the current, omicron-dominated phase are not due to vaccination: of the 428 participants in the omicron phase, 164 had been fully vaccinated at the time of infection, while 264 had been unvaccinated, and the frequencies of symptoms were largely the same in vaccinated and unvaccinated individuals (Figure 5). Of note, the only symptoms where we found a statistically significant difference in frequency between vaccinated and unvaccinated individuals are foreign body sensation in the eyes and loss of smell, where the frequencies were lower in unvaccinated than in vaccinated individuals (foreign body sensation: 4.2% vs. 9.8%; loss of smell 20.5% vs. 32.3%).
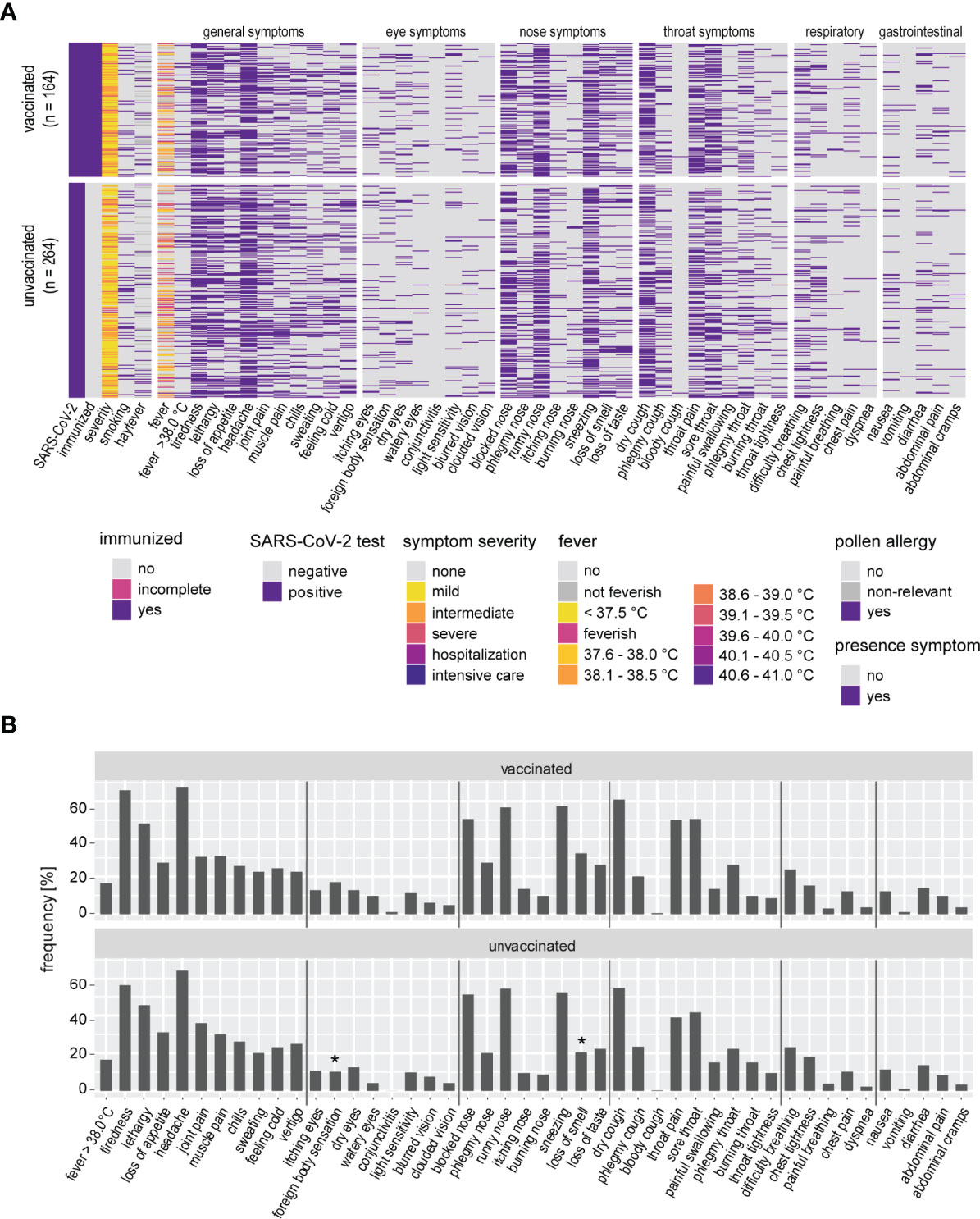
Figure 5 Symptoms reported during the omicron-dominated phase stratified by vaccination status. (A) Heatmap of SARS-CoV-2 infected vaccinated (upper section) and unvaccinated (lower section) individuals from the omicron-dominated phase. (B) Frequencies for each symptom were calculated for vaccinated and unvaccinated SARS-CoV-2 positive individuals. Statistically significant differences are indicated by * (p < 0.05, Fisher’s exact test).
A comparison with another detailed symptom study from the delta variant dominated phase by Vihta et al. reveals an interesting difference in the frequency of fever, which was reported to be increased for delta in comparison to G614 in a large community level cohort in the United Kingdom (20). We therefore subjected our fever data to a more detailed analysis (Figure 6). The decrease in frequency can be most strongly observed for the temperature range between 37.6°C and 39.0°C, which were most frequently reported in the G614 dominated phase (Figure 6A). It is noteworthy, however, that in the alpha and more so in the delta and omicron dominated phases, the frequency of respondents who did not measure their body temperature and replied by “I felt feverish, but didn’t measure my body temperature” or “I didn’t feel feverish, but didn’t measure my body temperature” increased, introducing higher uncertainty into the fever data for the later phases. Of note, “feverish” was not included in the “fever > 38.0°C” category. This increase in the “feverish” and “not feverish” replies cannot be solely attributed to the fact that a larger proportion of young people were infected in the variant phases – the frequency of infected people below 50 years of age increased from 50.1% in the G614 phase to 65.4%, 64.5% and 68.0% in the alpha, delta and omicron dominated phases, respectively - since these replies increased similarly across all age groups in the variant phases (Figure 6B).
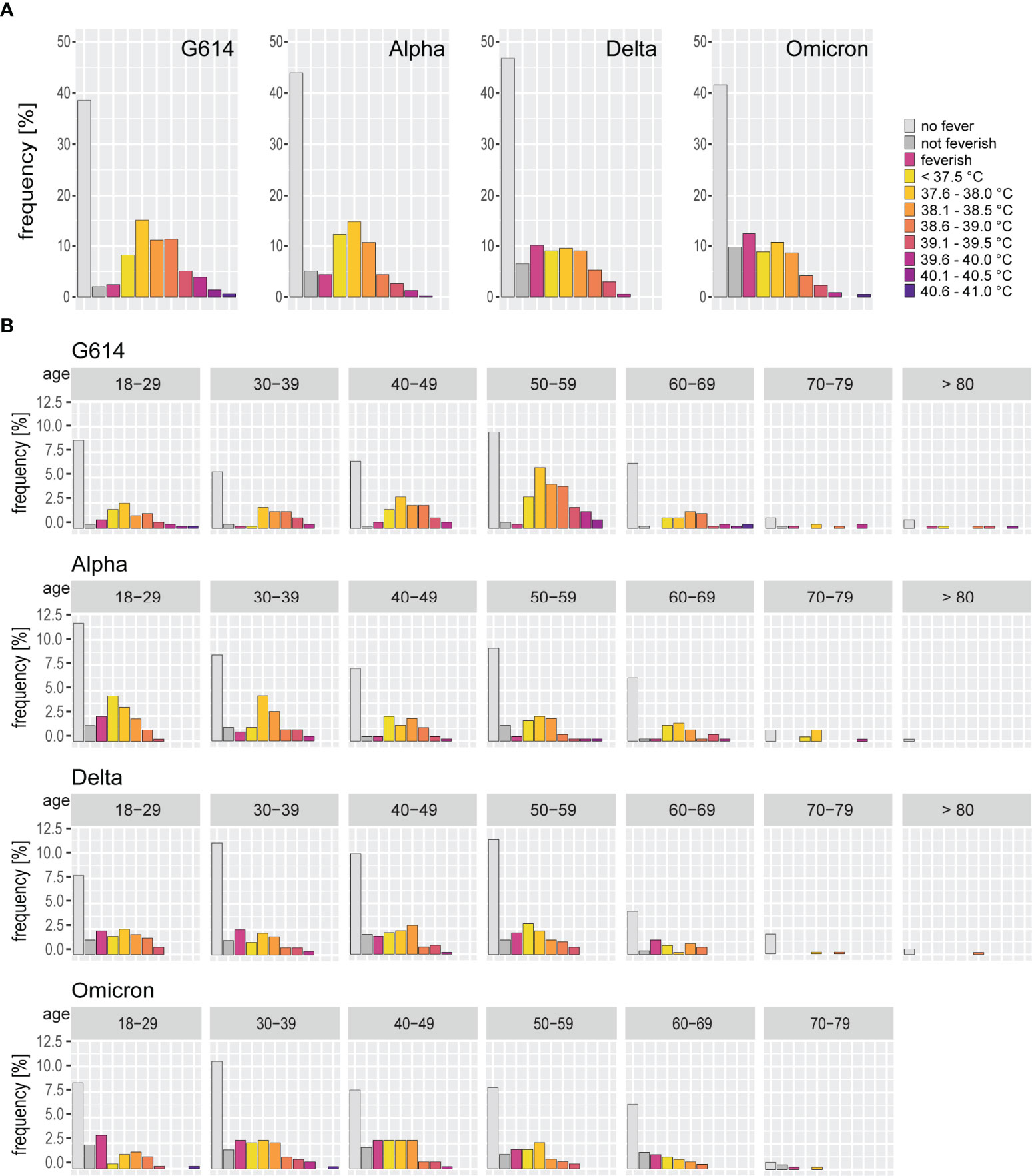
Figure 6 Detailed fever data stratified by SARS-CoV-2 variants and age groups. (A) Frequency of the fever levels reported by participants in the SARS-CoV-2 G614, alpha, delta and omicron dominated phases of the pandemic. (B) Frequency of fever levels reported by the participants in the SARS-CoV-2 G614, alpha, delta and omicron variant dominated phases stratified by age groups. The frequency of participants replying with “feverish” who had not measured their body temperature increased in the variant phases, introducing more uncertainty into the fever data. However, the frequency of participants reporting no fever or not feeling feverish increased, suggesting a reliable decrease in fever induced by the SARS-CoV-2 variants.
Discussion
The data presented here provide a detailed picture of the symptoms experienced by SARS-CoV-2 infected individuals, and of the changes in symptom patterns that have occurred with the spread of the alpha, delta and omicron variants.
The data collected in this survey show that a range of symptoms is observed in a majority of patients, such as tiredness, lethargy, loss of appetite, joint or muscle pains, headache and dry coughing. Since the delta variant became dominant, also sneezing and blocked or runny nose were reported by almost 60% of infected individuals. For some symptoms however, we found surprisingly low frequencies in infected individuals and therefore low odds ratios, including all throat symptoms other than dry cough. Furthermore, only roughly one fifth of SARS-CoV-2 infected individuals experienced throat pain during the earlier phases, which could be expected to be much higher since virus is routinely identified in throat swabs, showing that it is indeed infecting throat tissue. However, the frequency of these throat symptoms has increased in the current omicron-dominated phase. While an impaired or lost sense of smell or taste had been a hallmark symptom of SARS-CoV-2 infection that was identified early in the pandemic (21, 22), the spread of the omicron variant has led to a significant decline in frequency.
For symptoms reported from the first few months of the G614-dominated beginning of the pandemic, a bias may have been introduced into the dataset since some symptoms had been described early in the pandemic as typical for SARS-CoV-2 infection, and had therefore been criteria for testing while testing capacity was still limited. However, there was no apparent change in symptom patterns when testing capacities in Germany had become sufficient in early summer of 2020 to test all patients with respiratory symptoms, therefore, the frequencies reported for the G614 phase can be expected to be accurate. It should be noted that replies were sorted by the date of questionnaire completion, which may have introduced a slight offset in the allocation to the alpha-, delta- and omicron-dominated phases.
Other studies have been performed investigating symptoms of SARS-CoV-2 and their predictive value. Studies in the early days of the COVID-19 pandemic were reporting symptoms of hospitalized patients and thus missed the symptoms that are associated with mild disease courses (4, 5, 23). Since then, multiple other studies have been performed that focused on the symptoms experienced by SARS-CoV-2 infected individuals at a community level (22, 24–29). A large study has been performed during the first phase of the pandemic using a mobile phone app that included data from more than seven thousand participants who tested positive for SARS-CoV-2 in a cohort of more than 2 million people from the United Kingdom and the United States (28). In this study, the authors found frequencies for the symptoms “loss of smell and taste”, “fever”, “skipped meals” and “diarrhea” that are comparable to the frequencies reported by us and reported positive odds ratios for these symptoms, with the highest odds ratio for “loss of smell and taste”. The authors report a similar frequency of “persistent cough” in the SARS-CoV-2 positive individuals as we found for “dry cough”; in contrast to our data, the frequency of “dry cough” in SARS-CoV-2 negative individuals was lower than in our cohort, leading to a low positive odds ratio for the persistent cough, in contrast to our findings for the G614 phase.
A further large-scale study in the United Kingdom revealed similar changes in the symptoms for the delta variant as we have observed in our study, with the remarkable exception that the authors report an increase in fever for the delta variant compared to G614 (30). Our data show that the incidence of fever higher than 38.0°C decreased to below 20% for delta, compared to more than 35% for G614. In contrast to our survey, the UK survey asked participants if they had experienced fever during their SARS-CoV-2 infection, but did not ask for specific temperatures or specify a lower threshold for fever (personal communication). This could partly explain the difference between the survey outcomes, even though we would still see a slight increase in respondents who replied “no fever”, or a bit more pronounced in those who replied either “no fever” or “not feverish” from the G614 phase to the variant dominated phases, indicating that there is a true decline in our data. Vihta et al. also suggested that non-white ethnic groups were more likely to report fever than white ethnic groups (30), whether this explains the difference to our findings cannot be estimated since we do not have any information on ethnicity of our participants.
A subsequent large-scale study using the above mentioned mobile phone app analyzed data collected from 63,002 participants in the United Kingdom during the delta and omicron variant dominated phases of the pandemic (31). While many symptoms were similar in frequency during the delta and omicron phases, they also report a significant drop in the frequency of altered smell and more prominently in the frequency of loss of smell, which dropped from 50% during the delta dominated phase to below 20% during the omicron dominated phase, which is very similar to our findings. Interestingly, the frequency of many symptoms such as joint pains, muscle pains, runny nose, sneezing, nausea and diarrhea is higher in this report than in our data, for many of those symptoms by about 10%. A reason for the higher rates might be the daily reporting of symptoms in the mobile phone app, which could be leading to higher reporting rates.
A reduced frequency of loss of smell or taste in the omicron variant dominated phase as we have seen in our survey has also been described in some other reports, interestingly, the frequency in some reports was even lower than in our study, ranging from only 1.2% in a study in Jordan (32) and 2.5% in a study in Korea (33) to 8% in a study in the US (34) and 12% (loss of smell) and 23% (loss of taste) in a study in Norway (35). Loss of smell and taste was reported to be down to 13% in a technical briefing by the United Kingdom Health Security Agency (36); this report was based on the very large number of 182,133 individuals, but as a limitation of this analysis it was indicated that the symptom data was collected early, usually 3 to 4 days, after symptom onset. Very similar frequencies of impaired or lost sense of smell or taste in infected individuals were reported from studies in Italy (37), the Faroe Islands (38), the mobile phone app study mentioned above (31) as well as a community study in the United Kingdom (20). In the latter report from a large community study in the United Kingdom a similar drop in the frequency of loss of smell and taste as we have found in our survey was demonstrated, corroborating our observation. Interestingly, the authors also report a somewhat higher rate of loss of smell and loss of taste as well as of coughing in vaccinated compared to unvaccinated individuals, suggesting that this finding may not be due to an unknown bias but warrants further scrutiny. Of course, the higher frequency in vaccinated individuals found in our study might also be due to a reporting bias, since symptomatic vaccinated individuals, especially those with more pronounced symptoms, might be more motivated to participate in the survey.
The SARS-CoV-2 variants have been fully characterized on the genomic level, and some of the characteristic mutations readily lend themselves for an explanation of the observed changes in symptoms of infected individuals that occurred with the spread of the variants. As described above, a hallmark of the first globally spread SARS-CoV-2 was the mutation D614G, which was retained in later variants (see (13) for a detailed review of SARS-CoV-2 variants with a focus on the spike protein). This variation has been shown to reduce spike S1 subunit shedding (39–44), to stabilize the spike pre-fusion conformation (45–48), and to thereby increase infectivity and transmission due to high ACE2 expression levels in the upper respiratory tract (8, 49). Similarly, the additional N501Y mutation present in the alpha and omicron variants has been shown to directly strengthen the interaction of the SARS-CoV-2 RBD with ACE2 (50, 51). Similarly, mutations in the furin cleavage site (alpha and omicron: P681H; delta: P681R) can lead to increased cleavage of the S1 and S2 spike protein subunits, resulting in higher infectivity and transmissibility (52, 53). A high binding affinity to ACE2 and high fusogenicity of the spike proteins explains the efficient infection of sustentacular cells of the olfactory epithelium and of taste bud cells, which are thought to mechanistically underlie the loss of smell and taste in a large proportion of infected individuals (10, 11). Since the first affinity-enhancing mutation D614G was introduced into the orinial Wuhan Hu-1 strain after its spread into other countries, this may well explain why loss of smell and taste was not observed as a symptom during the earliest phase of SARS-CoV-2 spread in China. While it has been suggested in a large meta-analysis that ethnicity also plays a role in susceptibility of infected individuals for loss of smell and taste, and that individuals of Asian origin are less prone to it (7), viral factors appear to have a much larger role than host factors (54). In comparison to alpha and delta, omicron harbors even more mutations in the spike protein including regions of the RBD involved in ACE2 binding and around the furin cleavage site, and has been shown to have high ACE2 binding affinity (55). Interestingly, however, omicron exhibits an inefficient use of the cellular protease TMPRSS2, which is required for proteolytic cleavage of the spike protein at the S2’ site to liberate the fusion peptide, and relies more strongly on the endosomal uptake route (56), suggesting that ACE2/TMPRSS2-expressing cells would no longer be as strongly preferred target cells. This would explain the lower frequency of loss of smell and taste with the omicron variant. Sustentacular cells of the olfactory epithelium have been shown to express high levels of both ACE2 and TMPRSS2 (57), making them direct target cells of the previous SARS-CoV-2 variants as demonstrated both in the mouse model (10) and in human tissue (11). It could be shown in vivo in the hamster model that in comparison to G614 and delta, the infection of the olfactory epithelium by the omicron variant was markedly reduced and infection of nasal tissue shifted to the respiratory epithelium (58), which is in accordance with the significantly reduced frequency of loss of smell in omicron infected individuals shown in this study as well as by others.
The mechanism underlying the reduced induction of fever by the SARS-CoV-2 variants is less clear, and possibly more complex and involving proteins other than spike. It has been shown previously that SARS-CoV-2 infection induces cytokines such as interleukin 6 and tumor necrosis factor α (59) that are known mediators of fever. It was shown in the mouse model that the spike protein alone could induce production of these cytokines (60), and it was demonstrated in vitro that monocytes produced these cytokines when stimulated with the S1 spike subunit alone (61). Unfortunately, no comparative analysis with the different variants has been performed in these studies, since they were performed earlier in the pandemic. An in vivo mouse study showed a differential induction of cytokines by the delta and omicron variants in comparison to an early G614 isolate (62); and similarly, an in vivo study in hamsters showed reduced levels of a range of cytokines in omicron infected compared to delta infected animals (63), suggesting that the variants are indeed inducing different cytokine levels in vivo. One study has analyzed various SARS-CoV-2 proteins for the presence of cytokine-inducing peptides and found significant contribution of proteins other than spike to the cytokine response, which was found to be slightly reduced for omicron in comparison to Wuhan Hu-1, gamma (B.1.1.28.1) and delta derived proteins (64). Furthermore it has been shown in in vitro studies that omicron is more susceptible to inhibition by type I interferons and induces higher levels of type I interferons than delta (65, 66), which may also play a role in the development of fever. While no direct comparison was made, the authors suggested an increased susceptibility of the omicron variant to interferon, which may be associated with mutations in proteins other than spike, namely variations in the interferon antagonizing proteins non-structural protein 3 (nsp3), nsp12, nsp13, nsp14, M, nucleocapsid and ORF3a (65, 66).
Our study shows interesting changes of symptoms of SARS-CoV-2 infection over time, it does however have a number of limitations that need to be mentioned: since the survey was conducted as an online questionnaire, the participants were likely biased towards younger people, even though we know of instances where younger relatives assisted elderly participants. While the frequency of participants of the 50-59 years of age group was higher in the G614 dominated phase than in the alpha, delta and omicron dominated phases, the participants of the survey from the four phases were largely comparable with regard to age and gender distribution. It has to be noted, however, that there was a far larger proportion of female than male participants of the survey in all four phases of the pandemic. Since the main focus of the presented study was the comparison of the symptoms observed during the different variant dominated phases, this bias should be noted but does not hinder the interpretation of the data as presented here. Furthermore, even though we also saw participants who had been hospitalized or had even required intensive care treatment, we were aware from the beginning that an online questionnaire would be more likely to reach those with mild disease course. Still, those people with more symptoms, or with more pronounced symptoms, might have felt more motivated to participate in the survey, so it would be especially difficult to conclude the number of asymptomatic SARS-CoV-2 infected individuals from this survey, and the overall frequency of the symptoms might be overestimated. However, as discussed above, the frequencies of many symptoms reported in our study are largely in line with the findings of other symptom studies, therefore our detailed results can be regarded as a reliable contribution. While we did see a higher proportion of smoking respondents in the SARS-CoV-2 negative group than in the SARS-CoV-2 infected individuals, we did not perform further analysis on this finding since the present data is likely biased to symptomatic participants and is therefore neither amenable for a calculation of the overall frequency of asymptomatic infection nor to address the question if smoking leads to a reduced incidence of symptomatic SARS-CoV-2 infection. Finally, it also has to be mentioned that we had a very detailed questionnaire, which may have been difficult to complete for participants with an impaired concentration span or severe brain fog, a challenge that has been reported to us by a small number of participants.
With the spread of new variants, the symptoms experienced by SARS-CoV-2 infected individuals have changed during the pandemic, and are likely to change again in the coming months or years with the spread of future variants. Our data show that it remains important to track the symptoms of SARS-CoV-2 infection.
Data Availability Statement
The raw data supporting the conclusions of this article will be made available by the authors, without undue reservation.
Ethics Statement
The studies involving human participants were reviewed and approved by Ethik-Kommission Medizinische Fakultät Universität Duisburg-Essen Robert-Koch-Str. 9-11 45147 Essen Germany. The patients/participants provided their written informed consent to participate in this study.
Author Contributions
HS recruited participants, analyzed data and contributed to writing the manuscript. WB conceived of the study, created the online survey, analyzed data and wrote the manuscript. All authors contributed to the article and approved the submitted version.
Funding
We acknowledge support by the Open Access Publication Fund of the University of Duisburg-Essen.
Conflict of Interest
The authors declare that the research was conducted in the absence of any commercial or financial relationships that could be construed as a potential conflict of interest.
Publisher’s Note
All claims expressed in this article are solely those of the authors and do not necessarily represent those of their affiliated organizations, or those of the publisher, the editors and the reviewers. Any product that may be evaluated in this article, or claim that may be made by its manufacturer, is not guaranteed or endorsed by the publisher.
Acknowledgments
The authors thank all study participants for taking the time to complete the online questionnaire and contributing to our advancement in the recognition of SARS-CoV-2 symptoms. The authors also thank the employees of the public health offices in Hamm (Germany), Soest (Germany), Hochsauerlandkreis (Germany) and Dr. Hüning and nurses of the SARS-CoV-2 treatment center in Lünen (Germany) for distributing invitations to the online survey. The authors are very grateful to Prof. Dr. Daniel Hoffmann of the Bioinformatics and Computational Biophysics Department of the University Duisburg-Essen for his guidance, advice and helpful discussions.
References
1. Available at: https://promedmail.org/promed-post/?id=6864153%20#COVID19 (Accessed 2020/11/07). P.f.M.E. Disease, Undiagnosed Pneumonia - China (Hubei): Request for Information.
2. Spiteri G, Fielding J, Diercke M, Campese C, Enouf V, Gaymard A, et al. First Cases of Coronavirus Disease 2019 (COVID-19) in the WHO European Region, 24 January to 21 February 2020. Euro Surveill (2020) 25:2000178. doi: 10.2807/1560-7917.ES.2020.25.9.2000178
3. WHO. WHO Coronavirus Dashboard Data. Available at: https://covid19.who.int/WHO-COVID-19-global-table-data.csv (Accessed 2021-11-30).
4. Guan WJ, Ni ZY, Hu Y, Liang WH, Ou CQ, He JX, et al. Clinical Characteristics of Coronavirus Disease 2019 in China. N Engl J Med (2020) 382:1708–20. doi: 10.1056/NEJMoa2002032
5. Huang C, Wang Y, Li X, Ren L, Zhao J, Hu Y, et al. Clinical Features of Patients Infected With 2019 Novel Coronavirus in Wuhan, China. Lancet (2020) 395:497–506. doi: 10.1016/S0140-6736(20)30183-5
6. Miyamae Y, Hayashi T, Yonezawa H, Fujihara J, Matsumoto Y, Ito T, et al. Duration of Viral Shedding in Asymptomatic or Mild Cases of Novel Coronavirus Disease 2019 (COVID-19) From a Cruise Ship: A Single-Hospital Experience in Tokyo, Japan. Int J Infect Dis (2020) 97:293–5. doi: 10.1016/j.ijid.2020.06.020
7. von Bartheld CS, Hagen MM, Butowt R. Prevalence of Chemosensory Dysfunction in COVID-19 Patients: A Systematic Review and Meta-Analysis Reveals Significant Ethnic Differences. ACS Chem Neurosci (2020) 11:2944–61. doi: 10.1021/acschemneuro.0c00460
8. Korber B, Fischer WM, Gnanakaran S, Yoon H, Theiler J, Abfalterer W, et al. Tracking Changes in SARS-CoV-2 Spike: Evidence That D614G Increases Infectivity of the COVID-19 Virus. Cell (2020) 182:812–827.e19. doi: 10.1016/j.cell.2020.06.043
9. Ozono S, Zhang Y, Ode H, Sano K, Tan TS, Imai K, et al. SARS-CoV-2 D614G Spike Mutation Increases Entry Efficiency With Enhanced ACE2-Binding Affinity. Nat Commun (2021) 12:848. doi: 10.1038/s41467-021-21118-2
10. Ye Q, Zhou J, He Q, Li RT, Yang G, Zhang Y, et al. SARS-CoV-2 Infection in the Mouse Olfactory System. Cell Discovery (2021) 7:49. doi: 10.1038/s41421-021-00290-1
11. Khan M, Yoo SJ, Clijsters M, Backaert W, Vanstapel A, Speleman K, et al. Visualizing in Deceased COVID-19 Patients How SARS-CoV-2 Attacks the Respiratory and Olfactory Mucosae But Spares the Olfactory Bulb. Cell (2021) 184:5932–5949.e15. doi: 10.1016/j.cell.2021.10.027
12. Doyle ME, Appleton A, Liu QR, Yao Q, Mazucanti CH, Egan JM. Human Type II Taste Cells Express Angiotensin-Converting Enzyme 2 and Are Infected by Severe Acute Respiratory Syndrome Coronavirus 2 (SARS-CoV-2). Am J Pathol (2021) 191:1511–9. doi: 10.1016/j.ajpath.2021.05.010
13. Jackson CB, Farzan M, Chen B, Choe H. Mechanisms of SARS-CoV-2 Entry Into Cells. Nat Rev Mol Cell Biol (2022) 23:3–20. doi: 10.1038/s41580-021-00418-x
14. Rambaut A, Holmes EC, O'Toole A, Hill V, McCrone JT, Ruis C, et al. A Dynamic Nomenclature Proposal for SARS-CoV-2 Lineages to Assist Genomic Epidemiology. Nat Microbiol (2020) 5:1403–7. doi: 10.1038/s41564-020-0770-5
15. O'Toole Á., Hill V, Pybus O, Watts A, Bogoch I, Khan K, et al. Tracking the International Spread of SARS-CoV-2 Lineages B.1.1.7 and B.1.351/501Y-V2 With Grinch [Version 2; Peer Review: 3 Approved]. Wellcome Open Res 6 (2021) 6:121. doi: 10.12688/wellcomeopenres.16661.2
16. Cov-Lineages.Org, Global Report B.1.1.7. Available at: https://cov-lineages.org/global_report_B.1.1.7.html (Accessed 2022-05-17).
17. Cov-Lineages.Org, Global Report B.1.617.2. Available at: https://cov-lineages.org/global_report_B.1.617.2.html (Accessed 2022-05-17).
18. Cov-Lineages.Org, Global Report B1.1.529. Available at: https://cov-lineages.org/global_report_B.1.1.529.html (Accessed 2022-05-17).
20. Vihta K-D, Pouwels KB, Peto TEA, Pritchard E, House T, Studley R, et al. (2022). Omicron-Associated Changes in SARS-CoV-2 Symptoms in the United Kingdom. medRxiv 01(18):22269082. doi: 10.1101/2022.01.18.22269082
21. Streeck H, Schulte B, Kuemmerer B, Richter E, Hoeller T, Fuhrmann C, et al. Infection Fatality Rate of SARS-CoV-2 Infection in a German Community With a Super-Spreading Event. Nat Commun (2020) 11:5829. doi: 10.1038/s41467-020-19509-y
22. Sudre CH, Lee K, Ni Lochlainn M, Varsavsky T, Murray B, Graham MS, et al. Symptom Clusters in Covid19: A Potential Clinical Prediction Tool From the COVID Symptom Study App. Sci Adv (2020) 7(12)::eabd4177. doi: 10.1101/2020.06.12.20129056
23. Chen N, Zhou M, Dong X, Qu J, Gong F, Han Y, et al. Epidemiological and Clinical Characteristics of 99 Cases of 2019 Novel Coronavirus Pneumonia in Wuhan, China: A Descriptive Study. Lancet (2020) 395:507–13. doi: 10.1016/S0140-6736(20)30211-7
24. Ahamad MM, Aktar S, Rashed-Al-Mahfuz M, Uddin S, Lio P, Xu H, et al. A Machine Learning Model to Identify Early Stage Symptoms of SARS-Cov-2 Infected Patients. Expert Syst Appl (2020) 160:113661. doi: 10.1016/j.eswa.2020.113661
25. Giacomelli A, Pezzati L, Conti F, Bernacchia D, Siano M, Oreni L, et al. Self-Reported Olfactory and Taste Disorders in Patients With Severe Acute Respiratory Coronavirus 2 Infection: A Cross-Sectional Study. Clin Infect Dis (2020) 71:889–90. doi: 10.1093/cid/ciaa330
26. Maechler F, Gertler M, Hermes J, van Loon W, Schwab F, Piening B, et al. Epidemiological and Clinical Characteristics of SARS-CoV-2 Infections at a Testing Site in Berlin, Germany, March and April 2020-a Cross-Sectional Study. Clin Microbiol Infect (2020) 1685:e7–1685.e12. doi: 10.1016/j.cmi.2020.08.017
27. Menni C, Sudre CH, Steves CJ, Ourselin S, Spector TD. Quantifying Additional COVID-19 Symptoms Will Save Lives. Lancet (2020) 395:e107–8. doi: 10.1016/S0140-6736(20)31281-2
28. Menni C, Valdes AM, Freidin MB, Sudre CH, Nguyen LH, Drew DA, et al. Real-Time Tracking of Self-Reported Symptoms to Predict Potential COVID-19. Nat Med (2020) 26:1037–40. doi: 10.1038/s41591-020-0916-2
29. Shoer S, Karady T, Keshet A, Shilo S, Rossman H, Gavrieli A, et al. A Prediction Model to Prioritize Individuals for SARS-CoV-2 Test Built From National Symptom Surveys. Med (N Y) (2020) 2:196–208.e4. doi: 10.1016/j.medj.2020.10.002
30. Vihta KD, Pouwels KB, Peto T, Pritchard E, Eyre DW, House T, et al. Symptoms and SARS-CoV-2 Positivity in the General Population in the UK. Clin Infect Dis (2021), ciab945. doi: 10.1101/2021.08.19.21262231
31. Menni C, Valdes AM, Polidori L, Antonelli M, Penamakuri S, Nogal A, et al. Symptom Prevalence, Duration, and Risk of Hospital Admission in Individuals Infected With SARS-CoV-2 During Periods of Omicron and Delta Variant Dominance: A Prospective Observational Study From the ZOE COVID Study. Lancet (2022) 399:1618–24. doi: 10.1016/S0140-6736(22)00327-0
32. Hajjo R, AbuAlSamen MM, Alzoubi HM, Alqutob R. The Epidemiology of Hundreds of Individuals Infected With Omicron BA.1 in Middle-Eastern Jordan. medRxiv (2022) 01(23) 22269442. doi: 10.1101/2022.01.23.22269442
33. Kim MK, Lee B, Choi YY, Um J, Lee KS, Sung HK, et al. Clinical Characteristics of 40 Patients Infected With the SARS-CoV-2 Omicron Variant in Korea. J Korean Med Sci (2022) 37:e31. doi: 10.3346/jkms.2022.37.e31
34. Team CC-R. SARS-CoV-2 B.1.1.529 (Omicron) Variant - United States, December 1-8, 2021. MMWR Morb Mortal Wkly Rep (2021) 70:1731–4. doi: 10.15585/mmwr.mm7050e1
35. Brandal LT, MacDonald E, Veneti L, Ravlo T, Lange H, Naseer U, et al. Outbreak Caused by the SARS-CoV-2 Omicron Variant in Norway, November to December 2021. Euro Surveill (2021) 26:2101147. doi: 10.2807/1560-7917.ES.2021.26.50.2101147
36. Agency UHS. SARS-CoV-2 Variants of Concern and Variants Under Investigation in England. Tech Brief (2022) 34:1–36.
37. Boscolo-Rizzo P, Tirelli G, Meloni P, Hopkins C, Madeddu G, De Vito A, et al. Coronavirus Disease 2019 (COVID-19)-Related Smell and Taste Impairment With Widespread Diffusion of Severe Acute Respiratory Syndrome-Coronavirus-2 (SARS-CoV-2) Omicron Variant. Int Forum Allergy Rhinol (2022) 10.1002/:alr.22995. doi: 10.1002/alr.22995
38. Helmsdal G, Hansen OK, Moller LF, Christiansen DH, Petersen MS, Kristiansen MF. Omicron Outbreak at a Private Gathering in the Faroe Islands, Infecting 21 of 33 Triple-Vaccinated Healthcare Workers. Clin Infect Dis (2022):ciac089. doi: 10.1093/cid/ciac089
39. Cai Y, Zhang J, Xiao T, Peng H, Sterling SM, Walsh RM Jr., et al. Distinct Conformational States of SARS-CoV-2 Spike Protein. Science (2020) 369:1586–92. doi: 10.1126/science.abd4251
40. Zhang L, Jackson CB, Mou H, Ojha A, Peng H, Quinlan BD, et al. SARS-CoV-2 Spike-Protein D614G Mutation Increases Virion Spike Density and Infectivity. Nat Commun (2020) 11:6013. doi: 10.1038/s41467-020-19808-4
41. Fernandez A. Structural Impact of Mutation D614G in SARS-CoV-2 Spike Protein: Enhanced Infectivity and Therapeutic Opportunity. ACS Med Chem Lett (2020) 11:1667–70. doi: 10.1021/acsmedchemlett.0c00410
42. Michaud WA, Boland GM, Rabi SA. The SARS-CoV-2 Spike Mutation D614G Increases Entry Fitness Across a Range of ACE2 Levels, Directly Outcompetes the Wild Type, and is Preferentially Incorporated Into Trimers. bioRxiv (2020) 08(25):267500. doi: 10.1101/2020.08.25.267500
43. Juraszek J, Rutten L, Blokland S, Bouchier P, Voorzaat R, Ritschel T, et al. Stabilizing the Closed SARS-CoV-2 Spike Trimer. Nat Commun (2021) 12:244. doi: 10.1038/s41467-020-20321-x
44. Zhang J, Cai Y, Xiao T, Lu J, Peng H, Sterling SM, et al. Structural Impact on SARS-CoV-2 Spike Protein by D614G Substitution. Science (2021) 372:525–30. doi: 10.1126/science.abf2303
45. Yurkovetskiy L, Wang X, Pascal KE, Tomkins-Tinch C, Nyalile TP, Wang Y, et al. Structural and Functional Analysis of the D614G SARS-CoV-2 Spike Protein Variant. Cell (2020) 183:739–51.e8. doi: 10.1016/j.cell.2020.09.032
46. Gobeil SM, Janowska K, McDowell S, Mansouri K, Parks R, Manne K, et al. D614G Mutation Alters SARS-CoV-2 Spike Conformation and Enhances Protease Cleavage at the S1/S2 Junction. Cell Rep (2021) 34:108630. doi: 10.1016/j.celrep.2020.108630
47. Weissman D, Alameh MG, de Silva T, Collini P, Hornsby H, Brown R, et al. D614G Spike Mutation Increases SARS CoV-2 Susceptibility to Neutralization. Cell Host Microbe (2021) 29:23–31.e4. doi: 10.1016/j.chom.2020.11.012
48. Benton DJ, Wrobel AG, Roustan C, Borg A, Xu P, Martin SR, et al. The Effect of the D614G Substitution on the Structure of the Spike Glycoprotein of SARS-CoV-2. Proc Natl Acad Sci U.S.A. (2021) 118(9):e2022586118. doi: 10.1073/pnas.2022586118
49. Hou YJ, Okuda K, Edwards CE, Martinez DR, Asakura T, Dinnon KH 3rd, et al. SARS-CoV-2 Reverse Genetics Reveals a Variable Infection Gradient in the Respiratory Tract. Cell (2020) 182:429–446.e14. doi: 10.1016/j.cell.2020.05.042
50. Shang J, Ye G, Shi K, Wan Y, Luo C, Aihara H, et al. Structural Basis of Receptor Recognition by SARS-CoV-2. Nature (2020) 581:221–4. doi: 10.1038/s41586-020-2179-y
51. Starr TN, Greaney AJ, Hilton SK, Ellis D, Crawford KHD, Dingens AS, et al. Deep Mutational Scanning of SARS-CoV-2 Receptor Binding Domain Reveals Constraints on Folding and ACE2 Binding. Cell (2020) 182:1295–1310.e20. doi: 10.1016/j.cell.2020.08.012
52. Peacock TP, Goldhill DH, Zhou J, Baillon L, Frise R, Swann OC, et al. The Furin Cleavage Site in the SARS-CoV-2 Spike Protein is Required for Transmission in Ferrets. Nat Microbiol (2021) 6:899–909. doi: 10.1038/s41564-021-00908-w
53. Lubinski B, Frazier L, Phan M, Bugumbe D, Cunningham JL, Tang T, et al. Spike Protein Cleavage-Activation Mediated by the SARS-CoV-2 P681R Mutation: A Case-Study From its First Appearance in Variant of Interest (VOI) A.23.1 Identified in Uganda. bioRxiv (2022) 06(30):450632. doi: 10.1101/2021.06.30.450632
54. Bartheld CSv, Hagen MM, Butowt R. (2021). The D614G Virus Mutation Enhances Anosmia in COVID-19 Patients: Evidence From a Systematic Review and Meta-Analysis of Studies From South Asia. ACS Chem Neurosci 12(19)3535–49. doi: 10.1021/acschemneuro.1c00542
55. Kim S, Liu Y, Ziarnik M, Cao Y, Zhang XF, Im W. Binding of Human ACE2 and RBD of Omicron Enhanced by Unique Interaction Patterns Among SARS-CoV-2 Variants of Concern. bioRxiv (2022) 01(24):477633. doi: 10.1101/2022.01.24.477633
56. Meng B, Abdullahi A, Ferreira I, Goonawardane N, Saito A, Kimura I, et al. Altered TMPRSS2 Usage by SARS-CoV-2 Omicron Impacts Infectivity and Fusogenicity. Nature (2022) 603:706–14. doi: 10.1038/s41586-022-04474-x
57. Bilinska K, Jakubowska P, Von Bartheld CS, Butowt R. Expression of the SARS-CoV-2 Entry Proteins, ACE2 and TMPRSS2, in Cells of the Olfactory Epithelium: Identification of Cell Types and Trends With Age. ACS Chem Neurosci (2020) 11:1555–62. doi: 10.1021/acschemneuro.0c00210
58. Chen M, Pekosz A, Villano JS, Shen W, Zhou R, Kulaga H, et al. Evolution of Nasal and Olfactory Infection Characteristics of SARS-CoV-2 Variants. bioRxiv (2022) 04(12): 487379. doi: 10.1101/2022.04.12.487379
59. Del Valle DM, Kim-Schulze S, Huang HH, Beckmann ND, Nirenberg S, Wang B, et al. An Inflammatory Cytokine Signature Predicts COVID-19 Severity and Survival. Nat Med (2020) 26:1636–43. doi: 10.1038/s41591-020-1051-9
60. Gu T, Zhao S, Jin G, Song M, Zhi Y, Zhao R, et al. Cytokine Signature Induced by SARS-CoV-2 Spike Protein in a Mouse Model. Front Immunol (2020) 11:621441. doi: 10.3389/fimmu.2020.621441
61. Schroeder JT, Bieneman AP. The S1 Subunit of the SARS-CoV-2 Spike Protein Activates Human Monocytes to Produce Cytokines Linked to COVID-19: Relevance to Galectin-3. Front Immunol (2022) 13:831763. doi: 10.3389/fimmu.2022.831763
62. Suryawanshi RK, Chen IP, Ma T, Syed AM, Brazer N, Saldhi P, et al. Limited Cross-Variant Immunity After Infection With the SARS-CoV-2 Omicron Variant Without Vaccination. medRxiv (2022) 01(13):22269243. doi: 10.1101/2022.01.13.22269243
63. Yuan S, Ye Z-W, Liang R, Tang K, Zhang AJ, Lu G, et al. The SARS-CoV-2 Omicron (B.1.1.529) Variant Exhibits Altered Pathogenicity, Transmissibility, and Fitness in the Golden Syrian Hamster Model. bioRxiv (2022) 01(12):476031. doi: 10.1101/2022.01.12.476031
64. Barh D, Tiwari S, Gomes LGR, Pinto CHR, Andrade BS, Ahmad S, et al. SARS-CoV-2 Variants Show a Gradual Declining Pathogenicity and Pro-Inflammatory Cytokine Spur, an Increasing Antigenic and Antiinflammatory Cytokine Induction, and Rising Structural Protein Instability. bioRxiv (2022) 02(15):480592. doi: 10.1101/2022.02.15.480592
65. Bojkova D, Rothenburger T, Ciesek S, Wass MN, Michaelis M, Cinatl J Jr. SARS-CoV-2 Omicron Variant Virus Isolates are Highly Sensitive to Interferon Treatment. Cell Discovery (2022) 8:42. doi: 10.1038/s41421-022-00408-z
Keywords: SARS-CoV-2, omicron, COVID-19, variants, coronavirus
Citation: Schulze H and Bayer W (2022) Changes in Symptoms Experienced by SARS-CoV-2-Infected Individuals – From the First Wave to the Omicron Variant. Front.Virol. 2:880707. doi: 10.3389/fviro.2022.880707
Received: 21 February 2022; Accepted: 02 June 2022;
Published: 01 July 2022.
Edited by:
Hang Xie, United States Food and Drug Administration, United StatesReviewed by:
Christopher von Bartheld, University of Nevada, Reno, United StatesRuoxuan Xiang, United States Food and Drug Administration, United States
Copyright © 2022 Schulze and Bayer. This is an open-access article distributed under the terms of the Creative Commons Attribution License (CC BY). The use, distribution or reproduction in other forums is permitted, provided the original author(s) and the copyright owner(s) are credited and that the original publication in this journal is cited, in accordance with accepted academic practice. No use, distribution or reproduction is permitted which does not comply with these terms.
*Correspondence: Wibke Bayer, d2lia2UuYmF5ZXJAdW5pLWR1ZS5kZQ==