- 1Laboratory of Viruses, Microbiology Department, Biological Sciences Institute, Federal University of Minas Gerais, Minas Gerais, Brazil
- 2Department of Pediatrics, Division of Infectious Disease, Stanford University School of Medicine, Stanford, CA, United States
- 3Eduardo de Menezes Hospital, Belo Horizonte, Brazil
- 4Bendigo Heath Hospital, Bendigo, VIC, Australia
- 5Immunology of Viruses Diseases, René Rachou Institute, Oswaldo Cruz Foundation/FIOCRUZ, Minas Gerais, Brazil
- 6Cellular and Molecular Immunology, René Rachou Institute, Oswaldo Cruz Foundation/FIOCRUZ, Minas Gerais, Brazil
- 7Secretary of Health of Minas Gerais state, Belo Horizonte, Brazil
- 8Integrated Group of Biomarkers Research, René Rachou Institute, Oswaldo Cruz Foundation/FIOCRUZ, Minas Gerais, Brazil
Yellow fever virus (YFV) is the causative agent of yellow fever (YF), a hemorrhagic and viscerotropic acute disease. Severe YF has been described in approximately 15-25% of YF patients, with 20-50% of severe YF cases being fatal. Here we analyzed cerebrospinal fluid (CSF) samples collected during the YF outbreak in Brazil in 2018, aiming to investigate CNS neuroinvasion in fatal YFV cases. YFV RNA was screened by RT-qPCR targeting the 3’UTR region of the YFV genome in CSF. CSF samples were tested for the presence of anti-YFV IgM and neutralizing antibodies, coupled with routine laboratory examinations. Among the 13 patients studied, we detected anti-YFV IgM in CSF from eight patients and YFV RNA in CSF from five patients. YFV RNA genomic load in CSF samples ranged from 1.75x103 to 5.42x103 RNA copies/mL. We genotyped YFV from three CSF samples that grouped with other YFV samples from the 2018 outbreak in Brazil within the South-American I genotype. Even though descriptions of neurologic manifestations due to wild type YFV (WT-YFV) infection are rare, since the last YF outbreak in Brazil in 2017-2018, a few studies have demonstrated WT-YFV RNA in CSF samples from YF fatal cases. Serological tests indicated the presence of IgM and neutralizing antibodies against YFV in CSF samples from two patients. Although the presence of viral RNA, IgM and neutralizing antibodies in CSF samples could indicate neuroinvasiveness, further studies are needed to better elucidate the role of YFV neuroinvasion and possible impacts in disease pathogenesis.
Introduction
Yellow fever virus (YFV) causes approximately 30,000 deaths out of 200,000 infections annually in tropical Africa and South America (1, 2). Severe YF has been described in approximately 15-25% of yellow fever (YF) patients, with 20-50% of severe YF cases being fatal (1, 3). YF is a viscerotropic hemorrhagic disease in humans, causing a variety of signs and symptoms including fever, headache, vomiting, jaundice, chills, nausea, abdominal pain, myalgia, arthralgia, rash, diarrhea, and bleeding, among others (1, 4). In patients with severe clinical manifestations, high levels of aspartate (AST) and alanine aminotransferases (ALT), thrombocytopenia, and a multifactorial bleeding disorder caused by reduced synthesis of clotting factors are usually observed. Acute liver disorders are considered one of the main factors causing brain pathology. Indeed, cytokine storm developed during the YF viscerotropic disease could contribute to central nervous system inflammation and YFV neuroinvasion (5).
The currently attenuated vaccine strains derived from the lineage 17D were originated from wild-type virus strain Asibi (6). This vaccine can cause adverse events such as YF vaccine-associated viscerotropic disease (YEL-AVD), which resembles the disease caused by WT-YFV, and YF vaccine-associated neurotropic disease (YEL-AND) with infection of the central nervous system (CNS). YEL-AND includes at least one of the following signs and symptoms: fever higher than 38° C, headache, focal neurological symptom (including ataxia, aphasia, and paresis), meningeal signs, altered mental status, seizure and cerebrospinal fluid (CSF) pleocytosis (7). Both YEL-AVD and YEL-AND are rare but often fatal (6). It is believed that these severe adverse events are influenced mainly by host factors, including the immune response. In contrast, wild-type YF (WT-YYF) infection in brain tissue, causing viral encephalitis, is rarely described (8).
Despite the viscerotropic aspect of YFV infection, since the last YF outbreak in Brazil in 2016-2019, few studies have detected WT-YFV genomic RNA in CSF from fatal cases (3, 9, 10). CNS involvement has been associated with other flavivirus infections such as Japanese encephalitis virus, West Nile virus, Zika virus (ZIKV), and dengue virus (DENV) (9, 11–16). The clinicopathological presentations of flavivirus CNS infection range from mild meningitis to fulminant meningoencephalomyelitis (16). These viruses usually reach the CNS through blood and brain-blood barrier (BBB) disruption (16, 17) due to increased endothelial cell permeability induced by viral replication or neuroinflammation (18). More recently, a new extension of the CNS was found, described as a network of lymphatic vessels within the dura mater that runs alongside blood vessels. This network provides an alternate conduit for the drainage of immune cells and CSF from the brain (19), and could also be involved during CNS invasion. This data suggests that BBB permeability and CNS lymph drainage in the setting of an exaggerated proinflammatory cytokine response may lead to the shock phase of yellow fever (1). The goal of this study was to further investigate CNS neuroinvasion in fatal YFV cases during the YF outbreak in Brazil in 2018.
Methodology
Biological samples and ethics
All patients were admitted at Eduardo de Menezes Hospital (HEM), Belo Horizonte, Brazil, a reference hospital for infectious diseases in Minas Gerais state, during the 2018 YF outbreak. Diagnosis of YF was confirmed through positive YFV RT-qPCR, YFV isolation from serum sample, or detection of anti-YFV IgM in serum, followed by negative anti-DENV and anti-ZIKV IgM tests. Diagnostic tests were run at Reference Laboratory in Minas Gerais (Ezequiel Dias Foundation), following routine tests at this institution. Test results were referred to us. Of the 53 fatal cases admitted at HEM during the 2018 YF outbreak, 13 underwent postmortem CSF collection. CSF was collected at the Eduardo de Menezes Hospital and kept in liquid nitrogen until adequate transport to the Laboratory of Viruses/UFMG/Brazil and stored at -70 °C. Ethics Committee on Human Research approved the research at René Rachou Institute/FIOCRUZ on CAAE 65814417.0.0000.5091 and CAAE: 43000815.7.0000.5091 and at Stanford University School of Medicine, under the eProtocol number 53676.
YFV investigation
Total RNA extraction was done using 140 µL of CSF sample and QIAmp Viral RNA Mini kit (Qiagen, Germany), following the manufacturer’s instructions. Total RNA (5 µL) was used in RT-qPCR targeting the 3’UTR region of the YFV genome (20). Positive samples were then used for quantitative PCR, using Bio Gene Research Yellow Fever PCR kit, following manufacturer’s instructions (Bioclin, Brazil).
YFV genotyping
YFV RNA isolated from CSF samples were subjected to genotyping, as previously described (21). Briefly, part of NS5 gene was amplified (260 bp) (22) and sequenced (23). The sequences generated here were then aligned with a sequence panel previously used (21), using Clustal W, implemented on Mega7 (24). The Maximum-likelihood tree was generated using Kimura-2-parameters nucleotide substitution model with gamma distribution, and 1,000 bootstraps replicates, using MEGA7 (24).
Serological analysis
An anti-YFV IgM immunochromatographic test was run (ECO Diagnóstica, Brazil) on CSF samples. YFV RNA positive CSF samples that were also IgM anti-YFV reactive were used in plaque reduction neutralization test (PRNT), as previously described (25). Briefly, CSF samples were two-fold diluted from 1:20 to 1:1280. Dilutions of CSF were incubated with 150 plaque-forming units (PFUs) of YFV vaccine strain YFV-17DD. The mix of CSF and vaccine YFV-17DD were then used for Vero cells’ infection. After five days post-infection, cells were fixed, stained using crystal violet, and viral plaques were counted. Samples were considered positive when presented with a reduction of 50% of total counted plaques compared to virus control. Samples were tested in duplicate in PRNT.
Laboratory exams
As part of the Intensive Care Unit (ICU) routine of HEM, a complete hemogram was run, including counting of hemoglobin, hematocrit, platelets, neutrophils, leukocytes, lymphocytes, eosinophils. Routine tests also included blood urea, albumin, AST, ALT, gamma-glutamyl transferase (GGT), alkaline phosphatase (ALP), lactate, total (Tbil) and direct bilirubin (Dbil), creatinine (Cr), and International normalized ratio (INR). Values for each exam were following the Laboratory Test Ranges (26).
Statistical analysis
Considering the small number of patients in this study, we performed the nonparametric Mann-Whitney U test comparing laboratory variables regarding patients with and without YFV RNA detection in CSF. Analyzed variables were selected according to previous studies that have been shown predictive factors for severe YF disease (3), and hepatic injury markers, including neutrophil count, ALT, AST, INR, indirect bilirubin, and creatinine on day of admission. Significative difference was considered when p < 0.05.
Results
During the YF outbreak in Brazil in 2018, CSF samples were collected postmortem from 13 patients at HEM. Our results showed that, among those, five patients were RT-qPCR positive for YFV RNA in CSF. We estimated the YFV RNA genomic load in CSF samples from four patients, and values ranged from 1.75 x 103 to 5.42 x 103 RNA copies/mL (Table 1). Positive CSF samples were collected on the day of death, corresponding to 4 to 25 days post-symptoms (DPS) (Table 1).
The five YFV positive CSF samples were from male patients, ranging from 24 to 62 years of age (Table 2). At hospital admission, the most common clinical findings included fever, jaundice (5/5 patients), followed by headache, vomiting (4/5 patients); confusion, oliguria, myalgia (3/5 patients); asthenia, bleeding (2/5 patients); seizure, abdominal pain, diarrhea, dehydration, and palpable liver (1/5 patients) (Table 2). One patient died on the day of hospitalization (P #3) and four remained hospitalized from 4 up to 25 DPS (Table 2), until the day of death. Patients P #1, P #2, and P #5 had CNS signs at presentation (altered mental status). Patients #3 and #4 arrived at HEM already intubated, with a report of seizure and altered mental status.
Among the 13 CSF samples, 12 were tested using an IgM anti-YFV immunochromatographic test, and eight were IgM positive (Table 2). We could not run this test using CSF sample from P #13, due to insufficient quantity. Samples that had YFV RNA detected in CSF and were IgM reactive (P #1, P #2, P #4), were used in a PRNT assay. CSF samples from patients P #1 and P #4 presented neutralizing antibodies against YF 17DD strain (P #1: up to sera dilution 1:40 and P #4: up to sera dilution 1:640) (Table 1).
Regardless the fact that none of the patients had been vaccinated against YF up to 30 days before symptoms onset, we performed YFV genotyping using CSF from three patients that presented YFV RNA (P #1, P #3, and P #5). The other two patients were negative after the genotyping RT-qPCR. All YFV RNA sequences detected in CSF were assigned to the South-American I genotype cluster, together with other YFV samples from the 2018 outbreak in Brazil (Figure 1).
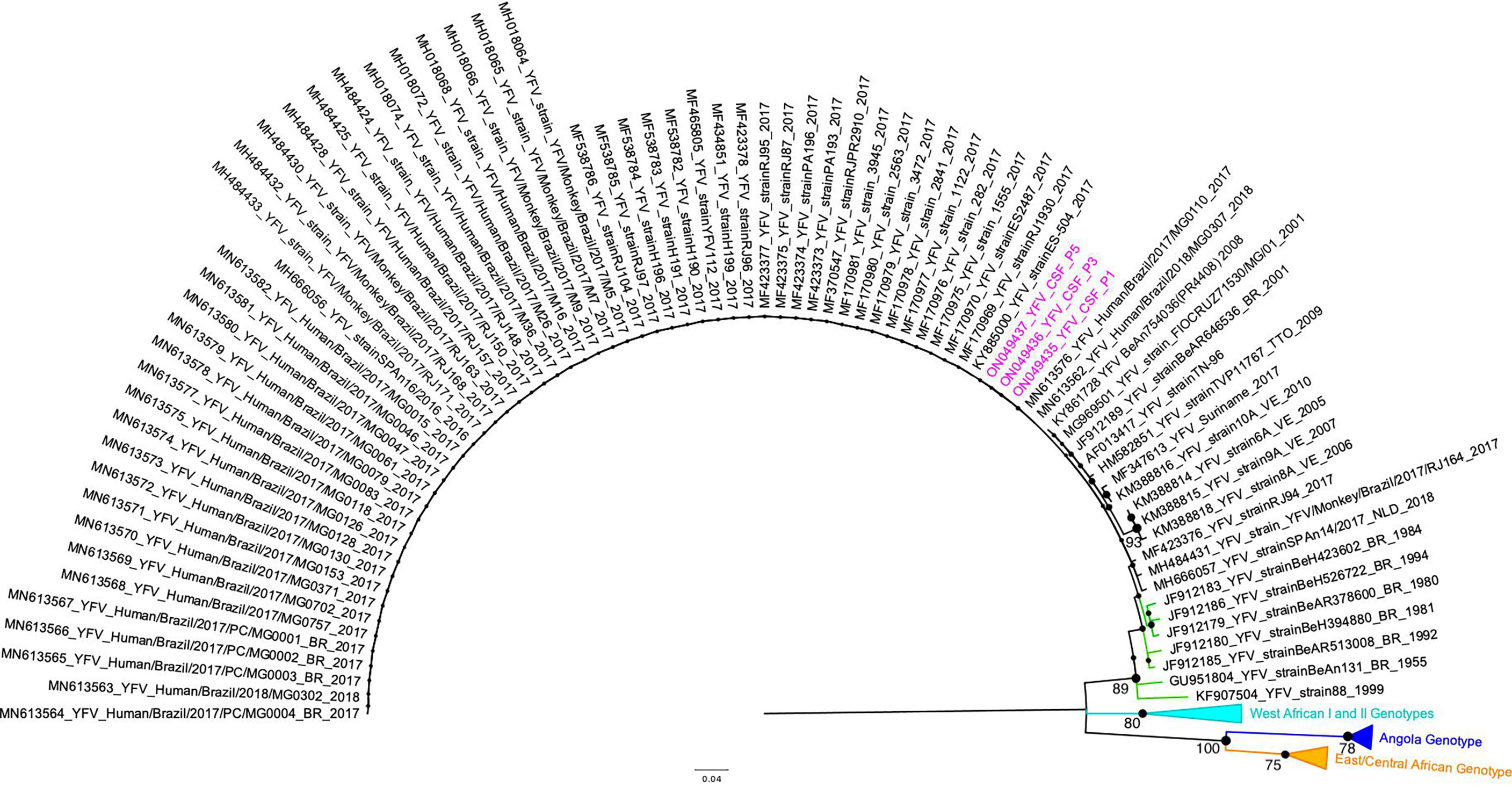
Figure 1 Maximum Likelihood tree of Yellow fever virus. The maximum clade credibility tree inferred using Yellow fever virus (YFV) sequences (187 nt) is shown (corresponding to position 9046 to 9232 compared to the YFV nucleotide sequence, GenBank accession number KY885001). The bootstrap values (1.000 replicates) are represented by circles drawn in scale in the nodes. Bootstrap values higher than 75 are indicated. Sequences generated in this study are highlighted in pink. The clade containing samples from the genotype South-American I is represented in black. The clade containing samples from genotype South-American II is represented in green. For clarity purposes, some branches representing different genotypes were collapsed and colored as follows: West African I and II (light blue), Angola (dark blue), and East/Central African (orange), respectively. The tree was reconstructed using the nucleotide substitution model kimura 2-parameters with 4-categories gamma distribution. The analysis was performed using MEGA7 and the tree was visualized and edited in FigTree v1.4.4.
Severe yellow fever cases could be associated with encephalopathy and altered liver injury markers, which can lead to an increase in the BBB permeability, facilitating the virus from crossing this barrier and being found in the central nervous system (16, 17). Considering previously described risk factors for severe YF (3) (older age, male sex, higher neutrophil counts, higher levels of AST, indirect bilirubin, creatinine, prolonged prothrombin time, and YFV RNA viral load in plasma) and hepatic injury markers, we performed statistical analysis comparing data from the five patients with positive CSF and the other eight patients with no detection of YFV RNA in CSF. None of the analyzed variables presented a statistically significant difference. However, all 13 patients presented with at least two risk factors for severe disease (Table 2).
Discussion
This study demonstrates the presence of wild type YFV RNA in CSF from five naturally infected YF patients during the 2018 YF outbreak in Brazil. We were also able to detect antibodies against YFV in CSF in three of those five patients. These results suggest that YFV can be neuroinvasive in severe disease, but more studies are needed to confirm the role of YFV in neuroinvasion given our small sample size. Although YFV genomic RNA has also been detected in CSF of naturally infected patients by other studies (3, 9, 10), encephalitis caused by YFV neuroinvasion is rarely described (1, 8) and more studies are needed to better understand this phenomenon in humans. While neurological disease can also occur as an adverse event following YF vaccination, neurologic manifestations due to WT-YFV infection are rare, and early studies described delirium, seizures (1, 8, 27), and coma as one of the events preceding death due to YF infection (1). It is known that these manifestations are more associated with cerebral edema and metabolic factors associated with severe disease than with the action of the virus itself in the CNS (8).
According to protocols of patient management recommended by the Ministry of Health in Brazil, all 13 patients analyzed in this study were classified as YF severe cases (group C) and hospitalized in an intensive care unit (ICU) (7). This classification includes the presence of signs and symptoms as oliguria, somnolence, lethargy, mental confusion, coma, seizure, bleeding, difficulty to breathing, hypotension, poor perfusion or AST or ALT ≥ 2,000 UI/L, CR ≥2, INR ≥ 1,5, and platelets < 50,000 cells/mL (7). In addition, Kallas and colleagues (2019) described older age (> 45 years old), male sex, higher neutrophil counts (> 4,000 cells/mL), higher levels of AST (≥ 3,500 UI/L), indirect bilirubin (≥ 0.64 mg/dL), creatinine (≥2.36 UI/L), prolonged prothrombin time (≥1.46), and YFV RNA viral load in plasma (≥ 5.1 log copies/mL) as risk factors for fatal cases (3).
Analyzing our patients on the day of their death, they presented with indirect bilirubin, INR, and creatinine above the cut-off found by Kallas etal. (3), and all were considered comatose on the day of death. Four patients showed AST and neutrophil counts higher than cut-off values described as risk factors for fatal disease (3). In addition, two patients studied here presented with serum YFV genomic RNA load higher than the established threshold for fatal YF (3). All patients presented with three or more signs and symptoms described by the Ministry of Health (7) as an important factor for severe YF clinical illness (Table 2), and at least two several markers for poor prognosis, described by Kallas etal. (3) (3, 7), that could be lead to neurologic manifestations (8). However, we did not find any risk factor that could be associated with detection of YFV RNA in CSF.
Together, our findings suggest severe cellular damage by YFV in the five patients with YFV RNA in CSF. The combination of liver, kidney, and bone marrow damage indicates the involvement of multiple organs, resulting in a systemic damage. In most cases, this damage might be irreversible due to systemic impairment and the interdependence of organ systems in their functioning. Liver failure probably contributes to encephalitis (28), and the already fragile BBB allowed virus crossing to CNS, likely mediated by cytokine storm produced during viscerotropic YF infection. It has been described for other flaviviruses, that this cytokine storm can alter the permeability of the endothelium through the disturbance of the tight junctions of BBB (reviewed by 29). Bearing in mind that WT- YFV must have a relative neurovirulence in humans, the virus probably reached the CNS due to the severe clinical illness of the studied patients during YF infection. The virus could have reached the CNS as a free particle or inside an infected cell (Trojan-horse entry mechanism) (30). Possibly as a consequence of immune response against YFV, infected cells could have crossed the BBB carrying viruses’ particles and immunoglobins to the CNS (reviewed by 30). Another pathway that might have allowed neuroinvasion by YFV could be the recently described network of lymphatic vessels within the dura mater that runs alongside blood vessels and is responsible for fluid flow and lymphatic drainage from the central nervous system (19).
While the presence of IgM in CSF without direct detection of virus could indicate neuroinvasiveness, it is important to note that this phenomenon might be a consequence of a passive crossing through the BBB and does not necessarily implicate the presence of YFV particle or active virus replication in CNS. However, the recent YF outbreak in Brazil was caused by a new viral lineage with unique mutations and amino acid substitutions (2, 4) and the possible impacts of these alterations in viral biology and pathogenesis still need to be addressed. Nevertheless, it is plausible to consider that the presence of YFV RNA, and possibly viral particles in CNS, could have harmed the outcome of these patients.
Conclusion
In this study YFV genomic RNA, IgM, and neutralizing antibodies were detected in CSF in fatal YF cases at HEM with presenting signs and symptoms of severe viscerotropic YF disease. Severe clinical illness may facilitate the virus invasion in CNS. Further studies are needed to better elucidate the role of CNS involvement in YF disease.
Data availability statement
The datasets presented in this study can be found in online repositories. The names of the repository/repositories and accession number(s) can be found in the article/Supplementary Material.
Ethics statement
The studies involving human participants were reviewed and approved by This study was approved by the institutional review boards at René Rachou Institute/FIOCRUZ on CAAE 65814417.0.0000.5091 and CAAE: 43000815.7.0000.5091 and at Stanford University School of Medicine, under the number eProtocol 53676. The patients/participants provided their written informed consent to participate in this study.
Author contributions
IR performed scientific experiments, results analysis, first draft, tables and figures conceptualization. IR and BD wrote the first draft. IR, TC, GO, MA and APG performed the scientific experiments. AC, LR, LM, IP and LP assisted with medical record analysis and medical discussion. LP and DR provided resources. IR, LP, and BD designed the study. DR and BD conceptualized the study. PA provided the sequencing facility, PA, EK, CC-S, OM-F, AT-C, AL and BD provided funding, and coordinated the project. All authors contributed to manuscript editing, and revision, and approved the submitted version. All authors contributed to the article and approved the submitted version.
Funding
This work was supported by different agencies. BD was supported by Fundação de Amparo à Pesquisa do Estado de Minas Gerais (FAPEMIG), under Grant APQ-01574-17. PA, BD, AT-C, OM-F were supported by Secretaria de Estado de Saúde de Minas Gerais (SES-MG)/Secretaria de Estado de Planejamento de Minas Gerais (SEPLAG-MG)/Instituto René Rachou/Fundação Oswaldo Cruz (IRR/FIOCRUZ) under grant yellow fever. IR, PA, BD, AT-C, OM-F, AL were supported by CNPq/NIH, under Grant 404192-2019-0/R01 AI149614. CC-S was supported by Fundação Oswaldo Cruz (Fiocruz), under grant VPPIS-004- FIO-18-32. PA was supported by FAPEMIG under grant APQ-01989-18. IR were supported by Coordenação de Aperfeiçoamento de Pessoal de Nı́vel Superior (CAPES)/Brazil graduate scholarship grant (0001). TC, and GO were supported by CNPq FAPEMIG, and CAPES undergraduate scholarship grants. BD, AT-C, OM-F are CNPq Research Fellows. The funders had no role in study design, data collection and analysis, decision to publish, or preparation of the manuscript.
Conflict of interests
The authors declare that the research was conducted in the absence of any commercial or financial relationships that could be construed as a potential conflict of interest.
Publisher’s note
All claims expressed in this article are solely those of the authors and do not necessarily represent those of their affiliated organizations, or those of the publisher, the editors and the reviewers. Any product that may be evaluated in this article, or claim that may be made by its manufacturer, is not guaranteed or endorsed by the publisher.
References
1. Monath TP. Yellow fever: an update. Lancet Infect Dis (2001) 1:11–20. doi: 10.1016/S1473-3099(01)00016-0
2. Sacchetto L, Drumond BP, Han BA, Nogueira ML, Vasilakis N. Re-emergence of yellow fever in the neotropics — quo vadis? Emerg Top Life Sci (2020) 4:411–22. doi: 10.1042/ETLS20200187
3. Kallas EG, D’Elia Zanella L. G. F. A. B., Moreira CHV, Buccheri R, Diniz GBF, Castiñeiras ACP, et al. Predictors of mortality in patients with yellow fever: an observational cohort study. Lancet Infect Dis (2019) 19:750–8. doi: 10.1016/S1473-3099(19)30125-2
4. Silva NIO, Sacchetto L, de Rezende IM, Trindade GDS, Labeaud AD, De Thoisy B, et al. Recent sylvatic yellow fever virus transmission in Brazil: The news from an old disease. Virol J (2020) 17:1–12. doi: 10.1186/s12985-019-1277-7
5. Hadjihambi A, Arias N, Sheikh M, Jalan R. Hepatic encephalopathy: a critical current review. Hepatol Int (2018) 12:135–47. doi: 10.1007/s12072-017-9812-3
6. Beck AS, Barrett AD. Current status and future prospects of yellow fever vaccines. Expert Rev Vaccines (2015) 14:1479–92. doi: 10.1586/14760584.2015.1083430
8. McEntire CRS, Song K-W, McInnis RP, Rhee JY, Young M, Williams E, et al. Neurologic manifestations of the world health organization’s list of pandemic and epidemic diseases. Front Neurol (2021) 12:634827. doi: 10.3389/fneur.2021.634827
9. Marinho PES, Alvarenga PPM, Crispim APC, Candiani TMS, Alvarenga AM, Bechler IM, et al. Wild-type yellow fever virus rna in cerebrospinal fluid of child. Emerg Infect Dis (2019) 25:1567–70. doi: 10.3201/eid2508/181479
10. Ribeiro AF, Cavalin RF, Suleiman JMAH, Da Costa JA, De Vasconcelos MJ, Málaque CMSA, et al. Yellow fever: Factors associated with death in a hospital of reference in infectious diseases, são paulo, Brazil 2018. Am J Trop Med Hyg (2019) 101:180–8. doi: 10.4269/ajtmh.18-0882
11. Carod-Artal FJ, Wichmann O, Farrar J, Gascón J. Neurological complications of dengue virus infection. Lancet Neurol (2013) 12:906–19. doi: 10.1016/S1474-4422(13)70150-9
12. Suthar MS, Diamond MS, Gale. West Nile Virus infection and immunity. Nat Rev Microbiol (2013) 11:115–28. doi: 10.1038/nrmicro2950
13. Oliveira DB, Machado G, de Almeida GMF, Ferreira PCP, Bonjardim CA, deTrindade GS, et al. Infection of the central nervous system with dengue virus 3 genotype I causing neurological manifestations in Brazil. Rev Soc Bras Med Trop (2016) 49:125–9. doi: 10.1590/0037-8682-0208-2015
14. Petersen LR, Jamieson DJ, Powers AM, Honein MA. Zika virus. N Engl J Med (2016) 374:1552–63. doi: 10.1056/NEJMra1602113
15. Li G-H, Ning Z-J, Liu Y-M, Li X-H. Neurological manifestations of dengue infection. Front Cell Infect Microbiol (2017) 7:449. doi: 10.3389/fcimb.2017.00449
16. Maximova OA, Pletnev AG. Flaviviruses and the central nervous system: Revisiting neuropathological concepts. Annu Rev Virol (2018) 5:255–72. doi: 10.1146/annurev-virology-092917-043439
17. Papa MP, Meuren LM, Coelho SVA, de Oliveira Lucas CG, Mustafá YM, Matassoli FL, et al. Zika virus infects, activates, and crosses brain microvascular endothelial cells, without barrier disruption. Front Microbiol (2017) 8:2557. doi: 10.3389/fmicb.2017.02557
18. Daniels B, Klein R. Viral sensing at the blood-brain barrier: New roles for innate immunity at the CNS vasculature. Clin Pharmacol Ther (2015) 97:372–9. doi: 10.1002/cpt.75
19. Absinta M, Ha S-K, Nair G, Sati P, Luciano NJ, Palisoc M, et al. Human and nonhuman primate meninges harbor lymphatic vessels that can be visualized noninvasively by MRI. Elife (2017) 6:e29738. doi: 10.7554/eLife.29738
20. Domingo C, Patel P, Yillah J, Weidmann M, Mendez JA, Nakoune ER, et al. Advanced yellow fever virus genome detection in point-of-Care facilities and reference laboratories. J Clin Microbiol (2012) 50:4054–60. doi: 10.1128/JCM.01799-12
21. Rezende IM, Alves PA, Arruda MS, Gonçalves AP, Oliveira GFG, Pereira LS, et al. Yellow fever virus genotyping tool and investigation of suspected adverse events following yellow fever vaccination. Vaccines (2019) 7:1–10. doi: 10.3390/vaccines7040206
22. Patel P, Landt O, Kaiser M, Faye O, Koppe T, Lass U, et al. Development of one-step quantitative reverse transcription PCR for the rapid detection of flaviviruses. Virol J (2013) 10:58. doi: 10.1186/1743-422X-10-58
23. Sanger F, Nicklen S. DNA Sequencing with chain-terminating inhibitors. Proc Natl Acad Sci USA (1977) 74:5463–7. doi: 10.1073/pnas.74.12.5463
24. Kumar S, Stecher G, Tamura K. MEGA7: Molecular evolutionary genetics analysis version 7.0 for bigger datasets. Mol Biol Evol (2016) 33:1870–4. doi: 10.1093/molbev/msw054
25. Wieten RW, Jonker EFF, Pieren DKJ, Hodiamont CJ, van Thiel PPAM, van Gorp ECM, et al. Comparison of the PRNT and an immune fluorescence assay in yellow fever vaccinees receiving immunosuppressive medication. Vaccine (2016) 34:1247–51. doi: 10.1016/j.vaccine.2016.01.037
26. ABIM. Laboratory test reference ranges (2020). Available at: https://www.abim.org/~/media/ABIM%20Public/Files/pdf/exam/laboratory-reference-ranges.pdf (Accessed January 13, 2020).
27. Stevenson LD. Pathologic changes in the nervous system in yellow fever. Arch Pathol (1939) 27:249–66.
28. Monath TP, Barrett ADT. Pathogenesis and pathophysiology of yellow fever. (2003) 60:3527. doi: 10.1016/S0065-3527(03)60009-6
29. Puccioni-Sohler M, Rosadas C. Advances and new insights in the neuropathogenesis of dengue infection. Arq Neuropsiquiatr (2015) 73:698–703. doi: 10.1590/0004-282X20150074
Keywords: Yellow fever virus, yellow fever, cerebrospinal fluid, wild-type Yellow fever virus, neurotropic, central nervous system
Citation: Rezende IMd, Cenachi ARC, Costa TA, Oliveira GFG, Rabelo L, Menezes LM, Penido I, Pereira LS, Arruda MS, Gonçalves AP, Alves PA, Kroon EG, Calzavara-Silva CE, Ramalho DB, Martins-Filho OA, Teixeira-Carvalho A, LaBeaud AD and Drumond BP (2022) Wild-type Yellow fever virus in cerebrospinal fluid from fatal cases in Brazil, 2018. Front. Virol. 2:936191. doi: 10.3389/fviro.2022.936191
Received: 04 May 2022; Accepted: 23 August 2022;
Published: 09 September 2022.
Edited by:
Gerald Misinzo, Sokoine University of Agriculture, TanzaniaReviewed by:
Raphaëlle Klitting, The Scripps Research Institute, United StatesPilar Moreno, Universidad de la República, Uruguay
Copyright © 2022 Rezende, Cenachi, Costa, Oliveira, Rabelo, Menezes, Penido, Pereira, Arruda, Gonçalves, Alves, Kroon, Calzavara-Silva, Ramalho, Martins-Filho, Teixeira-Carvalho, LaBeaud and Drumond. This is an open-access article distributed under the terms of the Creative Commons Attribution License (CC BY). The use, distribution or reproduction in other forums is permitted, provided the original author(s) and the copyright owner(s) are credited and that the original publication in this journal is cited, in accordance with accepted academic practice. No use, distribution or reproduction is permitted which does not comply with these terms.
*Correspondence: Izabela Mauricio de Rezende, aXJlemVuZGVAc3RhbmZvcmQuZWR1; aXphYmVsYW1hdXJpY2lvcmV6ZW5kZUBnbWFpbC5jb20=; Betânia Paiva Drumond, YmV0YW5pYWRydW1vbmRAZ21haWwuY29t; YmV0YW5pYWRydW1vbmRAdWZtZy5icg==
†Present address: Izabela Maurício de Rezendem, Department of Pediatrics, Division of Infectious Disease, Stanford University School of Medicine, Stanford, CA, United States
Leticia Mattos Menezes, Hospital das Clínicas, Faculdade de Medicina da Universidade de São Paulo, São Paulo, SP, Brazil