- 1Department of Otorhinolaryngology, Hannover Medical School, Hannover, Germany
- 2Cluster of Excellence “Hearing4all”, Hannover, Germany
Introduction: For several years now, also patients with significant acoustic hearing can receive a cochlear implant in order to combine acoustic and electric hearing. For this purpose, atraumatic electrodes were designed and used as standard. Tools were developed to monitor hearing preservation during surgery.
Methods: For this purpose, Auditory Steady State Responses (ASSRs) were recorded intraoperatively under general anesthesia directly before and after surgical intervention. In contrast to other common electrophysiological methods such as Auditory Brainstem Responses (ABR) or Electrocochleography (ECochG), where transient potentials are measured and evaluated in the time domain for the presence or absence of certain waveforms, with ASSR stationary potentials are measured and evaluated using the phase or frequency of the recorded signal and calculating the probability that a stimulus response is present. ASSR thresholds were recorded at six frequencies (250 Hz, 500 Hz, 1 kHz, 2 kHz, 4 kHz, and 8 kHz) using sine waves. The measurements were completed for 155 ears, with 78 ears being provided with a Nucleus SRA electrode, 55 ears had a Hybrid-L electrode and 22 ears were fitted with other types of electrode.
Results: ASSR threshold shifts correlated to hearing threshold shifts modestly but significantly at 1 kHz (r = 0.34, p < 0.01), where the recorded ASSR thresholds were closer to the hearing thresholds than at 250 and 500 Hz and less variance occurred.
Discussion: This suggests that, in many cases, damage to residual hearing is due to intraoperative adverse events, like trauma to the cochlea or mechanical dampening of the basilar membrane. Therefore, ASSR offers certain prospects for the early detection of damage to residual hearing and thus the option for early intervention. ASSR recordings of all frequencies are time consuming. However, based on the data presented here, we would recommend including a reduced measurement protocol, just at 1 kHz, in a future system for monitoring residual hearing during CI insertion complementary to other possible measurement methods. We would also suggest investigating whether the use of chirp stimuli instead of sine waves would lead to higher correlations.
1 Introduction
Cochlear implants (CIs) are electronic devices designed to restore hearing in patients with severe or profound hearing loss. However, some patients have significant residual hearing in the operated ear, which should be preserved as effectively as possible during surgery.
Indeed, several studies show the benefits, to post-operative speech perception, of combining acoustic and electric stimulation (James et al., 2005; Gantz et al., 2005; Baumgartner et al., 2007; Lenarz et al., 2009; Helbig et al., 2011; Von Ilberg et al., 2011; Incerti et al., 2013; Büchner et al., 2017; Roland et al., 2018).
To this end, particularly soft and thin electrodes and devices have been developed, since the early 2000s, for patients with residual hearing (Adunka et al., 2004; Risi, 2018; Lenarz et al., 2020). Also the surgical techniques were improved to further protect cochlear structures and residual hearing (Lehnhardt, 1993; Giordano et al., 2014; Khater and El-Anwar, 2017; Sikka et al., 2017). This is particularly important for patients with significant residual hearing or even a normal hearing ability in the lower frequency range and a partial deafness in the higher frequencies. The devices are complemented by a hearing aid amplifying the lower frequencies acoustically in the same ear. Different electrode lengths were developed to best match the frequency where the natural hearing of the patient drops down (Büchner et al., 2017; Lenarz et al., 2019; Dhanasingh and Hochmair, 2021). Nevertheless, the patient can only get the best benefit from the combination of acoustic and electrical hearing if the residual hearing is preserved. Most studies confirm that the majority of patients lose at least 10–20 dB in their hearing threshold during implantation (Lenarz et al., 2009; Hunter et al., 2016; Suhling et al., 2016; Lenarz et al., 2020; Schwam et al., 2021; Sipari et al., 2024).
It is not known if this impairment is caused directly during the surgery or by later processes initiated during the surgery, for example inflammatory processes (Seyyedi and Nadol, 2014; Simoni et al., 2020). Determining pure tone thresholds the day after surgery cannot fully answer this question since the first postoperative inflammatory processes have already begun. Therefore, objective measurements at the end of the operation allow more precise information. Also, for treating acutely impaired residual hearing, e.g., with cortisone, an immediate detection is beneficial. One study examines the audiogram within 5 h after surgery, which is already very close (Saoji et al., 2022), although still not immediate.
There are different methods available to monitor residual hearing. Various research groups are currently searching for the best possible objective measurement method for this purpose. In most patients possible residual hearing lies in the low frequency range. The measurement method used should detect stimulus responses as close to the hearing threshold as possible especially in the low frequency range, and the measured stimulus responses should be highly reproducible so that possible changes in the signal can actually be attributed to physiological and surgical influences and contain as few random fluctuations in the measurement signal as possible. The measurement signal should react sensitively to changes, ideally at a time when the surgeon can still stop or even reverse potential damage to the cochlea caused by insertion trauma. Furthermore, the measurement method should consume as little time as possible, since time is particularly precious during surgery. Therefore, several possible methods are currently being investigated.
In recent years, many clinics have monitored the state of the cochlea using Electrocochleography, for reviews see for example Kim (2020) and Barnes et al. (2021). With this method, the responses from the hair cells and the spiral ganglion cells can be measured either extra- or intracochlearly. Consequently, direct conclusions can be drawn as to possible trauma to the basilar membrane or hair cells. This means that the surgeon gets real-time feedback and can adjust his surgical technique immediately if necessary. ECochG comprises of several components measuring different anatomical structures, mainly Cochlear Microphonics (CM), Auditory Nerve Neurophonics (ANN), Compound Action Potentials (CAP), and Summating Potentials (SP) which are described thoroughly in the literature (Eggermont, 1974; Snyder and Schreiner, 1984; Forgues et al., 2014; Buechner et al., 2022; Haumann et al., 2024a). Although some studies found significant correlations between the course of extracochlearly recorded CMs and residual hearing preservation, other studies found no or only very small correlations (Radeloff et al., 2012; Fitzpatrick et al., 2014; Adunka et al., 2016; Dalbert et al., 2016; Abbas et al., 2017; Dalbert et al., 2018; Haumann et al., 2019; Walia et al., 2022; Haumann et al., 2024a). Thus, further improvements are being sought. A second approach for recording electrocochleography is intracochlear recording, directly in the cochlea. Here most commonly the CI electrode itself is used for recording during and after CI electrode insertion (Haumann et al., 2025 and references therein). The main drawback of this approach is that the ideal behavior of the recorded amplitude is not yet clear, as the recording site moves during insertion. There are now many approaches to predict residual hearing preservation from the amplitude behavior of the intracochlear recorded signal, but to date this has only been possible to a certain extent. In theory, the signal amplitude should rise during ongoing electrode insertion. In a recent multicenter study for example it was concluded that if a drop occurs, there is a high risk of later hearing loss, even if the amplitude recovers again (O'Leary et al., 2023). Overall, however, further research is necessary to better understand relationships.
This is why we decided to re-analyze data obtained with another measurement method. In our clinic, the recording of Auditory Steady State Responses (ASSR) was routinely performed for intraoperatively evaluating the residual hearing before ECochG became widespread. ASSR has different source generators in the auditory pathway than CMs or CAPs and, above all, frequency-specific measurements can be carried out here.
In contrast to other common electrophysiological methods such as Auditory Brainstem Responses (ABR) or Electrocochleography (ECochG), where transient potentials are measured and evaluated in the time domain for the presence or absence of certain waveforms, with ASSR stationary potentials are measured and evaluated using the phase or frequency of the recorded signal and calculating the probability that a stimulus response is present. ASSR was introduced 4 decades ago (Galambos et al., 1981) and comprehensive descriptions of the principle and reviews of enhancements were made 20 years ago (Picton et al., 2003; Mühler, 2004). The signal which is used for ASSR consists of a certain carrier frequency which is modulated by a certain modulation frequency. The carrier frequency corresponds to the audiometric testing frequency, and the modulation frequency is needed for signal detection. This steady state signal is presented to the subject.
Initially, the investigated audiometric pure tone frequencies were used as carrier frequencies. By now, chirps are used as a kind of carrier frequency (Elberling et al., 2007; Seidel et al., 2015; Ehrmann-Müller et al., 2021; Mühler et al., 2012). For chirps, the stimulus repetition rate is used instead of the modulation frequency. However, the basic principles remain the same. At the time of our data collection, there were no chirps in clinical ASSR devices available, so modulated sine waves were still used here.
ASSRs are generated throughout the auditory nervous system, with a source analysis suggesting that higher modulation frequencies around 80–90 Hz are generated mainly in the brainstem and lower modulation frequencies are generated mainly in the auditory cortex (Herdman et al., 2002; Alaerts et al., 2009). For clinical application, frequencies near 40 or 80 Hz are suitable. Frequencies near 40 Hz are recommended for waking subjects, as the recorded amplitudes are larger, and frequencies near 80 Hz are recommended for sleeping subjects, because they are less dependent on attention (Cohen et al., 1991; Pethe et al., 2001; Picton et al., 2003). Thus, in our data, high modulation frequencies around 80 Hz were used. The recorded steady state potential follows the modulation rate. Thus, with scalp or needle electrodes the EEG signal can be recorded, and with statistical methods, the presence of a response at the modulation frequency can be tested.
With the detection of a response at the modulation frequency it is assumed that the subject has also perceived the carrier frequency. Nevertheless, several studies show a large variety in closeness between the recorded ASSR threshold and the pure tone threshold with awake subjects, for sine wave ASSR (Tomlin et al., 2006; Tlumak et al., 2007; Liebler et al., 2008; Vander Werff, 2009; Hatzopoulos et al., 2010). The test-retest stability of ASSR amplitudes varies largely (Wilding et al., 2012), but the reproducibility of the detected ASSR thresholds is nevertheless considered acceptable (D'haenens et al., 2008).
As the accuracy of recorded ASSR threshold largely depends on the calmness of subjects (Don and Elberling, 1996; Picton et al., 2005; Mühler and Rahne, 2009), results obtained under general anesthesia are more reliable (Mühler and Rahne, 2009; Luts et al., 2006). Thus, we recorded ASSR thresholds intraoperatively, to evaluate hearing preservation during cochlear implantation. In this manuscript, the data is re-evaluated and the correlations to the hearing preservation were analyzed. The aim of this re-analysis is to investigate whether the relationships between intraoperative ASSR measurements and audiograms are closer than with ECochG measurements, and whether there are differences between different electrode lengths. For this purpose, three aspects were investigated: Firstly, the frequency-specific relationships between ASSR thresholds under general anesthesia (before the start of surgical intervention) and pure tone thresholds before CI surgery were investigated in order to analyze the differences between the thresholds determined with both methods. Secondly, a comparison of all recorded thresholds before and after surgery was conducted in order to investigate whether a change in the hearing threshold before and after surgery can already be detected by a change in the ASSR thresholds pre- and postop. Thirdly it is investigated whether ASSR threshold shifts in the low frequency range can be used to predict the later allocation to hearing preservation groups.
2 Methods
2.1 Surgery and recording setup for ASSR
Our clinical routine for implanting atraumatic electrodes included the intraoperative recording of ASSR. This retrospective analysis includes 155 data sets from adult patients with residual hearing who underwent regular cochlear implantation. The study was approved by the local ethics committee (approval number 1897–2013) and is in accordance with the ethical standards of the Declaration of Helsinki.
The recordings were done under general anesthesia directly before and after the surgical intervention. As recording device the clinical GSI Audera system was used (Eden Prairie, Minnesota, USA). The six carrier frequencies, which were investigated in a single stimulus paradigm with 64 sweeps per trace, covered 250 Hz−8 kHz. Sine waves with an amplitude modulation by modulation frequencies near 80 Hz were used. The details are given in Table 1. The surgical intervention was conducted according to our standard protocol reported elsewhere (Lenarz et al., 2022).

Table 1. Details of the applied stimuli for intraoperative ASSR and pure tone audiometry before and after cochlear implantation.
The stimuli were presented with insert earphones (TIP-50, GSI, Madison, WI, USA). Therefore, sterilized foam plugs were placed in the outer ear canal. The EEG signal was recorded with subdermal needle electrodes (MedTronic, Minneapolis, MI, USA) at the vertex (+), the ipsilateral earlobe (–) and the forehead (0).
The ASSR thresholds were determined in dB HL with a manual rule. For each carrier frequency the stimulus level was started 20 dB HL above the preoperative pure tone threshold, but at most at 90 dB HL. The recording of each loudness level was stopped either if a response could be detected on a probability of p > 99% or if no response could be detected within 64 sweeps. Initially, the level was varied in 10 dB steps; when coming closer to the threshold 5 dB steps were used. A presumed threshold was confirmed if at least the two responses 5 and 10 dB below the presumed threshold level failed. For the recording after surgical intervention the remaining liquid was carefully evacuated from the ear canals. For time saving reasons the stimulus was started at the level which was determined as threshold level before surgical intervention for each frequency. For accepting or declining a response, the same rule was applied as that used before surgical intervention.
The ASSR thresholds were compared to each other and to the pure tone thresholds. For this analysis the air conduction pure tone thresholds obtained pre-operatively and at the first fitting (5 weeks after surgery) were used. This was chosen, as the thresholds shortly after surgery are often affected by liquid in the middle ear. The pure tone thresholds were measured using the devices AD17 or AD2017 from Audio-DATA (Duvensee, Germany) together with the HDA200 headphone from Sennheiser (Wedemark, Germany). The maximum stimulation levels are given in Table 1.
2.2 Patients
In this analysis 151 patients (155 ears) with 57 male and 98 female ears were included. Seventy-eight of the fittings were with a Cochlear SRA (Nucleus CI422) electrode and 55 with a Cochlear Hybrid-L electrode (Nucleus CI24RE Hybrid-L24). 22 patients were provided with MED-EL electrodes of different lengths (2x EAS, 16x EAS20, 4x EAS24). All surgeries were performed unilaterally. For the four patients who received sequentially bilaterally two implants, both surgeries were analyzed independently. The demographics of the samples are given in Table 2.

Table 2. Demographic data, given in mean ± standard deviation (minimum – maximum), summarized for the SRA sample, the Hybrid-L sample and the full group.
2.3 Analysis
The pure tone audiogram data sets were classified into hearing preservation groups according to Suhling et al. (2016). Here the low frequency pure tone average (PTAlow) was calculated as mean of the pure tone thresholds of 250, 500, and 1000 Hz. In case of no stimulus response at the highest stimulation level for each stimulation frequency, the response was set, similar as to in Haumann et al. (2019), to 10 dB above the stimulation limit, for calculating the PTAlow (see Table 1). The PTAlow shift between pre- and postoperative data was classified to hearing preservation (HP) group 1 (< 15 dB shift), HP group 2 (15–30 dB shift) and HP group 3 (more than 30 dB shift). In a similar manner, the ASSRlow was calculated as mean of the thresholds at 250, 500, and 1000 Hz and frequencies with no response were set to 10 dB above the stimulation level. All data analysis was performed using MATLAB R2017a (The Mathworks, Inc., Natick, Massachusetts, USA).
3 Results
3.1 ASSR thresholds under general anesthesia vs. pure tone thresholds before CI surgery
Firstly, all preoperative ASSR thresholds under general anesthesia were compared to the pure tone thresholds before surgery. Therefore, the data of all patients at all frequencies was analyzed independent of the chosen implants. The average thresholds for pure tone and ASSR are given in Figure 1.
The differences between the ASSR thresholds and pure tone thresholds depended on the frequency. Pure tone was always better on average (Figure 2). For 250 Hz the difference in threshold was 24.8 ± 16.8 dB (mean ± standard deviation). For 500 Hz the difference was 22.8 ± 14.4 dB, for 1 kHz it was 13.0 ± 14.1 dB, for 2 kHz it was 5.8 ± 14.1 dB, for 4 kHz it was 9.3 ± 10.3 dB and for 8 kHz it was 10.3 ± 11.8 dB. There was good correlation between pure tone thresholds before surgery and preoperative ASSR thresholds under general anesthesia. With the exception of 8 kHz, where only few patients had measurable hearing anyway, all detected correlations were highly significant (p < 0.001). For 250 Hz the correlation coefficient was r = 0.67***, for 500 Hz it was r = 0.73***, for 1 kHz it was r = 0.62***, for 2 kHz it was r = 0.55***, for 4 kHz it was r = 0.79***, and for 8 kHz it was r = 0.30 (p = 0.3).
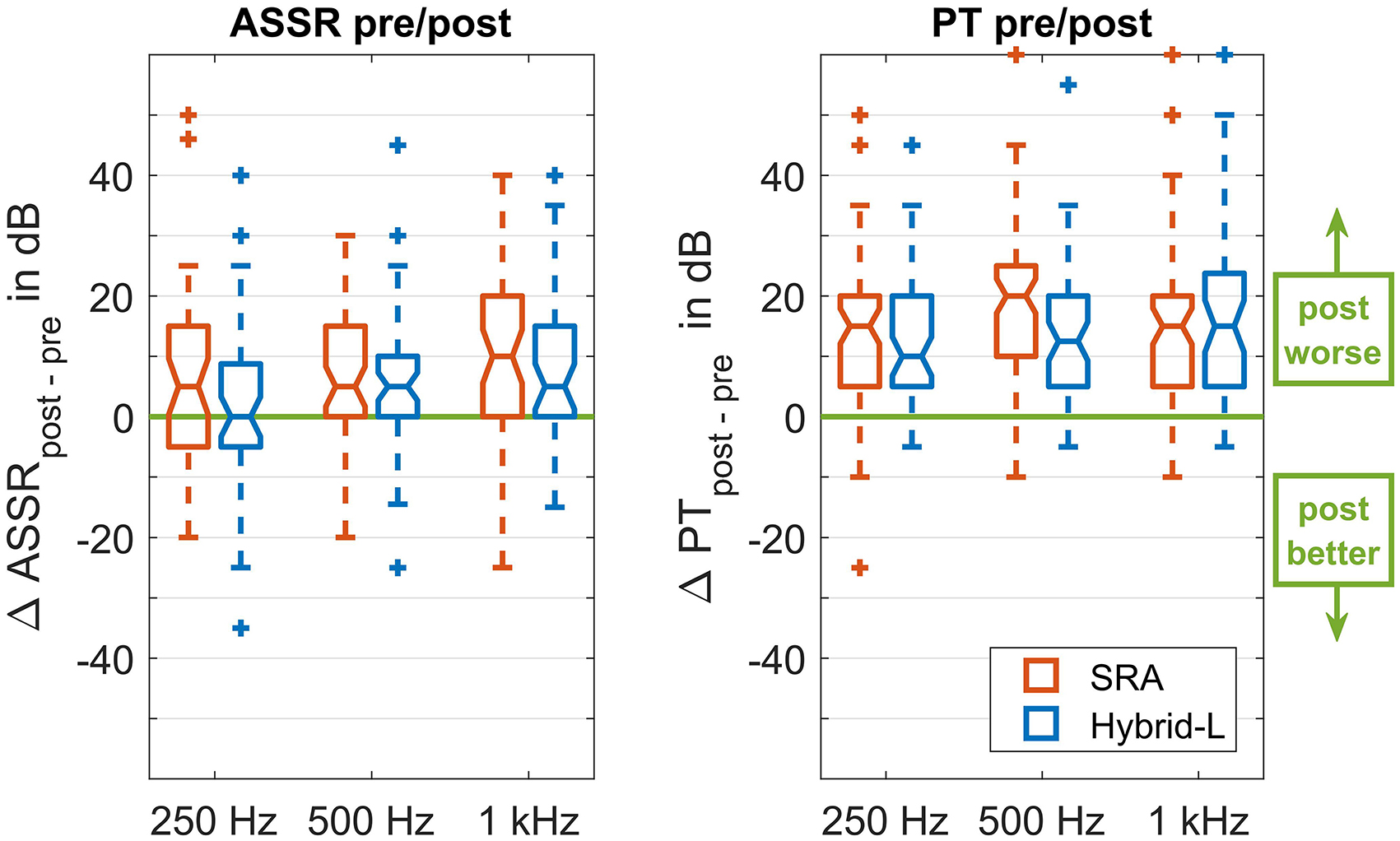
Figure 2. Group differences between the obtained thresholds, given as boxplots. The left panel represents the differences between the ASSR thresholds before and after the implantation, both recorded under general anesthesia. The right panel represents the differences between the pure tone threshold directly before surgery and 5 weeks after surgery at the first fitting appointment. In each panel there are three pairs of boxes, representing from left to right the three carrier frequencies (250, 500, and 1000 Hz). Each pair consists of a left (red) box representing the SRA sample and a right (blue) box representing the Hybrid-L sample.
The majority of patients had better residual hearing in the low frequency range than in the high frequency range. Accordingly, ASSR responses were found in most of the cases especially in this frequency range. However, there were some data points where no threshold could be obtained also at maximum stimulation level. Figure 3 shows these data points dependent on the pure tone hearing thresholds. However, in all patients there were preoperative ASSR responses detected in at least one frequency.
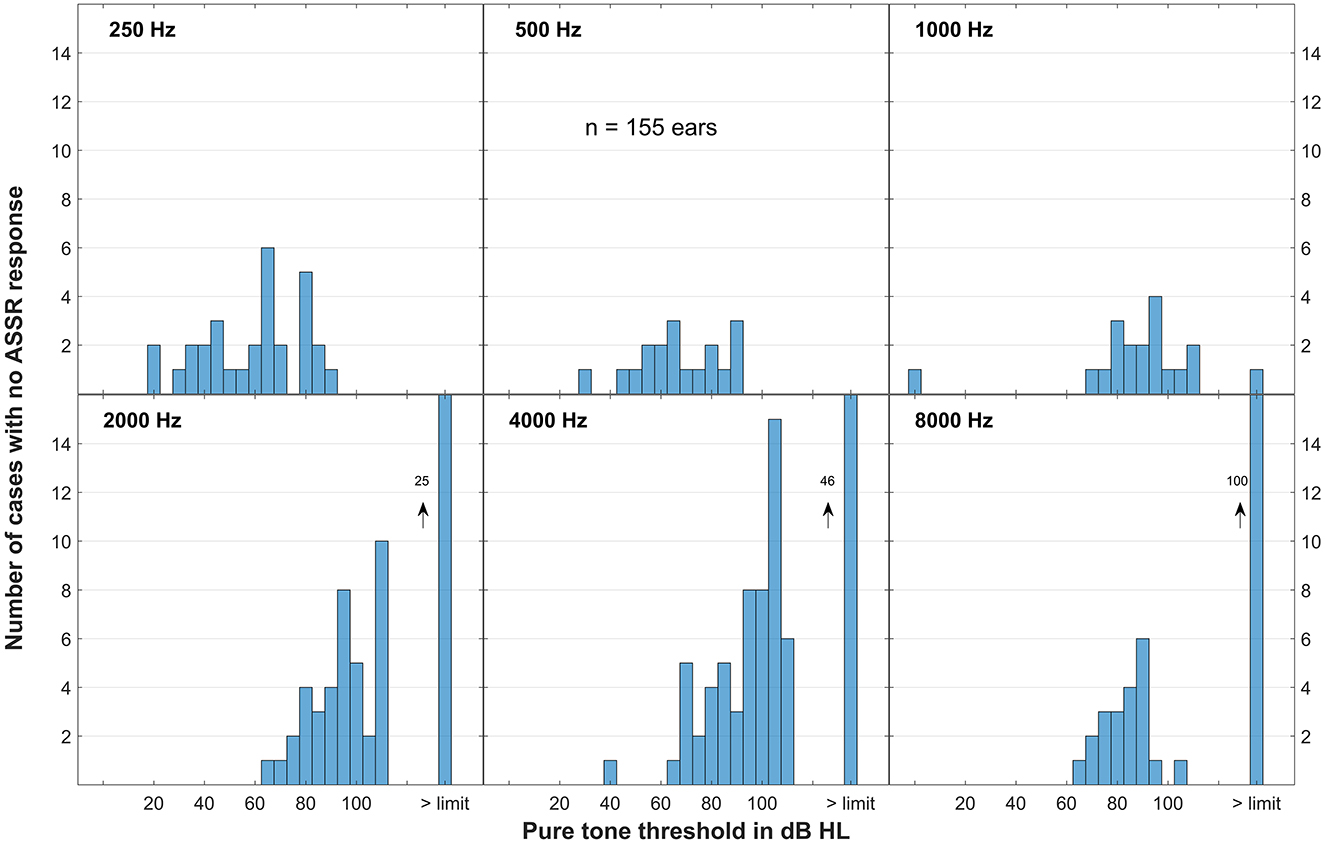
Figure 3. Data points where no ASSR responses could be obtained at maximum stimulation level of the ASSR recording device, presented as histogram for all frequencies. On the x-axis the pure tone thresholds are given, on the far right are the cases where the pure tone threshold also exceeded the audiometer limits. On the y-axis the numbers of the cases are given where no ASSR response could be obtained for the allocated pure tone threshold. Altogether there were 155 cases.
3.2 Thresholds before and after surgery
For a controlled analysis, from now on this analysis concentrates on patients receiving a Nucleus implant, separated into the two groups receiving a Hybrid-L electrode and an SRA electrode. Three subjects, one receiving a Hybrid-L and two an SRA, had to be excluded from analysis, because postoperative ASSR recording could not be performed for organizational or medical reasons. As pure tone thresholds, the data before surgery (usually 1 day before surgery) and postoperatively at the first fitting appointment (usually 5 weeks after surgery) were used. In five cases no audiogram was measured at the first fitting appointment, in these cases the audiogram after surgery was used for analysis. Figure 4 gives the thresholds before and after surgery for some example cases.
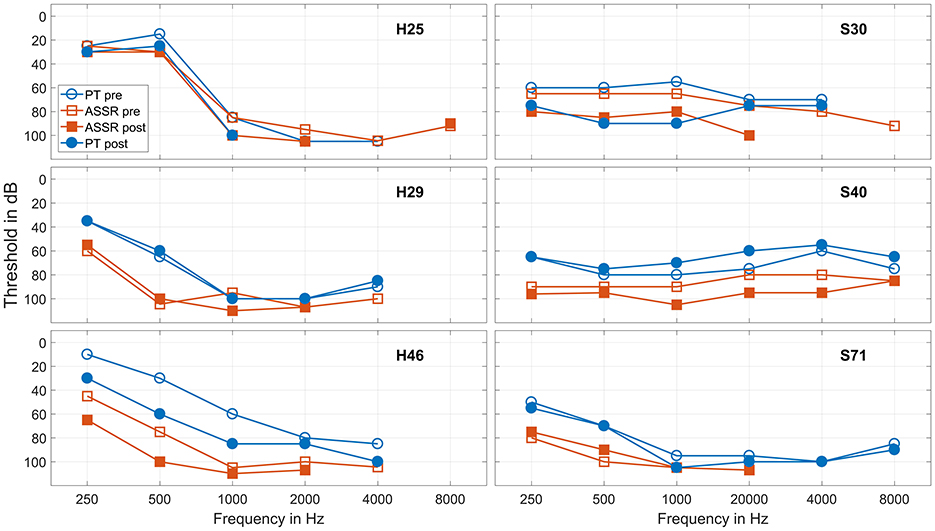
Figure 4. Recorded thresholds for six frequencies in six example cases. The three cases in the left panels received a Hybrid-L electrode, the three cases in the right panels a SRA electrode. The blue circles represent the pure tone thresholds before surgery and at the first fitting appointment, the red squares represent the ASSR thresholds under general anesthesia before and directly after surgical intervention. The hollow markers represent the data before and the full markers the data after surgery.
As in the overwhelming majority of the patients the residual hearing lies in the lower frequency range, the data sets in the higher frequency range were sparse. Following, this analysis concentrates onto the three frequencies 250, 500, and 1000 Hz. Also, the low frequencies are the range that is most relevant to the application described.
The obtained thresholds in the low frequency range, separated for the SRA and the Hybrid-L-samples, are given in Figure 5. The differences between both electrodes were analyzed using the Mann-Whitney-U-Test (MATLAB function ranksum). For 250 Hz all differences between the SRA sample and the Hybrid-L sample were highly significant (p < 0.001, in detail z = −7.53 for pure tone thresholds before surgery, z = −5.08 for ASSR thresholds in general anesthesia before surgical intervention, z = −5.88 for ASSR thresholds in general anesthesia after surgical intervention and z = −6.74 for pure tone thresholds at the time of the first fitting, 5 weeks after surgery). Also for 500 Hz all differences were detected to be highly significant (p < 0.001, in detail z = −7.14 for PT before surgery, z = −5.37 for ASSR thresholds before surgical intervention, z = −5.26 for ASSR thresholds after surgical intervention and z = −6.75 for PT at FF). For 1 kH, there were trends detected for the pure tone thresholds, but no significant differences for the ASSR thresholds (z = −1.75, p = 0.08 for PT before surgery, z = −0.81, p = 0.42 for ASSR thresholds before surgical intervention, z = −1.07, p = 0.29 for ASSR thresholds after surgical intervention and z = −1.70, p = 0.09 for PT at FF).
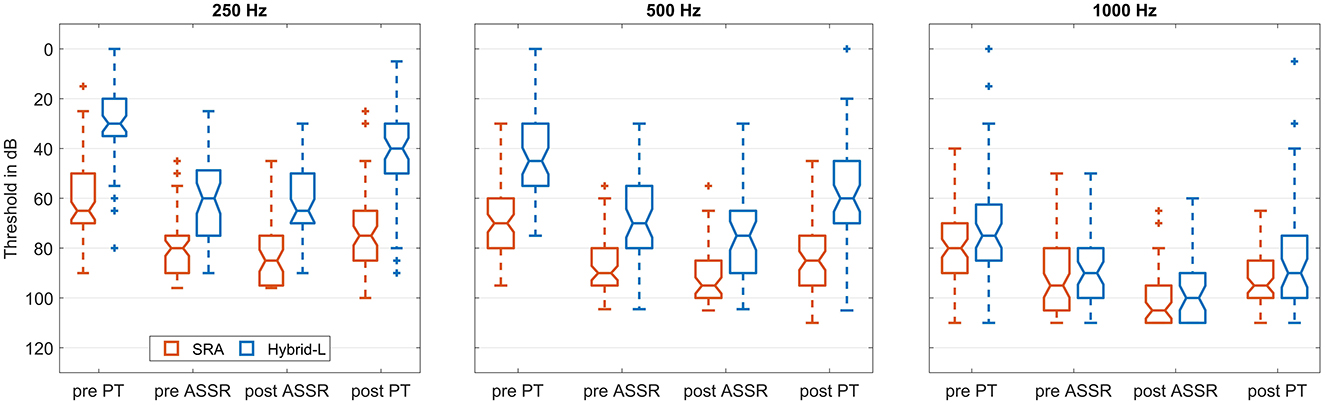
Figure 5. Boxplot showing obtained thresholds for SRA and Hybrid-L at the three indicated frequencies. The left (red) box of each pair represents the data from the SRA sample and the right (blue) box the data of the Hybrid-L sample. The four groups of box pairs represent (from left to right) the pure tone threshold before surgery, the ASSR threshold in general anesthesia before the surgical intervention, the ASSR threshold in general anesthesia after the surgical intervention and the pure tone threshold at the first fitting appointment 5 weeks after surgery.
The group differences between the obtained thresholds are illustrated in Figure 6 and analyzed using the Wilcoxon-Test (MATLAB function signrank). Nearly all differences were found to be highly significant (p < 0.001), if not, it is indicated separately. In detail, the z-values between the pure tone thresholds before surgery and at the first fitting appointment were z = −5.95 for the Hybrid-L sample and z = −6.13 for the SRA sample for 250 Hz, z = −6.18 for the Hybrid-L sample and z = −6.62 for the SRA sample for 500 Hz and z = −5.67 for the Hybrid-L sample and z = −6.21 for the SRA sample for 1 kHz. The z-values between the ASSR thresholds in general anesthesia before and after surgical intervention were: z = −0.93 (p = 0.35) for the Hybrid-L sample and z = −2.49 (p = 0.02) for the SRA sample for 250 Hz, z = −3.04 (p = 0.002) for the Hybrid-L sample and z = −3.68 for the SRA sample for 500 Hz and z = −3.88 for the Hybrid-L sample and z = −4.87 for the SRA sample for 1 kHz. The z-values between the pure tone thresholds before surgery and the ASSR thresholds in general anesthesia before surgical intervention were: z = −5.66 for the Hybrid-L sample and z = −6.10 for the SRA sample for 250 Hz, z = −5.85 for the Hybrid-L sample and z = −6.79 for the SRA sample for 500 Hz and z = −4.77 for the Hybrid-L sample and z = −6.14 for the SRA sample for 1 kHz. The z-values between the pure tone thresholds at FF and the ASSR thresholds in general anesthesia after surgical intervention were: z = −5.54 for the Hybrid-L sample and z = −4.58 for the SRA sample for 250 Hz, z = −5.50 for the Hybrid-L sample and z = −3.69 for the SRA sample for 500 Hz and z = −3.86 for the Hybrid-L sample and z = −3.53 for the SRA sample for 1 kHz.
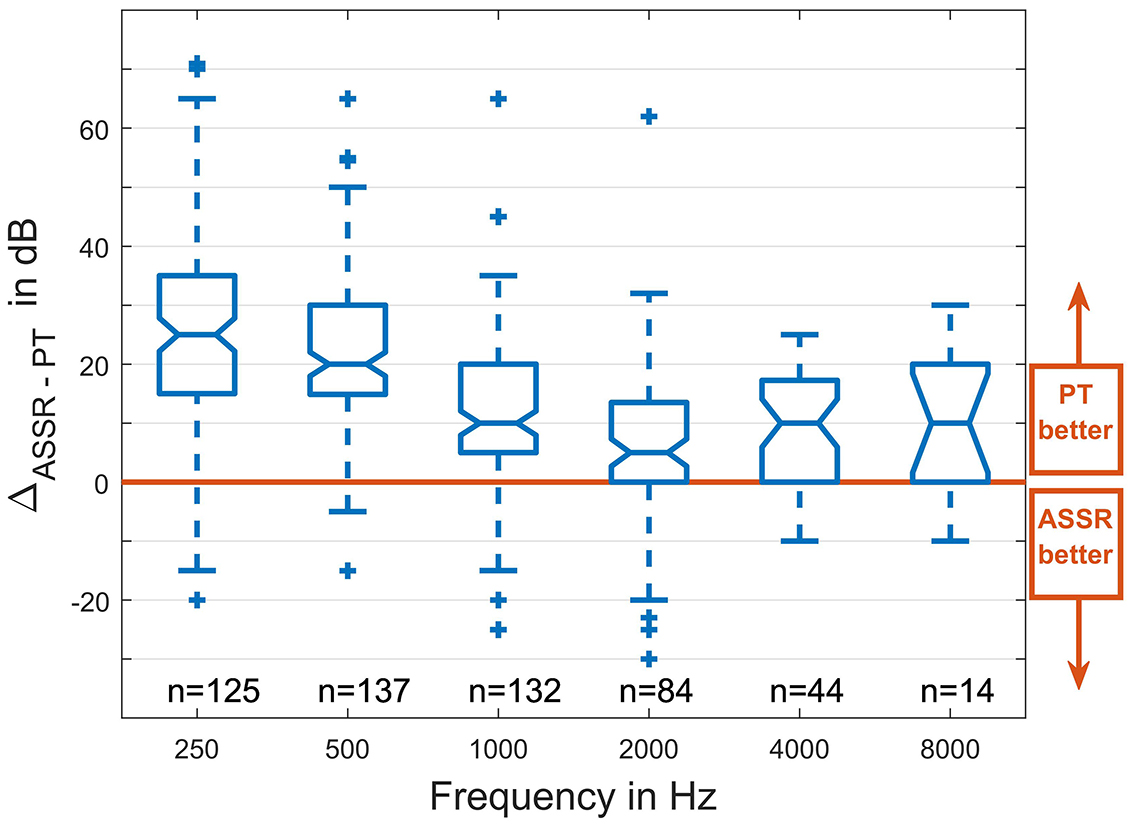
Figure 6. Differences between the recorded pure tone (PT) and ASSR thresholds for all six frequencies and all patients, presented as boxplots. All thresholds were obtained before surgery (PT), respectively, under general anesthesia before the surgical intervention was started (ASSR). The horizontal lines in the boxes indicate the median of the group, the lower and upper horizontal lines represent the lower and upper quartile (25% and 75% quartiles, respectively), and the whiskers indicate the last data point lying in the 1.5 times of the interquartile (the difference between the 25% and the 75% quartile). The small crosses indicate outliers.
The shifts in the pure tone audiogram are compared to the shifts in the ASSR thresholds in Figure 7. Pearson's correlation coefficients, as calculated with the MATLAB function corrcoef, are given.
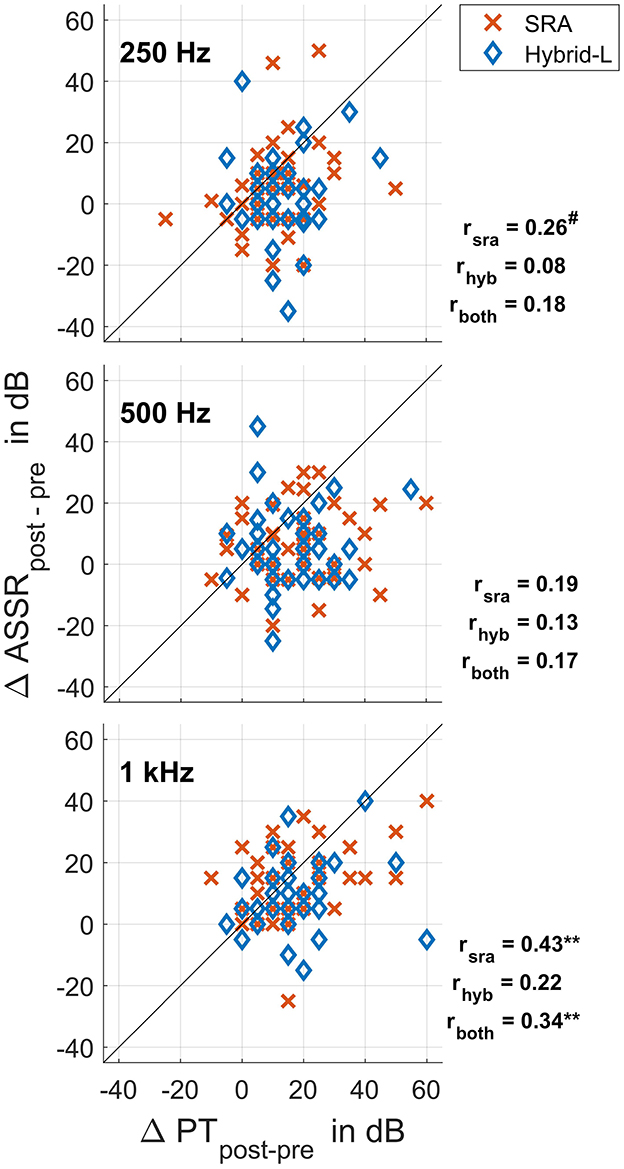
Figure 7. Differences in the ASSR thresholds recorded intraoperatively before and after surgical intervention plotted against differences in the pure tone thresholds before surgery and at the first fitting appointment (5 weeks after surgery), separated for the Hybrid-L and SRA. The drawn bisector marks the ideal relationship. Also the correlation coefficients are given with three correlations being marked as trend (#p < 0.1), respectively, high significant (**p < 0.01).
3.3 ASSR threshold shifts and hearing preservation groups
The patient data sets were classified into the three HP groups according to where Δ PTAlow falls with respect to cut-offs at 15 and 30 dB i.e., HP group 1 means Δ PTAlow ≤ 15 dB, HP group 2 means 15 dB < Δ PTAlow ≤ 30 dB and HP group 3 means Δ PTAlow > 30 dB (similar to Suhling et al., 2016). The distribution among the groups is shown in Table 3, and the relations to the recorded ASSR threshold shifts are illustrated in Figure 8. The differences between the groups were investigated using the Mann-Whitney-U-Test (MATLAB function ranksum). The ASSR threshold shifts did not differ significantly between HP group 1 and 2 (z = −1.58, p = 0.11 for the SRA sample, z = −0.18, p = 0.86 for the Hybrid-L sample and z = −1.38, p = 0.17 for the whole sample). The ASSR threshold shifts between HP group 2 and 3 differed significantly for the SRA sample (z = −2.16, p = 0.03) and the whole sample (z = −2.77, p = 0.006), but not for the Hybrid-L sample alone (z = −1.53, p = 0.13). Similarly, the ASSR threshold shifts between HP group 1 and 3 differed significantly for the SRA sample (z = −3.28, p = 0.001) and the whole sample (z = −3.77, p < 0.001), but not for the Hybrid-L sample alone (z = −1.54, p = 0.12). There were no significant differences in the ASSR shifts between the two samples (SRA and Hybrid-L) detected [z = −0.13, p = 0.90 within HP group 1, z = −1.48, p = 0.14 within HP group 2 and p = 0.77 within HP group 3 (z-value not determinable)].

Table 3. Distribution of the hearing preservation groups, similar to Suhling et al. (2016).
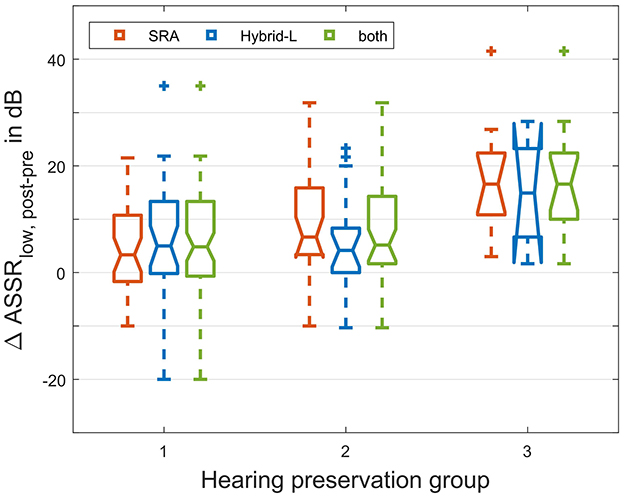
Figure 8. Allocation of the shift in the intraoperative ASSR threshold to the hearing preservation group, separated for the SRA sample and the Hybrid-L sample and given for the whole group. The low frequency ASSR threshold is calculated as mean of the threshold at 250, 500, and 1000 Hz. HP group 1 means Δ PTAlow ≤ 15 dB, HP group 2 means 15 dB < Δ PTAlow ≤ 30 dB, and HP group 3 means Δ PTAlow > 30 dB (similar to Suhling et al., 2016).
4 Discussion
Here we evaluated residual hearing preservation during cochlear implantation by retrospective analysis of ASSR recordings of large patient collective receiving different kinds of cochlear implant (CI) electrodes. Electrocochleography is currently the most widely used recording method. Various research groups have found a mixed picture about the relationships between extracochlear ECochG measurements and cochlear health. Also with intracochlear measurements, some questions still remain unanswered. ASSR has different source generators in the auditory pathway than CMs or CAPs and frequency-specific measurements can be carried out here. The aim of this re-analysis is to investigate whether the relationships between intraoperative ASSR measurements and audiograms are closer than with ECochG measurements, and whether there are differences between different electrode lengths. To our knowledge, no other clinic has used ASSR for evaluating hearing preservation during cochlear implantation up to now. Thus, we have re-analyzed our ASSR data here, with an eye to possible combined measurement protocols which could merge the advantages of both methods.
4.1 Hearing preservation as measured by pure tone audiometry
The hearing thresholds before surgery, as measured by pure tone audiometry, were better in the Hybrid-L sample than in the SRA sample. This effect can of course be explained by the indication, as patients with better residual hearing received a Hybrid-L electrode and patients with less residual hearing received an SRA electrode. For evaluating hearing preservation the pure tone thresholds, at the first fitting appointment 5 weeks after surgery, were used. This appointment was chosen, as directly after surgery, there may be some liquid in the middle ear, which should be resolved at the time of the first fitting. On the other hand, after 5 weeks the hearing thresholds are more influenced by factors related to the surgery (e.g., electrode insertion trauma or foreign body responses), but the underlying disease leading to the hearing loss of the patient should not be much more advanced (Haumann et al., 2024b).
The majority of the data sets presented here have already been examined for residual hearing preservation in Jurawitz et al. (2014), so the focus of this work lies on the ASSR recordings. The SRA electrode is longer than the hybrid electrode and is inserted deeper into the cochlea. The shorter electrode has a better chance of preserving residual hearing ant thus electric-acoustic stimulation, but the longer electrode is better for speech perception with CI only if residual hearing is ultimately lost (Jurawitz et al., 2014; Büchner et al., 2017). Therefore, the ASSR measurements for the two electrodes are evaluated separately in order to obtain approaches as to whether different measurement frequencies might be better suited to represent residual hearing preservation for different electrode lengths.
4.2 Stimulus response thresholds as recorded by ASSR
ASSR is a widely used method for objectively determining hearing thresholds, which is mainly relevant in the clinic for children, but of course also has to be examined on adults in order to be able to draw comparisons to the hearing threshold. A major advantage of ASSR measurements is that they can be carried out frequency-specifically. There are several publications where the accuracy of hearing threshold determination in adults is examined, but the number of patients is usually small. Therefore, in the first part of our study we compared the accuracy of the hearing threshold estimation based on the difference between pre-insertion sine wave ASSR thresholds and preoperative pure-tone hearing thresholds.
The individual studies are not entirely comparable with each other because there are differences between the accuracy of the hearing threshold estimation in the sense of small differences between ASSR thresholds and audiometric pure tone thresholds. This depends, for example, on whether the measurements are conducted on people with normal hearing or people with hearing loss (Picton et al., 2005). Here, the absolute differences were smaller in hearing impaired patients than in normal hearing subjects, but the standard deviations were higher. This was confirmed in our data in that the deviations are significantly higher in the frequencies with less hearing loss than with the greater hearing loss. However, the standard deviations are also higher in the frequencies with less hearing loss, which contradicts the study cited. In clinical practice, absolute differences between the hearing threshold and the ASSR threshold are in general not so relevant for evaluating the measurement method, as these can be corrected using linear factors. The standard deviation is more problematic for threshold estimations.
Another highly relevant factor for the accuracy of the hearing threshold estimate is the patient's calmness or restlessness. Mühler et al. showed that high EEG amplitudes in awake patients reduced the accuracy in the recorded ASSR thresholds (Mühler and Rahne, 2009). This means that the measurements under general anesthesia which we performed here in our study should produce better results. Nevertheless, our recordings were done in a noisy operation theater, and in the cited works the recordings were performed with the subjects lying in a shielded booth.
Considering these limitations, our study provides similar accuracies in hearing threshold estimation using sine wave ASSR as described in the literature and therewith confirms the results on a large patient collective. So, although the method appears to be applicable for hearing threshold estimation in a clinical setting, it is not optimal. However, after conducting our study presented here, a significant improvement in hearing threshold estimation was developed with the implementation of the Chirp-ASSR which is now standard in all clinical devices. The accuracy of the detected thresholds is clearly better (Mühler et al., 2012; Seidel et al., 2015), so also for future applications of ASSR to hearing preservation in CI surgery, chirp stimuli should be used.
4.3 Hearing preservation as recorded by ASSR
For the application described in this analysis, the test-retest stability of the recording method is the most important property. Only if the recorded thresholds at two different points in time are comparable on an individual level can differences in the thresholds measured pre- and postoperatively be attributed to a change in residual hearing. According to Wilding et al. (2012), the ASSR amplitudes cannot be used, as they were detected to vary largely across appointments. But according to D'haenens et al. (2008) the test-retest-stability of the thresholds lies in a clinically acceptable range, so we chose the threshold detection for our protocol.
A central question about preserving residual hearing is whether deterioration of residual hearing is due to adverse events during CI electrode insertion, e.g., intracochlear trauma or mechanical dampening of the basilar membrane by the inserted electrode (Haumann et al., 2025 and therein cited literature). If the recorded ASSR thresholds before and after surgical intervention are stable and the test-retest stability of the method lies in an acceptable range, the residual hearing should be preserved at the end of surgery and in case of deterioration of hearing this would occur afterwards due to e.g., inflammatory processes (Simoni et al., 2020). In the case of adverse events the intraoperatively recorded threshold after surgical intervention will likely be lower than before. Answering this question generally helps to find approaches to the extent to which surgical techniques need to be further improved in the future and to what extent inflammatory processes need to be prevented. Based on the measurement results, a decision can also be made about drug therapy in the individual cases, for example. This question cannot be fully answered with objective measurements before and after insertion because both test-retest stability and the surgical intervention between the two measuring points have a large influence onto the recordings. However, approximations can be made via various points. In our data, ASSR thresholds before and after surgery differed significantly for the SRA sample at 250 Hz and for both samples at 500 and 1000 Hz, which suggests that in a significant number of patients, harmful influences on their residual hearing occurred during the operation. In the Hybrid-L group there were no significant differences detected at 250 Hz. The reason for this could be that the Hybrid-L electrode is shorter than the SRA electrode and therefore remains further away from the tonotopic region of 250 Hz, so that the residual hearing at 250 Hz should be less at risk for intracochlear trauma.
If deterioration of residual hearing is due to adverse intraoperative events, the shifts in the recorded ASSR thresholds at the beginning and end of surgery should correspond to the shifts in the hearing threshold before and after surgery. The correlations between the threshold shifts found for the Hybrid-L sample were lower than for the SRA sample. This could be because the patients receiving a Hybrid-L electrode have more residual hearing due to the indication and therefore have more room for variance or postoperative deterioration. Statistical analyses revealed a trend for 250 Hz for the SRA sample, but neither for the Hybrid-L sample nor for the whole group. For 500 Hz no significant relationships could be detected at all, but for 1 kHz high significant correlations were detected for the SRA sample alone as well as for the whole group. Although the correlation is statistically significant, it is modest and explains only a relatively small part of the variance. This nevertheless suggests that intraoperative adverse events could be detected at 1 kHz to some extent. One reason for the best correlation at 1 kHz could be that the recorded ASSR thresholds were closer to the hearing thresholds at 1 kHz than at 250 and 500 Hz and less variance occurred which could be shown in our preoperative data. This effect would make the correlations stand out more clearly.
If residual hearing preservation is divided more roughly into three groups according to Suhling et al. (2016) the question is to what extent the intraoperative low frequency ASSR threshold shift can predict allocation to hearing preservation groups with Δ PTAlow ≤ 15 dB, 15 dB < Δ PTAlow ≤ 30 dB, and Δ PTAlow > 30 dB. For the Hybrid-L sample alone no significant correlations between ASSR threshold shift and allocation to hearing preservation groups could be detected. For the SRA sample there were significant correlations between ASSR threshold shift and allocation to best and worst as well as allocation to medium and worst hearing preservation group. However, there was no correlation between ASSR threshold shift and allocation to best and medium hearing preservation group. Thus, ASSR threshold shifts seem to be useful for predicting a deterioration of hearing of more than 30 dB.
4.4 ASSR vs. electrocochleography
Extracochlear electrocochleography recordings can also be used to measure before and after insertion. In most clinical protocols for extracochlear Electrocochleography is measured directly before and immediately after CI electrode insertion, while we measured ASSR at the beginning and end of the entire surgical intervention. Several research groups investigated the correlations between extracochlear ECochG and preservation of residual hearing. An important metric is the threshold or amplitude shift of ECochG before and after insertion vs. the audiometric threshold shift before and after surgery. Radeloff et al. (2012) performed the recording only at n = 6 subjects. Due to the small number of participants, there is no statistical analysis performed, but they state that large traumata to the cochlear may be detected. Dalbert et al. (2016) provided similar results. Due to the small number of subjects (n = 14), they did not calculate statistics, but also stated that large traumata to the cochlea may be detected with extracochlear ECochG. In a later study they included n = 20 subjects and detected a correlation between the low frequency ECochG response shift before and after CI electrode insertion and the audiometric threshold shift before and after surgery (Dalbert et al., 2018). The correlation coefficient was r = −0.44, explaining 19.4% of the variance, but it was statistically only a trend (p = 0.055), as the number of subjects was still quite small. Adunka et al. (2016) performed their study with n = 31 subjects. They did not provide the exact correlation values, but stated that it was not significant. Haumann et al. (2024a), too, compared the shifts in the measured electrophysiological thresholds to the shifts in the audiometric thresholds between preoperative appointment and 5 weeks after surgery. The data was obtained on a large patient collective (n = 121 ears), Here, it was initially evaluated whether the patient collective was large enough for a statistically valid statement. However, this study only revealed the correlations of r = 0.18 (explaining 3.2% of the variance) at 250 Hz, r = 0.09 (explaining 0.8% of the variance) at 500 Hz and r = −0.003 (explaining no variance) at 1 kHz, none of them being statistically significant.
To our knowledge, ASSR was not used for monitoring hearing preservation during CI surgery in the literature. Our analysis included a large patient collective (n = 133) and revealed the correlations of r = 0.18 (explaining 3.2% of the variance) at 250 Hz, not significant, r = 0.17 (explaining 2.9% of the variance) at 500 Hz, not significant, and r = 0.34 (explaining 11.6% of the variance) at 1 kHz, being statistically high significant (p < 0.01). Therefore, ASSR seems to be superior to electrocochleography with respect to relations between electrophysiological and audiometric threshold shifts.
In Haumann et al. (2024a), also the prognostic capacity of amplitude curves groups during CI electrode insertion for allocating to hearing preservation group was analyzed, finding several trends but no significant correlations. Our finding of a significant correlation thus underlines the superiority of ASSR to extracochlear electrocochleography.
One disadvantage of electrocochleography is that the signals are recorded in the inner ear and therefore downstream anatomical structures of the auditory pathway are not covered. With ASSR, this disadvantage is largely overcome.
The biggest disadvantage of ASSR recordings is the comparatively long measurement time, which is why we discontinued the measurement in favor of electrocochleography. Due to the long measurement time, it is important to consider whether the results justify the effort. On the other hand, experience with extracochlear electrocochleography has shown that the use of ASSR provides better information. Also with intracochlear recordings, not all questions can yet be answered. So realistic supplementary options should definitely be considered, for example by limiting the measurement frequency to 1 kHz where the best correlations were detected.
An ASSR recording during insertion of the CI electrode cannot realistically be carried out. The intraoperative procedure before and after the surgical intervention also takes much time, especially if all frequencies are measured. A reduction to one frequency could potentially be a breakthrough. However, today's clinical devices are optimized for simultaneous multi-frequency measurements. Due to different internal evaluation algorithms, the measurement for the individual traces takes longer than with single frequency paradigms which is why not as much time can be saved when measuring individual frequencies. With the analysis algorithm of the older GSI Audera, a measurement track (one measurement frequency and one stimulation level) lasts a maximum of 1 min. With the multi-frequency analysis algorithms of current devices, a measurement track (all measurement frequencies and one stimulation level) takes up to 6 min. For a realistic implementation of the ASSR measurement as online monitoring, one would have to return to the previous algorithm and even improve it. The best frequency would be 1 kHz, where high significant correlations could be detected to residual hearing preservation.
Despite the procedural difficulties, ASSR provides more comprehensive information about the status of residual hearing preservation than electrocochleography especially in terms of frequency specificity and correlation to hearing preservation. This information is available long before a postoperative hearing test, allowing for an early intervention.
5 Conclusion
In this retrospective analysis, we found intraoperative ASSR recordings helpful for monitoring residual hearing preservation during cochlear implantation. The best correlation was found for threshold shifts of ASSR and PT at 1 kHz. This suggests that in many cases damage to residual hearing is due to intraoperative events, and this offers good prospects for the early detection of damage to residual hearing and thus the option for early intervention. The main advantage of ASSR compared to the widely used electrocochleography lies in the frequency specificity especially in the low frequency range. The disadvantage is the long measurement time, especially when the recordings are performed with all available frequencies. We used sine-wave ASSR, but with Chirp-ASSR better results should be possible. Stimulation with these chirps is currently standard, so we expect even better results in future implementation. Due to the long measurement time, it is important to consider whether the results justify the effort. For future hearing preservation monitoring systems, we would therefore suggest integrating an ASSR measurement as complementary recording method, but only at 1 kHz in order to reduce measurement time. However, the device manufacturers would still have to improve the speed of the evaluation algorithms in order to allow to use ASSR as a quasi online method for monitoring residual hearing during slow electrode insertion in cochlear implantation.
Data availability statement
The raw data supporting the conclusions of this article will be made available by the authors, without undue reservation.
Ethics statement
The studies involving humans were approved by Ethikkommission der Medizinischen Hochschule Hannover, Carl-Neuberg-Straße 1, 30625 Hannover. The studies were conducted in accordance with the local legislation and institutional requirements. The ethics committee/institutional review board waived the requirement of written informed consent for participation from the participants or the participants' legal guardians/next of kin because this study is a retrospective analysis of data collected as part of clinical routine.
Author contributions
SH: Conceptualization, Data curation, Investigation, Methodology, Writing – original draft. AB: Conceptualization, Methodology, Supervision, Writing – review & editing. TL: Conceptualization, Methodology, Supervision, Writing – review & editing. RS: Conceptualization, Methodology, Supervision, Writing – review & editing.
Funding
The author(s) declare that financial support was received for the research and/or publication of this article. This work was funded by the Deutsche Forschungsgemeinschaft (DFG, German Research Foundation) under Germany's Excellence Strategy – EXC 2177/1 - Project ID 390895286. This manuscript only reflects the authors' views and funding agencies are not liable for any use that may be made of the information contained herein.
Acknowledgments
We would like to thank our surgeons, our audiometrists, and our staff from the operation theater for their great support.
Conflict of interest
The authors declare that the research was conducted in the absence of any commercial or financial relationships that could be construed as a potential conflict of interest.
Generative AI statement
The author(s) declare that no Gen AI was used in the creation of this manuscript.
Publisher's note
All claims expressed in this article are solely those of the authors and do not necessarily represent those of their affiliated organizations, or those of the publisher, the editors and the reviewers. Any product that may be evaluated in this article, or claim that may be made by its manufacturer, is not guaranteed or endorsed by the publisher.
References
Abbas, P. J., Tejani, V. D., Scheperle, R. A., and Brown, C. J. (2017). Using neural response telemetry to monitor physiological responses to acoustic stimulation in hybrid cochlear implant users. Ear Hear. 38:409. doi: 10.1097/AUD.0000000000000400
Adunka, O., Kiefer, J., Unkelbach, M. H., Lehnert, T., and Gstoettner, W. (2004). Development and evaluation of an improved cochlear implant electrode design for electric acoustic stimulation. Laryngoscope 114, 1237–1241. doi: 10.1097/00005537-200407000-00018
Adunka, O. F., Giardina, C. K., Formeister, E. J., Choudhury, B., Buchman, C. A., and Fitzpatrick, D. C. (2016). Round window electrocochleography before and after cochlear implant electrode insertion. Laryngoscope 126, 1193–1200. doi: 10.1002/lary.25602
Alaerts, J., Luts, H., Hofmann, M., and Wouters, J. (2009). Cortical auditory steady-state responses to low modulation rates. Int. J. Audiol. 48, 582–593. doi: 10.1080/14992020902894558
Barnes, J. H., Yin, L. X., Saoji, A. A., and Carlson, M. L. (2021). Electrocochleography in cochlear implantation: development, applications, and future directions. World J. Otorhinolaryngol. Head Neck Surg. 7, 94–100. doi: 10.1016/j.wjorl.2020.04.006
Baumgartner, W.-D., Jappel, A., Morera, C., Gstöttner, W., Müller, J., Kiefer, J., et al. (2007). Outcomes in adults implanted with the FLEX soft electrode. Acta Oto-laryngol. 127, 579–586. doi: 10.1080/00016480600987784
Büchner, A., Illg, A., Majdani, O., and Lenarz, T. (2017). Investigation of the effect of cochlear implant electrode length on speech comprehension in quiet and noise compared with the results with users of electro-acoustic-stimulation, a retrospective analysis. PLoS ONE 12:e0174900. doi: 10.1371/journal.pone.0174900
Buechner, A., Bardt, M., Haumann, S., Geissler, G., Salcher, R., and Lenarz, T. (2022). Clinical experiences with intraoperative electrocochleography in cochlear implant recipients and its potential to reduce insertion trauma and improve postoperative hearing preservation. PLoS ONE 17:e0266077. doi: 10.1371/journal.pone.0266077
Cohen, L. T., Rickards, F. W., and Clark, G. M. (1991). A comparison of steady-state evoked potentials to modulated tones in awake and sleeping humans. J. Acoust. Soc. Am. 90, 2467–2479. doi: 10.1121/1.402050
Dalbert, A., Huber, A., Veraguth, D., Roosli, C., and Pfiffner, F. (2016). Assessment of cochlear trauma during cochlear implantation using electrocochleography and cone beam computed tomography. Otol. Neurotol. 37, 446–453. doi: 10.1097/MAO.0000000000000998
Dalbert, A., Pfiffner, F., Hoesli, M., Koka, K., Veraguth, D., Roosli, C., et al. (2018). Assessment of cochlear function during cochlear implantation by extra-and intracochlear electrocochleography. Front. Neurosci. 12:18. doi: 10.3389/fnins.2018.00018
D'haenens, W., Vinck, B. M., De Vel, E., Maes, L., Bockstael, A., Keppler, H., et al. (2008). Auditory steady-state responses in normal hearing adults: a test-retest reliability study. Int. J. Audiol. 47, 489–498. doi: 10.1080/14992020802116136
Dhanasingh, A., and Hochmair, I. (2021). Special electrodes for demanding cochlear conditions. Acta Oto-laryngol. 141, 157–177. doi: 10.1080/00016489.2021.1888506
Don, M., and Elberling, C. (1996). Use of quantitative measures of auditory brain-stem response peak amplitude and residual background noise in the decision to stop averaging. J. Acoust. Soc. Am. 99, 491–499. doi: 10.1121/1.414560
Eggermont, J. J. (1974). Basic principles for electrocochleography. Acta Oto-laryngol. 77, 7–16. doi: 10.1080/16512251.1974.11675742
Ehrmann-Müller, D., Shehata-Dieler, W., Alzoubi, A., Hagen, R., and Cebulla, M. (2021). Using ASSR with narrow-band chirps to evaluate hearing in children and adults. Euro. Arch. Oto-Rhino-Laryngol. 278, 49–56. doi: 10.1007/s00405-020-06053-0
Elberling, C., Don, M., Cebulla, M., and Stürzebecher, E. (2007). Auditory steady-state responses to chirp stimuli based on cochlear traveling wave delay. J. Acoust. Soc. Am. 122, 2772–2785. doi: 10.1121/1.2783985
Fitzpatrick, D. C., Campbell, A., Choudhury, B., Dillon, M., Forgues, M., Buchman, C. A., et al. (2014). Round window electrocochleography just prior to cochlear implantation: relationship to word recognition outcomes in adults. Otol. Neurotol. 35:64. doi: 10.1097/MAO.0000000000000219
Forgues, M., Koehn, H. A., Dunnon, A. K., Pulver, S. H., Buchman, C. A., Adunka, O. F., et al. (2014). Distinguishing hair cell from neural potentials recorded at the round window. J. Neurophysiol. 111, 580–593. doi: 10.1152/jn.00446.2013
Galambos, R., Makeig, S., and Talmachoff, P. J. (1981). A 40-Hz auditory potential recorded from the human scalp. Proc. Natl. Acad. Sci. U.S.A. 78, 2643–2647. doi: 10.1073/pnas.78.4.2643
Gantz, B. J., Turner, C., Gfeller, K. E., and Lowder, M. W. (2005). Preservation of hearing in cochlear implant surgery: advantages of combined electrical and acoustical speech processing. Laryngoscope 115, 796–802. doi: 10.1097/01.MLG.0000157695.07536.D2
Giordano, P., Hatzopoulos, S., Giarbini, N., Prosser, S., Petruccelli, J., Simoni, E., et al. (2014). A soft-surgery approach to minimize hearing damage caused by the insertion of a cochlear implant electrode: a guinea pig animal model. Otol. Neurotol. 35, 1440–1445. doi: 10.1097/MAO.0000000000000440
Hatzopoulos, S., Prosser, S., Ciorba, A., Giarbini, N., and Martini, A. (2010). Threshold estimation in adult normal-and impaired–hearing subjects using auditory steady-state responses. Med. Sci. Monit. 16:CR21-27.
Haumann, S., Imsiecke, M., Bauernfeind, G., Büchner, A., Helmstaedter, V., Lenarz, T., et al. (2019). Monitoring of the inner ear function during and after cochlear implant insertion using electrocochleography. Trends Hear. 23, 1–18. doi: 10.1177/2331216519833567
Haumann, S., Mynarek, M., Maier, H., Helmstaedter, V., Büchner, A., Lenarz, T., et al. (2024a). Does intraoperative extracochlear electrocochleography correlate with postoperative audiometric hearing thresholds in cochlea implant surgery – a retrospective analysis on cochlear monitoring. Trends Hear. 28, 1–22. doi: 10.1177/23312165241252240
Haumann, S., Timm, M. E., Büchner, A., Lenarz, T., and Salcher, R. B. (2024b). Intracochlear recording of electrocochleography during and after cochlear implant insertion dependent on the location in the cochlea. Trends Hear. 28, 1–16. doi: 10.1177/23312165241248973
Haumann, S., Timm, M. E., Büchner, A., Lenarz, T., and Salcher, R. B. (2025). Does the audiogram shape influence the intracochlear recording of electrocochleography during and after cochlear implantation? Front. Neurosci. 18, 1–13. doi: 10.3389/fnins.2024.1530216
Helbig, S., Van de Heyning, P., Kiefer, J., Baumann, U., Kleine-Punte, A., Brockmeier, H., et al. (2011). Combined electric acoustic stimulation with the PULSARCI100 implant system using the FLEXEAS electrode array. Acta Oto-laryngol. 131, 585–595. doi: 10.3109/00016489.2010.544327
Herdman, A. T., Lins, O., Van Roon, P., Stapells, D. R., Scherg, M., and Picton, T. W. (2002). Intracerebral sources of human auditory steady-state responses. Brain Topogr. 15, 69–86. doi: 10.1023/A:1021470822922
Hunter, J. B., Gifford, R. H., Wanna, G. B., Labadie, R. F., Bennett, M. L., Haynes, D. S., et al. (2016). Hearing preservation outcomes with a mid-scala electrode in cochlear implantation. Otol. Neurotol. 37:235. doi: 10.1097/MAO.0000000000000963
Incerti, P. V., Ching, T. Y., and Cowan, R. (2013). A systematic review of electric-acoustic stimulation: device fitting ranges, outcomes, and clinical fitting practices. Trends Amplif. 17, 3–26. doi: 10.1177/1084713813480857
James, C., Albegger, K., Battmer, R., Burdo, S., Deggouj, N., Deguine, O., et al. (2005). Preservation of residual hearing with cochlear implantation: how and why. Acta Oto-laryngol. 125, 481–491. doi: 10.1080/00016480510026197
Jurawitz, M.-C., Büchner, A., Harpel, T., Schüssler, M., Majdani, O., Lesinski-Schiedat, A., et al. (2014). Hearing preservation outcomes with different cochlear implant electrodes: Nucleus® Hybrid™-L24 and Nucleus Freedom™ CI422. Audiol. Neurotol. 19, 293–309. doi: 10.1159/000360601
Khater, A., and El-Anwar, M. W. (2017). Methods of hearing preservation during cochlear implantation. Int. Arch. Otorhinol. 21, 297–301. doi: 10.1055/s-0036-1585094
Kim, J.-S. (2020). Electrocochleography in cochlear implant users with residual acoustic hearing: a systematic review. Int. J. Environ. Res. Public Health 17:7043. doi: 10.3390/ijerph17197043
Lehnhardt, E. (1993). Intracochlear placement of cochlear implant electrodes in soft surgery technique. Hno 41, 356–359.
Lenarz, T., Büchner, A., and Illg, A. (2022). Cochlear implantation: concept, results outcomes and quality of life. Laryngo-Rhino-Otologie 101, S36–S78. doi: 10.1055/a-1731-9321
Lenarz, T., Buechner, A., Lesinski-Schiedat, A., Timm, M., and Salcher, R. (2020). Hearing preservation with a new atraumatic lateral wall electrode. Otol. Neurotol. 41, e993–e1003. doi: 10.1097/MAO.0000000000002714
Lenarz, T., Stöver, T., Buechner, A., Lesinski-Schiedat, A., Patrick, J., and Pesch, J. (2009). Hearing conservation surgery using the Hybrid-L electrode. Audiol. Neurotol. 14, 22–31. doi: 10.1159/000206492
Lenarz, T., Timm, M. E., Salcher, R., and Büchner, A. (2019). Individual hearing preservation cochlear implantation using the concept of partial insertion. Otol. Neurotol. 40, e326–e335. doi: 10.1097/MAO.0000000000002127
Liebler, S., Hoth, S., and Plinkert, P. K. (2008). Stationäre evozierte potenziale des auditorischen systems. HNO 10, 1025–1039. doi: 10.1007/s00106-008-1694-1
Luts, H., Desloovere, C., and Wouters, J. (2006). Clinical application of dichotic multiple-stimulus auditory steady-state responses in high-risk newborns and young children. Audiol. Neurotol. 11, 24–37. doi: 10.1159/000088852
Mühler, R. (2004). Auditory steady-state response: an der Schwelle zur klinischen Nutzung? HNO 52, 779–782. doi: 10.1007/s00106-004-1140-y
Mühler, R., Mentzel, K., and Verhey, J. (2012). Fast hearing-threshold estimation using multiple auditory steady-state responses with narrow-band chirps and adaptive stimulus patterns. Sci. World J. 2012, 1–7. doi: 10.1100/2012/192178
Mühler, R., and Rahne, T. (2009). Audiometric thresholds estimated by auditory steady-state responses: influence of EEG amplitude and test duration on accuracy. HNO 57, 44–50. doi: 10.1007/s00106-008-1849-0
O'Leary, S., Mylanus, E., Venail, F., Lenarz, T., Birman, C., Di Lella, F., et al. (2023). Monitoring cochlear health with intracochlear electrocochleography during cochlear implantation: findings from an international clinical investigation. Ear Hear. 44, 358–370. doi: 10.1097/AUD.0000000000001288
Pethe, J., Specht, H. v., Mühler, R., and Hocke, T. (2001). Amplitude modulation following responses in awake and sleeping humans-a comparison for 40 Hz and 80 Hz modulation frequency. Scand. Audiol. 30, 152–155. doi: 10.1080/010503901300007371
Picton, T. W., Dimitrijevic, A., Perez-Abalo, M.-C., and Van Roon, P. (2005). Estimating audiometric thresholds using auditory steady-state responses. J. Am. Acad. Audiol. 16, 140–156. doi: 10.3766/jaaa.16.3.3
Picton, T. W., John, M. S., Dimitrijevic, A., and Purcell, D. (2003). Human auditory steady-state responses. Int. J. Audiol. 42, 177–219. doi: 10.3109/14992020309101316
Radeloff, A., Shehata-Dieler, W., Scherzed, A., Rak, K., Harnisch, W., Hagen, R., et al. (2012). Intraoperative monitoring using cochlear microphonics in cochlear implant patients with residual hearing. Otol. Neurotol. 33, 348–354. doi: 10.1097/MAO.0b013e318248ea86
Risi, F. (2018). Considerations and rationale for cochlear implant electrode design-past, present and future. J. Int. Adv. Otol. 14:382. doi: 10.5152/iao.2018.6372
Roland, Jr., J. T., Gantz, B. J., Waltzman, S. B., and Parkinson, A. J. (2018). Long-term outcomes of cochlear implantation in patients with high-frequency hearing loss. Laryngoscope 128, 1939–1945. doi: 10.1002/lary.27073
Saoji, A. A., Graham, M. K., Adkins, W. J., Nassiri, A. M., Neff, B. A., Carlson, M. L., et al. (2022). Relationship between intraoperative electrocochleography responses and immediate postoperative bone conduction thresholds in cochlear implantation. Otol. Neurotol. 43, e880–e887. doi: 10.1097/MAO.0000000000003620
Schwam, Z. G., Kaul, V. F., Perez, E., Wanna, G. B., and Cosetti, M. K. (2021). Initial experience with a recently developed lateral wall electrode. Laryngoscope 131, 2782–2788. doi: 10.1002/lary.29779
Seidel, D. U., Flemming, T. A., Park, J. J.-H., and Remmert, S. (2015). Hearing threshold estimation by auditory steady-state responses with narrow-band chirps and adaptive stimulus patterns: implementation in clinical routine. Euro. Arch. Oto-Rhino-Laryngol. 272, 51–59. doi: 10.1007/s00405-013-2830-4
Seyyedi, M., and Nadol, Jr., J. B. (2014). Intracochlear inflammatory response to cochlear implant electrodes in the human. Otol. Neurotol. 35:1545. doi: 10.1097/MAO.0000000000000540
Sikka, K., Kairo, A., Singh, C. A., Roy, T. S., Lalwani, S., Kumar, R., et al. (2017). An evaluation of the surgical trauma to intracochlear structures after insertion of cochlear implant electrode arrays: a comparison by round window and antero-inferior cochleostomy techniques. Indian J. Otolaryngol. Head Neck Surg. 69, 375–379. doi: 10.1007/s12070-017-1143-0
Simoni, E., Gentilin, E., Candito, M., Borile, G., Romanato, F., Chicca, M., et al. (2020). Immune response after cochlear implantation. Front. Neurol. 11:341. doi: 10.3389/fneur.2020.00341
Sipari, S., Iso-Mustajärvi, M., Linder, P., and Dietz, A. (2024). Insertion results and hearing outcomes of a slim lateral wall electrode. J. Int. Adv. Otol. 20:1. doi: 10.5152/iao.2024.22962
Snyder, R. L., and Schreiner, C. E. (1984). The auditory neurophonic: basic properties. Hear. Res. 15, 261–280. doi: 10.1016/0378-5955(84)90033-9
Suhling, M.-C., Majdani, O., Salcher, R., Leifholz, M., Büchner, A., Lesinski-Schiedat, A., et al. (2016). The impact of electrode array length on hearing preservation in cochlear implantation. Otol. Neurotol. 37, 1006–1015. doi: 10.1097/MAO.0000000000001110
Tlumak, A. I., Rubinstein, E., and Durrant, J. D. (2007). Meta-analysis of variables that affect accuracy of threshold estimation via measurement of the auditory steady-state response (ASSR). Int. J. Audiol. 46, 692–710. doi: 10.1080/14992020701482480
Tomlin, D., Rance, G., Graydon, K., and Tsialios, I. (2006). A comparison of 40 Hz auditory steady-state response (ASSR) and cortical auditory evoked potential (CAEP) thresholds in awake adult subjects: comparación de umbrales con 40 Hz ASSR y CAEP en adultos despiertos. Int. J. Audiol. 45, 580–588. doi: 10.1080/14992020600895170
Vander Werff, K. R. (2009). Accuracy and time efficiency of two ASSR analysis methods using clinical test protocols. J. Am. Acad. Audiol. 20, 433–452. doi: 10.3766/jaaa.20.7.5
Von Ilberg, C. A., Baumann, U., Kiefer, J., Tillein, J., and Adunka, O. F. (2011). Electric-acoustic stimulation of the auditory system: a review of the first decade. Audiol. Neurotol. 16, 1–30. doi: 10.1159/000327765
Walia, A., Shew, M. A., Lee, D. S., Lefler, S. M., Kallogjeri, D., Wick, C. C., et al. (2022). Promontory electrocochleography recordings to predict speech-perception performance in cochlear implant recipients. Otol. Neurotol. 43, 915–923. doi: 10.1097/MAO.0000000000003628
Keywords: cochlear implants, hearing preservation, Auditory Steady State Responses, monitoring, objective audiometry
Citation: Haumann S, Büchner A, Lenarz T and Salcher RB (2025) Hearing preservation after cochlear implantation evaluated using Auditory Steady State Responses. Front. Audiol. Otol. 3:1560648. doi: 10.3389/fauot.2025.1560648
Received: 13 February 2025; Accepted: 31 March 2025;
Published: 30 April 2025.
Edited by:
Jorge Humberto Ferreira Martins, Polytechnic Institute of Porto, PortugalReviewed by:
Amit Walia, Washington University in St. Louis, United StatesSten Olof Martin Hellström, Karolinska Institutet (KI), Sweden
Copyright © 2025 Haumann, Büchner, Lenarz and Salcher. This is an open-access article distributed under the terms of the Creative Commons Attribution License (CC BY). The use, distribution or reproduction in other forums is permitted, provided the original author(s) and the copyright owner(s) are credited and that the original publication in this journal is cited, in accordance with accepted academic practice. No use, distribution or reproduction is permitted which does not comply with these terms.
*Correspondence: Sabine Haumann, aGF1bWFubi5zYWJpbmVAbWgtaGFubm92ZXIuZGU=