- 1Gastroenterology and Hepatology Unit, Department of Internal Medicine, Al-Azhar University, Cairo, Egypt
- 2Hepatology and Gastroenterology Unit, Department of Internal Medicine, Mansoura University, Mansoura, Egypt
- 3Chemistry Department, Faculty of Science, Helwan University, Cairo, Egypt
- 4School of International Health, Graduate School of Medicine, The University of Tokyo, Tokyo, Japan
- 5Department of Virology II, National Institute of Infectious Diseases, Tokyo, Japan
- 6Department of Internal Medicine, Faculty of Medicine, Al-Azhar University, Assiut, Egypt
- 7Nephrology Unit, Department of Internal Medicine, Al-Azhar University, Cairo, Egypt
- 8Gastroenterology Unit, Department of Internal Medicine, Sultan Qaboos University Hospital, Muscat, Oman
The risk of hepatocellular carcinoma (HCC) diminishes in patients with hepatitis C virus (HCV)-related advanced chronic liver disease after virological cure. However, despite viral clearance, HCV-induced epigenetic alterations, immune dysregulations, and hepatic parenchymal injuries remain, contributing to de novo HCC occurrence. While HCC incidence is low (0.45 – 0.5%) in patients with advanced fibrosis (F3), the presence of liver cirrhosis and clinically significant portal hypertension increases the HCC risk. The cost-effectiveness of lifelong HCC surveillance in patients with compensated advanced chronic liver disease (cACLD) has sparked debate, raising questions about the most reliable noninvasive tests and stratification models for predicting HCC in patients with sustained virological response (SVR). Furthermore, identifying cACLD patients who may not require long-term HCC surveillance after SVR remains crucial. Several HCC risk stratification scores have been suggested for patients with cACLD, and emerging evidence supports individualized care based on personalized risk assessments. This review focuses on revising the pretreatment and posttreatment predictors of HCC, as well as the indications for HCC surveillance in cACLD patients treated with direct-acting antivirals.
1 Introduction
The introduction of direct-acting antivirals (DAAs) has decreased the incidence of hepatocellular carcinoma (HCC) in chronic hepatitis C (CHC) patients (1, 2). Achieving a virological cure after DAAs is associated with 71% reduction in the risk of developing HCC compared to treatment failure, during a mean 6.1 years of follow up (3). DAAs therapy has also demonstrated improved survival outcomes in hepatitis C virus (HCV)-related HCC patients by reducing disease progression and delaying hepatic decompensation (4).
However, the risk of HCC remains even after HCV clearance. Particularly in patients with liver cirrhosis, HCC remains the most common liver-related complication following sustained virological response (SVR) (5). Early studies conducted following DAA introduction have reported an increased risk of HCC after SVR (6, 7). The early recurrence rate of liver cancer after DAA has been ranged from 12.7%–28.8% (7, 8). A meta-analysis revealed an HCC recurrence rate of 20% person-years (PY) following DAA treatment (9). Furthermore, a three-year incidence of de novo HCC occurrence after SVR was found to be 5.9% (10). Notably, HCC that arises after DAA-induced viral clearance may exhibit aggressive behavior and may limit the patient’s eligibility for curative treatment options (11–13).
Eradicating HCV and achieving SVR serve as a primary means to prevent HCC and reduce HCC-related mortality. D’Ambrosio et al. demonstrated that HCC is a frequent liver event in cirrhotic patients after DAA treatment, regardless of liver dysfunction or previous liver complications (5, 14). Moreover, patients with a history of HCC who experienced HCC recurrence after SVR had higher mortality rates than those without recurrence (5, 14). Recent studies have revealed that liver cancer accounts for 51.9%–90% of liver-related deaths in DAA-treated patients after SVR (5, 15, 16). These findings collectively highlight the significant impact of HCC occurrence on patient outcomes and underscore the importance of HCC surveillance despite achieving viral clearance after DAAs treatment.
Advanced liver fibrosis is widely accepted as a main underlying mechanism of HCC after SVR. However, the occurrence of HCC in noncirrhotic livers and its aggressive characteristics following DAA treatment have created a disparity in expectations regarding the underlying oncogenic mechanisms (12, 17). HCV-induced HCC involves both direct and indirect mechanisms, including the activation of hepatic fibrogenic pathways, cellular pathways and survival pathways, as well as interactions with the immune and metabolic pathways. Genomic studies have identified polymorphisms in different signaling systems associated with an increased HCC risk (18–20). Furthermore, HCV eradication may trigger a transient immunosuppression that potentially creates favorable situation for the growth of latent micronodules (21). Therefore, gaining more data about the impact of virological cure on HCV-induced oncogenic mechanisms will aid in identifying the persistent epigenetic modifications and inflammatory responses responsible for HCC after SVR, ultimately facilitating the prediction of individual susceptibility to HCC. This, in turn, will guide HCC risk stratification and enable personalized HCC screening for patients after achieving SVR.
Advanced chronic liver disease (ACLD) patients have a significantly increased incidence of HCC (1, 2, 22). The Baveno VI consensus conference in 2015 introduced the concept of compensated ACLD (cACLD), removing the borderline between cirrhosis and noncirrhotic status. This term encompasses both advanced liver fibrosis (F3) and compensated cirrhosis (F4) patients (23, 24). Prior to treatment, assessing liver stiffness (25–29) and utilizing noninvasive blood tests for liver fibrosis are valuable tools for excluding and diagnosing cACLD at baseline (30). In addition, monitoring the dynamic changes in TE and noninvasive blood tests over time appears to enhance the prognostic capability beyond single measurements in assessing cACLD after achieving SVR. Notably, evaluating these noninvasive tools may aid physicians in accurately predicting the HCC risk (low/high) in patients with cACLD following a virological cure with DAA.
This review focuses on a comprehensive analysis of the literature on predictors of de novo HCC occurrence and the indications for HCC surveillance in cACLD patients after achieving a DAA-mediated virological cure.
2 Incidence of de novo HCC after SVR
The use of pegylated interferon (IFN) plus ribavirin resulted in a significant reduction in HCV-related complications among responders versus nonresponders. Despite this reduction, HCC still occur after SVR (31). Advanced liver fibrosis (≥F3) was found to have a higher risk of de novo HCC than earlier stages of fibrosis, regardless of the response to therapy. Morgan et al.’s meta-analysis revealed that 17.8% of HCV patients with cACLD (F3–F4) and no SVR developed HCC, with an incidence rate of 3.3% PY. However, among HCV patients with cACLD (F3–F4) and SVR after IFN-therapy, 4.2% developed HCC, with an incidence rate of 1.05% PY (32).
According to a large retrospective study, the HCC incidence in cirrhotic patients after virological cure was lower in patients treated with DAA (2.12% PY) and IFN (2.28% PY) than in untreated patients (4.53% PY) (33).
Recently, a total HCC incidence of 1.46% PY was reported in DAA-treated patients after SVR (34). The HCC incidence was 2.31% PY and 0.45% PY in cirrhotic patients and in patients with advanced fibrosis (F3), respectively. Similar HCC incidences of 2.10% PY among patients with cirrhosis and 0.50% PY among patients with F3 fibrosis, were reported in a large metanalysis (35).
3 Persistence of HCV-induced oncogenic changes after SVR
HCV-induced epigenetic alterations (36), immune system dysregulations, metabolic disorders, host genetic mutations, and hepatic parenchymal injuries (Figure 1) may persist after DAA treatment and correlate with HCC occurrence after SVR.
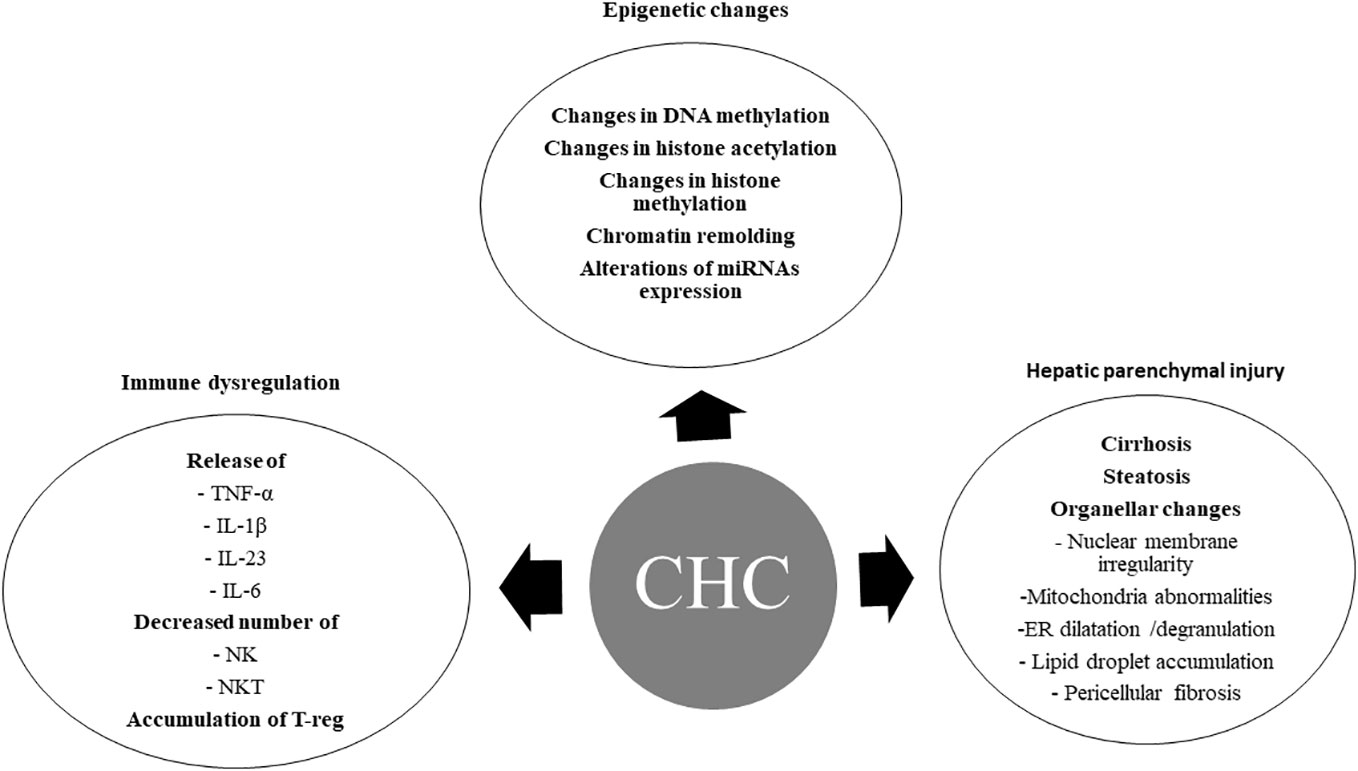
Figure 1 Chronic hepatitis C-induced oncogenic changes. CHC, chronic hepatitis C; DNA, Deoxyribonucleic acid; miRNAs, micro ribonucleic acids; TNF-α, tumor necrosis factor -alpha; IL-1β, interleukin-1 beta; IL-23, interleukin – 23; IL-6, interleukin -6; NK, natural killer cells; NKT, natural killer T cells; T-reg, regulatory T cells; ER, endoplasmic reticulum.
3.1 Persistence of HCV-induced epigenetic changes
Hepatitis C-infected cells and HCV-induced inflammatory and/or fibrotic responses in the liver microenvironment can produce hepatic epigenetic changes. Increasing evidences indicated that CHC infection induces changes in DNA methylation, histone modification and chromatin remodeling, and miRNAs expression. These epigenetic changes may play a pivotal role in hepatocarcinogenesis (37) (Figure 1).
In vitro and in vivo studies have demonstrated the oncogenic behavior of HCV proteins (NS3, NS5A, and the core protein) (38–41). Indeed, DNA methyltransferase 1 (DNMT1) and DNA methyltransferase 3B (DNMT3B) levels were significantly increased in HCV core protein expressing HepG2 cells and in Huh-7 cells (42–45). Furthermore, the correlation between CHC infection and aberrant methylation of multiple genes has been established in HCV-positive HCC (42, 44, 46–49). Impaired expression of these genes contributes to hepatocarcinogenesis by promoting cell proliferation, invasion, and immune evasion (42, 44, 46–49).
Chronic hepatitis C infection is associated with changes in histone acetylation (50) and histone methylation (51, 52). In vivo CHC infection induced specific changes in H3K27ac, which correlated with the expression of HCV messenger ribonucleic acids and HCV proteins (36). The HCV core protein increased the levels of DNMT1 and histone deacetylases 1 (HDAC1) and stimulated their binding to the secreted frizzled related protein 1 (SFRP1) promoter. Silencing of SRFP1 led to activation of the Wnt/β-catenin signaling and may contribute to hepatocarcinogenesis (50). Similarly, in vitro CHC infection induces significant enrichment of the transcriptional active chromatin markers H3K9ac and H3K4me, and of the transcriptional silent chromatin marker H3K9me3, changes that may be associated with hepatocarcinogenesis (53).
Chronic hepatitis C infection induces changes in cellular miRNAs expression (54–57). The HCV core protein can stimulate tumor migration and invasion by DNMT1/methylation-mediated inhibition of miR-124 expression, and thus preventing miR-124-induced silencing of set and mynd domain containing 3 (SMYD3) gene (56). Moreover, HCV core protein can affect H3K27me3 through a miR-124/enhancer of zeste 2 polycomb repressive complex 2 subunit (EZH2) pathway (58).
While in vitro results showed the reversion of epigenetic markers levels to a level comparable to uninfected cells after INF-based HCV clearance (53), in vivo experiment support the persistence of epigenetic changes after DAA- and IFN-based HCV clearance (36). The persistence of these epigenetic changes has been implicated in HCC occurrence after a DAA viral clearance (36), (Figure 2). Indeed, HCV infection induced H3K27ac modifications was associated with HCC risk after viral clearance (36). The functional knockout of Sphingosine kinase 1 gene and sex-determining region Y-box-9 (SOX-9) gene can inhibit HCC growth (36). While the expression levels of both genes increased during chronic hepatitis C, they remained unaltered after the DAA viral clearance (36). Notably, dysregulation of Wnt/β-catenin signaling plays a paramount role in HCC development; it can be activated by directly binding to HCV-NS5A protein; and promotes proliferation, angiogenesis, and epithelial–mesenchymal transition (59). Of note, Wnt/β-catenin signaling can be switched on in spite of DAA-induced HCV clearance (60), possibly due to the dual effect of DAA treatment; eradicating HCV while activating Wnt/β-catenin signaling (60). The exact mechanism of this phenomenon remains unidentified (60). In cirrhosis, exosomes are crucial modifiers of the tumor microenvironment, and miR-122 downregulation is related with HCC occurrence. In fact, liver-specific miR-122 levels exhibited a significant drop after DAA therapy (61) and remained low among SVR achieved patients (62). In cirrhotic tissue, Angiopoietin-2 (ANGPT2) expression before DAA treatment significantly relates to circulating vascular endothelial growth factor (VEGF) and independently relates to HCC occurrence after DAA. Notably, VEGF and ANGPT2 increased following DAA treatment and up to 3-month follow-up (63, 64). Interestingly, the epigenetic signature induced by CHC and persist after DAA treatment is reverted in vitro by the epidermal growth factor receptor (EGFR) inhibitor, Erlotinib (53).
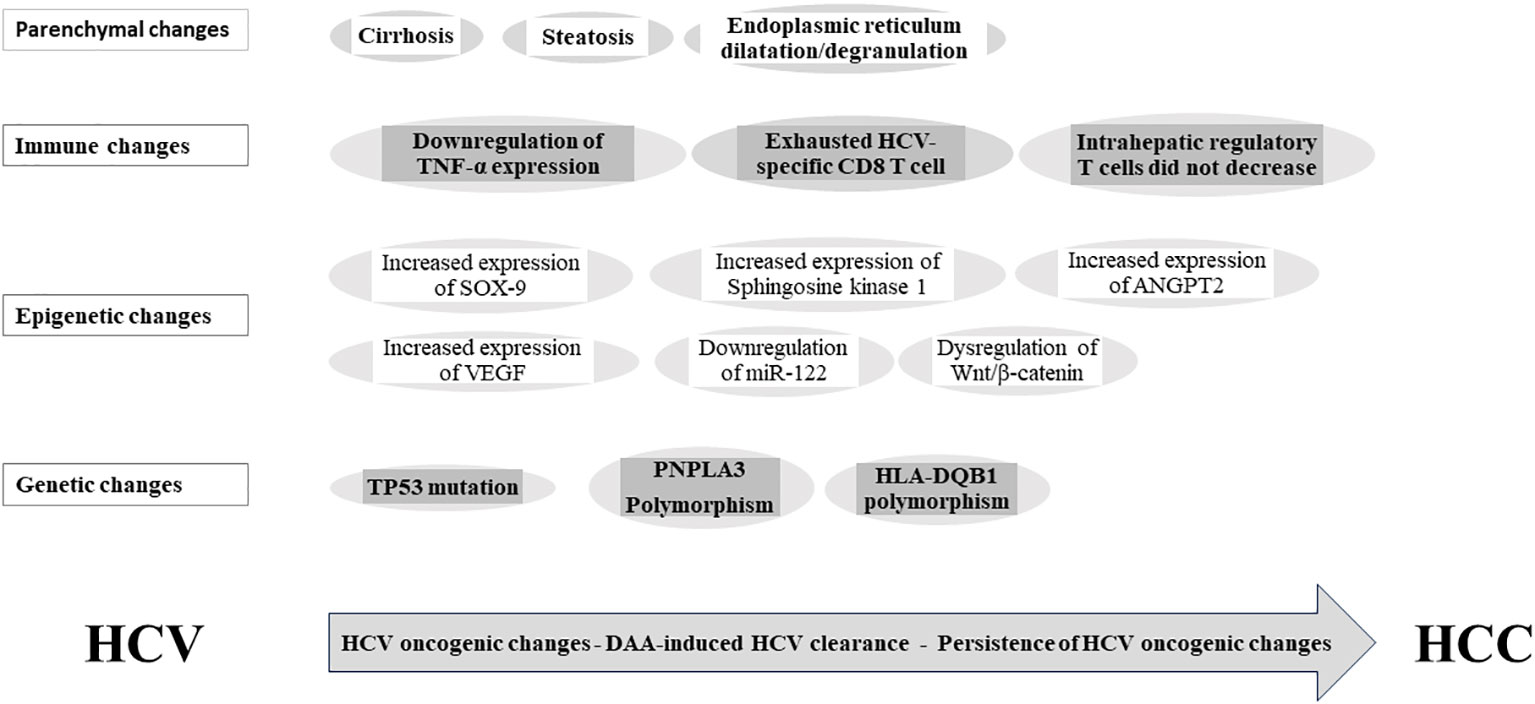
Figure 2 Persistence of hepatitis C-induced oncogenic changes after viral clearance. SOX-9, SYR-box transcriptional factor 9; VEGF, vascular endothelial growth factor; ANGPT2, Angiopoietin-2; miR-122, miRNA-122; TP53, tumor protein P53; PNPLA3, Patatin-like phospholipase domain-containing protein 3; HLA-DQB1, Major histocompatibility complex, class II, DQ beta1; HCV, hepatitis C virus; DAA, direct-acting antiviral; HCC, hepatocellular carcinoma.
3.2 Persistence of immune dysregulation
Inflammation is a major constituent of HCV-induced HCC. The inflammatory cells predispose to HCC occurrence by reactive oxygen species (ROS) and reactive nitrogen species production, and lipid peroxidation. Numerous inflammatory cytokines secreted during chronic HCV infection, such as tumor necrosis factor-alpha (TNF-α), interleukin-1β (IL-1β), interleukin-23 (IL-23), and interleukin-6 (IL-6), are associated with HCC occurrence (65–67). The decreased members of innate immunity, particularly natural killer and natural killer T (NKT) cells, and the increased number of regulatory T (T-reg) cells may correlate with HCC occurrence in chronic HCV infection (68), (Figure 1).
The downregulation of IFN-stimulated genes was rapid after a DAA-induced virological cure (69). The incomplete restoration of innate immune surveillance following DAA-induced HCV eradication has been related to the rapid decrease or normalization of immunosurveillance, potentially leading to early HCC development post-DAA treatment (21, 70). Notably, serum TNF-α expression was persistently downregulated and significantly related to the HCC risk after SVR (71). Moreover, the exhausted phenotype of the HCV-specific CD8 T cell response is not restored by DAA-induced SVR (72–74). Similarly, the intrahepatic regulatory CD4 T cells and CD4(+) CD25(+) FoxP3(+) T-reg cells did not decrease after DAA-induced virological cure (75, 76). This failure of immune function restoration after DAA-induced virological cure may be implicated in HCC occurrence after achieving SVR (77), (Figure 2).
3.3 Persistence of liver injury
The persistence of advanced liver fibrosis and liver injury after DAA treatment are associated with HCC development (78), (Figure 1). Emerging data regarding cirrhosis regression after DAA-induced HCV cure are primarily derived from transient elastography (TE)-based liver stiffness measurement (LSM) (79–84). However, true liver fibrosis regression cannot be solely equated with regressed liver stiffness (85). The hepatic venous pressure gradient (HVPG) stands as the most accurate predictor for liver-related outcomes in cACLD patients (86). Indeed, the disappearance of clinically significant portal hypertension (CSPH) is a rare event after SVR. TE measurements after SVR do not correlate with the HVPG measurements (87, 88), suggesting that viral clearance does not indicate regression of liver cirrhosis (85) and indicating a persistent risk of clinical progression and occurrence of liver-related events after SVR. Liver fibrosis progression after SVR may be triggered by obesity, diabetes mellitus, and alcohol consumption (89). Recently, it was suggested that the persistence of abnormal endoplasmic reticulum after a virological cure is associated with de novo HCC occurrence (90). Collectively, these data proposed that overlapping liver injury associated with viral and nonviral causes may lead to the persistence of HCC risk after SVR (Figure 2).
4 Pretreatment predictors of de novo HCC after SVR
Pretreatment risk stratification for HCC is useful for identifying patients who require long-term surveillance following DAA treatment (Table 1). The prognostic importance of baseline demographic characteristics and noninvasive tests in predicting HCC risk after DAA treatment has been reported (91).
4.1 Host-related risk factors
4.1.1 Age and male sex
Age has been recognized as an independent risk factor (92) and a valuable marker for de novo HCC following SVR (93). The rates of HCC occurrence in patients aged ≥73 and <73 years were 2.2% and 0.5%, 3.9% and 0.7%, 6.1% and 2.1%, and 7.6% and 3.3% at one, two, three, and four years, respectively. Notably, patients aged 75–84 years carried a higher risk of HCC even after DAA-induced virological cure, irrespective of the existence of baseline cirrhosis (94). Early development of HCC after SVR is independently associated with male sex (95), as evidenced by a higher 3-year estimated cumulative HCC occurrence rate in males (9%) than in females (2%) (96).
4.1.2 Continuous alcohol intake
Alcohol can increase HCC risk via the formation of active carcinogen molecules from pro-carcinogens (97). Kanwal et al. (1) recorded a higher annual occurrence rate of HCC in alcohol consumed-patients (1.01% PY) compared to those without alcohol use (0.72% PY) after achieving SVR post-DAA treatment (1). A recent multicenter retrospective study (98) linked baseline alcohol intake to an elevated risk of HCC development after a virological cure.
4.1.3 Diabetes mellitus
Degasperi et al. (96) recognized diabetes mellitus (DM) as a potent independent risk factor for de novo HCC. Notably, early HCC occurrence was independently related to the existence of DM (95). Moreover, the 3-year estimated cumulative rate of HCC occurrence after DAA treatment was 16% in DM patients compared to 4% in patients without DM (96).
4.1.4 Host genetics
Whole-exome sequencing was performed on HCC specimens that developed in HCV patients after treatment. The analysis detected higher incidence of TP53 mutations in HCV-SVR-DAA tumors than in HCV-SVR-IFN tumors (99). Host genetic variations can contribute to HCC development after HCV eradication. Recent studies have identified PNPLA3 and HLA-DQB1 polymorphisms as independent risk factors for HCC occurrence following SVR (100) (Figure 2). These findings hold the potential for refining and customizing HCC risk stratification models and surveillance guidelines after SVR.
4.2 Virus-related risk factors
4.2.1 HCV genotype
HCV genotype 3 patients exhibit a more progressive liver disease than those with other genotypes (101). An elevated occurrence of de novo HCC has been identified in HCV genotype 3 patients after DAA treatment, particularly among those with pre-existing liver cirrhosis (102).
4.2.2 HCV/HIV coinfection
HCV-infected patients with advanced liver fibrosis, coinfected with human immunodeficiency virus (HIV), appears to have a lower risk of HCC after SVR. The occurrence rates of HCC were recorded as 0.3% PY (103) and 1.2% PY (104) after median follow-up periods of 31.6 and 43 months, respectively. The specific underlying causes that result in the reduced HCC risk among HCV/HIV-coinfected patients remain unknown (104).
4.3 Liver-related risk factors
4.3.1 Hepatic steatosis
In CHC patients, liver steatosis is independently associated with the progression to advanced fibrosis, and both liver steatosis and advanced fibrosis are significant risk factors for HCC in CHC (105). Liver steatosis exhibit higher occurrence rate of HCC (5.23% PY) in comparison with advanced fibrosis (3.51% PY). When categorized based on their baseline fibrosis and steatosis status, patients with liver steatosis without advanced fibrosis have higher rates of HCC incidence compared to those with advanced fibrosis but without steatosis (106).
4.3.2 Noncharacterized hepatic nodule
DAA treatment has been associated with an early elevated HCC incidence in patients with noncharacterized hepatic nodules (107). The presence of noncharacterized hepatic nodules before DAA treatment is accompanied by an approximate 30% cumulative risk of de novo HCC within the first year following treatment (34). Similarly, the presence of noncharacterized hepatic nodules prior to DAA treatment is accompanied by a threefold increased risk of HCC (98). Notably, in patients with Child-Pugh class A, the incidence of HCC in those with noncharacterized hepatic nodules before DAA therapy was 7.24% PY compared to 2.77% PY in those without noncharacterized nodules (98). These noncharacterized hepatic nodules are presumed to represent low/high-grade dysplastic nodules, macro regenerative nodules (98), or microscopically undetectable tumors (108). The temporal relation between initiating DAA therapy and HCC development, along with the existence of noncharacterized hepatic nodules at baseline, indicates that antiviral treatment may trigger a mechanism that promotes the growth and clinical recognition of HCC shortly after treatment (98). This association emphasizes the importance of rigorous surveillance when noncharacterized hepatic nodules are present prior to initiating DAA therapy (10).
4.4 Noninvasive tests
Various baseline noninvasive tests are available for anticipating HCC in cACLD patients after a DAA-induced virological cure.
4.4.1 Alpha-fetoprotein
An elevated pretreatment alpha-fetoprotein (AFP) level before DAA therapy strongly correlates with hepatocarcinogenesis (109). This elevated AFP level may indicate liver regeneration, ongoing inflammation, liver fibrosis, or microscopic HCC. The significant decrease in AFP levels from pretreatment to the end of treatment (EOT), along with the normalization of liver enzymes and viral suppression, suggests that the elevated pretreatment AFP level may reflect the degree of liver inflammation (110). However, a nonreduction in the elevated pretreatment AFP level during treatment is independently related to HCC occurrence (110). In addition, if this level remains elevated after achieving a virological cure, it may suggest advanced liver fibrosis or microscopic HCC.
4.4.2 Mac-2 binding protein (M2BP) glycosylation isomer (M2BPGi)
Liver fibrosis is a major risk factor for developing hepatic carcinogenesis. The M2BPGi level is highly associated with the liver fibrosis stage (111–116). Yamasaki et al. found that the average serum levels of M2BPGi were 1.3, 2.2, 3.3, and 5.2 in patients with fibrosis stages of F0–F1, F2, F3, and F4, respectively, as determined by liver histopathology (117). M2BPGi cutoff values of less than 1.51, 2.48, and 3.50 can exclude the existence of ≥F2, ≥F3, and F4, with negative predictive values of 95.9%, 95.1%, and 99.6%, respectively. However, M2BPGi cutoff values of more than 2.08, 2.87, and 4.35 can propose the existence of ≥F2, ≥F3, and F4, with positive predictive values of 94.2%, 83.1%, and 85.3%, respectively. Several studies have reported that an elevated serum M2BPGi levels more than 1.70–2.00 is associated with increased risk of HCC occurrence or recurrence (118–124). Indeed, the risk of HCC steadily increasing when the serum M2BPGi levels are ranked as <1.00, 1.00–4.00, and ≥4.00 (117).
4.4.3 FIB-4 index
A baseline FIB-4 index ≥3.25 is strongly related to HCC risk (1, 125). The persistent elevation of FIB-4 ≥3.25 before treatment is linked to an increased HCC risk (5.07% PY) in HCV-related ACLD following DAA treatment. Conversely, a persistently lower FIB-4 level (<3.25) before treatment is linked to the lowest HCC risk (1.0% PY) (126). Notably, changes in the FIB-4 score before treatment and after achieving SVR are correlated with modifications in HCC risk. A reduction in FIB-4 from >3.25 to <3.25 after SVR is linked to a decline in HCC risk, while an elevation from <3.25 to ≥3.25 is linked to a raised HCC risk (126).
4.4.4 Liver stiffness
Patients with cACLD are of particular interest, as a significant proportion of them (50%–60%) have CSPH (127). Among the cACLD patients it is crucial to identify those with low HCC risk. LSM has emerged as a useful tool for stratifying cACLD patients who have attained SVR based on their HCC risk (128, 129). Populations with a high pretreatment LSM are at an elevated potential of developing de novo HCC (7, 96). The failure to reduce pretreatment LSM by <30% after treatment is independently linked to HCC development (130). Notably, the five-year occurrence rate of HCC increased with higher pretreatment LSM values (9.7% for LSM ≥15 kPa and 11.4% for LSM ≥20 kPa). Different pretreatment LSM cutoff values (14.3 kPa, 20 kPa (34), and ≥30 kPa (96)) have been suggested as the best predictors of de novo HCC following SVR. Collectively, these varying cutoff values indicate that increased LSM at the bassline implies increased attributed risk. Thus, rigorous inclusion of patients with pretreatment LSM ≥20 kPa in HCC surveillance programs is recommended (34, 131).
4.5 DAA-related risk
It was found that early HCC incidence is linked to sofosbuvir (SOF)-based therapy without ribavirin, whereas ribavirin usage seems to exert an immunomodulating protective effect on HCC occurrence (95, 132). While SOF increased the cell proliferation in OR-6 and Huh 7.5.1 cells, the cell proliferation was not significantly influenced after treatment of OR-6 and Huh 7.5.1 cells with different doses of interferon-α and ribavirin (133). Next generation sequencing (NGS) studies revealed that PHOSPHO2-KLHL23, TSNAX-DISC1, TRIM39, RPP21 were upregulated by SOF in OR-6 cells. Indeed, the SOF-increased cell proliferation is improved after siRNA mediated knockdown of PHOSPHO2-KLHL23, TSNAXDISC1, TRIM39 and RPP21 in OR-6 and Huh 7.5.1 cell cultures. Tsai et al. concluded that SOF-induced gene expression in mouse liver cells increased HCC proliferation and migration, which may be related to HCC occurrence (133). However, Ogawa et al. (134) concluded that DAA–SOF treatment was not linked to HCC occurrence within five years after treatment.
5 Post treatment predictors of de novo HCC after SVR
Post treatment DM, alcohol intake, and hepatic steatosis are linked to elevated HCC risk. The sustained elevation of pretreatment AFP and FIB-4 values, alongside with the failure of liver stiffness regression after virological cure, strongly relates to HCC occurrence. The dynamic changes in noninvasive liver fibrosis markers following SVR have been correlated with HCC risk (91) (Table 1).
DM was found as a risk factor for HCC in DAA-treated populations after achieving SVR (135) and maintained a significant risk factor for HCC following four years of SVR (136). Although the HCC–DM interrelationship is incompletely understood, hyperinsulinemia and oxidative stress are considered the main factors facilitating DM progression to cancer (137). Hyperinsulinemia is directly involved in carcinogenesis, as it promotes cancer initiation and progression, and is indirectly involved via its stimulation of insulin-like growth factor 1 (IGF-1). In patients with diabetes, increased oxidative stress is linked to DNA damage, mutation in oncogenes, and development of cancer (138, 139).
Alcohol’s effects on hepatic fibrogenesis persist post-SVR in alcohol-consuming individuals (1), as evidenced by the increased annual rate of HCC reported among DAA-treated populations who consumed alcohol post-SVR (1). Similarly, while hepatic steatosis is linked to increased HCC risk after DAA (116), liver steatosis is identified as a major predictor of HCC up to two years following SVR, irrespective of fibrosis stage (116).
EOT-AFP (140, 141) and SVR24-AFP levels (142, 143) were identified as predictors of HCC occurrence in DAA treated patients. An increased one-year cumulative HCC occurrence rate correlated with an elevated EOT-AFP level (144). FIB-4 ≥3.25 estimated at SVR or at any time post-SVR was linked to HCC risk, while FIB-4 ≤1.45 was not linked to HCC risk post-SVR in IFN-treated patients (145). Patients treated with DAA whose FIB-4 elevated post-SVR to at least 3.25 had a higher HCC occurrence rate than those who remained persistently below 3.25 (2.29% vs. 1.02%) (93, 126). In addition, patients whose FIB-4 decreased to <1.50 or persisted at <1.50 during follow-up post-SVR had extremely low rates of hepatocarcinogenesis (146). Finally, many studies have confirmed that repeated measurements of FIB-4 is an accurate and cheap method to differentiate patients who may require intensive HCC surveillance after a DAA-induced virological cure (147, 148).
The long-term HCC risk profile in ACLD patients, after DAA-mediated HCV eradication, suggested the reliable predictability of portal hypertension indicators (149). Indeed, posttreatment platelet count ≤ 120,000/µl is independently linked to de novo HCC occurrence, after age adjustment (149).
A reduction in LSM of less than 30% in cACLD patients after SVR is an independent risk factor for HCC (96). The estimated three-year incidence of de novo HCC was 20% and 5% in patients with LSM > 30 kPa and ≤ 30 kPa, respectively.
Collectively, these data indicate that patients with cACLD should be closely monitored after SVR for de novo HCC occurrence in the presence of severe portal hypertension, elevated AFP, and other risk factors for ongoing liver injury (e.g., DM, alcohol drinking, and steatosis).
6 HCC surveillance after SVR
The recent introduction of DAA for treating HCV has significantly influenced the disease outcome course, in particular the occurrence of de novo HCC (1, 150). HCC surveillance primarily aims to early detection of HCC to enable curative management, and increasing overall survival (Table 2). Following a DAA-induced virological cure, society guidelines recommend HCC surveillance in cirrhotic (F4) patients, often with biannual abdominal ultrasonography with or without AFP. However, there has been considerable discussion regarding the cost-effectiveness and potential risks of lifelong HCC surveillance in patients with advanced fibrosis (F3) following SVR.
6.1 Cost-effectiveness of HCC surveillance
Although a higher frequency of ultrasound scans during the two years before HCC diagnosis increases the chance of receiving curative treatment (151), surveillance is deemed cost-effective and advantageous to an individual if it increases life expectancy at a cost of less than $50,000 per additional year (152). Lin et al. assessed the cost-effectiveness of HCC surveillance in cirrhotic patients and found that biannual AFP and annual abdominal ultrasonography were effective, with a cost-effectiveness ratio of less than $50,000 per quality-adjusted life-year (QALY) (153). Moreover, a recent meta-analysis revealed the beneficial effect of HCC surveillance programs for cirrhotic patients, revealing a lower risk of mortality in the continued surveillance versus nonsurveillance arms. Surveillance was also associated with a greater ability to detect early-stage tumors and receive curative treatment (154). In contrast, biannual HCC surveillance after virological cure was not cost-effective for patients with F3 fibrosis (155).
6.2 Potential harms of HCC surveillance
Physicians must consider both the clear advantages and possible drawbacks of HCC surveillance. These may include financial, psychological, and physical costs, as well as the efficiency of biannual abdominal ultrasound. Patients may suffer physical harm due to false positive or inconclusive surveillance results. Financial harms include the price of diagnostic testing and screening, as well as travel expenses and lost workdays. Being constantly informed of one’s chance of contracting fatal cancer and having to wait to learn the outcome every six months can be emotionally exhausting and result in fear, anxiety, and despair (156). The low efficiency of biannual abdominal ultrasound was recently highlighted, as 10,376 abdominal ultrasound scans were performed to allow curative treatment for only 49 patients (151). Taken together, although the benefits of HCC surveillance significantly outweighing the risks (157, 158), additional research is required to accurately quantify the financial and psychological burdens of this surveillance (156).
6.3 Individualization of HCC surveillance
Patients with compensated cirrhosis (F4) and advanced liver fibrosis (F3) were enrolled in the cACLD group. Long-term monitoring is necessary for patients with liver cirrhosis who receive DAA treatment due to their high risk of developing HCC after SVR (159). Notably, patients with cirrhosis were found to benefit financially from biannual HCC surveillance following SVR, whereas those with F3 fibrosis were not (155), demonstrating that more specified stratification models are required to estimate the risk magnitude and benefits of long-term surveillance in the latter.
Numerous HCC risk categorization scores for cACLD patients who obtained SVR after DAA treatment have been reported (160, 161). Liu and colleagues developed a recently simplified risk analysis approach for de novo HCC due to LSM and the FIB-4 index (162). Patients with pretreatment LSM 12 kPa and SVR-FIB-4 3.7 had the lowest likelihood of developing de novo HCC after SVR; those who did not meet one or both of these criteria were classified as intermediate-risk (3.6% PY) or high-risk (5% PY), respectively. The low-risk group’s HCC incidence was below the cost-effectiveness cutoff for HCC surveillance (163). Semmler et al. developed an algorithm for correctly categorizing patients with cACLD based on their risk of de novo HCC after SVR. This algorithm was based on posttreatment age, albumin, LSM, and, optionally, AFP and alcohol use. In both the derivation and validation cohorts, around two-thirds of patients with cACLD were found to have an HCC risk of less than 1% PY, and falling below the cost-effectiveness criterion for HCC surveillance (129).
Although these data open the door for a more individualized surveillance approach for patients with cACLD after a virological cure, no HCC risk score has been demonstrated to be significantly better than others or adequately validated for incorporation into existing standard practice. However, a number of models are currently undergoing late-stage clinical validation and could become widely available soon (164).
6.4 Duration of post-SVR surveillance
As no rule specifies a certain age beyond which surveillance should end, Mueller et al., 2022 conducted a comparative cost-effectiveness study determining the age at which the advantages of HCC surveillance outweigh the costs by modeling the life course of people who have been cured of HCV (165). They came to the conclusion that abdominal ultrasound-based biennial screening for HCC is cost-effective in patients with cirrhosis up to age 70 and in those with severe (F3) fibrosis up to age 60.
6.5 Patients with ACLD who are ineligible for HCC surveillance
HCC surveillance is not advised for people with Child-Pugh class C cirrhosis who are ineligible for transplantation, as HCC surveillance will not offer longer life expectancy with the increased mortality risk from liver failure in those patients. Similarly, evidence suggesting that patients without cirrhosis or advanced fibrosis (F3) deserve a universal surveillance approach is insufficient (166). In addition, those with advanced (F3) fibrosis and extremely low estimated risk for HCC based on the recent stratification algorithms could have a more prolonged interval or even discontinue surveillance, particularly when resources are limited. Ciancio et al. (2023) did a recent study in Italy over a span of 2 years and involving 1000 cACLD patients demonstrated that patients with baseline LSM between 9.5 and 14.5 kPa, FIB-4 below 3.25, and APRI below 1.5, who have achieved SVR posttreatment with DAAs, could be considered eligible for suspension from HCC surveillance programs due to the negligible risk (0.09% PY) for HCC occurrence (148).
6.6 Patient adherence to surveillance
Despite clear advantages, such as early tumor diagnosis, only 20% of cirrhotic patients receive HCC surveillance (148, 151, 167). The underutilization of HCC surveillance can be attributed to some screening process errors, including the failure of the provider to recognize liver disease, identify the silent progression to cirrhosis, the failure to order HCC screening tests, and the failure of the patient to follow screening advice (167).
Based on 2015 survey (167), it was discovered that 65% of the physicians claimed to order annual HCC screening, whereas only 15% reported to request biannual screening. Furthermore, there are significant differences in the rate of HCC screening utilization between individuals followed in gastroenterology clinics (73.7%) and those in population-based cohorts (8.8%) (168). Notably, interventions such public health outreach, reminder and recall systems, and patient-provider education are thought to be beneficial for improving the rates of HCC screening utilization (168).
7 Interventions reducing HCC risk after SVR
7.1 Metformin
Metformin could reduce HCC incidence in patients who achieved a virological cure after INF-based therapy (169). The five-year cumulative HCC incidence was 10.9% in DM-non-metformin users and 2.6% in DM-metformin users, compared to 3.0% in patients without DM. In this study, DM-non-metformin use was significantly associated with HCC risk (169). Interestingly, metformin was found to revert Wnt/β-catenin signaling after DAA-induced virological cure through PKA/GSK-3β-mediated β-catenin degradation (60). These data indicate that metformin may stop the growth of HCC by blocking Wnt/β-catenin signaling and may be recommended to reduce the risk of HCC after SVR.
7.2 Zinc
Hosui et al. (170), showed that zinc supplementation reduced the long‐term cumulative incidence of de novo HCC in DAA‐treated patients after SVR. Zinc administration is required for the activation of many liver enzymes, such as superoxide dismutase (SOD), which has strong antioxidant activity. SOD deactivation increases ROS formation, subsequently inducing DNA damage, and protein modification, which are characteristics of hepatocarcinogenesis. The cellular loss of zinc in malignant hepatoma cells compared with the higher zinc levels in normal hepatocytes establishes the consistency of the zinc role in HCC (171). Furthermore, zinc supplementation directly inhibits fibrosis progression, which may decrease the risk of hepatocarcinogenesis (172). Similarly, zinc deficiency delays extracellular ATP clearance, which promote inflammation and the risk of hepatocarcinogenesis (173). Overall, these data indicate that zinc therapy may reduce the risk of HCC in cACLD patients after a virological cure.
8 Conclusion
cACLD encompasses a diverse range of patients with varying risks of HCC after HCV eradication. Although individualized risk stratification of cACLD patients appears reasonable and appealing, no validated HCC risk score has been established for tailoring HCC surveillance after achieving SVR. However, certain factors such as old age, DM, ongoing alcohol intake, and hepatic steatosis indicate a high risk for HCC and require close monitoring. In addition, the existence of noncharacterized hepatic nodules before DAA treatment indicates the need for short-term, meticulous HCC surveillance. Specifically, pretreatment values of FIB-4 index ≥3.25, M2BPGi >1.70–2.00, and LSM ≥20 kPa warrant rigorous HCC surveillance. Prolonged HCC surveillance is recommended when the pretreatment values of AFP, FIB-4 index, and LSM remain elevated after SVR. Conversely, pretreatment values of FIB-4 <1.5, M2BPGi <1, and LSM <12 kPa suggest a lower HCC risk, allowing for the suspension of HCC surveillance after virological cure.
A more clarification of the impact of DAA-induced HCV eradication on the persistence of HCV oncogenic effects may facilitate the prediction of individual susceptibility to HCC, leading to more accurate risk categorization for patients with cACLD.
Author contributions
HHA and AElb conceptualized the review. HA, AM, AEli, AAls, ME, AM, AAla, SM, AAlw, wrote all drafts. HHA and AElb reviewed and edited the manuscript. HHA provided supervision. All authors contributed to the article and approved the submitted version.
Funding
The author(s) declare financial support was received for the research, authorship, and/or publication of this article. Grant in aid for scientific research: 23K06575. This study was funded by the Japanese Agency for Medical Research and Development (23fk0310503j0102, 23fk0310513j0702, and 23fk0310507j0902).
Conflict of interest
The authors declare that the research was conducted in the absence of any commercial or financial relationships that could be construed as a potential conflict of interest.
The authors HHA and MA declared that they were an editorial board member of Frontiers, at the time of submission. This had no impact on the peer review process and the final decision.
Publisher’s note
All claims expressed in this article are solely those of the authors and do not necessarily represent those of their affiliated organizations, or those of the publisher, the editors and the reviewers. Any product that may be evaluated in this article, or claim that may be made by its manufacturer, is not guaranteed or endorsed by the publisher.
References
1. Kanwal F, Kramer J, Asch SM, Chayanupatkul M, Cao Y, El-Serag HB. Risk of hepatocellular cancer in HCV patients treated with direct-acting antiviral agents. Gastroenterology (2017) 153(4):996–1005.e1. doi: 10.1053/j.gastro.2017.06.012
2. Carrat F, Fontaine H, Dorival C, Simony M, Diallo A, Hezode C, et al. Clinical outcomes in patients with chronic hepatitis C after direct-acting antiviral treatment: a prospective cohort study. Lancet (2019) 393(10179):1453–64. doi: 10.1016/S0140-6736(18)32111-1
3. Ioannou GN, Green PK, Berry K. HCV eradication induced by direct-acting antiviral agents reduces the risk of hepatocellular carcinoma. J Hepatol (2017) S0168-8278(17):32273-0. doi: 10.1016/j.jhep.2017.08.030
4. Celsa C, Stornello C, Giuffrida P, Giacchetto CM, Grova M, Rancatore G, et al. Direct-acting antiviral agents and risk of hepatocellular carcinoma: Critical appraisal of the evidence. Ann Hepatol (2022) Suppl 1:100568. doi: 10.1016/j.aohep.2021.100568
5. D’Ambrosio R, Degasperi E, Anolli MP, Fanetti I, Borghi M, Soffredini R, et al. Incidence of liver- and non-liver-related outcomes in patients with HCV-cirrhosis after SVR. J Hepatol (2022) 76(2):302–10. doi: 10.1016/j.jhep.2021.09.013
6. Reig M, Mariño Z, Perelló C, Iñarrairaegui M, Ribeiro A, Lens S, et al. Unexpected high rate of early tumor recurrence in patients with HCV-related HCC undergoing interferon-free therapy. J Hepatol (2016) 65(4):719–26. doi: 10.1016/j.jhep.2016.04.008
7. Conti F, Buonfiglioli F, Scuteri A, Crespi C, Bolondi L, Caraceni P, et al. Early occurrence and recurrence of hepatocellular carcinoma in HCV-related cirrhosis treated with direct-acting antivirals. J Hepatol (2016) 65(4):727–33. doi: 10.1016/j.jhep.2016.06.015
8. ANRS collaborative study group on hepatocellular carcinoma (ANRS CO22 HEPATHER, CO12 CirVir and CO23 CUPILT cohorts). Electronic address:c3RhbmlzbGFzLnBvbEBhcGhwLmZy. Lack of evidence of an effect of direct-acting antivirals on the recurrence of hepatocellular carcinoma: Data from three ANRS cohorts. J Hepatol (2016) 65(4):734–40. doi: 10.1016/j.jhep.2016.05.045
9. Sapena V, Enea M, Torres F, Celsa C, Rios J, Rizzo GEM, et al. Hepatocellular carcinoma recurrence after direct-acting antiviral therapy: an individual patient data meta-analysis. Gut (2022) 71(3):593–604. doi: 10.1136/gutjnl-2020-323663
10. Nahon P, Layese R, Bourcier V, Cagnot C, Marcellin P, Guyader D, et al. Incidence of hepatocellular carcinoma after direct antiviral therapy for HCV in patients with cirrhosis included in surveillance programs. Gastroenterology (2018) 155(5):1436–1450.e6. doi: 10.1053/j.gastro.2018.07.015
11. Abdelaziz AO, Nabil MM, Abdelmaksoud AH, Shousha HI, Hashem MB, Hassan EM, et al. Tumor behavior of hepatocellular carcinoma after hepatitis C treatment by direct-acting antivirals: comparative analysis with non-direct-acting antivirals-treated patients. Eur J Gastroenterol Hepatol (2019) 31(1):75–9. doi: 10.1097/MEG.0000000000001264
12. El Fayoumie M, Abdelhady M, Gawish A, Hantour U, Abdelkhaleek I, Abdelraheem M, et al. Changing patterns of hepatocellular carcinoma after treatment with direct antiviral agents. Gastrointest Tumors (2020) 7(1-2):50–60. doi: 10.1159/000505326
13. Brozzetti S, Tancredi M, Bini S, De Lucia C, Antimi J, D’Alterio C, et al. HCC in the era of direct-acting antiviral agents (DAAs): surgical and other curative or palliative strategies in the elderly. Cancers (Basel) (2021) 13(12):3025. doi: 10.3390/cancers13123025
14. Caviglia GP, Troshina G, Santaniello U, Rosati G, Bombaci F, Birolo G, et al. Long-term hepatocellular carcinoma development and predictive ability of non-invasive scoring systems in patients with HCV-related cirrhosis treated with direct-acting antivirals. Cancers (Basel) (2022) 14(3):828. doi: 10.3390/cancers14030828
15. Calvaruso V, Petta S, Cacciola I, Cabibbo G, Cartabellotta F, Distefano M, et al. Liver and cardiovascular mortality after hepatitis C virus eradication by DAA: Data from RESIST-HCV cohort. J Viral Hepat (2021) 28(8):1190–9. doi: 10.1111/jvh.13523
16. Hattori N, Ikeda H, Watanabe T, Satta Y, Ehira T, Suzuki T, et al. Risk factors for liver-related mortality of patients with hepatitis C virus after sustained virologic response to direct-acting antiviral agents. JGH Open (2022) 6(10):685–91. doi: 10.1002/jgh3.12805
17. Brozzetti S, Bini S, Fazzi K, Chiarella LL, Ceccarossi V, De Lucia C, et al. Case-report: Metastases in a low-stage middle-graded HCC in cleared HCV infection, non-cirrhotic liver: Surgical therapy. Int J Surg Case Rep (2018) 47:19–21. doi: 10.1016/j.ijscr.2018.04.013
18. Goossens N, Hoshida Y. Hepatitis C virus-induced hepatocellular carcinoma. Clin Mol Hepatol (2015) 21(2):105–14. doi: 10.3350/cmh.2015.21.2.105
19. Shirvani-Dastgerdi E, Schwartz RE, Ploss A. Hepatocarcinogenesis associated with hepatitis B, delta and C viruses. Curr Opin Virol (2016) 20:1–10. doi: 10.1016/j.coviro.2016.07.009
20. Liu P, Ge M, Hu J, Li X, Che L, Sun K, et al. A functional mammalian target of rapamycin complex 1 signaling is indispensable for c-Myc-driven hepatocarcinogenesis. Hepatology (2017) 66(1):167–81. doi: 10.1002/hep.29183
21. Chu PS, Nakamoto N, Taniki N, Ojiro K, Amiya T, Makita Y, et al. On-treatment decrease of NKG2D correlates to early emergence of clinically evident hepatocellular carcinoma after interferon-free therapy for chronic hepatitis C. PloS One (2017) 12(6):e0179096. doi: 10.1371/journal.pone.0179096
22. Kanwal F, Kramer JR, Asch SM, Cao Y, Li L, El-Serag HB. Long-term risk of hepatocellular carcinoma in HCV patients treated with direct acting antiviral agents. Hepatology (2020) 71(1):44–55. doi: 10.1002/hep.30823
23. de Franchis R, Baveno VI Faculty. Expanding consensus in portal hypertension: Report of the Baveno VI Consensus Workshop: Stratifying risk and individualizing care for portal hypertension. J Hepatol (2015) 63(3):743–52. doi: 10.1016/j.jhep.2015.05.022
24. Berzigotti A. Advances and challenges in cirrhosis and portal hypertension. BMC Med (2017) 15(1):200. doi: 10.1186/s12916-017-0966-6
25. de Franchis R, Bosch J, Garcia-Tsao G, Reiberger T, Ripoll C, Baveno VII Faculty. Baveno VII - Renewing consensus in portal hypertension. J Hepatol (2022) 76(4):959–74. doi: 10.1016/j.jhep.2021.12.022
26. Mendoza Y, Cocciolillo S, Murgia G, Chen T, Margini C, Sebastiani G, et al. Noninvasive markers of portal hypertension detect decompensation in overweight or obese patients with compensated advanced chronic liver disease. Clin Gastroenterol Hepatol (2020) 18(13):3017–3025.e6. doi: 10.1016/j.cgh.2020.04.018
27. Poynard T, Vergniol J, Ngo Y, Foucher J, Munteanu M, Merrouche W, et al. Staging chronic hepatitis C in seven categories using fibrosis biomarker (FibroTest™) and transient elastography (FibroScan®). J Hepatol (2014) 60(4):706–14. doi: 10.1016/j.jhep.2013.11.016
28. Petta S, Sebastiani G, Viganò M, Ampuero J, Wai-Sun Wong V, Boursier J, et al. Monitoring occurrence of liver-related events and survival by transient elastography in patients with nonalcoholic fatty liver disease and compensated advanced chronic liver disease. Clin Gastroenterol Hepatol (2021) 19(4):806–815.e5. doi: 10.1016/j.cgh.2020.06.045
29. Rasmussen DN, Thiele M, Johansen S, Kjærgaard M, Lindvig KP, Israelsen M, et al. Prognostic performance of 7 biomarkers compared to liver biopsy in early alcohol-related liver disease. J Hepatol (2021) 75(5):1017–25. doi: 10.1016/j.jhep.2021.05.037
30. Rockey DC, Caldwell SH, Goodman ZD, Nelson RC, Smith AD. American association for the study of liver diseases. Liver biopsy. Hepatol (2009) 49(3):1017–44. doi: 10.1002/hep.22742
31. Morgan TR, Ghany MG, Kim HY, Snow KK, Shiffman ML, De Santo JL, et al. Outcome of sustained virological responders with histologically advanced chronic hepatitis C. Hepatology (2010) 52(3):833–44. doi: 10.1002/hep.23744
32. Morgan RL, Baack B, Smith BD, Yartel A, Pitasi M, Falck-Ytter Y. Eradication of hepatitis C virus infection and the development of hepatocellular carcinoma: a meta-analysis of observational studies. Ann Intern Med (2013) 158(5 Pt 1):329–37. doi: 10.7326/0003-4819-158-5-201303050-00005
33. Li DK, Ren Y, Fierer DS, Rutledge S, Shaikh OS, Lo Re V 3rd, et al. The short-term incidence of hepatocellular carcinoma is not increased after hepatitis C treatment with direct-acting antivirals: An ERCHIVES study. Hepatology (2018) 67(6):2244–53. doi: 10.1002/hep.29707
34. Leal C, Strogoff-de-Matos J, Theodoro C, Teixeira R, Perez R, Guaraná T, et al. Incidence and risk factors of hepatocellular carcinoma in patients with chronic hepatitis C treated with direct-acting antivirals. Viruses (2023) 15(1):221. doi: 10.3390/v15010221
35. Lockart I, Yeo MGH, Hajarizadeh B, Dore GJ, Danta M. HCC incidence after hepatitis C cure among patients with advanced fibrosis or cirrhosis: A meta-analysis. Hepatology (2022) 76(1):139–54. doi: 10.1002/hep.32341
36. Hamdane N, Jühling F, Crouchet E, El Saghire H, Thumann C, Oudot MA, et al. HCV-induced epigenetic changes associated with liver cancer risk persist after sustained virologic response. Gastroenterology (2019) 156(8):2313–2329.e7. doi: 10.1053/j.gastro.2019.02.038
37. Pietropaolo V, Prezioso C, Moens U. Role of virus-induced host cell epigenetic changes in cancer. Int J Mol Sci (2021) 22(15):8346. doi: 10.3390/ijms22158346
38. Mui UN, Haley CT, Tyring SK. Viral oncology: molecular biology and pathogenesis. J Clin Med (2017) 6:111. doi: 10.3390/jcm6120111
39. Arzumanyan A, Reis HM, Feitelson MA. Pathogenic mechanisms in HBV- and HCV-associated hepatocellular carcinoma. Nat Rev Cancer (2013) 13:123–35. doi: 10.1038/nrc3449
40. Rusyn I, Lemon SM. Mechanisms of HCV-induced liver cancer: What did we learn from in vitro and animal studies? Cancer Lett (2014) 345:210–5. doi: 10.1016/j.canlet.2013.06.028
41. McGivern DR, Lemon SM. Virus-specific mechanisms of carcinogenesis in hepatitis C virus associated liver cancer. Oncogene (2011) 30:1969–83. doi: 10.1038/onc.2010.594
42. Arora P, Kim EO, Jung JK, Jang KL. Hepatitis C virus core protein downregulates E-cadherin expression via activation of DNA methyltransferase 1 and 3b. Cancer Lett (2008) 261:244–52. doi: 10.1016/j.canlet.2007.11.033
43. Ripoli M, Barbano R, Balsamo T, Piccoli C, Brunetti V, Coco M, et al. Hypermethylated levels of E-cadherin promoter in Huh-7 cells expressing the HCV core protein. Virus Res (2011) 160:74–81. doi: 10.1016/j.virusres.2011.05.014
44. Park SH, Lim JS, Lim SY, Tiwari I, Jang KL. Hepatitis C virus Core protein stimulates cell growth by down-regulating p16 expression via DNA methylation. Cancer Lett (2011) 310:616–8. doi: 10.1016/j.canlet.2011.06.012
45. Benegiamo G, Vinciguerra M, Mazzoccoli G, Piepoli A, Andriulli A, Pazienza V. DNA methyltransferases 1 and 3b expression in Huh-7 cells expressing HCV core protein of different genotypes. Dig Dis Sci (2012) 57:1598–603. doi: 10.1007/s10620-012-2160-1
46. Rongrui L, Na H, Zongfang L, Fanpu J, Shiwen J. Epigenetic mechanism involved in the HBV/HCV-related hepatocellular carcinoma tumorigenesis. Curr Pharm Des (2014) 20:1715–25. doi: 10.2174/13816128113199990533
47. Pazienza V, Panebianco C, Andriulli A. Hepatitis viruses exploitation of host DNA methyltransferases functions. Clin Exp Med (2016) 16:265–72. doi: 10.1007/s10238-015-0372-3
48. Mekky MA, Salama RH, Abdel-Aal MF, Ghaliony MA, Zaky S. Studying the frequency of aberrant DNA methylation of APC, P14, and E-cadherin genes in HCV-related hepatocarcinogenesis. Cancer biomark (2018) 22:503–9. doi: 10.3233/CBM-171156
49. Zhou Y, Wang XB, Qiu XP, Shuai Z, Wang C, Zheng F. CDKN2A promoter methylation and hepatocellular carcinoma risk: A meta-analysis. Clin Res Hepatol Gastroenterol (2018) 42:529–41. doi: 10.1016/j.clinre.2017.07.003
50. Baharudin R, Tieng FYF, Lee LH, Ab Mutalib NS. Epigenetics of SFRP1: the dual roles in human cancers. Cancers (2020) 12:445. doi: 10.3390/cancers12020445
51. Kasai H, Mochizuki K, Tanaka T, Yamashita A, Matsuura Y, Moriishi K. Induction of HOX genes by hepatitis C virus infection via impairment of histone H2A monoubiquitination. J Virol (2021) 95:e01784–20. doi: 10.1128/JVI.01784-20
52. Duong FH, Christen V, Lin S, Heim MH. Hepatitis C virus-induced up-regulation of protein phosphatase 2A inhibits histone modification and DNA damage repair. Hepatology (2010) 51:741–51. doi: 10.1002/hep.23388
53. Perez S, Kaspi A, Domovitz T, Davidovich A, Lavi-Itzkovitz A, Meirson T, et al. Hepatitis C virus leaves an epigenetic signature post cure of infection by direct-acting antivirals. PloS Genet (2019) 15:e1008181. doi: 10.1371/journal.pgen.1008181
54. Pascut D, Hoang M, Nguyen NNQ, Pratama MY, Tiribelli C. HCV proteins modulate the host cell miRNA expression contributing to hepatitis C pathogenesis and hepatocellular carcinoma development. Cancers (2021) 13:2485. doi: 10.3390/cancers13102485
55. Ura S, Honda M, Yamashita T, Ueda T, Takatori H, Nishino R, et al. Differential microRNA expression between hepatitis B and hepatitis C leading disease progression to hepatocellular carcinoma. Hepatology (2009) 49:1098–112. doi: 10.1002/hep.22749
56. Zeng B, Li Z, Chen R, Guo N, Zhou J, Zhou Q, et al. Epigenetic regulation of miR-124 by hepatitis C virus core protein promotes migration and invasion of intrahepatic cholangiocarcinoma cells by targeting SMYD3. FEBS Lett (2012) 586:3271–8. doi: 10.1016/j.febslet.2012.06.049
57. Oura K, Morishita A, Masaki T. Molecular and functional roles of microRNAs in the progression of hepatocellular carcinomaA review. Int J Mol Sci (2020) 21:8362. doi: 10.3390/ijms21218362
58. Zheng F, Liao YJ, Cai MY, Liu YH, Liu TH, Chen SP, et al. The putative tumour suppressor microRNA-124 modulates hepatocellular carcinoma cell aggressiveness by repressing ROCK2 and EZH2. Gut (2012) 61:278–89. doi: 10.1136/gut.2011.239145
59. D’souza S, Lau KC, Coffin CS, Patel TR. Molecular mechanisms of viral hepatitis induced hepatocellular carcinoma. World J Gastroenterol (2020) 26(38):5759–83. doi: 10.3748/wjg.v26.i38.5759
60. Lin D, Reddy V, Osman H, Lopez A, Koksal AR, Rhadhi SM, et al. Additional inhibition of wnt/β-catenin signaling by metformin in DAA treatments as a novel therapeutic strategy for HCV-infected patients. Cells (2021) 10(4):790. doi: 10.3390/cells10040790
61. Santangelo L, Bordoni V, Montaldo C, Cimini E, Zingoni A, Battistelli C, et al. Hepatitis C virus direct-acting antivirals therapy impacts on extracellular vesicles microRNAs content and on their immunomodulating properties. Liver Int (2018) 38(10):1741–50. doi: 10.1111/liv.13700
62. Köberle V, Waidmann O, Kronenberger B, Andrei A, Susser S, Füller C, et al. Serum microRNA-122 kinetics in patients with chronic hepatitis C virus infection during antiviral therapy. J Viral Hepat (2013) 20(8):530–5. doi: 10.1111/jvh.12075
63. Villani R, Facciorusso A, Bellanti F, Tamborra R, Piscazzi A, Landriscina M, et al. DAAs Rapidly Reduce Inflammation but Increase Serum VEGF Level: A Rationale for Tumor Risk during Anti-HCV Treatment. PloS One (2016) 11(12):e0167934. doi: 10.1371/journal.pone.0167934
64. Faillaci F, Marzi L, Critelli R, Milosa F, Schepis F, Turola E, et al. Liver angiopoietin-2 is a key predictor of de novo or recurrent hepatocellular cancer after hepatitis C virus direct-acting antivirals. Hepatology (2018) 68(3):1010–24. doi: 10.1002/hep.29911
65. Grivennikov SI, Greten FR, Karin M. Immunity, inflammation, and cancer. Cell (2010) 140(6):883–99. doi: 10.1016/j.cell.2010.01.025
66. Nakagawa H, Maeda S, Yoshida H, Tateishi R, Masuzaki R, Ohki T, et al. Serum IL-6 levels and the risk for hepatocarcinogenesis in chronic hepatitis C patients: an analysis based on gender differences. Int J Cancer (2009) 125(10):2264–9. doi: 10.1002/ijc.24720
67. Aroucha DC, do Carmo RF, Moura P, Silva JL, Vasconcelos LR, Cavalcanti MS, et al. High tumor necrosis factor-α/interleukin-10 ratio is associated with hepatocellular carcinoma in patients with chronic hepatitis C. Cytokine (2013) 62(3):421–5. doi: 10.1016/j.cyto.2013.03.024
68. Piconese S, Timperi E, Pacella I, Schinzari V, Tripodo C, Rossi M, et al. Human OX40 tunes the function of regulatory T cells in tumor and nontumor areas of hepatitis C virus-infected liver tissue. Hepatology (2014) 60(5):1494–507. doi: 10.1002/hep.27188
69. Alao H, Cam M, Keembiyehetty C, Zhang F, Serti E, Suarez D, et al. Baseline intrahepatic and peripheral innate immunity are associated with hepatitis C virus clearance during direct-acting antiviral therapy. Hepatology (2018) 68(6):2078–88. doi: 10.1002/hep.29921
70. Kamimura H, Yamagiwa S, Tsuchiya A, Takamura M, Matsuda Y, Ohkoshi S, et al. Reduced NKG2D ligand expression in hepatocellular carcinoma correlates with early recurrence. J Hepatol (2012) 56(2):381–8. doi: 10.1016/j.jhep.2011.06.017
71. Lu MY, Yeh ML, Huang CI, Wang SC, Tsai YS, Tsai PC, et al. Dynamics of cytokines predicts risk of hepatocellular carcinoma among chronic hepatitis C patients after viral eradication. World J Gastroenterol (2022) 28(1):140–53. doi: 10.3748/wjg.v28.i1.140
72. Wieland D, Kemming J, Schuch A, Emmerich F, Knolle P, Neumann-Haefelin C, et al. TCF1+ hepatitis C virus-specific CD8+ T cells are maintained after cessation of chronic antigen stimulation. Nat Commun (2017) 8:15050. doi: 10.1038/ncomms15050
73. Aregay A, Owusu Sekyere S, Deterding K, Port K, Dietz J, Berkowski C, et al. Elimination of hepatitis C virus has limited impact on the functional and mitochondrial impairment of HCV-specific CD8+ T cell responses. J Hepatol (2019) 71(5):889–99. doi: 10.1016/j.jhep.2019.06.025
74. Vranjkovic A, Deonarine F, Kaka S, Angel JB, Cooper CL, Crawley AM. Direct-acting antiviral treatment of HCV infection does not resolve the dysfunction of circulating CD8+ T-cells in advanced liver disease. Front Immunol (2019) 10:1926. doi: 10.3389/fimmu.2019.01926
75. Claassen MA, de Knegt RJ, Janssen HL, Boonstra A. Retention of CD4+ CD25+ FoxP3+ regulatory T cells in the liver after therapy-induced hepatitis C virus eradication in humans. J Virol (2011) 85(11):5323–30. doi: 10.1128/JVI.02551-10
76. Spaan M, Claassen MA, Hou J, Janssen HL, de Knegt RJ, Boonstra A. The intrahepatic T cell compartment does not normalize years after therapy-induced hepatitis C virus eradication. J Infect Dis (2015) 212(3):386–90. doi: 10.1093/infdis/jiv059
77. Langhans B, Nischalke HD, Krämer B, Hausen A, Dold L, van Heteren P, et al. Increased peripheral CD4+ regulatory T cells persist after successful direct-acting antiviral treatment of chronic hepatitis C. J Hepatol (2017) 66(5):888–96. doi: 10.1016/j.jhep.2016.12.019
78. Saldarriaga OA, Dye B, Pham J, Wanninger TG, Millian D, Kueht M, et al. Comparison of liver biopsies before and after direct-acting antiviral therapy for hepatitis C and correlation with clinical outcome. Sci Rep (2021) 11(1):14506. doi: 10.1038/s41598-021-93881-7
79. D’Ambrosio R, Aghemo A, Rumi MG, Ronchi G, Donato MF, Paradis V, et al. A morphometric and immunohistochemical study to assess the benefit of a sustained virological response in hepatitis C virus patients with cirrhosis. Hepatology (2012) 56(2):532–43. doi: 10.1002/hep.25606
80. D’Ambrosio R, Aghemo A, Fraquelli M, Rumi MG, Donato MF, Paradis V, et al. The diagnostic accuracy of Fibroscan for cirrhosis is influenced by liver morphometry in HCV patients with a sustained virological response. J Hepatol (2013) 59(2):251–6. doi: 10.1016/j.jhep.2013.03.013
81. Bachofner JA, Valli PV, Kröger A, Bergamin I, Künzler P, Baserga A, et al. Direct antiviral agent treatment of chronic hepatitis C results in rapid regression of transient elastography and fibrosis markers fibrosis-4 score and aspartate aminotransferase-platelet ratio index. Liver Int (2017) 37(3):369–76. doi: 10.1111/liv.13256
82. Sporea I, Lupuşoru R, Mare R, Popescu A, Gheorghe L, Iacob S, et al. Dynamics of liver stiffness values by means of transient elastography in patients with HCV liver cirrhosis undergoing interferon free treatment. J Gastrointest Liver Dis (2017) 26(2):145–50. doi: 10.15403/jgld.2014.1121.262.dyn
83. Pockros P, Crissien-Martinez A, Frenette C, Skillin C, Bao F, Du E, et al. Degree of Liver Fibrosis Regression Predicted by Transient Elastography after Cure of Chronic Hepatitis C with Direct Acting Antivirals Is Overestimated but Confirmed by Liver Biopsy. J Hepatol (2017) 66:S108. doi: 10.1016/S0168-8278(17)30475-0
84. Afdhal N, Everson GT, Calleja JL, McCaughan GW, Bosch J, Brainard DM, et al. Effect of viral suppression on hepatic venous pressure gradient in hepatitis C with cirrhosis and portal hypertension. J Viral Hepat (2017) 24(10):823–31. doi: 10.1111/jvh.12706
85. Seth A, Sherman KE. Hepatitis C virus cure is the start of the end for patients with advanced fibrosis/cirrhosis. Clin Infect Dis (2020) 71(10):2730–1. doi: 10.1093/cid/ciaa509
86. Suk KT. Hepatic venous pressure gradient: clinical use in chronic liver disease. Clin Mol Hepatol (2014) 20(1):6–14. doi: 10.3350/cmh.2014.20.1.6
87. Díez C, Berenguer J, Ibañez-Samaniego L, Llop E, Pérez-Latorre L, Catalina MV, et al. Persistence of clinically significant portal hypertension after eradication of hepatitis C virus in patients with advanced cirrhosis. Clin Infect Dis (2020) 71(10):2726–9. doi: 10.1093/cid/ciaa502
88. Lens S, Alvarado-Tapias E, Mariño Z, Londoño MC, LLop E, Martinez J, et al. Effects of all-oral anti-viral therapy on HVPG and systemic hemodynamics in patients with hepatitis C virus-associated cirrhosis. Gastroenterology (2017) 153(5):1273–1283.e1. doi: 10.1053/j.gastro.2017.07.016
89. Tacke F, Boeker KHW, Klinker H, Heyne R, Buggisch P, Pathil A, et al. Baseline risk factors determine lack of biochemical response after SVR in chronic hepatitis C patients treated with DAAs. Liver Int (2020) 40(3):539–48. doi: 10.1111/liv.14186
90. Aoyagi H, Iijima H, Gaber ES, Zaitsu T, Matsuda M, Wakae K, et al. Hepatocellular organellar abnormalities following elimination of hepatitis C virus. Liver Int (2023) 43(8):1677–90. doi: 10.1111/liv.15624
91. Alonso López S, Manzano ML, Gea F, Gutiérrez ML, Ahumada AM, Devesa MJ, et al. A model based on noninvasive markers predicts very low hepatocellular carcinoma risk after viral response in hepatitis C virus-advanced fibrosis. Hepatology (2020) 72(6):1924–34. doi: 10.1002/hep.31588
92. Tani J, Morishita A, Sakamoto T, Takuma K, Nakahara M, Fujita K, et al. Simple scoring system for prediction of hepatocellular carcinoma occurrence after hepatitis C virus eradication by direct-acting antiviral treatment: All Kagawa Liver Disease Group Study. Oncol Lett (2020) 19(3):2205–12. doi: 10.3892/ol.2020.11341
93. Mawatari S, Kumagai K, Oda K, Tabu K, Ijuin S, Fujisaki K, et al. Features of patients who developed hepatocellular carcinoma after direct-acting antiviral treatment for hepatitis C Virus. PloS One (2022) 17(1):e0262267. doi: 10.1371/journal.pone.0262267
94. Ogawa E, Nomura H, Nakamuta M, Furusyo N, Kajiwara E, Dohmen K, et al. Development of hepatocellular carcinoma in patients aged 75-84 years with chronic hepatitis C treated with direct-acting antivirals. J Infect Dis (2022) 226(3):431–40. doi: 10.1093/infdis/jiaa359
95. Rinaldi L, Perrella A, Guarino M, De Luca M, Piai G, Coppola N, et al. Incidence and risk factors of early HCC occurrence in HCV patients treated with direct acting antivirals: a prospective multicentre study. J Transl Med (2019) 17(1):292. doi: 10.1186/s12967-019-2033-x
96. Degasperi E, D’Ambrosio R, Iavarone M, Sangiovanni A, Aghemo A, Soffredini R, et al. Factors associated with increased risk of de novo or recurrent hepatocellular carcinoma in patients with cirrhosis treated with direct-acting antivirals for HCV infection. Clin Gastroenterol Hepatol (2019) 17(6):1183–1191.e7. doi: 10.1016/j.cgh.2018.10.038
97. Lieber CS, Seitz HK, Garro AJ, Worner TM. Alcohol-related diseases and carcinogenesis. Cancer Res (1979) 39(7 Pt 2):2863–86.
98. Mariño Z, Darnell A, Lens S, Sapena V, Díaz A, Belmonte E, et al. Time association between hepatitis C therapy and hepatocellular carcinoma emergence in cirrhosis: Relevance of non-characterized nodules. J Hepatol (2019) 70(5):874–84. doi: 10.1016/j.jhep.2019.01.005
99. Imamura T, Okamura Y, Ohshima K, Uesaka K, Sugiura T, Ito T, et al. Hepatocellular carcinoma after a sustained virological response by direct-acting antivirals harbors TP53 inactivation. Cancer Med (2022) 11(8):1769–86. doi: 10.1002/cam4.4571
100. Miki D, Akita T, Kurisu A, Kawaoka T, Nakajima T, Hige S, et al. PNPLA3 and HLA-DQB1 polymorphisms are associated with hepatocellular carcinoma after hepatitis C virus eradication. J Gastroenterol (2020) 55(12):1162–70. doi: 10.1007/s00535-020-01731-6
101. Lee SS, Kim CY, Kim BR, Cha RR, Kim WS, Kim JJ, et al. Hepatitis C virus genotype 3 was associated with the development of hepatocellular carcinoma in Korea. J Viral Hepat (2019) 26(4):459–65. doi: 10.1111/jvh.13047
102. Tayyab GUN, Rasool S, Nasir B, Rubi G, Abou-Samra AB, Butt AA. Hepatocellular carcinoma occurs frequently and early after treatment in HCV genotype 3 infected persons treated with DAA regimens. BMC Gastroenterol (2020) 20(1):93. doi: 10.1186/s12876-020-01249-4
103. Corma-Gómez A, Macías J, Téllez F, Freyre-Carrillo C, Morano L, Rivero-Juárez A, et al. Liver stiffness at the time of sustained virological response predicts the clinical outcome in people living with human immunodeficiency virus and hepatitis C virus with advanced fibrosis treated with direct-acting antivirals. Clin Infect Dis (2020) 71(9):2354–62. doi: 10.1093/cid/ciz1140
104. Corma-Gómez A, Macías J, Lacalle-Remigio JR, Téllez F, Morano L, Rivero A, et al. Human immunodeficiency virus (HIV) infection is associated with lower risk of hepatocellular carcinoma after sustained virological response to direct-acting antivirals in hepatitis C infected patients with advanced fibrosis. Clin Infect Dis (2021) 73(7):e2109–16. doi: 10.1093/cid/ciaa1111
105. Leandro G, Mangia A, Hui J, Fabris P, Rubbia-Brandt L, Colloredo G, et al. Relationship between steatosis, inflammation, and fibrosis in chronic hepatitis C: a meta-analysis of individual patient data. Gastroenterology (2006) 130(6):1636–42. doi: 10.1053/j.gastro.2006.03.014
106. Peleg N, Issachar A, Sneh Arbib O, Cohen-Naftaly M, Harif Y, Oxtrud E, et al. Liver steatosis is a major predictor of poor outcomes in chronic hepatitis C patients with sustained virological response. J Viral Hepat (2019) 26(11):1257–65. doi: 10.1111/jvh.13167
107. Sangiovanni A, Alimenti E, Gattai R, Filomia R, Parente E, Valenti L, et al. Undefined/non-malignant hepatic nodules are associated with early occurrence of HCC in DAA-treated patients with HCV-related cirrhosis. J Hepatol (2020) 73(3):593–602. doi: 10.1016/j.jhep.2020.03.030
108. Romano A, Angeli P, Piovesan S, Noventa F, Anastassopoulos G, Chemello L, et al. Newly diagnosed hepatocellular carcinoma in patients with advanced hepatitis C treated with DAAs: A prospective population study. J Hepatol (2018) 69(2):345–52. doi: 10.1016/j.jhep.2018.03.009
109. Akuta N, Kobayashi M, Suzuki F, Sezaki H, Fujiyama S, Kawamura Y, et al. Liver fibrosis and body mass index predict hepatocarcinogenesis following eradication of hepatitis C virus RNA by direct-acting antivirals. Oncology (2016) 91(6):341–7. doi: 10.1159/000450551
110. Masetti C, Lionetti R, Lupo M, Siciliano M, Giannelli V, Ponziani FR, et al. Lack of reduction in serum alpha-fetoprotein during treatment with direct antiviral agents predicts hepatocellular carcinoma development in a large cohort of patients with hepatitis C virus-related cirrhosis. J Viral Hepat (2018) 25(12):1493–500. doi: 10.1111/jvh.12982
111. Kuno A, Ikehara Y, Tanaka Y, Ito K, Matsuda A, Sekiya S, et al. A serum “sweet-doughnut” protein facilitates fibrosis evaluation and therapy assessment in patients with viral hepatitis. Sci Rep (2013) 3:1065. doi: 10.1038/srep01065
112. Shirabe K, Bekki Y, Gantumur D, Araki K, Ishii N, Kuno A, et al. Mac-2 binding protein glycan isomer (M2BPGi) is a new serum biomarker for assessing liver fibrosis: more than a biomarker of liver fibrosis. J Gastroenterol (2018) 53(7):819–26. doi: 10.1007/s00535-017-1425-z
113. Xu H, Kong W, Liu L, Chi X, Wang X, Wu R, et al. Accuracy of M2BPGi, compared with Fibro Scan®, in analysis of liver fibrosis in patients with hepatitis C. BMC Gastroenterol (2017) 17(1):62. doi: 10.1186/s12876-017-0618-5
114. Saleh SA, Salama MM, Alhusseini MM, Mohamed GA. M2BPGi for assessing liver fibrosis in patients with hepatitis C treated with direct-acting antivirals. World J Gastroenterol (2020) 26(21):2864–76. doi: 10.3748/wjg.v26.i21.2864
115. Ueda N, Kawaoka T, Imamura M, Aikata H, Nakahara T, Murakami E, et al. Liver fibrosis assessments using FibroScan, virtual-touch tissue quantification, the FIB-4 index, and mac-2 binding protein glycosylation isomer levels compared with pathological findings of liver resection specimens in patients with hepatitis C infection. BMC Gastroenterol (2020) 20(1):314. doi: 10.1186/s12876-020-01459-w
116. Chuaypen N, Chittmittrapap S, Avihingsanon A, Siripongsakun S, Wongpiyabovorn J, Tanpowpong N, et al. Liver fibrosis improvement assessed by magnetic resonance elastography and Mac-2-binding protein glycosylation isomer in patients with hepatitis C virus infection receiving direct-acting antivirals. Hepatol Res (2021) 51(5):528–37. doi: 10.1111/hepr.13630
117. Yamasaki K, Tateyama M, Abiru S, Komori A, Nagaoka S, Saeki A, et al. Elevated serum levels of Wisteria floribunda agglutinin-positive human Mac-2 binding protein predict the development of hepatocellular carcinoma in hepatitis C patients. Hepatology (2014) 60(5):1563–70. doi: 10.1002/hep.27305
118. European Association for the Study of the Liver. Electronic address:ZWFzbG9mZmljZUBlYXNsb2ZmaWNlLmV1; Clinical Practice Guidelines Panel: Chair:; EASL Governing Board representative:; Panel members:. EASL recommendations on treatment of hepatitis C: Final update of the series. J Hepatol (2020) 73(5):1170–218. doi: 10.1016/j.jhep.2020.08.018
119. AASLD-IDSA HCV Guidance Panel. Hepatitis C guidance 2018 update: AASLD-IDSA recommendations for testing, managing, and treating hepatitis C virus infection. Clin Infect Dis (2018) 67(10):1477–92. doi: 10.1093/cid/ciy585
120. Omata M, Kanda T, Wei L, Yu ML, Chuang WL, Ibrahim A, et al. APASL consensus statements and recommendation on treatment of hepatitis C. Hepatol Int (2016) 10(5):702–26. doi: 10.1007/s12072-016-9717-6
121. Yugawa K, Maeda T, Nagata S, Sakai A, Edagawa M, Omine T, et al. Mac-2-binding protein glycosylation isomer as a novel predictor of hepatocellular carcinoma recurrence in patients with hepatitis C virus eradication. Ann Surg Oncol (2022) 29(4):2711–9. doi: 10.1245/s10434-021-11011-z
122. Jun T, Hsu YC, Ogawa S, Huang YT, Yeh ML, Tseng CH, et al. Mac-2 binding protein glycosylation isomer as a hepatocellular carcinoma marker in patients with chronic hepatitis B or C infection. Hepatol Commun (2019) 3(4):493–503. doi: 10.1002/hep4.1321
123. Sasaki R, Yamasaki K, Abiru S, Komori A, Nagaoka S, Saeki A, et al. Serum Wisteria Floribunda Agglutinin-Positive Mac-2 Binding Protein Values Predict the Development of Hepatocellular Carcinoma among Patients with Chronic Hepatitis C after Sustained Virological Response. PloS One (2015) 10(6):e0129053. doi: 10.1371/journal.pone.0129053
124. Nagata H, Nakagawa M, Nishimura-Sakurai Y, Asano Y, Tsunoda T, Miyoshi M, et al. Serial measurement of Wisteria floribunda agglutinin positive Mac-2-binding protein is useful for predicting liver fibrosis and the development of hepatocellular carcinoma in chronic hepatitis C patients treated with IFN-based and IFN-free therapy. Hepatol Int (2016) 10(6):956–64. doi: 10.1007/s12072-016-9754-1
125. Ioannou GN, Green P, Lowy E, Mun EJ, Berry K. Differences in hepatocellular carcinoma risk, predictors and trends over time according to etiology of cirrhosis. PloS One (2018) 13(9):e0204412. doi: 10.1371/journal.pone.0204412
126. Ioannou GN, Beste LA, Green PK, Singal AG, Tapper EB, Waljee AK, et al. Increased risk for hepatocellular carcinoma persists up to 10 years after HCV eradication in patients with baseline cirrhosis or high FIB-4 scores. Gastroenterology (2019) 157(5):1264–1278.e4. doi: 10.1053/j.gastro.2019.07.033
127. Abraldes JG, Bureau C, Stefanescu H, Augustin S, Ney M, Blasco H, et al. Noninvasive tools and risk of clinically significant portal hypertension and varices in compensated cirrhosis: The “Anticipate” study. Hepatology (2016) 64(6):2173–84. doi: 10.1002/hep.28824
128. Pons M, Rodríguez-Tajes S, Esteban JI, Mariño Z, Vargas V, Lens S, et al. Non-invasive prediction of liver-related events in patients with HCV-associated compensated advanced chronic liver disease after oral antivirals. J Hepatol (2020) 72(3):472–80. doi: 10.1016/j.jhep.2019.10.005
129. Semmler G, Meyer EL, Kozbial K, Schwabl P, Hametner-Schreil S, Zanetto A, et al. HCC risk stratification after cure of hepatitis C in patients with compensated advanced chronic liver disease. J Hepatol (2022) 76(4):812–21. doi: 10.1016/j.jhep.2021.11.025
130. Ravaioli F, Conti F, Brillanti S, Andreone P, Mazzella G, Buonfiglioli F, et al. Hepatocellular carcinoma risk assessment by the measurement of liver stiffness variations in HCV cirrhotics treated with direct acting antivirals. Dig Liver Dis (2018) 50(6):573–9. doi: 10.1016/j.dld.2018.02.010
131. Reinoso-Pereira GL, Paranaguá-Vezozzo DC, Mazo DF, França JID, Ono SK, Carrilho FJ. High values of liver stiffness play an important role in stratifying the risk of hepatocellular carcinoma in cirrhotic hepatitis C patients. Arq Gastroenterol (2022) 59(2):204–11. doi: 10.1590/S0004-2803.202202000-38
132. Kobayashi T, Nakatsuka K, Shimizu M, Tamura H, Shinya E, Atsukawa M, et al. Ribavirin modulates the conversion of human CD4(+) CD25(-) T cell to CD4(+) CD25(+) FOXP3(+) T cell via suppressing interleukin-10-producing regulatory T cell. Immunology (2012) 137(3):259–70. doi: 10.1111/imm.12005
133. Tsai WL, Cheng JS, Liu PF, Chang TH, Sun WC, Chen WC, et al. Sofosbuvir induces gene expression for promoting cell proliferation and migration of hepatocellular carcinoma cells. Aging (Albany NY) (2022) 14(14):5710–26. doi: 10.18632/aging.204170
134. Ogawa E, Nomura H, Nakamuta M, Furusyo N, Kajiwara E, Dohmen K, et al. Incidence of hepatocellular carcinoma after treatment with sofosbuvir-based or sofosbuvir-free regimens in patients with chronic hepatitis C. Cancers (2020) 12(9):2602. doi: 10.3390/cancers12092602
135. Hedenstierna M, Nangarhari A, Weiland O, Aleman S. Diabetes and cirrhosis are risk factors for hepatocellular carcinoma after successful treatment of chronic hepatitis C. Clin Infect Dis (2016) 63(6):723–9. doi: 10.1093/cid/ciw362
136. Yamada R, Hiramatsu N, Oze T, Urabe A, Tahata Y, Morishita N, et al. Incidence and risk factors of hepatocellular carcinoma change over time in patients with hepatitis C virus infection who achieved sustained virologic response. Hepatol Res (2019) 49(5):570–8. doi: 10.1111/hepr.13310
137. Giovannucci E. Insulin and colon cancer. Cancer Causes Control (1995) 6(2):164–79. doi: 10.1007/BF00052777
138. Hu FB, Manson JE, Liu S, Hunter D, Colditz GA, Michels KB, et al. Prospective study of adult-onset diabetes mellitus (type 2) and risk of colorectal cancer in women. J Natl Cancer Inst (1999) 91(6):542–7. doi: 10.1093/jnci/91.6.542
139. Lee SC, Chan JC. Evidence for DNA damage as a biological link between diabetes and cancer. Chin Med J (2015) 128(11):1543–8. doi: 10.4103/0366-6999.157693
140. Ida H, Hagiwara S, Kono M, Minami T, Chishina H, Arizumi T, et al. Hepatocellular carcinoma after achievement of sustained viral response with daclatasvir and asunaprevir in patients with chronic hepatitis C virus infection. Dig Dis (2017) 35(6):565–73. doi: 10.1159/000480183
141. Watanabe T, Tokumoto Y, Joko K, Michitaka K, Horiike N, Tanaka Y, et al. Predictors of hepatocellular carcinoma occurrence after direct-acting antiviral therapy in patients with hepatitis C virus infection. Hepatol Res (2019) 49(2):136–46. doi: 10.1111/hepr.13278
142. Hirashima N, Shimada M, Urata N, Tsunekawa T, Unita S, Kondo T, et al. Predictive factors differ between hepatocellular carcinoma occurrence and recurrence after sustained virological responses by direct-acting antivirals in patients with hepatitis C. Open J Gastroenterol (2020) 10:1–13. doi: 10.4236/ojgas.2020.101001
143. Hagiwara H, Ito Y, Ohta T, Nozaki Y, Iwamoto T, Hosui A, et al. Incidence and risk factors of hepatocellular carcinoma in patients with hepatitis C who achieved a sustained virological response through direct-acting antiviral agents among the working population in Japan. JGH Open (2022) 6(6):395–401. doi: 10.1002/jgh3.12745
144. Ogawa E, Furusyo N, Nomura H, Dohmen K, Higashi N, Takahashi K, et al. Short-term risk of hepatocellular carcinoma after hepatitis C virus eradication following direct-acting anti-viral treatment. Aliment Pharmacol Ther (2018) 47(1):104–13. doi: 10.1111/apt.14380
145. Toyoda H, Tada T, Yasuda S, Mizuno K, Ito T, Kumada T. Dynamic evaluation of liver fibrosis to assess the risk of hepatocellular carcinoma in patients with chronic hepatitis C who achieved sustained virologic response. Clin Infect Dis (2020) 70:1208–14. doi: 10.1093/cid/ciz359
146. Kumada T, Toyoda H, Yasuda S, Tada T, Tanaka J. Usefulness of serial FIB-4 score measurement for predicting the risk of hepatocarcinogenesis after hepatitis C virus eradication. Eur J Gastroenterol Hepatol (2021) 33:e513–21. doi: 10.1097/MEG.0000000000002139
147. Tamaki N, Kurosaki M, Yasui Y, Mori N, Tsuji K, Hasebe C, et al. Change in fibrosis 4 index as predictor of high risk of incident hepatocellular carcinoma after eradication of hepatitis C virus. Clin Infect Dis (2021) 73(9):e3349–54. doi: 10.1093/cid/ciaa1307
148. Ciancio A, Ribaldone DG, Spertino M, Risso A, Ferrarotti D, Caviglia GP, et al. Who should not be surveilled for HCC development after successful therapy with DAAS in advanced chronic hepatitis C? Results of a long-term prospective study. Biomedicines (2023) 11:166. doi: 10.3390/biomedicines11010166
149. Kondili LA, Quaranta MG, Cavalletto L, Calvaruso V, Ferrigno L, D’Ambrosio R, et al. Profiling the risk of hepatocellular carcinoma after long-term HCV eradication in patients with liver cirrhosis in the PITER cohort. Dig Liver Dis (2023) 10:S1590–8658(23)00164-0. doi: 10.1016/j.dld.2023.01.153
150. Singer AW, Reddy KR, Telep LE, Osinusi AO, Brainard DM, Buti M, et al. Direct-acting antiviral treatment for hepatitis C virus infection and risk of incident liver cancer: a retrospective cohort study. Aliment Pharmacol Ther (2018) 47(9):1278–87. doi: 10.1111/apt.14593
151. Hamill V, Gelson W, MacDonald D, Richardson P, Ryder SD, Aldersley M, et al. Delivery of biannual ultrasound surveillance for individuals with cirrhosis and cured hepatitis C in the UK. Liver Int (2023) 43(4):917–27. doi: 10.1111/liv.15528
152. Laupacis A, Feeny D, Detsky AS, Tugwell PX. How attractive does a new technology have to be to warrant adoption and utilization? Tentative guidelines for using clinical and economic evaluations. CMAJ (1992) 146(4):473–81.
153. Lin OS, Keeffe EB, Sanders GD, Owens DK. Cost-effectiveness of screening for hepatocellular carcinoma in patients with cirrhosis due to chronic hepatitis C. Aliment Pharmacol Ther (2004) 19(11):1159–72. doi: 10.1111/j.1365-2036.2004.01963.x
154. Singal AG, Zhang E, Narasimman M, Rich NE, Waljee AK, Hoshida Y, et al. HCC surveillance improves early detection, curative treatment receipt, and survival in patients with cirrhosis: A meta-analysis. J Hepatol (2022) 77(1):128–39. doi: 10.1016/j.jhep.2022.01.023
155. Farhang Zangneh H, Wong WWL, Sander B, Bell CM, Mumtaz K, Kowgier M, et al. Cost effectiveness of hepatocellular carcinoma surveillance after a sustained virologic response to therapy in patients with hepatitis C virus infection and advanced fibrosis. Clin Gastroenterol Hepatol (2019) 17(9):1840–1849.e16. doi: 10.1016/j.cgh.2018.12.018
156. Ahn JC, Lee YT, Agopian VG, Zhu Y, You S, Tseng HR, et al. Hepatocellular carcinoma surveillance: current practice and future directions. Hepatoma Res (2022) 8:10. doi: 10.20517/2394-5079.2021.131
157. Atiq O, Tiro J, Yopp AC, Muffler A, Marrero JA, Parikh ND, et al. An assessment of benefits and harms of hepatocellular carcinoma surveillance in patients with cirrhosis. Hepatology (2017) 65(4):1196–205. doi: 10.1002/hep.28895
158. Singal AG, Patibandla S, Obi J, Fullington H, Parikh ND, Yopp AC, et al. Benefits and harms of hepatocellular carcinoma surveillance in a prospective cohort of patients with cirrhosis. Clin Gastroenterol Hepatol (2021) 19(9):1925–1932.e1. doi: 10.1016/j.cgh.2020.09.014
159. Isfordink CJ, Maan R, de Man RA, van Erpecum KJ, van der Meer AJ. Should we continue surveillance for hepatocellular carcinoma and gastroesophageal varices in patients with cirrhosis and cured HCV infection? Eur J Intern Med (2021) 94:6–14. doi: 10.1016/j.ejim.2021.08.023
160. Abe K, Wakabayashi H, Nakayama H, Suzuki T, Kuroda M, Yoshida N, et al. Factors associated with hepatocellular carcinoma occurrence after HCV eradication in patients without cirrhosis or with compensated cirrhosis. PloS One (2020) 15(12):e0243473. doi: 10.1371/journal.pone.0243473
161. Ahumada A, Rayón L, Usón C, Bañares R, Alonso Lopez S. Hepatocellular carcinoma risk after viral response in hepatitis C virus-advanced fibrosis: Who to screen and for how long? World J Gastroenterol (2021) 27(40):6737–49. doi: 10.3748/wjg.v27.i40.6737
162. D’Ambrosio R, Degasperi E, Lampertico P. Predicting hepatocellular carcinoma risk in patients with chronic HCV infection and a sustained virological response to direct-acting antivirals. J Hepatocell Carcinoma (2021) 8:713–39. doi: 10.2147/JHC.S292139
163. Liu YC, Cheng YT, Chen YC, Hsieh YC, Jeng WJ, Lin CY, et al. Comparing predictability of non-invasive tools for hepatocellular carcinoma in treated chronic hepatitis C patients. Dig Dis Sci (2023) 68(1):323–32. doi: 10.1007/s10620-022-07621-6
164. Singal AG, Sanduzzi-Zamparelli M, Nahon P, Ronot M, Hoshida Y, Rich N, et al. International Liver cancer Association (ILCA) White Paper on Hepatocellular carcinoma Risk Stratification and Surveillance. J Hepatol (2023) 26:S0168–8278(23)00111-3. doi: 10.1016/j.jhep.2023.02.022
165. Mueller PP, Chen Q, Ayer T, Nemutlu GS, Hajjar A, Bethea ED, et al. Duration and cost-effectiveness of hepatocellular carcinoma surveillance in hepatitis C patients after viral eradication. J Hepatol (2022) 77(1):55–62. doi: 10.1016/j.jhep.2022.01.027
166. European Association for the Study of the Liver. Electronic address:ZWFzbG9mZmljZUBlYXNsb2ZmaWNlLmV1; European Association for the Study of the Liver. EASL Clinical Practice Guidelines: Management of hepatocellular carcinoma. J Hepatol (2018) 69(1):182–236. doi: 10.1016/j.jhep.2018.03.019
167. Simmons OL, Feng Y, Parikh ND, Singal AG. Primary care provider practice patterns and barriers to hepatocellular carcinoma surveillance. Clin Gastroenterol Hepatol (2019) 17(4):766–73. doi: 10.1016/j.cgh.2018.07.029
168. Wolf E, Rich NE, Marrero JA, Parikh ND, Singal AG. Use of hepatocellular carcinoma surveillance in patients with cirrhosis: A systematic review and meta-analysis. Hepatology (2021) 73(2):713–25. doi: 10.1002/hep.31309
169. Tsai PC, Kuo HT, Hung CH, Tseng KC, Lai HC, Peng CY, et al. Metformin reduces hepatocellular carcinoma incidence after successful antiviral therapy in patients with diabetes and chronic hepatitis C in Taiwan. J Hepatol (2023) 78(2):281–92. doi: 10.1016/j.jhep.2022.09.019
170. Hosui A, Tanimoto T, Okahara T, Ashida M, Ohnishi K, Wakahara Y, et al. Oral zinc supplementation decreases the risk of HCC development in patients with HCV eradicated by DAA. Hepatol Commun (2021) 5:2001–8. doi: 10.1002/hep4.1782
171. Costello LC, Franklin RB. The status of zinc in the development of hepatocellular cancer: an important, but neglected, clinically established relationship. Cancer Biol Ther (2014) 15(4):353–60. doi: 10.4161/cbt.27633
172. Takahashi M, Saito H, Higashimoto M, Hibi T. Possible inhibitory effect of oral zinc supplementation on hepatic fibrosis through downregulation of TIMP-1: A pilot study. Hepatol Res (2007) 37(6):405–9. doi: 10.1111/j.1872-034X.2007.00065.x
Keywords: HCC, cACLD, DAA, HCV, predictors, screening
Citation: Elbahrawy A, Atalla H, Mahmoud AA, Eliwa A, Alsawak A, Alboraie M, Madian A, Alashker A, Mostafa S, Alwassief A and Aly HH (2023) Prediction and surveillance of de novo HCC in patients with compensated advanced chronic liver disease after hepatitis C virus eradication with direct antiviral agents. Front. Virol. 3:1227317. doi: 10.3389/fviro.2023.1227317
Received: 23 May 2023; Accepted: 09 October 2023;
Published: 24 October 2023.
Edited by:
Akio Adachi, Tokushima University, JapanReviewed by:
Pirjo Spuul, Tallinn University of Technology, EstoniaGiuseppe Mazzella, University of Bologna, Italy
Mauro Viganò, University of Milan, Italy
Copyright © 2023 Elbahrawy, Atalla, Mahmoud, Eliwa, Alsawak, Alboraie, Madian, Alashker, Mostafa, Alwassief and Aly. This is an open-access article distributed under the terms of the Creative Commons Attribution License (CC BY). The use, distribution or reproduction in other forums is permitted, provided the original author(s) and the copyright owner(s) are credited and that the original publication in this journal is cited, in accordance with accepted academic practice. No use, distribution or reproduction is permitted which does not comply with these terms.
*Correspondence: Hussein H. Aly, YWh1c3NlaW5AbmloLmdvLmpw