- 1Department of Clinical Sciences Lund, Orthopaedics, Faculty of Medicine, Lund University, Lund, Sweden
- 2Department of Clinical Sciences Lund, Infection Medicine, Faculty of Medicine, Lund University, Lund, Sweden
Knee injury increases the risk of developing knee osteoarthritis (OA). Recent evidence suggests involvement of oxidative stress induced by inflammation and bleeding in the joint. This study investigates the role in this process of α1-microglobulin (A1M), a plasma and tissue antioxidant protein with reducing function, and heme- and radical-binding properties. We studied matched knee synovial fluid (sf) and serum (s) samples from 122 subjects (mean age 40 years, 31% females): 10 were knee healthy references, 13 had acute inflammatory arthritis (AIA), 79 knee injury 0–10 years prior to sampling, and 20 knee OA. Using immunoassays, we measured sf-A1M and s-A1M, sf-hemoglobin (sf-Hb), sf-total free heme (sf-Heme), and sf-carbonyl groups (sf-Carbonyl). We explored associations by partial correlation, or linear regression models with adjustments for age, sex and diagnosis, and evaluated diagnostic capacity by area under the receiver operator characteristics curve (AUC). The AIA group had 1.2- to 1.7-fold higher sf-A1M and s-A1M concentrations compared to the other diagnostic groups; other biomarkers showed no between-group differences. sf-A1M and s-A1M were with AUC of 0.76 and 0.78, respectively, diagnostic for AIA. In the injury group, the amount of bleeding in the joint was inversely correlated to time after injury when measured as sf-Heme (r = -0.41, p < 0.001), but not when measured as sf-Hb (r = -0.19, p = 0.098). A similar inverse association with time after injury was noted for sf-A1M (r = -0.30, p = 0.007), but not for s-A1M and sf-Carbonyl. Linear regression models showed that sf-Heme was more strongly associated with sf-A1M and sf-Carbonyl than sf-Hb. Independent of diagnosis, sf-Heme explained 5.7% of the variability in sf-A1M and 3.0% in the variability in sf-Carbonyl, but appeared unrelated to s-A1M. High sf-A1M and low sf-Heme or sf-Hb were independently associated with low sf-Carbonyl. In conclusion, our results demonstrate that independent of disease, Hb and heme within a knee joint correlates with an increased sf-A1M concentration that appears to be protective of oxidative damage, i.e., a reduction in carbonyl groups. High concentrations of A1M in synovial fluid and serum was further diagnostic for AIA.
Introduction
Knee osteoarthritis (OA) is the most common joint disease and is a major cause of pain and disability in the older population worldwide (Liu et al., 2015). It is a complex and multifactorial disorder that represents a pathological imbalance of degenerative and regenerative processes involving the whole joint (Loeser et al., 2012). Obesity, genetic factors, and aging are some of the most prominent risk factors (Sharma et al., 2006), and in the younger population, previous knee injury is an important contributor to increased prevalence of knee OA – as much as half of those sustaining a knee injury in their 20s had developed post-traumatic knee OA in the following 12 to 14 years (Lohmander et al., 2004, 2007; von Porat et al., 2004).
Lately, much evidence has emerged to support a central role for participation of local inflammatory pathways in the disease processes (Scanzello, 2017), particularly so after knee injury (Goldring and Otero, 2011). As a part of the inflammatory response after injury, oxidative stress due to formation of reactive oxygen species (ROS) is involved both in direct damage of cartilage components and as integral factors in cell signaling leading to cartilage degradation (Henrotin et al., 2003). In addition to inflammation, cell-free hemoglobin (Hb) released via hemolysis is a potent inducer of oxidative stress. Spontaneous decomposition of Hb via auto-oxidation leads to the formation of ROS, free heme groups and free iron, which are highly reactive and have the ability to damage lipids, proteins, and DNA (Olsson et al., 2012).
Protection from Hb and heme toxicity exists on several levels, both by mechanisms removing cell-free Hb and heme, and by a complex network of antioxidant mechanisms that inhibit and eliminate the oxidative compounds and repair the oxidative damage caused. On the extracellular level, within the circulation, haptoglobin (Hp) and hemopexin (Hpx) are two of the most prominent scavenger proteins, with antioxidative properties through their capacity to remove cell-free Hb (by Hp) and heme (by Hpx). A major intracellular antioxidant is heme oxygenase-1 (HO-1) which, through its heme-degrading activity, plays a critical role in the protection of cells. However, during disease and damage these antioxidant defense systems are not sufficient to protect against harmful reactive compounds, damage to DNA, lipids and proteins arises. Oxidation of proteins result in stable carbonyl groups on amino acid side chains, and protein carbonyl content is one of the most widely used marker of protein oxidation and a general indicator of oxidative stress (Dalle-Donne et al., 2003).
Another protein that in the last decade has been shown to play a central role as a protective protein against oxidative stress is α1-microglobulin (A1M) (Åkerström and Gram, 2014). It is a 26 kDa plasma and tissue protein with the capacity to bind free radicals and heme groups, and the capacity to repair oxidative damage by chemical reduction. A1M is mainly produced in the liver, and a rapid flow of A1M through the blood and tissues binds harmful molecules and delivers them to the kidneys where they are degraded or excreted in the urine. In addition to the liver, smaller quantities are also expressed in most other cells in the body (Åkerström and Gram, 2014). Of particular interest for this investigation is that the expression of A1M has been shown to be up-regulated in several cells and tissues during oxidative stress conditions in response to elevated levels of ROS, Hb, and free heme (Olsson et al., 2007, 2011).
The main objective of this work was to study the role of A1M and bleeding-induced oxidative stress in the development of osteoarthritis after knee injury. Using a cross-sectional knee arthropathy cohort with matched serum and synovial fluid samples, we measured A1M and protein carbonyl groups as biomarkers of oxidative stress and Hb and free heme as biomarkers of bleeding, hypothesizing that increased A1M buffers against oxidative damage in synovial fluid.
Materials and Methods
Subjects and Samples
From a convenience cohort of previously studied individuals (Lohmander et al., 1989, 1999; Larsson et al., 2009; Kumahashi et al., 2015), we selected 122 subjects with matched knee synovial fluid and serum samples that were diagnosed as knee healthy references, having acute inflammatory arthritis (AIA) in the knee, knee injury (anterior cruciate ligament tear and/or meniscus injury), or knee osteoarthritis (Table 1). Diagnosis was made by arthroscopy, radiography, assessment of synovial fluid and clinical examination (Lohmander et al., 1989). Serum was prepared from whole blood that had been allowed to clot at room temperature (RT), with the clot removed by centrifuging at 1800 g for 10 min. Synovial fluid samples were aspirated without lavage, centrifuged at 3000 g for 10 min. Aliquots of serum and synovial fluid supernatants were stored at -80°C. All patient-related procedures were approved by the ethics review committee of the Medical Faculty, Lund University.
α1-Microglobulin (A1M)-Concentrations
We used a radioimmunoassay (RIA) developed in-house for the detection of A1M in serum (s-A1M) and synovial fluid (sf-A1M) (Gram et al., 2015). Radiolabeling of A1M with 125I (Perkin Elmer Life Sciences) was done using the chloramine T method (Greenwood et al., 1963). Protein-bound iodine was separated from free iodide by gel-chromatography on a Sephadex G-25 column (PD10, GE Healthcare, Stockholm, Sweden). A specific activity of around 0.1–0.2 MBq/μg protein was obtained. The RIA was performed as described (Plesner et al., 1975). Briefly, goat antiserum against human A1M (diluted 1:6000) was mixed with 125I-labeled A1M (approximately 0.05 pg/ml) and unknown patient samples (serum, diluted 400x; synovial fluid, diluted 100x) or calibrator A1M-concentrations. After incubating overnight at RT, antibody-bound antigen was precipitated by adding bovine serum and 15% polyethylene glycol, centrifuged at 1600 g for 40 min, after which the 125I activity of the pellets was measured in a Wallac Wizard 1470 gamma counter (Perkin Elmer Life Sciences).
Total Hemoglobin (Hb-Total) Concentrations
To determine the concentration of total Hb in synovial fluid (sf-Hb) we used a Human Hb ELISA Quantification Kit from Genway Biotech, Inc. (San Diego, CA, United States) according to the manufacturer’s instructions. Samples were analyzed diluted 1:2000, at which the lowest detection limit for samples was 12.5 μg/ml. Absorbance was read at 450 nm using a Wallac 1420 Multilabel Counter.
Heme Concentrations
To determine the concentration of heme in synovial fluid (sf-Heme) we used QuantiChromTM Heme Assay kit (Gentaur BVBA, Brussels, Belgium; cat. no. DIHM-250) following instructions in the kit and diluting samples 10x with PBS.
Protein Carbonyl Groups
Determination of the concentration of protein carbonyl groups in synovial fluid (sf-Carbonyl) was done by an ELISA, modified from the original description by Buss et al. (1997). Briefly, samples were diluted 4x in 6 M guanidine-HCl, 0.5 M K3PO4, pH 2.5, containing 10 mM DNP-hydrazine, incubated at RT for 45 min, mixed 1:200 with PBS and coated overnight in 96-well microtiter plates at 4°C. After washing and blocking for 1 h at RT with 0.1% reduced bovine serum albumin (BSA) in PBS, the plates were incubated with rabbit anti-DNP (Invitrogen, diluted 1000x) in PBS, 0.1% reduced BSA, 0.25% Tween-20 for 1 h at RT, washed and incubated 1 h at RT with horseradish peroxidase-conjugated porcine anti-rabbit IgG (Dako, diluted 2000x) in the same buffer. The wells were finally washed and developed by addition of a ready-to-use 3,3′,5,5′-tetramethylbenzidine substrate solution (TMB, Life Technologies, Stockholm, Sweden), stopping the reaction after 5 min by addition of 1 M HCl. The absorbance was read at 450 nm using a Wallac 1420 Multilabel Counter (Perkin Elmer Life Sciences, Waltham, MA, United States). Reduced BSA for blocking and incubation buffers, and oxidized BSA for standard carbonyl groups, were prepared as described (Buss et al., 1997). Measured concentrations are described as absorbance at 450 nm divided by total protein content of the sample (abs./tot. prot.). Total protein content in synovial fluid (diluted 10x) was determined with PierceTM BCA Protein Assay Kit (ThermoFisher Scientific, Inc.).
Statistical Analysis
Synovial fluid samples with Hb concentrations below the lower limit of detection (12.5 μg/ml) were given the concentration 12.5 μg/ml. We created the ratio of A1M concentrations in synovial fluid to serum (A1M ratio sf/s) for evaluation of the relative influence of local versus systemic concentrations of A1M. Normal distribution was evaluated by visual inspection of histograms, normal q–q plots and box plots. Student’s t-test was used to compare A1M concentrations between sexes. For comparisons of biomarker concentrations between diagnostic groups we used univariate ANCOVA adjusted for age and sex with evaluation of residuals in histograms and normal p–p plots. Adjustments were included since groups were heterogeneous for these variables, and s-A1M was shown to increase with increasing age (Takagi et al., 1980), and to be higher in males compared to females (Takagi et al., 1980; Itoh and Kawai, 1990). For the same reason, we adjusted for age and sex in partial correlation analysis between biomarkers and time after injury (where longer time after injury was associated with higher age, r = 0.357, p = 0.001). Age, sex, and diagnosis was adjusted for in (a) linear regression models exploring joint bleeding (sf-Hb or sf-Heme) as explanatory factors for other biomarkers, and (b) linear regression models exploring the interaction of joint bleeding (sf-Hb or sf-Heme) and sf-A1M as explanatory factors for oxidative damage in the joint (sf-Carbonyl). In the linear regression models, sf-Heme, sf-Hb, sf-Carbonyl and the interaction variables ratio sf-A1M/sf-Heme and ratio sf-A1M/sf-Hb were log10 transformed to get linear relationships between variables, with evaluation of residuals in histograms and normal p–p plots.
In receiver operator characteristics (ROCs) analyses we evaluated A1M concentrations as a diagnostic tool for correctly diagnosing subjects with AIA. Evaluations were based on the area under the ROC curve (AUC) with cut-offs chosen to maximize the sum of the sensitivity and specificity.
We used SPSS version 24 for all statistical calculations, and made no adjustment for multiple comparisons due to the exploratory nature of the study.
Results
α1-Microglobulin by Diagnosis
α1-Microglobulin concentrations were normally distributed within the diagnostic groups, and were overall 2.6 times higher in serum compared to synovial fluid (Figure 1A), without difference between males and females (p = 0.80 and 0.62 for sf-A1M and s-A1M, respectively). The highest concentrations of A1M, both in synovial fluid and serum, were found in the AIA (Figure 1A). The between-group-differences were largest in synovial fluid, where the mean A1M concentration in the AIA group was 1.5-fold higher compared to the OA and knee injury groups (p = 0.005 and 0.045, respectively), while the 1.7-fold difference in means between AIA and the reference group failed to reach statistical significance (p = 0.068). In serum, the A1M concentration in the AIA group was between 1.3- and 1.4-fold higher compared to the injury and osteoarthritis groups (p = 0.035 and 0.030, respectively), whereas the 1.2-fold higher mean concentration compared to the reference group was not statistically significant (p = 0.15; Figure 1A).
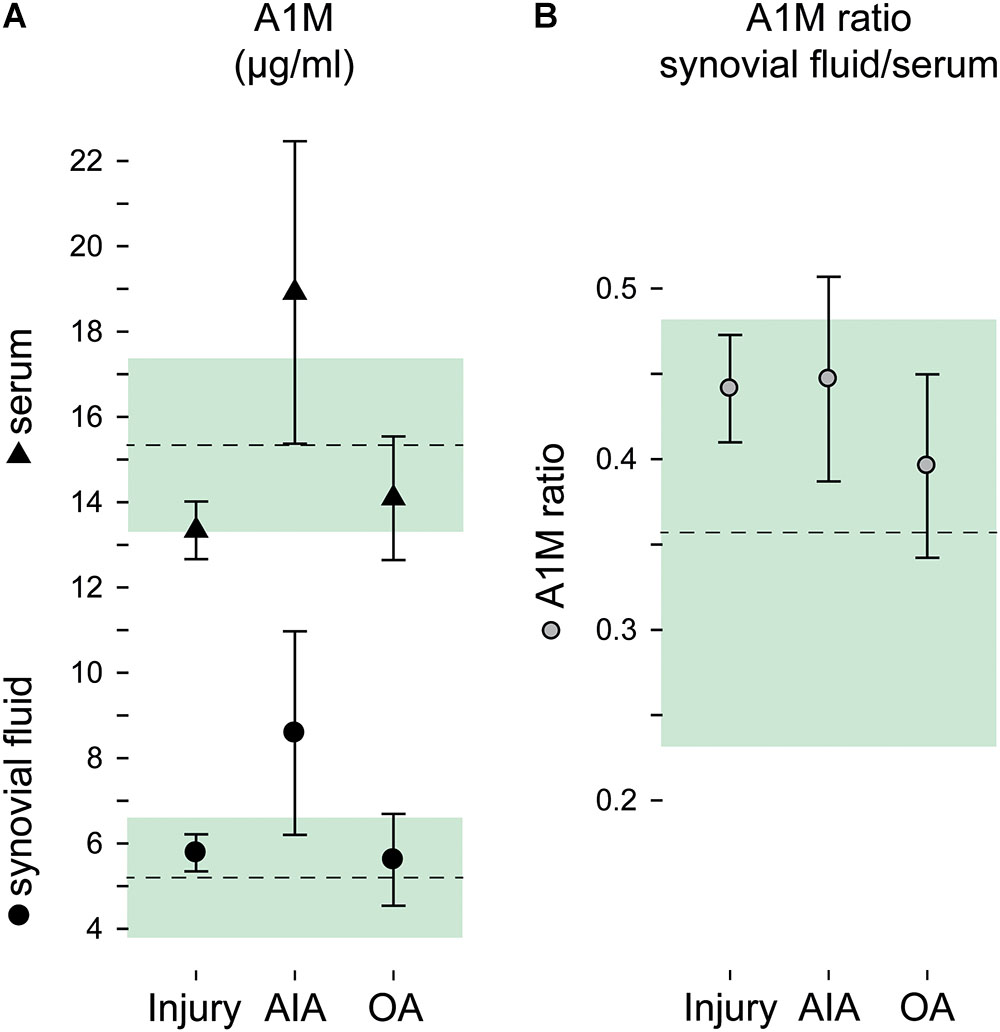
FIGURE 1. A1M by diagnostic groups. Concentrations of A1M in (A) synovial fluid and serum and (B) as a ratio in concentrations synovial fluid to serum. Group means and 95% confidence intervals are plotted as circles and triangles with error bars for the injury, acute inflammatory arthritis (AIA) and osteoarthritis (OA) groups, and as a dashed line and green area for the reference group. (Plotted data and statistics are reported in full in Supplementary Table S1).
The A1M ratio in synovial fluid to serum ranged between 0.36 and 0.45 in the four diagnostic groups, without statistically significant differences between groups (p = 0.36; Figure 1B).
Since the AIA group was standing out as the only group with increased A1M levels in synovial fluid as well as in serum, we performed a ROC curve analysis for having AIA using A1M in both fluids as the diagnostic tool. We found that with similar AUC close to 0.8, sf-A1M had higher specificity but lower sensitivity than s-A1M (Table 2).
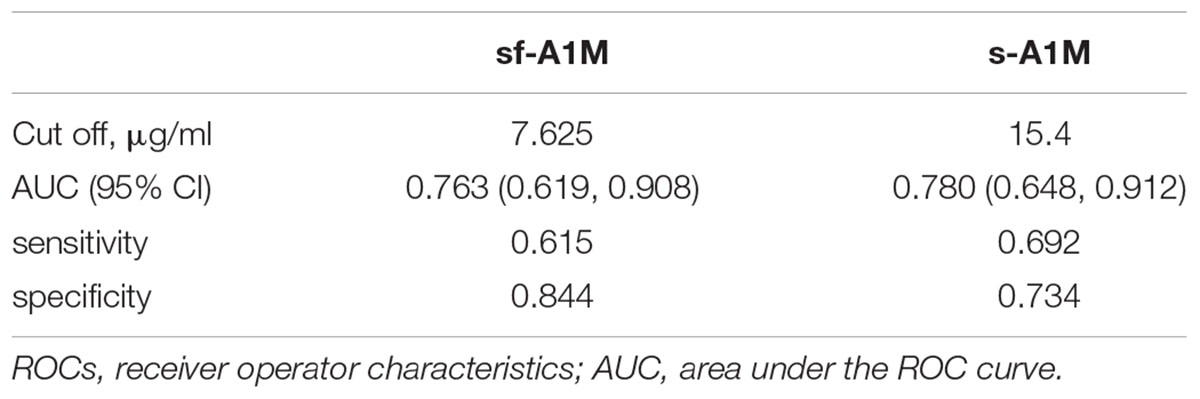
TABLE 2. Receiver operator characteristic (ROC) curve analysis of sf-A1M and s-A1M as diagnostic for having acute inflammatory arthritis.
Markers of Bleeding and Oxidative Damage
Fifty-three out of 122 synovial fluid samples (43%) had sf-Hb concentrations below the lower limit of detection. Synovial fluid concentrations of Hb, total heme, and protein carbonyl groups were skewed, but were approximately normally distributed after log10 transformation. Intra-articular bleeding was more evident in the injury group, especially when measured as total heme (Figures 2A,B), but no statistically significant between-group differences were noted (p = 0.58 and 0.90 for sf-Heme and sf-Hb, respectively).
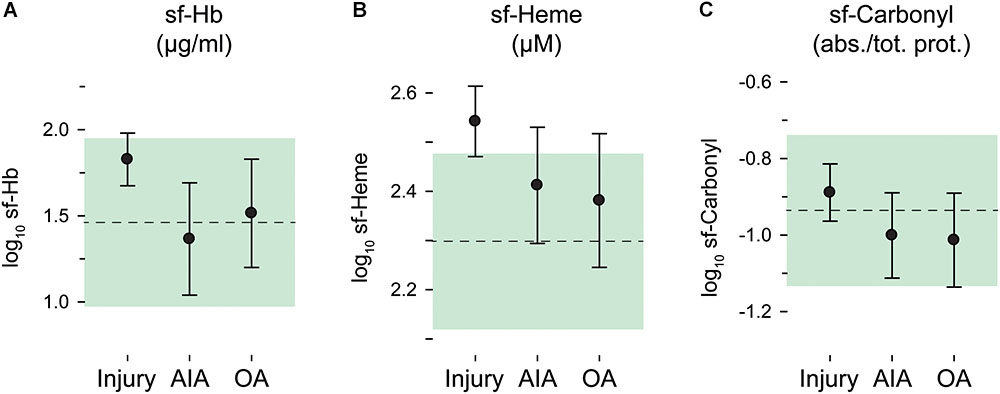
FIGURE 2. Oxidative stress in synovial fluid by diagnostic groups. Log10 transformed concentrations in synovial fluid (sf) of (A) hemoglobin (Hb), (B) total heme, and (C) carbonyl groups. Group means and 95% confidence intervals are plotted as circles with error bars for the injury, AIA and osteoarthritis (OA) groups, and as a dashed line and green area for the reference group. sf-Carbonyl values are given relative to total protein concentration. (Plotted data and statistics are reported in full in Supplementary Table S1).
Oxidative damage in the joints, measured as protein carbonyl groups in synovial fluid, was highly similar and without statistically significant differences between diagnostic groups (p = 0.49) (Figure 2C).
Temporal Variation in Biomarkers After Injury
In the injury group, the levels of the biomarkers of bleeding, Hb and heme, in the joint was highest early after injury, and longer times after injury were associated with less bleeding (Figure 3A). This inverse relationship was strongest when measured as sf-Heme, for which we (after adjusting for age and sex) observed a statistically significant inverse correlation with time after injury (r = -0.46), and less accentuated, and not statistically significant, when measured as sf-Hb (r = -0.19) (Figure 3A). A similar temporal pattern and inverse association with time after injury was noted for sf-A1M (r = -0.30) and for the ratio of sf-A1M/s-A1M (r = -0.47) but not for s-A1M and sf-Carbonyl (Figures 3B,C).
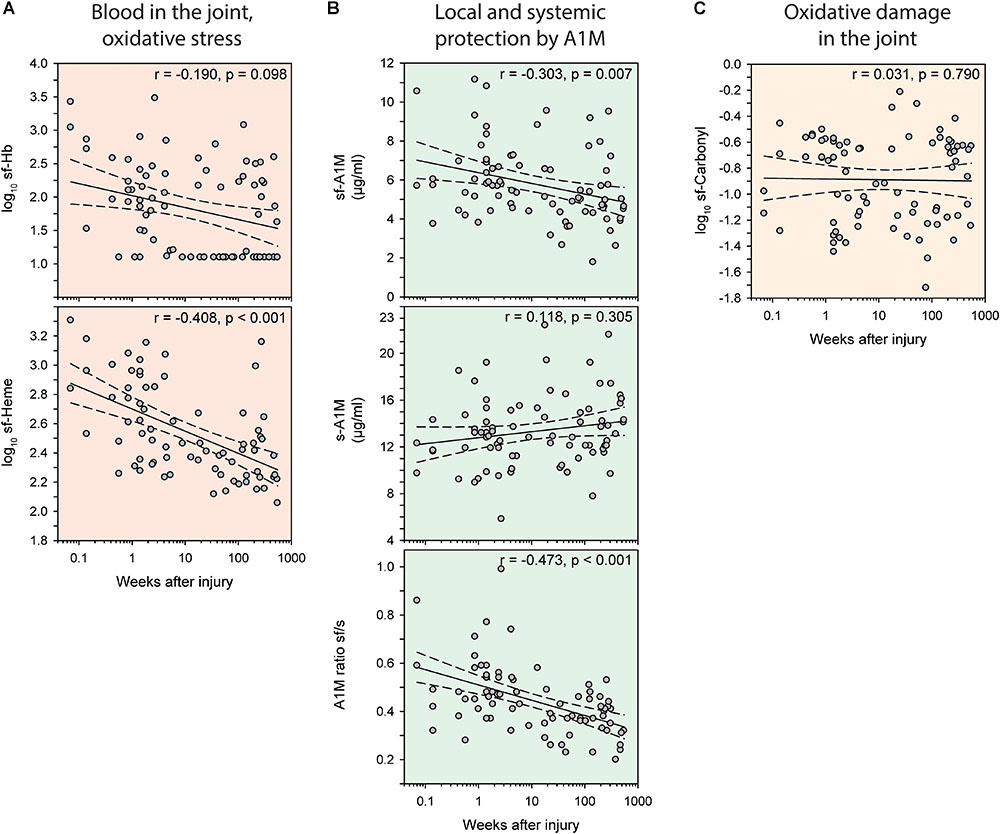
FIGURE 3. Temporal variations in biomarkers of oxidative stress after knee injury. Bivariate scatterplots of biomarker concentrations against weeks after injury with regression lines (solid) and 95% confidence intervals of the regression lines (dashed). (A, red) Blood in the joint measured as hemoglobin (Hb) or total heme in synovial fluid (sf). (B, green) Concentrations of A1M in synovial fluid and serum, and the ratio of A1M in synovial fluid to serum. (C, yellow) Oxidative damage measured as protein carbonyl groups in synovial fluid. Partial correlation coefficients (r) indicate correlations adjusted for age and sex.
Bleeding in the Joint as Explanatory Factor for Concentrations of A1M and Protein Carbonyl Groups Independent of Diagnosis
In hierarchical linear regression models including all 122 subjects, we examined how bleeding in the joint, independent of diagnosis, affected biomarker response after adjusting for age, sex and diagnostic group. This analysis showed that sf-Heme had a greater influence than sf-Hb on biomarkers of oxidative stress, since sf-Heme concentrations were associated with statistically significant effects on sf-A1M, the A1M ratio sf/s and sf-Carbonyl, whereas sf-Hb was only associated with an effect on the A1M ratio sf/s (Table 3). All associations had positive regression coefficients, i.e., higher concentrations of sf-Heme were associated with higher concentrations of sf-A1M, A1M ratios sf/s and sf-Carbonyl, and higher concentrations of sf-Hb were associated with higher A1M ratios sf/s (Table 3).
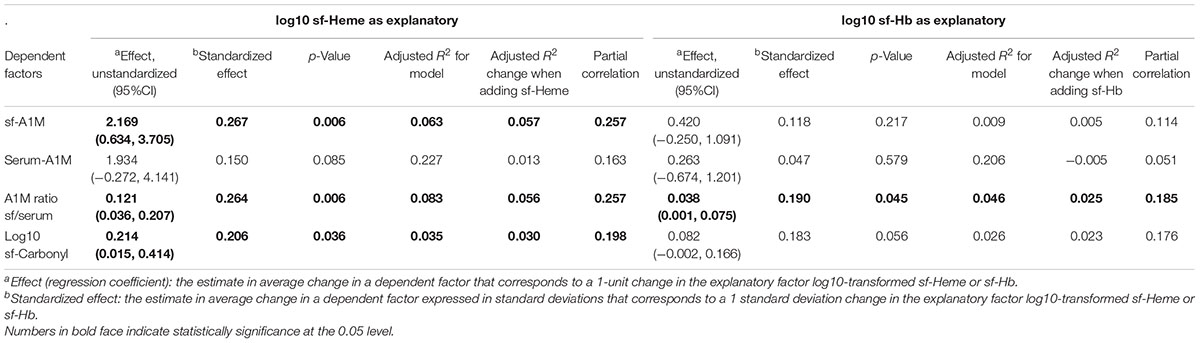
TABLE 3. Hierarchical linear regression models exploring joint bleeding as explanatory factors for other oxidative stress biomarkers in all 122 subjects with Heme or Hb entered last after age, sex, and diagnostic group.
The relative influence of sf-Heme and sf-Hb on sf-A1M, A1M ratio sf/s and sf-Carbonyl can be evaluated by comparing the standardized effect sizes, partial correlations and R2 changes in the models. These comparisons show that sf-Heme had the largest influence on sf-A1M and A1M ratio sf/s (with standardized effects and partial correlation coefficients of approximately 0.26), explaining 5.7 and 5.6% of the variability in sf-A1M and A1M ratio sf/s, respectively (R2 change = 0.057 and 0.056) (Table 3). The relative influence of sf-Heme on sf-Carbonyl was slightly lower (standardized effect size and partial correlation both close to 0.20), where the R2 change indicated that sf-Heme explained 3.5% of the variability of sf-Carbonyl in the model (Table 3). The lowest relative influence was noted for sf-Hb, which explained 2.5% of the variability of the A1M ratio sf/s (R2 change = 0.025, with standardized effect size and partial correlation below 0.2), without statistically significant influence on the individual concentrations of sf-A1M or s-A1M (Table 3).
Interaction Between A1M and Hb or Heme as Explanatory Factors for Oxidative Damage Measured as Protein Carbonyl Groups Independent of Diagnosis
To investigate the combined effect of intra-articular bleeding and A1M on oxidative damage in the joint, we created two interaction variables, ratio sf-A1M/sf-Heme and ratio sf-A1M/sf-Hb. In two linear regression models, using heme as proxy for bleeding in model 1 and Hb in model 2, we then (in two steps, or blocks) explored the relationship between sf-Carbonyl and bleeding and sf-A1M alone (block 1) or in combination with the two interaction variables (block 2). Results in both models show that, when entered without an interaction variable, higher sf-Heme or sf-Hb were independently associated with higher oxidative damage measured as sf-Carbonyl (statistically significant positive effects), and higher sf-A1M was independently associated with lower sf-Carbonyl (statistically significant negative effects) (blocks 1, Table 4). Although different in direction, the relative influence of bleeding in the joint and sf-A1M on sf-Carbonyl was comparable in size (as judged by similarities in the magnitude of the standardized effects sizes and partial correlation coefficients in block 1 in both models) (Table 4). The model including sf-Heme and sf-A1M without an interaction variable explained 9.4% in the variability in sf-Carbonyl (adjusted R2 = 0.094) and the model including sf-Hb and sf-A1M explained 6.5% (Table 4).
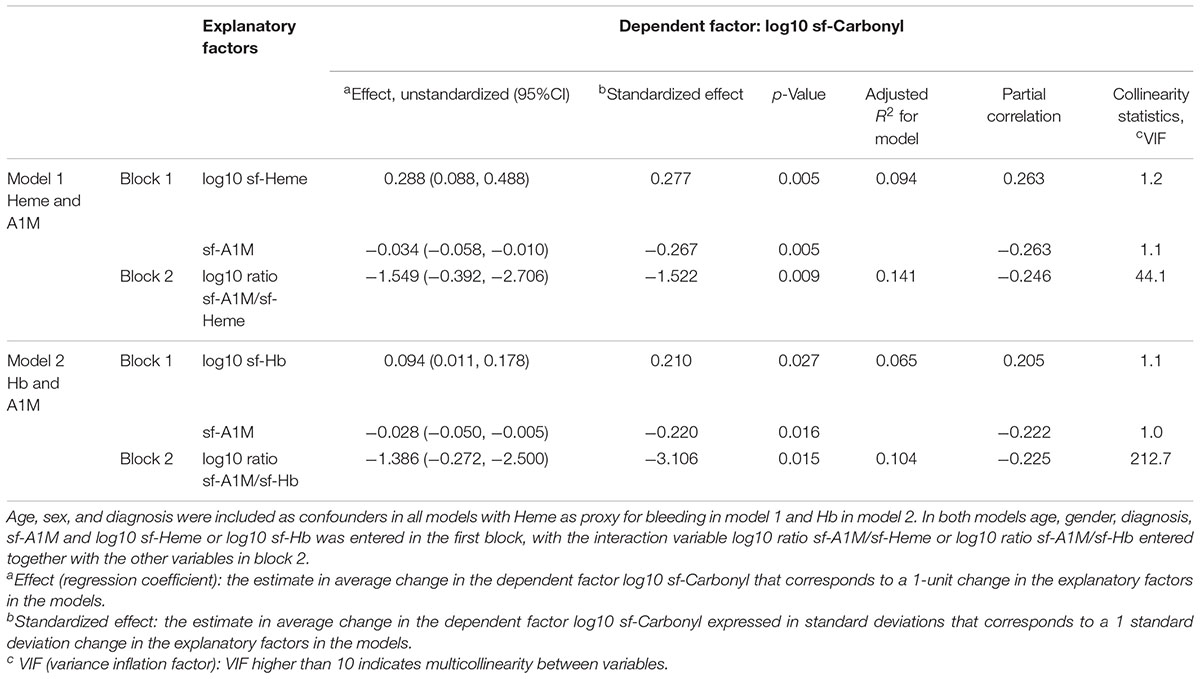
TABLE 4. Linear regression models exploring the interaction of joint bleeding and A1M as explanatory factors for oxidative damage in the joint in all 122 subjects.
Adding the interaction variables (in block 2 of both models) increased the power of the models to explain variability in sf-Carbonyl from 9.4 to 14.1% when adding the ratio sf-A1M/sf-Heme, and from 6.5 to 10.4% when adding the ratio sf-A1M/s-Hb (Table 4). Based on the statically significant negative regression coefficients (unstandardized and standardized effects) (Table 4), higher ratios sf-A1M/sf-Heme or sf-A1M/sf-Hb were independently associated with lower concentrations of sf-Carbonyl.
When adding the interaction variables in block 2, multicollinearity between variables and their ratios was substantial in both models, as indicated by variance inflation factors (VIF) far beyond 10 (Table 4). This influenced the magnitudes of the effect sizes, making comparisons of effect sizes with and without the interaction variable present difficult. However, partial correlation coefficients for the interaction variables (ratios) and the individual concentrations were of the same magnitude (ranging between 0.20 and 0.26) (Table 4). This indicates that the relative influence on sf-Carbonyl of the ratio between sf-A1M and sf-Heme or sf-Hb is similar to that of the individual concentrations of sf-A1M, sf-Heme and sf-Hb.
Discussion
This exploratory cohort study of oxidative stress in joint disease reveals a complex interplay between pro- and antioxidative molecular markers in knee injury, inflammation, or osteoarthritis. The study focuses on antioxidative reactions and protection from bleeding in the knee joint, and its main finding is that—without disease dependency—bleeding in the knee joint was associated with increased oxidative damage (in the form of higher concentrations of protein carbonyl groups in synovial fluid), as well as with higher concentrations of the antioxidative protein A1M. In addition, we saw that the net amount of oxidative damage was dependent not only on the absolute concentrations of Hb, heme or A1M, but that the ratios between pro- and antioxidative molecules were as important as their absolute concentrations. When adjusted for their respective absolute concentrations, the higher the proportion sf-A1M to sf-heme or sf-Hb, the less oxidative damage measured as protein carbonyl groups in synovial fluid. These novel data support a role for A1M in the protection against oxidative damage due to extracellular Hb and heme in the joint in various knee arthropathies.
The diagnostic group for which these findings may be of greatest value is the injury group, which had the highest synovial fluid concentrations of extracellular Hb and heme. For this group, we noted that the time-related pattern of cell-free Hb and heme in synovial fluid after injury was mimicked by a similar pattern of A1M in synovial fluid, whereas serum A1M and synovial fluid carbonyl groups appeared as unaffected by time after injury. Altogether, our best interpretation of these data is that increased levels of cell-free Hb and heme in synovial fluid early after injury triggered an increase in the synovial fluid A1M concentration that appeared to be protective of oxidative damage.
The ratio of A1M in synovial fluid to serum followed a similar temporal pattern after injury and was more strongly associated with time after injury than the synovial fluid concentration by itself. This provides support that the reaction to knee injury and/or cell-free Hb and heme is localized to the injured knee, rather than being a systemic response. Such a local response may be the result of an upregulated local production of A1M, similar to previous reports of an upregulation of the protein in liver, skin, placenta, retina, and blood cells upon oxidative stimulation (Olsson et al., 2007, 2011; Cederlund et al., 2013). However, it cannot be excluded that an increased influx of systemic A1M also contributes to the synovial accumulation of A1M, since (i) the overall concentration of A1M in serum is three times higher compared to synovial fluid and (ii) reports have shown that the permeability of the synovial membrane and its blood vessels of small molecules like A1M, is increased in the early phases after joint injury and by hemorrhage (Soren et al., 1978; Hettinga, 1979).
There are many examples of studies that have shown increased A1M in serum or urine to be a biomarker of diseases such as preeclampsia (Anderson et al., 2011, 2016; Gram et al., 2015), and kidney failure (Terzi et al., 2014; Kang et al., 2015). Our study extends those findings to include elevated A1M in both serum and synovial fluid as a biomarker of AIA, as suggested by the ROC-curves with AUCs close to 0.8. It has previously been speculated that the plasma concentration of A1M does not change during inflammation unless it is secondary to disorders affecting the liver and kidney functions in humans (Itoh and Kawai, 1990), or rats (Falkenberg et al., 1997). Although we have no record of illnesses secondary to the AIA that the subjects of our study were seeking health care for, and therefore cannot rule out involvement of kidney or liver disease in some of the subjects, we find it unlikely that illnesses secondary to the AIA would explain the elevations of A1M seen in these subjects at group level in both serum and synovial fluid.
Studies on A1M in synovial fluid are rare. The only two reports we find are proteomic studies of subjects with knee OA (Gobezie et al., 2007; Sohn et al., 2012). One of those studies reported presence of A1M without comparison to other diseases or references (Sohn et al., 2012), the other found A1M to be upregulated in synovial fluid in subjects with OA compared to normal synovial fluid (Gobezie et al., 2007). In similarity with both studies, we found detectable concentrations of A1M in synovial fluid in subjects with knee OA. However, we did not see different concentrations between healthy and osteoarthritic subjects as was reported by Gobezie et al. (2007), which may be due to differences in methodology (mass spectrometry versus RIA), in the selection of patients, or in the power between the studies.
Expression and synthesis of A1M has been shown to be upregulated in cells after exposure to heme and ROS (Olsson et al., 2007, 2011). This is consistent with our findings here that both heme and Hb were associated with increased levels of synovial fluid A1M. Thus, we may speculate that local production of A1M in the knee joint is induced by synovial fluid free Hb or heme. However, with A1M increased in both synovial fluid and serum in AIA, the present study further suggests that, in addition to heme and ROS, A1M may be regulated by inflammatory cytokines. In further corroboration for an inflammatory link, we note that the temporal pattern of synovial fluid A1M seen after injury in this cross-section study, is highly similar to findings in a longitudinal study of ACL injured subjects, in which synovial fluid levels of inflammatory cytokines were initially elevated with subsequent decreasing levels over 5 years (Struglics et al., 2015; Larsson et al., 2017). This type of regulation by cytokines has been proposed for another major scavenger of ROS, extracellular superoxide dismutase (EC-SOD or SOD3) (Strålin and Marklund, 1994, 2000).
α1-Microglobulin has been proposed as a therapeutic or diagnostic tool for several different conditions and diseases. The focus has so far mainly been on two areas of application: diagnosis and treatment of preeclampsia (Gunnarsson et al., 2017), and kidney protection in radiation therapy (Ahlstedt et al., 2015). The present study suggests that treatment with A1M following knee injury may prevent development of OA. In addition to the obvious protective benefit of exogenous A1M binding and neutralizing cell-free Hb and heme, it may also be beneficial as a repair mechanism in already damaged cartilage, since in vitro studies showed that collagen fibrils were restored to normal by addition of recombinant A1M after destruction already had begun (Olsson et al., 2011).
One of the novelties and strengths of this study—that we studied biomarkers of oxidative stress in matched samples of serum and synovial fluid—also proved to be a limitation, since it drastically reduced the number of available subjects to include from our convenience cohort of subjects with various knee-related illnesses. This may have increased the risk for spurious results, and reduced the likelihood of finding statistically significant differences between diagnostic groups. The study was further limited by its cross-sectional design, which reduced the possibility to draw firm conclusions on temporal change after injury since no repeated sampling was made within individual subjects. Lack of information on secondary or concomitant illnesses that may have influenced our results represents a further limitation. Finally, the presence of imputed values for sf-Hb may have influenced and/or concealed associations with time after injury, or with other biomarkers.
Conclusion
Our results suggest (as summarized in Figure 4) that independent of disease, cell-free Hb and heme within a knee joint triggers an increased synovial concentration of A1M that appears to be protective of oxidative damage. We further conclude that the net amount of oxidative damage depends not only on the absolute concentrations of oxidative Hb and heme, or antioxidative A1M, but that the ratios between pro- and antioxidative molecules appears as important. Finally, we note that in AIA A1M is increased both locally in the joint fluid and systemically in serum, whereas in knee injury A1M is increased only in the joint fluid.
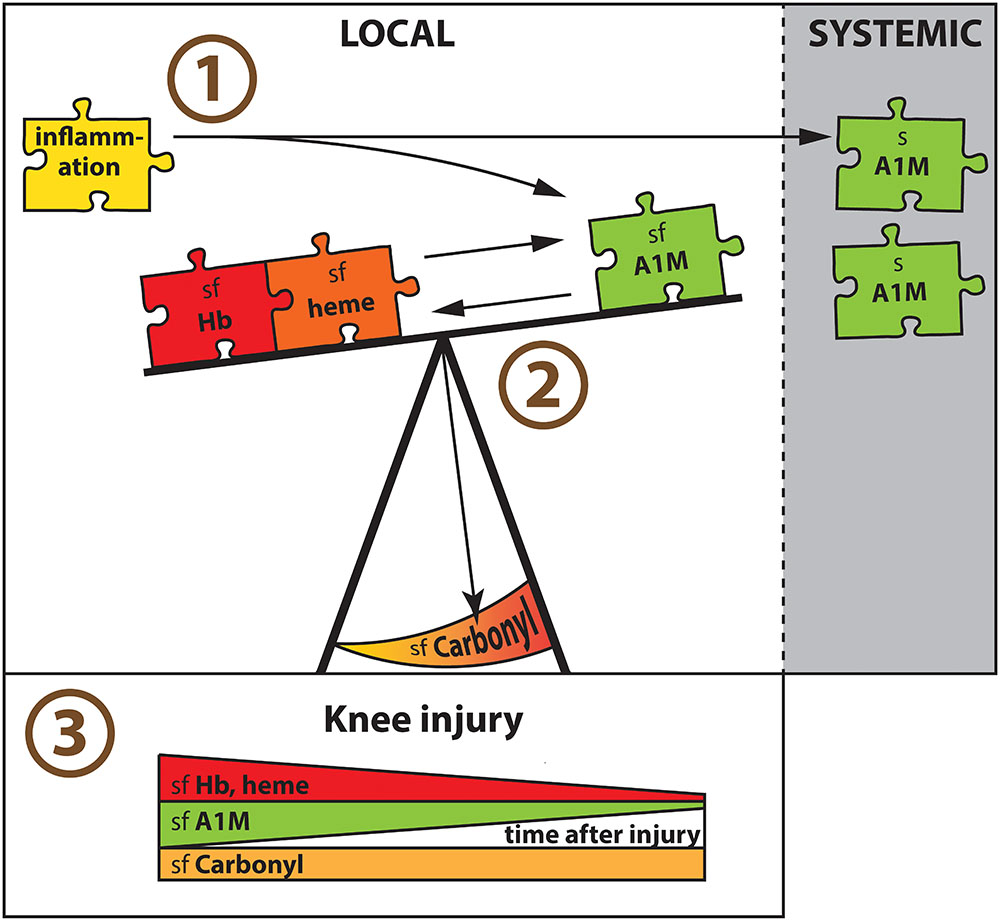
FIGURE 4. Major findings in summary. (1) Having AIA in a knee joint was associated with increased A1M concentrations locally in synovial fluid (sf) and systemically in serum (s). With area under the ROC curve of close to 0.8, A1M was a biomarker for AIA in both synovial fluid and serum. (2) Independent of disease, cell-free Hb and heme within a knee joint triggered an increased synovial concentration of A1M that appeared to be protective of oxidative damage measured as sf-Carbonyl. The net amount of local oxidative damage (sf-Carbonyl) was dependent not only on the absolute concentrations of oxidative Hb and heme, or antioxidative A1M, but the ratio between pro- and antioxidative molecules appeared as important. (3) In knee injured there was a similar temporal pattern in the synovial concentrations of both oxidants (Hb and heme) and antioxidants (A1M), which were highest early after injury, without a corresponding temporal change in oxidative damage (sf-Carbonyl).
Author Contributions
SL drafted the manuscript and full access to all of the data in the study and takes responsibility for the integrity of the data and the accuracy of the data analysis. SL, BÅ, LL, and AS were involved in study conception and design. BÅ and SL in the acquisition of data. All authors were involved in revising it critically for important intellectual content, approved the final version to be published, and the analysis and interpretation of data.
Funding
This work was funded by grants from the Swedish Research Council (VR), Governmental funding of clinical research within the National Health Services (ALF), the Swedish Rheumatism Association, the Anna-Greta Crafoord Foundation, Greta and Johan Kocks Foundations, Olof and Ruth Uppgrens Foundation, the Dagmar Perssons Fund, the Österlunds Foundation, and A1M Pharma AB.
Conflict of Interest Statement
BÅ and MG are co-founders and shareholders of A1M Pharma, Lund, Sweden.
The remaining authors declare that the research was conducted in the absence of any commercial or financial relationships that could be construed as a potential conflict of interest.
Acknowledgments
The authors thank Maria E. Johansson (Lund University) for performing the quantification of Hb, heme and oxidative biomarkers, and Tommy Schyman (biostatistician at Lund University, Skåne University Hospital, Lund) for guidance in the statistical analysis.
Supplementary Material
The Supplementary Material for this article can be found online at: https://www.frontiersin.org/articles/10.3389/fphys.2018.01596/full#supplementary-material
References
Ahlstedt, J., Tran, T. A., Strand, S. E., Gram, M., and Åkerström, B. (2015). Human Anti-Oxidation Protein A1M–A potential kidney protection agent in peptide receptor radionuclide therapy. Int. J. Mol. Sci. 16, 30309–30320. doi: 10.3390/ijms161226234
Åkerström, B., and Gram, M. (2014). A1M, an extravascular tissue cleaning and housekeeping protein. Free Radic. Biol. Med. 74, 274–282. doi: 10.1016/j.freeradbiomed.2014.06.025
Anderson, U. D., Gram, M., Ranstam, J., Thilaganathan, B., Åkerström, B., and Hansson, S. R. (2016). Fetal hemoglobin, alpha1-microglobulin and hemopexin are potential predictive first trimester biomarkers for preeclampsia. Pregnancy Hypertens. 6, 103–109. doi: 10.1016/j.preghy.2016.02.003
Anderson, U. D., Olsson, M. G., Rutardottir, S., Centlow, M., Kristensen, K. H., Isberg, P. E., et al. (2011). Fetal hemoglobin and alpha1-microglobulin as first- and early second-trimester predictive biomarkers for preeclampsia. Am. J. Obstet. Gynecol. 204, e1–e5. doi: 10.1016/j.ajog.2011.01.058
Buss, H., Chan, T. P., Sluis, K. B., Domigan, N. M., and Winterbourn, C. C. (1997). Protein carbonyl measurement by a sensitive ELISA method. Free Radic. Biol. Med. 23, 361–366. doi: 10.1016/S0891-5849(97)00104-4
Cederlund, M., Ghosh, F., Arner, K., Andreasson, S., and Åkerström, B. (2013). Vitreous levels of oxidative stress biomarkers and the radical-scavenger alpha1-microglobulin/A1M in human rhegmatogenous retinal detachment. Graefes Arch. Clin. Exp. Ophthalmol. 251, 725–732. doi: 10.1007/s00417-012-2113-6
Dalle-Donne, I., Rossi, R., Giustarini, D., Milzani, A., and Colombo, R. (2003). Protein carbonyl groups as biomarkers of oxidative stress. Clin. Chim. Acta 329, 23–38. doi: 10.1016/S0009-8981(03)00003-2
Falkenberg, C., Blom, A., Fries, E., Ekström, G., Särnstrand, B., Salier, J. P., et al. (1997). Alpha1-microglobulin and bikunin in rats with collagen II-induced arthritis: plasma levels and liver mRNA content. Scand. J. Immunol. 46, 122–128. doi: 10.1046/j.1365-3083.1997.d01-103.x
Gobezie, R., Kho, A., Krastins, B., Sarracino, D. A., Thornhill, T. S., Chase, M., et al. (2007). High abundance synovial fluid proteome: distinct profiles in health and osteoarthritis. Arthritis Res. Ther. 9:R36. doi: 10.1186/ar2172
Goldring, M. B., and Otero, M. (2011). Inflammation in osteoarthritis. Curr. Opin. Rheumatol. 23, 471–478. doi: 10.1097/BOR.0b013e328349c2b1
Gram, M., Anderson, U. D., Johansson, M. E., Edström-Hägerwall, A., Larsson, I., Jälmby, M., et al. (2015). The human endogenous protection system against cell-free hemoglobin and heme is overwhelmed in preeclampsia and provides potential biomarkers and clinical indicators. PLoS One 10:e0138111. doi: 10.1371/journal.pone.0138111
Greenwood, F. C., Hunter, W. M., and Glover, J. S. (1963). The preparation of I-131-labelled human growth hormone of high specific radioactivity. Biochem. J. 89, 114–123. doi: 10.1042/bj0890114
Gunnarsson, R., Åkerström, B., Hansson, S. R., and Gram, M. (2017). Recombinant alpha-1-microglobulin: a potential treatment for preeclampsia. Drug Discov. Today 22, 736–743. doi: 10.1016/j.drudis.2016.12.005
Henrotin, Y. E., Bruckner, P., and Pujol, J. P. (2003). The role of reactive oxygen species in homeostasis and degradation of cartilage. Osteoarthritis Cartilage 11, 747–755. doi: 10.1016/S1063-4584(03)00150-X
Hettinga, D. L. (1979). I. Normal joint structures and their reaction to injury. J. Orthop. Sports Phys. Ther. 1, 16–22. doi: 10.2519/jospt.1979.1.1.16
Itoh, Y., and Kawai, T. (1990). Human alpha 1-microglobulin: its measurement and clinical significance. J. Clin. Lab. Anal. 4, 376–384. doi: 10.1002/jcla.1860040511
Kang, J., Liu, J., Ding, H., Li, X., Wang, Q., Guo, X., et al. (2015). Urine alpha1-microglobulin is a better marker for early tubular dysfunction than beta2-microglobulin among tenofovir-exposed human immunodeficiency virus-infected men who have sex with men. Braz. J. Infect. Dis. 19, 410–416. doi: 10.1016/j.bjid.2015.05.004
Kumahashi, N., Swärd, P., Larsson, S., Lohmander, L. S., Frobell, R., and Struglics, A. (2015). Type II collagen C2C epitope in human synovial fluid and serum after knee injury - associations with molecular and structural markers of injury. Osteoarthritis Cartilage 23, 1506–1512. doi: 10.1016/j.joca.2015.04.022
Larsson, S., Lohmander, L. S., and Struglics, A. (2009). Synovial fluid level of aggrecan ARGS fragments is a more sensitive marker of joint disease than glycosaminoglycan or aggrecan levels: a cross-sectional study. Arthritis Res. Ther. 11:R92. doi: 10.1186/ar2735
Larsson, S., Struglics, A., Lohmander, L. S., and Frobell, R. (2017). Surgical reconstruction of ruptured anterior cruciate ligament prolongs trauma-induced increase of inflammatory cytokines in synovial fluid: an exploratory analysis in the KANON trial. Osteoarthritis Cartilage 25, 1443–1451. doi: 10.1016/j.joca.2017.05.009
Liu, Q., Niu, J., Huang, J., Ke, Y., Tang, X., Wu, X., et al. (2015). Knee osteoarthritis and all-cause mortality: the Wuchuan Osteoarthritis Study. Osteoarthritis Cartilage 23, 1154–1157. doi: 10.1016/j.joca.2015.03.021
Loeser, R. F., Goldring, S. R., Scanzello, C. R., and Goldring, M. B. (2012). Osteoarthritis: a disease of the joint as an organ. Arthritis Rheum. 64, 1697–1707. doi: 10.1002/art.34453
Lohmander, L. S., Dahlberg, L., Ryd, L., and Heinegård, D. (1989). Increased levels of proteoglycan fragments in knee joint fluid after injury. Arthritis Rheum. 32, 1434–1442. doi: 10.1002/anr.1780321113
Lohmander, L. S., Englund, P. M., Dahl, L. L., and Roos, E. M. (2007). The long-term consequence of anterior cruciate ligament and meniscus injuries: osteoarthritis. Am. J. Sports Med. 35, 1756–1769. doi: 10.1177/0363546507307396
Lohmander, L. S., Ionescu, M., Jugessur, H., and Poole, A. R. (1999). Changes in joint cartilage aggrecan after knee injury and in osteoarthritis. Arthritis Rheum. 42, 534–544. doi: 10.1002/1529-0131(199904)42:3<534::AID-ANR19>3.0.CO;2-J
Lohmander, L. S., Östenberg, A., Englund, M., and Roos, H. (2004). High prevalence of knee osteoarthritis, pain, and functional limitations in female soccer players twelve years after anterior cruciate ligament injury. Arthritis Rheum. 50, 3145–3152. doi: 10.1002/art.20589
Olsson, M. G., Allhorn, M., Bülow, L., Hansson, S. R., Ley, D., Olsson, M. L., et al. (2012). Pathological conditions involving extracellular hemoglobin: molecular mechanisms, clinical significance, and novel therapeutic opportunities for alpha(1)-microglobulin. Antioxid. Redox Signal. 17, 813–846. doi: 10.1089/ars.2011.4282
Olsson, M. G., Allhorn, M., Larsson, J., Cederlund, M., Lundqvist, K., Schmidtchen, A., et al. (2011). Up-regulation of A1M/alpha1-microglobulin in skin by heme and reactive oxygen species gives protection from oxidative damage. PLoS One 6:e27505. doi: 10.1371/journal.pone.0027505
Olsson, M. G., Allhorn, M., Olofsson, T., and Åkerström, B. (2007). Up-regulation of alpha1-microglobulin by hemoglobin and reactive oxygen species in hepatoma and blood cell lines. Free Radic. Biol. Med. 42, 842–851. doi: 10.1016/j.freeradbiomed.2006.12.017
Plesner, T., Nörgaard-Pedersen, B., and Boenisch, T. (1975). Radioimmunoassay of beta2-microglobulin. Scand. J. Clin. Lab. Invest. 35, 729–735. doi: 10.3109/00365517509095804
Scanzello, C. R. (2017). Role of low-grade inflammation in osteoarthritis. Curr. Opin. Rheumatol. 29, 79–85. doi: 10.1097/BOR.0000000000000353
Sharma, L., Kapoor, D., and Issa, S. (2006). Epidemiology of osteoarthritis: an update. Curr. Opin. Rheumatol. 18, 147–156. doi: 10.1097/01.bor.0000209426.84775.f8
Sohn, D. H., Sokolove, J., Sharpe, O., Erhart, J. C., Chandra, P. E., Lahey, L. J., et al. (2012). Plasma proteins present in osteoarthritic synovial fluid can stimulate cytokine production via Toll-like receptor 4. Arthritis Res. Ther. 14:R7. doi: 10.1186/ar3555
Soren, A., Klein, W., and Huth, F. (1978). The synovial changes in post-traumatic synovitis and osteoarthritis. Rheumatol. Rehabil. 17, 38–45. doi: 10.1093/rheumatology/17.1.38
Strålin, P., and Marklund, S. L. (1994). Effects of oxidative stress on expression of extracellular superoxide dismutase, CuZn-superoxide dismutase and Mn-superoxide dismutase in human dermal fibroblasts. Biochem. J. 298(Pt 2), 347–352. doi: 10.1042/bj2980347
Strålin, P., and Marklund, S. L. (2000). Multiple cytokines regulate the expression of extracellular superoxide dismutase in human vascular smooth muscle cells. Atherosclerosis 151, 433–441. doi: 10.1016/S0021-9150(99)00427-X
Struglics, A., Larsson, S., Kumahashi, N., Frobell, R., and Lohmander, L. S. (2015). Changes in cytokines and aggrecan ARGS neoepitope in synovial fluid and serum and in C-Terminal crosslinking telopeptide of type II collagen and n-terminal crosslinking telopeptide of type I Collagen in urine over five years after anterior cruciate ligament rupture: an exploratory analysis in the knee anterior cruciate ligament. Nonsurgical versus surgical treatment trial. Arthritis Rheumatol. 67, 1816–1825. doi: 10.1002/art.39146
Takagi, K., Kin, K., Itoh, Y., Enomoto, H., and Kawai, T. (1980). Human alpha 1-microglobulin levels in various body fluids. J. Clin. Pathol. 33, 786–791. doi: 10.1136/jcp.33.8.786
Terzi, I., Papaioannou, V., Papanas, N., Dragoumanis, C., Petala, A., Theodorou, V., et al. (2014). Alpha1-microglobulin as an early biomarker of sepsis-associated acute kidney injury: a prospective cohort study. Hippokratia 18, 262–268.
Keywords: α1-microglobulin, heme, hemoglobin, hemolysis, knee injury, knee osteoarthritis, oxidative stress, synovial fluid
Citation: Larsson S, Åkerström B, Gram M, Lohmander LS and Struglics A (2018) α1-Microglobulin Protects Against Bleeding-Induced Oxidative Damage in Knee Arthropathies. Front. Physiol. 9:1596. doi: 10.3389/fphys.2018.01596
Received: 01 June 2018; Accepted: 24 October 2018;
Published: 16 November 2018.
Edited by:
Narasaiah Kolliputi, University of South Florida, United StatesReviewed by:
Marcos Lopez, The University of Chicago, United StatesSanjay Khandelwal, Duke University, United States
Copyright © 2018 Larsson, Åkerström, Gram, Lohmander and Struglics. This is an open-access article distributed under the terms of the Creative Commons Attribution License (CC BY). The use, distribution or reproduction in other forums is permitted, provided the original author(s) and the copyright owner(s) are credited and that the original publication in this journal is cited, in accordance with accepted academic practice. No use, distribution or reproduction is permitted which does not comply with these terms.
*Correspondence: Staffan Larsson, c3RhZmZhbi5sYXJzc29uQG1lZC5sdS5zZQ==