- 1Max Delbrück Center for Molecular Medicine in the Helmholtz Association, Berlin, Germany
- 2Partner Site Berlin, German Centre for Cardiovascular Research, Berlin, Germany
- 3Experimental and Clinical Research Center, Max Delbrück Center for Molecular Medicine, Charité – Universitätsmedizin Berlin, Berlin, Germany
- 4Department of Physics, Humboldt-Universität zu Berlin, Berlin, Germany
- 5Partner Site Greifswald, German Centre for Cardiovascular Research, Greifswald, Germany
- 6Klinik und Poliklinik für Innere Medizin B, Universitätsmedizin Greifswald, Greifswald, Germany
- 7Charité – Universitätsmedizin Berlin, Berlin, Germany
- 8Berlin Institute of Health, Berlin, Germany
- 9Institute of Biology, University of Lübeck, Lübeck, Germany
Spinal cord injury (SCI) above mid-thoracic levels leads to autonomic dysfunction affecting both the cardiovascular system and thermoregulation. The renin-angiotensin system (RAS) which is a potent regulator of blood pressure, including its novel beneficial arm with the receptor Mas could be an interesting target in post-SCI hemodynamics. To test the hypothesis that hemodynamics, activity and diurnal patterns of those are more affected in the Mas deficient mice post-SCI we used a mouse model of SCI with complete transection of spinal cord at thoracic level 4 (T4-Tx) and performed telemetric monitoring of blood pressure (BP) and heart rate (HR). Our data revealed that hypothermia deteriorated physiological BP and HR control. Preserving normothermia by keeping mice at 30∘C prevented severe hypotension and bradycardia post-SCI. Moreover, it facilitated rapid return of diurnal regulation of BP, HR and activity in wild type (WT) mice. In contrast, although Mas deficient mice had comparable reacquisition of diurnal HR rhythm, they showed delayed recovery of diurnal rhythmicity in BP and significantly lower nocturnal activity. Exposing mice with T4-Tx (kept in temperature-controlled cages) to 23∘C room temperature for one hour at different time-points post-SCI, demonstrated their inability to maintain core body temperature, Mas deficient mice being significantly more impaired than WT littermates. We conclude that Mas deficient mice were more resistant to acute hypotension, delayed nocturnal recovery, lower activity and more severely impaired thermoregulation. The ambient temperature had significant effect on hemodynamics and, thus it should be taken into account when assessing cardiovascular parameters post-SCI in mice.
Introduction
Spinal cord injury (SCI) above the major splanchnic sympathetic outflow impairs cardiovascular homeostasis inducing end organ damage and cardiovascular disease (Garshick et al., 2005; Hou and Rabchevsky, 2014). Cardiac problems contribute to poikilothermia – the inability to respond adequately to thermal challenges and it in turn can cause cardiovascular complications such as bleeding, metabolic acidosis, arrhythmia, decline in cardiac contractility, and exercise-induced hyperthermia (Greene et al., 1989; Colachis, 2002; Price and Campbell, 2003; Tsuei and Kearney, 2004; Price, 2006).
The renin-angiotensin system (RAS), a potent regulator of blood pressure (BP), with its classical harmful components (angiotensin II, AngII and its receptor AT1) becomes activated after high-level SCI (Frankel et al., 1972), initiating target organ damage and possibly autonomic dysregulation (Mathias et al., 1975; Groothuis et al., 2010). However, there are also protective arms of the RAS comprising angiotensin-(1-7), Mas and AT2 receptors counteracting the detrimental effects of the classical RAS, stimulation of which has been proven to be efficient in many cardiovascular disease states (Bader, 2013). Whereas the AT2 receptor has mainly anti-inflammatory and regenerative functions (Steckelings et al., 2010), we have shown recently that Mas plays a role in autonomic dysreflexia following SCI (Jarve et al., 2019). Mas, widely expressed including in the central nervous system (CNS) (Becker et al., 2007), acts protective in the cardiovascular system by lowering BP and suppressing inflammation and oxidative stress. In contrast to AngII, Ang-(1-7), the ligand of Mas, attenuates the nerve stimulation-induced norepinephrine (NE) release (Westfall et al., 2013; Al Dera and Brock, 2014).
Telemetric monitoring of BP in conscious freely moving mice combined with SCI models and transgenic technology would be a potent strategy to evaluate molecular factors involved in cardiovascular dysfunction following SCI. The feasibility of this approach in mice remains to be explored. Therefore, we conducted a pilot study, employing telemetry to characterize acute and chronic hemodynamic reaction to SCI in wild type (WT) and Mas deficient (Mas-/-) mice with focus on the development of alterations in diurnal BP and heart rate (HR) rhythms and thermodysregulation. We hypothesized that in the absence of protective Mas the hemodynamic response to SCI would be more severe.
Materials and Methods
Animals
Experiments with female WT and Mas-/- mice back-crossed into C57Bl/6N background (Walther et al., 1998), weighing 20 to 25 g, and 3–4.5 months of age, with food and water ad libitum and a constant 12:12-h light/dark cycle, were conducted according to the National Institutes of Health Guide for the Care and Use of Laboratory Animals, and with the protocols previously approved by the local Animal Care and Use Committee from Berlin LAGeSo (G0132/14).
Telemetry
We conducted a within animal pre- and post-SCI design, omitting the sham-operated mice in telemetry. All operative interventions were done under intraperitoneal Ketamine (100 mg/kg) – Xylazine (10 mg/kg) anesthesia in combination with Isoflurane (1.5–1.8%) inhalation. As described in detail elsewhere (Gross et al., 2005), the tip of the radiotelemetric device TA11PA-C10 (Data Sciences Int., United States) was implanted into the left femoral artery pointing toward the abdominal aorta. This catheter position is preferred for mice in terms of survival and baroreceptor disturbances compared to a catheter position in the aorta or carotid artery, respectively (unpublished observation) and (Carlson and Wyss, 2000). The transmitter was implanted in a subcutaneous pocket. Recordings were started 7 days after surgery, when hemodynamics had normalized. During the whole study, beat-by-beat arterial BP and HR were continuously monitored 24 h/day. Data were collected using the Dataquest ART system, version 2.1 (Data Sciences International).
Spinal Cord Injury
On a heating plate (37°C), a dorsal midline incision was made in the superficial muscle overlying a region from the 7th cervical to the 3rd thoracic (C7-T3) vertebrae. The dura was opened at the T2-T3 intervertebral gap and the spinal cord was completely transected using microscissors. Complete transection was confirmed by pulling a needle twice between the rostral and caudal spinal cord ends. Gelfoam was placed above the spinal cord to achieve hemostasis. The muscle and skin were closed with absorbable sutures (Vicryl, 4-0, Ethicon GmbH). Animals received pre-warmed saline (1 ml, s.c.) and recovered on a heating pad for 3–6 h. Body surface temperature was measured by non-contact infrared thermometer held 5 cm from the mouse by pointing the beam at flank. After we had determined that mice do not recover thermoregulation (WT, n = 4) the single-housed cages were kept on heating pads (37°C) such that in the cage the temperature was 30°C (for the telemetry experiment: WT: n = 4, Mas-/-: n = 4 mice; for the thermoregulation experiment: WT: n = 10, Mas-/-: n = 10). For analgesia, mice were treated with Carprofen (4 mg/kg, s.c.) directly after operation and the next day with a 12 h interval or longer if necessary. The bladder was manually emptied three times daily for the entire duration period of the experiment, as bladder function did not recover.
Assessment of Diurnal Rhythmicity in Hemodynamics and Locomotor Activity
Systolic blood pressure (SBP), heart rate (HR) and gross locomotory activity in the cages were measured and averaged on a 3 h basis (starting 7:30 PM, 10:30 PM; 1:30 AM, 4:30 AM, 7:30 AM, 10:30 AM, 1:30 PM, and 4:30 PM). Hemodynamic variables obtained during the night (7:30 PM–7:30 AM) were compared with values obtained during daytime (7:30 AM–7:30 PM).
Body-Core Temperature Experiment
In a separate group of mice (WT SCI: n = 10, WT sham: n = 5, Mas-/- SCI: n = 10, Mas-/- sham: n = 5) rectal body temperature was measured by inserting the rectal probe (TMF-3402) connected to the Temperature-Control-Module (TKM-0903, FMI GmbH, Seeheim, Germany) at 9:00 a.m and after 1 or 2 h (as indicated) of group housing at room temperature of 23°C at 4, 10, 17 and 26 days post T4-Tx SCI. Insertion depth was 2 cm and as such reflects core body temperature (Meyer et al., 2017).
Statistical Analyses
Results are presented as mean ± SEM. Data were analyzed statistically using the GraphPad Prism 5 software (GraphPad Software, CA, United States). One-way and 2-way ANOVA with Bonferroni post-tests were used to detect significant differences in telemetry and thermoregulation as well as body weight loss data, respectively. A value of two-sided P < 0.05 was considered statistically significant.
Results
Autonomic Regulation at Room Temperature Following SCI
T4-Tx SCI in wild type mice caused systolic blood pressure (SBP) to fall from baseline 110.9 ± 1.3 to 62.4 ± 1.1 mmHg (p < 0.0001, Student’s t-test) and heart rate (HR) from 607.8 ± 5.4 to 216.2 ± 13.2 bpm (p < 0.0001, Student’s t-test) within the first 2 (days post-injury) dpi. The surface body temperature of mice measured by a non-contact thermometer fell from 38.2 ± 0.1 to 32.3 ± 1.0°C (p < 0.0001, Student’s t-test). At 3 dpi we stopped telemetry and kept the mice in the temperature-controlled cage at 30°C for 4 h, after which hemodynamics improved (Supplementary Figure 1). Mice with T4-Tx have thermodysregulation which prevented successful blood pressure assessment unless the mice were kept in temperature-controlled cages.
Protecting Core Body Temperature Improved Hypotension, Bradycardia and Locomotor Activity Post-SCI
Wild type as well as Mas-/- mice kept in the temperature-controlled cages at 30°C had significant, however, less pronounced reduction in the SBP and HR following T4-Tx compared to mice at 23°C (Figures 1A,B). Normothermia, thus, helped to prevent dramatic hypotension and bradycardia following SCI in mice. Interestingly, the SBP fall in the acute phase (1-7 dpi) was significantly smaller in Mas-/- mice, however, for the rest of the 4 weeks it was similar in both strains (Figures 1D–F). Heart rate, in contrast, was similar in the acute phase and became significantly higher in WT mice during the chronic phase (Figures 1G–I). Expectedly low locomotor activity post-SCI was observed which remained significantly reduced in Mas-/- compared to WT mice in both the acute as well as the chronic phase of SCI (Figures 1C,J–L).
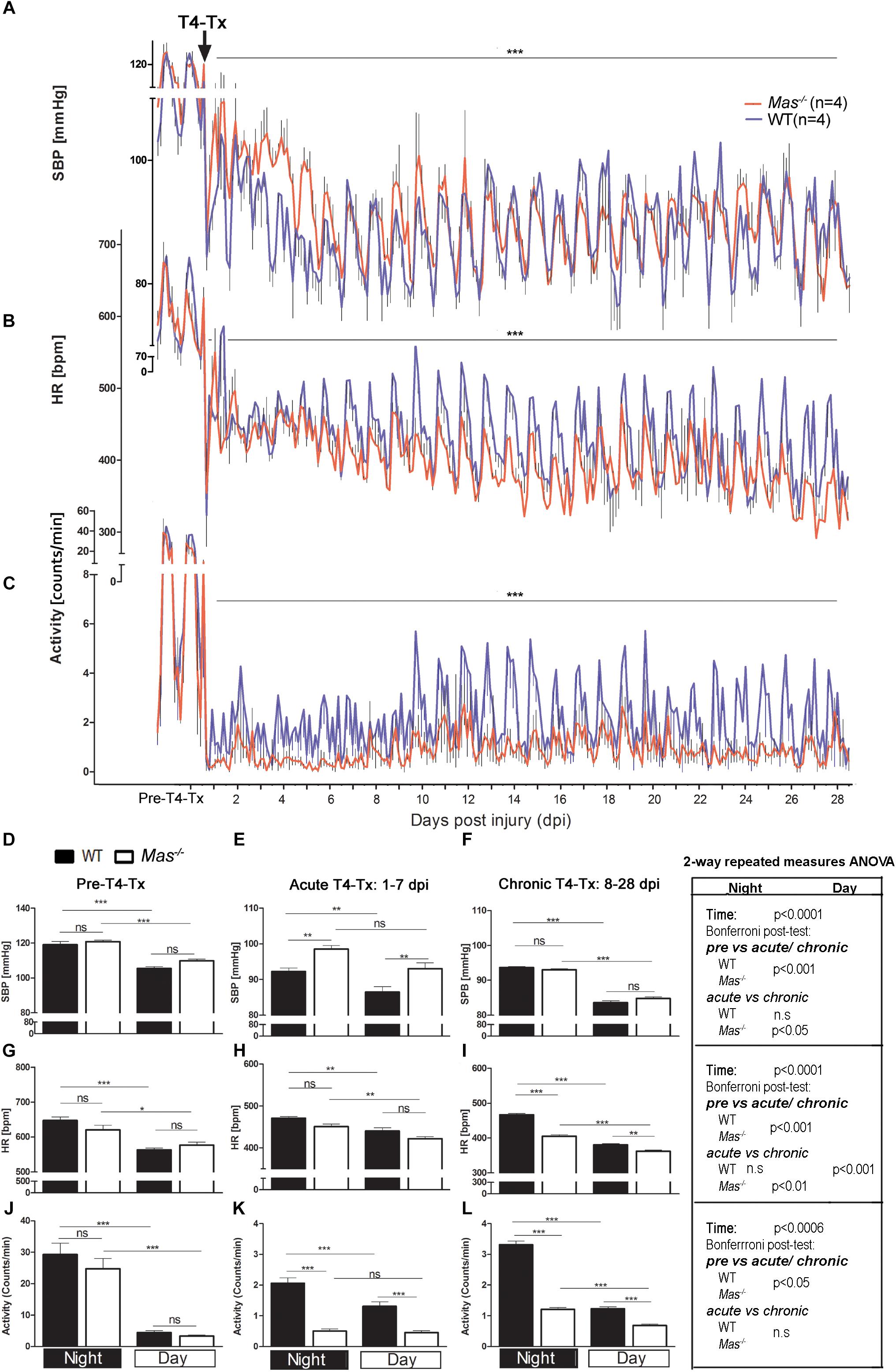
Figure 1. Autonomic functions at 30°C. (A) Systolic blood pressure (SBP), (B) heart rate (HR) and (C) locomotor activity in the cage prior to and after spinal cord injury (SCI). Data are presented as mean values of every 3 h and standard error of the mean (SEM). Significances pre-injury vs. post-T4-Tx for SBP, HR and activity in WT and Mas-/- groups, respectively, Student’s t-test ∗∗∗p < 0.001. Baseline, acute phase and chronic phase SBP (D–F, respectively), HR (G–I) and activity (J–L) for daytime and night time. Significances ∗(∗∗/∗∗∗)p < 0.05 (0.01/0.001) one-way ANOVA with Bonferroni-adjusted post hoc analysis, (above bar graphs); right side box for the effect of time point (pre, acute and chronic phase of SCI) 2-way repeated measures ANOVA with Bonferroni-adjusted post hoc analysis, ns, not significant.
Normothermia Facilitated the Return of Diurnal Regulation of Hemodynamics
Typical diurnal rhythmicity in cardiovascular control, as characterized by the significantly lower SBP and HR during day when the mice are usually quiescent, was lost acutely following SCI (Figures 1A,B,D–I). Diurnal regulation of SBP and locomotor activity recovered in WT mice kept at 30°C in the acute phase, and in Mas-/- mice only in the chronic phase of SCI (Figures 1E,F,K,L). Diurnal rhythmicity in HR at 30°C was present in both strains already in the acute phase of SCI (Figures 1H,I), however, with greater 24-h amplitude in WT. Taken together, preventing hypothermia facilitated the recovery of nocturnal regulation of SPB, HR and activity, although with significant delay in Mas-/- mice.
Mas Protects Core Body Temperature Following SCI
In order to determine when thermoregulation might recover, another cohort of mice with T4-Tx were constantly warmed at 30°C and challenged with the 23°C room temperature (RT) for an hour at 4, 10, 17, and 26 days post injury (dpi) before and after which their rectal core body temperature (CBT) was determined. As expected sham-operated WT and Mas-/- mice kept their mean CBT during an hour at RT, whereas mice with T4-Tx showed a decrease in CBT (Figure 2). Mas-/- mice showed a significantly stronger decrease in CBT during an hour at RT compared to WT mice (Figure 2). Taken together, the ability to maintain CBT would not recover after SCI in the absence of Mas.
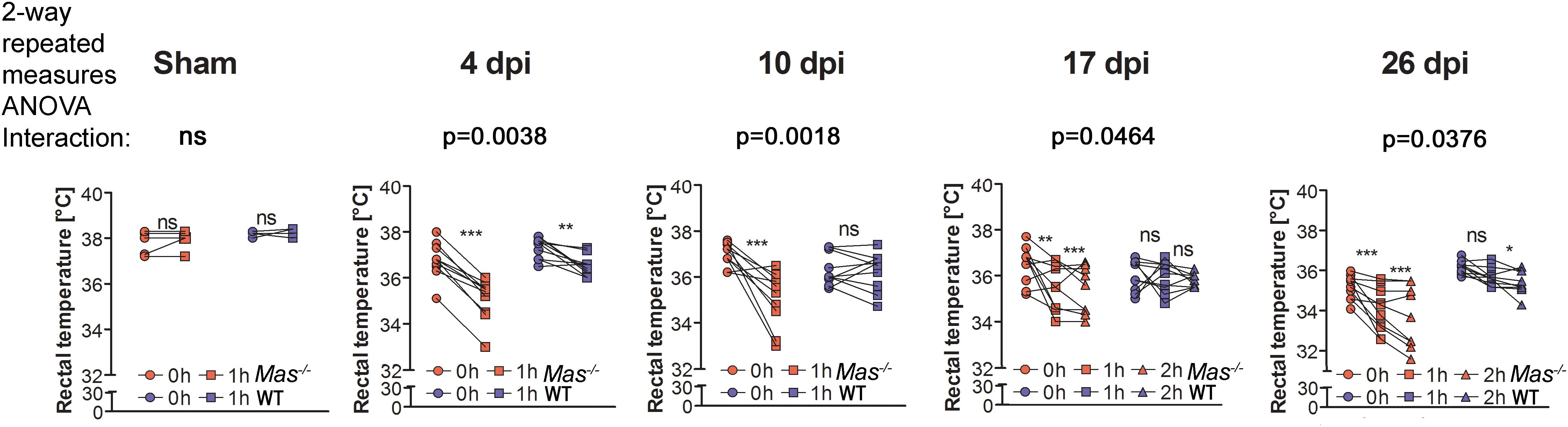
Figure 2. Body core temperature of mice with sham-OP or T4-Tx at indicated time-points post surgery measured prior to (at 30°C) and 1 h after keeping the mice at room temperature (23°C). On 17 and 26 days post injury (dpi) mice were additionally exposed to a 2nd hour at RT. Sham: WT n = 5 and Mas-/-n = 5, T4-Tx: WT n = 10 and Mas-/- n = 10. 2-way repeated measures ANOVA, significance of interaction between genotype (WT, Mas-/-) and time (0, 1, and 2 h at RT) displayed on figure, Bonferroni-adjusted post hoc analysis, ∗(∗∗/∗∗∗) p < 0.05 (0.01/0.001).
Discussion
Our study is the first to examine resting hemodynamics as well as thermoregulation before and after SCI in WT and Mas-deficient mice. We found that (i) T4-Tx SCI in mice caused persistent hypothermia together with severe hypotension and bradycardia; (ii) normothermia improved hypotension, bradycardia and locomotor activity and furthermore facilitated reappearance of their diurnal regulation; (iii) Mas-/- mice had significantly impaired thermoregulation post-SCI.
Thermodysregulation and Its Effect on Hemodynamics
We report that hypothermia post-SCI is drastically affecting the regulation of cardiovascular parameters in mice at room temperature. In contrast to mice, rats present only an acute decrease in body temperature at 1–2 dpi following T3/T4-Tx (Laird et al., 2006; West et al., 2015). Smaller size of mice predestines them for a higher metabolic rate and because of a higher body surface-to-volume ratio they lose proportionally more heat than rats. Consequently, maintaining normothermia by heating the cages improved hemodynamic reactions to SCI and facilitated locomotor activity. Theoretically, the smaller body surface-to-volume ratio of humans should protect them from thermodysregulation, but there are no such data on SCI patients.
Diurnal Patterns
Thermoneutrality was necessary for rapid restoration of diurnal rhythm in both hemodynamic parameters and in locomotor activity. In comparison, rats with T3/T4 lesion kept in temperature-uncontrolled cages, while normothermic, had rhythmicity returned as late as 2 weeks post-SCI (West et al., 2015). Diurnal desynchrony is involved in the progression of organ dysfunction and development of cardiac disease (Martino et al., 2007; Douma and Gumz, 2017). Quicker return of diurnal rhythmicity in hemodynamics in our study might therefore help to prevent pathology of the cardiovascular system.
Mas in Hemodynamics
Mas-/- mice had higher SBP in acute phase likely reflecting higher vascular tonus (lack of local vasodilation) with diminished local vasodilatory effect of bradykinin and NO as well as Ang-(1-7) (Xu et al., 2008). In addition, circulating AngII activates AT1 receptors expressed in the circumventricular organs, which also drives vasopressin release, and this likely contributes to recovery of BP following SCI (Capone et al., 2012). This could be potentiated in the absence of Mas. Despite of higher SBP Mas-/- mice had very low activity suggesting activity-independent circadian regulation of SBP (Sei et al., 1997).
Putative Role of Mas in Thermoregulation
Mas seems to be required for thermoregulation post-SCI as Mas-/- mice could not keep a constant CBT at RT when compared to WT mice. Accordingly, a previous study by our laboratory with an endotoxemia mouse model showed significantly larger loss of CBT in Mas-/- mice (Souza et al., 2014). The responsible mechanism in these models might be different and affect thermal sensing, central regulation and efferent axis with thermogenesis and blood flow regulation. Shivering thermogenesis by muscular contraction or non-shivering thermogenesis originating from brown adipose tissue (BAT) could be both impaired in Mas-/- mice. Because of paralyzed hind limbs mice could shiver only with the upper body. Mas-/- mice lost more skeletal muscle mass as well as body mass following T4-Tx SCI (Supplementary Figure 2) consistent with the effect of Mas deficiency in other muscular dystrophy models (Acuna et al., 2014). Therefore, they might have shivered less effectively and lost more temperature at RT. Indeed, antagonism of Mas leads to highly deteriorated muscular architecture with diminished muscle strength (Acuna et al., 2014). Treatment with Ang-(1-7) prevented atrophy in disuse skeletal muscles, and this effect was absent in the Mas-/- mice (Morales et al., 2016). On the other hand, central Ang-(1-7) was reported to increase BAT thermogenesis along with higher level of phosphorylated hormone sensitive lipase which drives BAT lipolysis, processes which conversely could be affected in the absence of Mas (Evangelista and Bartness, 2015). Also mice lacking Mas exhibited a 55% decrease in blood flow in the BAT (Botelho-Santos et al., 2012). Taken together, it seems that Mas is required for thermoregulation involving thermogenesis.
Conclusion
High-level SCI in mice leads to hypotension, bradycardia and hypothermia. Mas-/- mice had in acute phase less severe hypotension, delayed recovery of diurnal rhythmicity in BP and significantly lower nocturnal activity and could not keep body core temperature as efficiently as wild type mice, furthermore, they had more reduced leg muscles. The major factor influencing hemodynamics and its rhythmicity post SCI was the ambient temperature. Future studies are warranted to explore the effects also in male mice, to find the mechanisms for the differences in the absence of Mas.
Author Contributions
AJ performed the experiments, analyzed the data, and wrote the manuscript. MT performed the experiments and interpreted the data. MK and FF performed the experiments. JK, RP, and NW analyzed and interpreted the telemetry data. NA and JF revised the manuscript. MB helped by planning the experiments and manuscript revision.
Funding
This work was supported by the Wings for Life (Research Grant 2014–2016), the Deutsche Forschungsgemeinschaft (DFG, FI 965/5-2) and the DZHK (81Z5400153). MDC-library funded open access publication fees.
Conflict of Interest Statement
The authors declare that the research was conducted in the absence of any commercial or financial relationships that could be construed as a potential conflict of interest.
Acknowledgments
We acknowledge technical assistance of Andrea Rodak and Sibylle Schmidt.
Supplementary Material
The Supplementary Material for this article can be found online at: https://www.frontiersin.org/articles/10.3389/fphys.2018.01930/full#supplementary-material
References
Acuna, M. J., Pessina, P., Olguin, H., Cabrera, D., Vio, C. P., Bader, M., et al. (2014). Restoration of muscle strength in dystrophic muscle by angiotensin-1-7 through inhibition of TGF-beta signalling. Hum. Mol. Genet. 23, 1237–1249. doi: 10.1093/hmg/ddt514
Al Dera, H., and Brock, J. A. (2014). Spinal cord injury increases the reactivity of rat tail artery to angiotensin II. Front. Neurosci. 8:435. doi: 10.3389/fnins.2014.00435
Bader, M. (2013). ACE2, angiotensin-(1-7), and Mas: the other side of the coin. Pflugers Arch. 465, 79–85. doi: 10.1007/s00424-012-1120-0
Becker, L. K., Etelvino, G. M., Walther, T., Santos, R. A., and Campagnole-Santos, M. J. (2007). Immunofluorescence localization of the receptor Mas in cardiovascular-related areas of the rat brain. Am. J. Physiol. Heart Circ. Physiol. 293, H1416–H1424. doi: 10.1152/ajpheart.00141.2007
Botelho-Santos, G. A., Bader, M., Alenina, N., and Santos, R. A. (2012). Altered regional blood flow distribution in Mas-deficient mice. Ther. Adv. Cardiovasc. Dis. 6, 201–211. doi: 10.1177/1753944712461164
Capone, C., Faraco, G., Peterson, J. R., Coleman, C., Anrather, J., Milner, T. A., et al. (2012). Central cardiovascular circuits contribute to the neurovascular dysfunction in angiotensin II hypertension. J. Neurosci. 32, 4878–4886. doi: 10.1523/JNEUROSCI.6262-11.2012
Carlson, S. H., and Wyss, J. M. (2000). Long-term telemetric recording of arterial pressure and heart rate in mice fed basal and high NaCl diets. Hypertension 35, e1–e5. doi: 10.1161/01.HYP.35.2.e1
Colachis, S. C. III (2002). Hypothermia associated with autonomic dysreflexia after traumatic spinal cord injury. Am. J. Phys. Med. Rehabil. 81, 232–235. doi: 10.1097/00002060-200203000-00013
Douma, L. G., and Gumz, M. L. (2017). Circadian clock-mediated regulation of blood pressure. Free Radic. Biol. Med. 119, 108–114. doi: 10.1016/j.freeradbiomed.2017.11.024
Evangelista, F., and Bartness, T. (2015). Central Ang 1-7 modulates lipolysis and thermogenesis in adipose tissue. FASEB J. 29, 981.1.
Frankel, H. L., Michaelis, L. S., Golding, D. R., and Beral, V. (1972). The blood pressure in paraplegia. I. Paraplegia 10, 193–200. doi: 10.1038/sc.1972.32
Garshick, E., Kelley, A., Cohen, S. A., Garrison, A., Tun, C. G., Gagnon, D., et al. (2005). A prospective assessment of mortality in chronic spinal cord injury. Spinal Cord 43, 408–416. doi: 10.1038/sj.sc.3101729
Greene, P. S., Cameron, D. E., Mohlala, M. L., Dinatale, J. M., and Gardner, T. J. (1989). Systolic and diastolic left ventricular dysfunction due to mild hypothermia. Circulation 80, III44–III48.
Groothuis, J. T., Thijssen, D. H., Rongen, G. A., Deinum, J., Danser, A. H., Geurts, A. C., et al. (2010). Angiotensin II contributes to the increased baseline leg vascular resistance in spinal cord-injured individuals. J. Hypertens 28, 2094–2101. doi: 10.1097/HJH.0b013e32833cd2f4
Gross, V., Tank, J., Obst, M., Plehm, R., Blumer, K. J., Diedrich, A., et al. (2005). Autonomic nervous system and blood pressure regulation in RGS2-deficient mice. Am. J. Physiol. Regul. Integr. Comp. Physiol. 288, R1134–R1142. doi: 10.1152/ajpregu.00246.2004
Hou, S., and Rabchevsky, A. G. (2014). Autonomic consequences of spinal cord injury. Compr. Physiol. 4, 1419–1453. doi: 10.1002/cphy.c130045
Jarve, A., Todiras, M., Lian, X., Filippelli-Silva, R., Qadri, F., Martin, R. P., et al. (2019). Distinct roles of angiotensin receptors in autonomic dysreflexia following high-level spinal cord injury in mice. Exp. Neurol. 311, 173–181. doi: 10.1016/j.expneurol.2018.10.003
Laird, A. S., Carrive, P., and Waite, P. M. (2006). Cardiovascular and temperature changes in spinal cord injured rats at rest and during autonomic dysreflexia. J. Physiol. 577, 539–548. doi: 10.1113/jphysiol.2006.116301
Martino, T. A., Tata, N., Belsham, D. D., Chalmers, J., Straume, M., Lee, P., et al. (2007). Disturbed diurnal rhythm alters gene expression and exacerbates cardiovascular disease with rescue by resynchronization. Hypertension 49, 1104–1113. doi: 10.1161/HYPERTENSIONAHA.106.083568
Mathias, C. J., Christensen, N. J., Corbett, J. L., Frankel, H. L., Goodwin, T. J., and Peart, W. S. (1975). Plasma catecholamines, plasma renin activity and plasma aldosterone in tetraplegic man, horizontal and tilted. Clin. Sci. Mol. Med. 49, 291–299. doi: 10.1042/cs0490291
Meyer, C. W., Ootsuka, Y., and Romanovsky, A. A. (2017). Body temperature measurements for metabolic phenotyping in mice. Front. Physiol. 8:520. doi: 10.3389/fphys.2017.00520
Morales, M. G., Abrigo, J., Acuna, M. J., Santos, R. A., Bader, M., Brandan, E., et al. (2016). Angiotensin-(1-7) attenuates disuse skeletal muscle atrophy in mice via its receptor, Mas. Dis. Model. Mech. 9, 441–449. doi: 10.1242/dmm.023390
Price, M. J. (2006). Thermoregulation during exercise in individuals with spinal cord injuries. Sports Med. 36, 863–879. doi: 10.2165/00007256-200636100-00005
Price, M. J., and Campbell, I. G. (2003). Effects of spinal cord lesion level upon thermoregulation during exercise in the heat. Med. Sci. Sports Exerc. 35, 1100–1107. doi: 10.1249/01.MSS.0000074655.76321.D7
Sei, H., Furuno, N., and Morita, Y. (1997). Diurnal changes of blood pressure, heart rate and body temperature during sleep in the rat. J. Sleep Res. 6, 113–119. doi: 10.1046/j.1365-2869.1997.00038.x
Souza, L. L., Duchene, J., Todiras, M., Azevedo, L. C., Costa-Neto, C. M., Alenina, N., et al. (2014). Receptor MAS protects mice against hypothermia and mortality induced by endotoxemia. Shock 41, 331–336. doi: 10.1097/SHK.0000000000000115
Steckelings, U. M., Rompe, F., Kaschina, E., Namsolleck, P., Grzesiak, A., Funke-Kaiser, H., et al. (2010). The past, present and future of angiotensin II type 2 receptor stimulation. J. Renin Angiotensin Aldosterone Syst. 11, 67–73. doi: 10.1177/1470320309347791
Tsuei, B. J., and Kearney, P. A. (2004). Hypothermia in the trauma patient. Injury 35, 7–15. doi: 10.1016/S0020-1383(03)00309-7
Walther, T., Balschun, D., Voigt, J. P., Fink, H., Zuschratter, W., Birchmeier, C., et al. (1998). Sustained long term potentiation and anxiety in mice lacking the Mas protooncogene. J. Biol. Chem. 273, 11867–11873. doi: 10.1074/jbc.273.19.11867
West, C. R., Popok, D., Crawford, M. A., and Krassioukov, A. V. (2015). Characterizing the temporal development of cardiovascular dysfunction in response to spinal cord injury. J. Neurotrauma 32, 922–930. doi: 10.1089/neu.2014.3722
Westfall, T. C., Macarthur, H., Byku, M., Yang, C. L., and Murray, J. (2013). Interactions of neuropeptide y, catecholamines, and angiotensin at the vascular neuroeffector junction. Adv. Pharmacol. 68, 115–139. doi: 10.1016/B978-0-12-411512-5.00006-3
Keywords: blood pressure, heart rate, telemetry, renin-angiotensin system, diurnal rhythm
Citation: Järve A, Todiras M, Kny M, Fischer FI, Kraemer JF, Wessel N, Plehm R, Fielitz J, Alenina N and Bader M (2019) Angiotensin-(1-7) Receptor Mas in Hemodynamic and Thermoregulatory Dysfunction After High-Level Spinal Cord Injury in Mice: A Pilot Study. Front. Physiol. 9:1930. doi: 10.3389/fphys.2018.01930
Received: 28 September 2018; Accepted: 21 December 2018;
Published: 11 January 2019.
Edited by:
Valdir Andrade Braga, Federal University of Paraíba, BrazilReviewed by:
Stephen B. G. Abbott, University of Virginia, United StatesEric Lazartigues, LSU Health Sciences Center New Orleans, United States
Jose Luiz De Brito Alves, Federal University of Paraíba, Brazil
Copyright © 2019 Järve, Todiras, Kny, Fischer, Kraemer, Wessel, Plehm, Fielitz, Alenina and Bader. This is an open-access article distributed under the terms of the Creative Commons Attribution License (CC BY). The use, distribution or reproduction in other forums is permitted, provided the original author(s) and the copyright owner(s) are credited and that the original publication in this journal is cited, in accordance with accepted academic practice. No use, distribution or reproduction is permitted which does not comply with these terms.
*Correspondence: Anne Järve, anne.jaerve@mdc-berlin.de