- Centre for Urology Research, Faculty of Health Sciences & Medicine, Bond University, Gold Coast, QLD, Australia
Current pharmacological treatment options for many bladder contractile dysfunctions are not suitable for all patients, thereby bringing interest to the investigation of therapies that target a combination of receptors. This study aimed to compare responses of PGE2 on the urinary bladder urothelium with lamina propria (U&LP, also called the bladder mucosa) or detrusor smooth muscle and attempt to identify the receptor subtypes mediating PGE2 contractile responses in these tissues. In the presence of selective EP1 – 4 receptor antagonists, varying concentrations of PGE2 were applied to isolated strips of porcine U&LP and detrusor that were mounted in organ baths filled with Krebs-bicarbonate solution and gassed with carbogen. The addition of PGE2 (1 and 10 μM) and PGF2α (10 μM) to U&LP preparations caused significant increases in the baseline tension and in the spontaneous phasic contractile frequency. In detrusor preparations, significant increases in the baseline tension were observed in response to PGE2 (1 and 10 μM) and PGFα (10 μM), and spontaneous phasic contractions were initiated in 83% of preparations. None of the selective PGE2 receptor antagonists inhibited the increases in baseline tension in both U&LP and detrusor. However, the antagonism of PGF2α receptor showed significantly inhibited contractile responses in both layers of the bladder. This study presents prostaglandin receptor systems as a potential regulator of urinary bladder contractility. The main contractile effects of PGE2 in both U&LP and detrusor are mediated via the FP receptor with no observed contribution from any of the four EP receptors.
Introduction
Bladder contractile dysfunctions, such as overactive bladder (OAB) and underactive bladder (UAB), are common lower urinary tract dysfunctions that lower the overall quality of life in people living with it (Haylen et al., 2010; D’ancona et al., 2019), heightens the likelihood of developing depression and anxiety, as well as increase healthcare usage (Milsom et al., 2012). Despite extensive research investigating the mechanisms that potentially mediate bladder contractions, the cause and development of many lower urinary tract dysfunctions are poorly understood. Bladder contractions are under the control of the parasympathetic nervous system (De Groat et al., 1981) and mediated by the release of acetylcholine that acts upon the M2 and M3 muscarinic receptors found in the detrusor smooth muscle (Hegde et al., 1997; Sigala et al., 2002). It has been recognized that the urothelium, which lines the lumen of the bladder, is capable of responding to mechanical, chemical and thermal stimuli (Birder, 2006; Stromberga et al., 2019, 2020b). Furthermore, it is capable of releasing several signaling molecules in response to stretch, including acetylcholine (Moro et al., 2011) ATP and nitric oxide (Ferguson et al., 1997; Fry and Vahabi, 2016) that are involved in the modulation of the micturition reflex. Myofibroblasts (also referred to as interstitial cells) found in the underlying lamina propria are thought to be involved in modulating bladder behavior by amplifying sensory responses to stretch occurring during the filling phase (Fry et al., 2007). Therefore, the involvement of the urothelium and the underlying lamina propria should be considered as potential contributors to bladder contractile pathology.
Prostaglandins have been suggested to be involved in the modulation of bladder function for decades. They are synthesized from arachidonic acid that is released from the cell membrane via the hydrolysis of the SN-2 bond by the phospholipase A2 enzyme (PLA2) (Clark et al., 1991). Prostaglandins can also be produced locally within the U&LP and smooth muscle layers in the human urinary bladder (Abrams et al., 1979; Jeremy et al., 1987). Two cyclooxygenase isoforms COX-1 and COX-2 metabolize arachidonic acid into PGH2, which is subsequently converted into five primary prostaglandins via their respective synthases: PGE2, PGD2, PGF2α, PGI2 and TXA2 (Woodward et al., 2011). These signaling molecules exert their function through the stimulation of nine specific G-protein coupled receptors (GPCRs): EP1–EP4, DP1, DP2, FP2, IP, and TP, respectively. Both COX-1 and COX-2 are expressed within the bladder wall (De Jongh, 2007, 2009) specifically on the urothelium, lamina propria and on the surface of the inner muscle bundles of the detrusor. COX-1 is predominately expressed in the basal and intermediate layers of the urothelium and on the interstitial cells located in the lamina propria, indicating that these cells are capable of respond to prostaglandins (De Jongh, 2009). The production of prostaglandins differs between species. For example, in the rat bladder, PGI2 is the major prostaglandin produced (Jeremy et al., 1984), whereas in the rabbit bladder it is PGE2 (Leslie et al., 1984). In the human bladder, the primary prostaglandins synthesized are PGI2, followed by PGE2, PGF2α, and TXA2 (Jeremy et al., 1984; Masunaga et al., 2006). However, there are significant differences in the bladder contractile responses between species for the different prostaglandin receptor subtypes (Root et al., 2015).
Studies involving human detrusor have shown that the release of prostaglandins has direct influences on the micturition reflex (Bultitude et al., 1976; Andersson et al., 1977). Overproduction of prostaglandins have been observed in several conditions, including bladder outlet obstruction (Masick et al., 2001), bladder overactivity due to spinal cord injury (Masunaga et al., 2006), and in inflammation (Wheeler et al., 2001). Furthermore, contractions of the detrusor smooth muscle in response to acetylcholine and ATP are enhanced by prostaglandins in both rabbit (Anderson, 1982) and guinea-pig animal models (Nile et al., 2010). There are complex interactions between the three chemicals, as ATP enhances PGE2 release via a P2-purinoreceptor-mediated mechanism (Kasakov and Vlaskovska, 1985), and acetylcholine is a modulator of PGE2 release (Nile and Gillespie, 2012). The interactions between these mediators can potentially result in amplification of molecule signaling and therefore in increased contractile activity, further supporting the interest in investigating prostaglandin involvement in contractile disorders such as OAB. Other research has also shown interest in prostaglandin release from the lower urinary tract. For example, the presence of PGE2 in the urine has been linked to bladder dysfunction and proposed as a potential future biomarker (Reyes and Klahr, 1990). To further this, urine samples collected from female patients with overactive bladder showed that there were significant increases in the PGE2 and PGF2α levels when compared with the control group (Kim et al., 2006).
Prostaglandin E2, in particular, is thought to be the most likely contributor to bladder disorders as it can modulate bladder function via afferent signaling (Andersson, 2010) and potentially detrusor overactivity by sensitizing capsaicin-sensitive afferent nerve endings (Maggi, 1988; Park et al., 1999). It is released upon degranulation of mast cells (Schmauder-Chock and Chock, 1989) together with histamine, which is also capable of impacting the bladder contractility (Stromberga et al., 2019, 2020a). Moreover, PGE2 is produced locally in the bladder in response to distension and inflammation (Funk, 2001). Notably, the excitatory effects exerted on the bladder wall via the stimulation of PGE2 receptors has previously been studied in the rat (Chuang et al., 2012) and mouse (Schroder et al., 2004; Mccafferty et al., 2008) animal models. There are four identified prostaglandin E2 receptor subtypes: EP1, EP2, EP3, and EP4. The presence of the EP1 and EP2 receptor subtypes has been shown in guinea pig urothelium and lamina propria (Rahnama’i et al., 2010) and the expression of all four PGE2 receptor subtypes (EP1–EP4) has been identified in the canine bladder using in situ hybridization and immunohistochemistry (Ponglowhapan et al., 2010). Generally, activation of EP1 and EP3 receptors is associated with an excitatory response, whereas EP2 and EP4 are associated with inhibition of smooth muscle contractility (Woodward et al., 2011). Furthermore, clinical uses of the prostaglandin E2 agonist dinoprostone, include the stimulation of uterine smooth muscle contractions during labor (Stephenson and Wing, 2015) with the prostaglandin F2α agonist, carboprost, being a viable alternative to oxytocin during the management of labor (Sunil Kumar et al., 2016). Therefore, the involvement of EP1, EP3 and FP receptors is of particular interest in the modulation of lower urinary tract contractility due to their excitatory effect when stimulated.
Alterations in bladder physiology in disease states has been thought to primarily occur through the regulation of detrusor smooth muscle contractility. However, it is increasingly recognized that the bladder urothelium with lamina propria (U&LP, also called the bladder mucosa) plays an important role in the overall contractility of the bladder and in its intracellular signaling. As such, recent research has focused on the effects of pharmacological mediators on the physiology of the U&LP and the detrusor as separate layers. This study aimed to compare responses of the urothelium with lamina propria to PGE2 with those of the detrusor and identify the receptor subtypes responsible for the PGE2-mediated contractions in these tissues.
Materials and Methods
Tissue Preparation
Urinary bladders were obtained from female Large-White-Landrace pigs (approximately 6 months old, with a live-weight of 80–100 kg) from the local abattoir after slaughter for the routine commercial provision of food. Isolated strips (10 mm × 5 mm) of porcine bladder were removed from the anterior wall of the bladder dome region and urothelium with lamina propria (U&LP) carefully dissected from the underlying detrusor layer. Two adjacent strips were taken from each animal’s bladder and paired together as control-experimental tissues, with each paired strip preparation expressed as “n” where comparisons or changes are recorded. n equates to the number of individual bladders used in this study.
Strips of U&LP and detrusor tissue were suspended in 10 mL organ baths (Labglass, Brisbane, QLD, Australia) containing Krebs-bicarbonate solution (NaCl 118.4 mM, NaHCO3 24.9 mM, CaCl2 1.9 mM, MgSO4 2.41 mM, KCl 4.6 mM, KH2PO4 1.18 mM, and D-glucose 11.7 mM) at 37°C and perfused with a gas mixture of 95% oxygen and 5% carbon dioxide. The preparations were washed three times with Krebs-bicarbonate solution and tension adjusted to approximately 2 g, which then became the tissue baseline. A single dose of agonist was added to the U&LP and detrusor preparations after an incubation period with an antagonist or vehicle control. The concentrations specific for each receptor subtype were determined using affinity values appearing in the literature utilizing similar methodology and isolated tissue bath experiments. Tissue strips exposed to antagonists or vehicle controls were incubated for 30 min to allow full equilibration with the receptor. Each experiment was completed within 20 min after the addition of the agonist. Baseline tension, frequency and amplitude of spontaneous phasic contractions were recorded simultaneously using isometric force transducers (MCT050/D, ADInstruments, Castle Hill, NSW, Australia) on a PowerLab system using LabChart v7 software (ADInstruments). Porcine urinary bladders are a well-recognized animal model for bladder research (Cheng et al., 2011; Parsons et al., 2012; Mitsui et al., 2019; Stromberga et al., 2019) with similar physiology and pharmacology to human bladders. As no animals were bred, harmed, culled, interfered, or interacted with as part of this research project, Animal Ethics Approval was not required for bladder use (Queensland Government, 2016).
Pharmaceutical Agents
Prostaglandin E2, F2α, EP1 receptor antagonist SC-19220, EP1, and EP2 receptor antagonist AH6809, EP3 receptor antagonist L-798106, EP4 receptor antagonist AH23848, FP receptor antagonist AL-8810 and ATP receptor agonist α,β-methylene ATP were obtained from Cayman Chemicals, Ann Arbor, MI, United States. The muscarinic receptor antagonist atropine, cyclooxygenase (COX) inhibitor indomethacin, and the nitric oxide synthase inhibitor Nω-Nitro-L-arginine were obtained from Sigma Aldrich, St. Louis, MO, United States. Prostaglandin E2, prostaglandin F2α, AH6809 and indomethacin were dissolved in 100% ethanol and diluted as needed with distilled H2O. Atropine, Nω-Nitro-L-arginine, and α,β-methylene ATP were dissolved in distilled H2O and diluted as required. SC19220, L-798196, AH23848, and AL-8810 were dissolved in DMSO and diluted with distilled H2O.
Experimental Procedure
A single dose of prostaglandin E2 (1 or 10 μM) or prostaglandin F2α (10 μM) was applied to an isolated strip of U&LP or detrusor after a 30-minute equilibration period with a selective receptor antagonist. Baseline tensions and the amplitude and frequency of spontaneous phasic contractions of U&LP were measured before the application of the agonist and 5 min after addition. As spontaneous contractions were only observed in 21% of detrusor samples, only baseline tension was measured (before the application of the agonist, 10 min after and 20 min after).
Measurements and Statistical Analysis
The changes in the baseline tension were measured before the addition of an agonist and at the peak contractile response. The frequency of spontaneous contractions was measured over a period of 5 min and expressed as the number of spontaneous phasic contraction cycles per minute (cpm). The average amplitude was measured from base to peak of each spontaneous contraction. Measurements for frequency and amplitude were taken before any agonist was added and during the peak contractile response to the agonist. Data was graphed and analyzed using GraphPad Prism version 8.1.1 for Windows (GraphPad Software, La Jolla, CA, United States) and results shown as the mean change ± SEM. Responses between control and experimental tissues were compared using a Student’s t-test with p < 0.05 considered significant.
Results
Prostaglandin E2 on U&LP Preparations
In the absence of prostaglandin E2 or any receptor antagonists, strips of urothelium with lamina propria (U&LP) exhibited spontaneous phasic contractions at frequencies of 3.34 ± 0.06 cycles per min–1 (cpm) with amplitudes of 0.52 ± 0.02 g (n = 215 for both). In response to prostaglandin E2 (1 μM), U&LP baseline tensions increased by 91 ± 9% (n = 38, p < 0.001), the frequency of spontaneous phasic contractions increased by 39 ± 7% (n = 38, p < 0.001) and amplitudes decreased by 18 ± 5% (n = 38, p < 0.001) during the peak response (Table 1 and Figure 1). The addition of an even higher concentration of prostaglandin E2 (10 μM) caused increases in the U&LP baseline tensions by 106 ± 9% (n = 42, p < 0.001), frequencies of spontaneous phasic contractions by 40 ± 10% (n = 42, p < 0.01) and amplitude decreases by 28 ± 6% (n = 22, p < 0.001) during peak response (Table 1 and Figure 1).
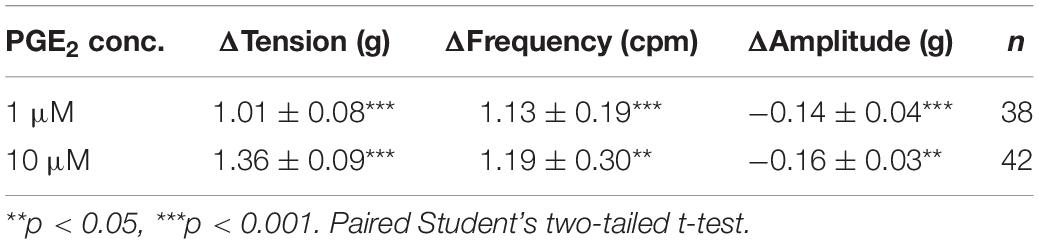
Table 1. U&LP effect of prostaglandin E2 (1 and 10 μM) on the baseline tension and on the frequency and amplitude of spontaneous phasic contractions (mean change ± SEM).
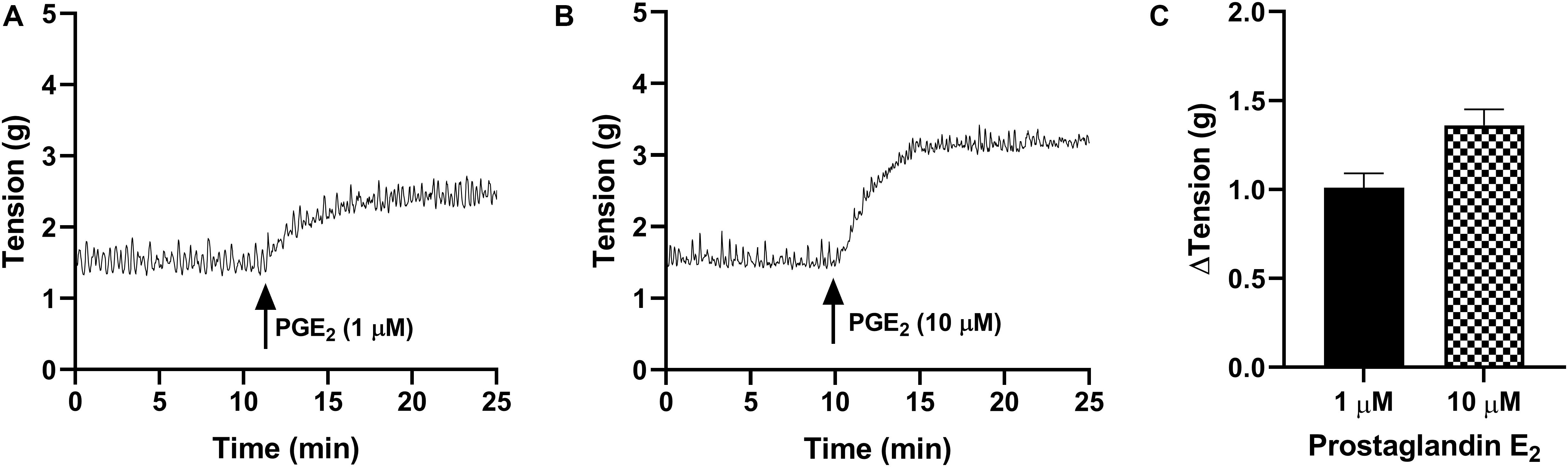
Figure 1. Traces showing changes to baseline tension of U&LP in response to PGE2 at concentrations of 1 μM (A) and 10 μM (B) over 15 min. (C) Peak responses after the addition of PGE2 (1 μM, n = 38) and PGE2 (10 μM, n = 42).
Prostaglandin E2 on Detrusor Preparations
In detrusor preparations, PGE2 caused concentration-dependent increases in baseline tension over the increasing concentrations (1–10 μM, Table 2 and Figure 2). Detrusor preparations did not reach a visible peak in response to PGE2 within the experimental timeframe, so each tension recording was completed at set intervals of 10 and 20 min. In response to prostaglandin E2 (1 μM, Table 2 and Figure 2), an increase of 80 ± 13% (n = 38, p < 0.001) was observed 10 min after the addition of the agonist, and in response to prostaglandin E2 (10 μM, Table 2 and Figure 2) an increase of 155 ± 21% (n = 34, p < 0.001) was observed 10 min after the addition of agonist. Twenty minutes after the addition of prostaglandin E2 (1 μM, Table 2), baseline tensions had increased by 107 ± 13% (n = 38, p < 0.001), whereas 20 min after the treatment with prostaglandin E2 (10 μM, Table 2), had increased by 175 ± 21% (n = 34, p < 0.001).
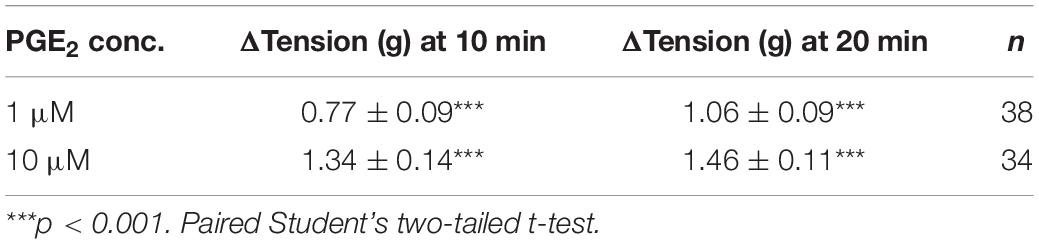
Table 2. Detrusor effect of prostaglandin E2 (1 and 10 μM) on the baseline tension and on the frequency and amplitude of spontaneous phasic contractions (mean change ± SEM).
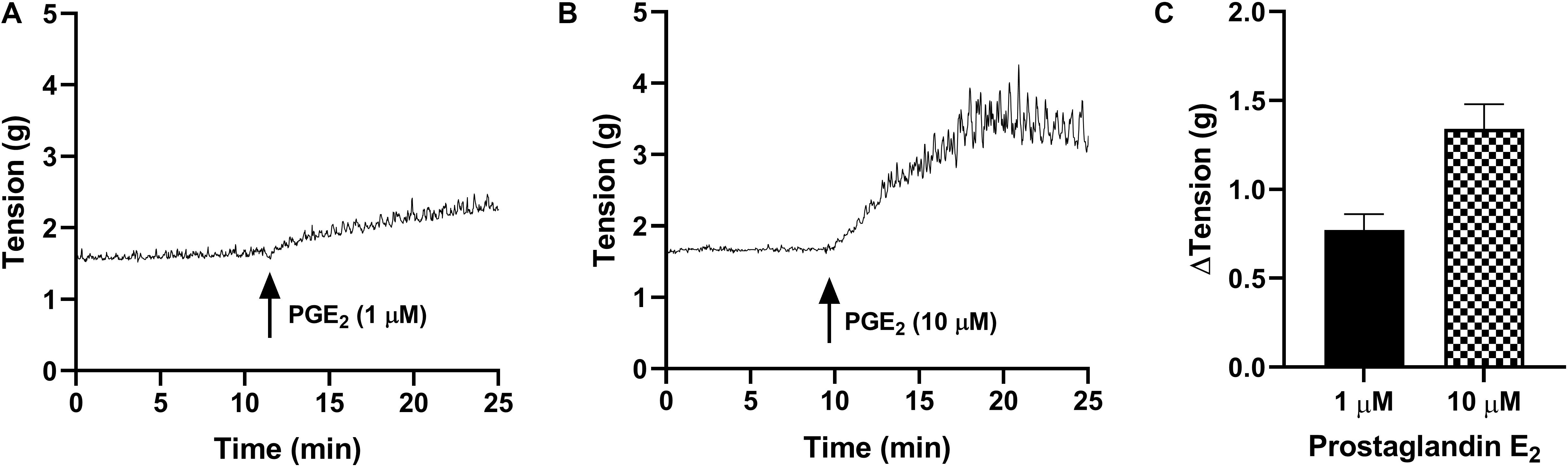
Figure 2. Traces showing changes to baseline tension of detrusor smooth muscle in response to PGE2 at concentrations of 1 μM (A) and 10 μM (B) over 15 min. (C) Peak responses after the addition of PGE2 (1 μM, n = 38) and PGE2 (10 μM, n = 34).
Only 21% of all detrusor preparations exhibited spontaneous phasic contractions at baseline and as such, the frequency and amplitude of spontaneous activity was not calculated. Of those that did not exhibit baseline activity, spontaneous phasic contractions were initiated after the addition of PGE2 in 83% of preparations.
Receptor-Selective Antagonists in U&LP Preparations
The responses to prostaglandin E2 were observed in the presence of the selective receptor antagonists: SC-19220 (EP1 antagonist, 10 μM), AH6809 (EP1/EP2 antagonist, 10 μM), L-798106 (EP3 antagonist, 100 nM) and AH23848 (EP4 antagonist, 10 μM). None of the prostaglandin E2 receptor antagonists had any effect on baseline tension, frequency or amplitude of spontaneous phasic contractions and they also had no effect on U&LP in response to prostaglandin E2 (1 μM, Table 3). The time required to reach peak baseline tension was investigated in U&LP tissues treated with PGE2 in the presence and absence of EP receptor antagonists. In response to prostaglandin E2 (1 μM), U&LP tissue strips took 4.48 ± 0.28 min to reach their peak baseline tensions. Tissues incubated with SC-19220 (1 μM, n = 8, p < 0.05) took 6.37 ± 0.39 min to reach peak. There were no significant differences in times taken to reach peak baseline tension in tissues treated with either AH6809, L-798106 or AH23848.

Table 3. Baseline tension and phasic contraction responses to U&LP treatment with prostaglandin E2 (1 μM) in the absence and presence of prostaglandin E2 receptor antagonists (mean change ± SEM).
The potential influence of receptor systems, other than prostaglandin E2, to contribute contractile responses to PGE2 were investigated. Neither the baseline tension, frequency nor amplitude of spontaneous phasic contractions in response to prostaglandin E2 (10 μM) were affected by the presence of the muscarinic receptor antagonist atropine (1 μM), cyclooxygenase (COX) inhibitor indomethacin (5 μM), nitric oxide synthase inhibitor Nω-Nitro-L-arginine (L-NNA, 100 μM) or P2X receptor desensitizing agonist α,β-methylene ATP (αβm-ATP, 10 μM, Table 4). The tissue did not exhibit changes in the tension or in the frequency of phasic contractions in response to the addition of the FP receptor antagonist AL-8810 (5 μM). However, the presence of AL-8810 (5 μM) significantly inhibited increases in the baseline tension in response to PGE2 (10 μM, n = 8, p < 0.05, Figure 3). In the absence of any antagonist, contractions to PGE2 increased the tension by 103 ± 12%. In the presence of AL-8810 (5 μM) this increase was inhibited with the remaining contraction being 77 ± 8% (n = 8, p < 0.05, Table 4).
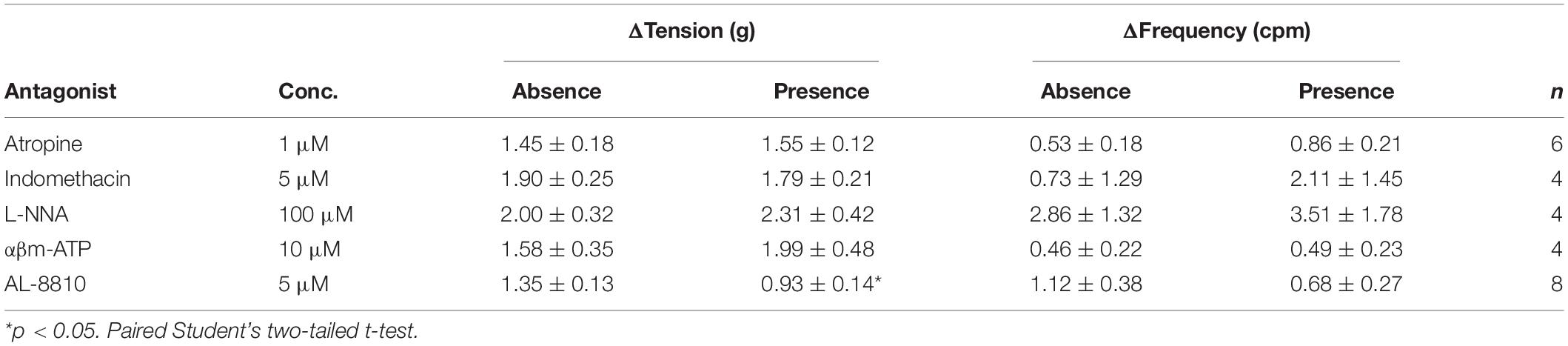
Table 4. U&LP responses to prostaglandin E2 (10 μM) in the absence and presence of atropine (muscarinic antagonist), indomethacin (cyclooxygenase antagonist), Nω-Nitro-L-arginine (L-NNA, nitric oxide antagonist), α,β-methylene ATP (αβm-ATP, ATP agonist), and AL-8810 (FP antagonist) (mean change ± SEM).
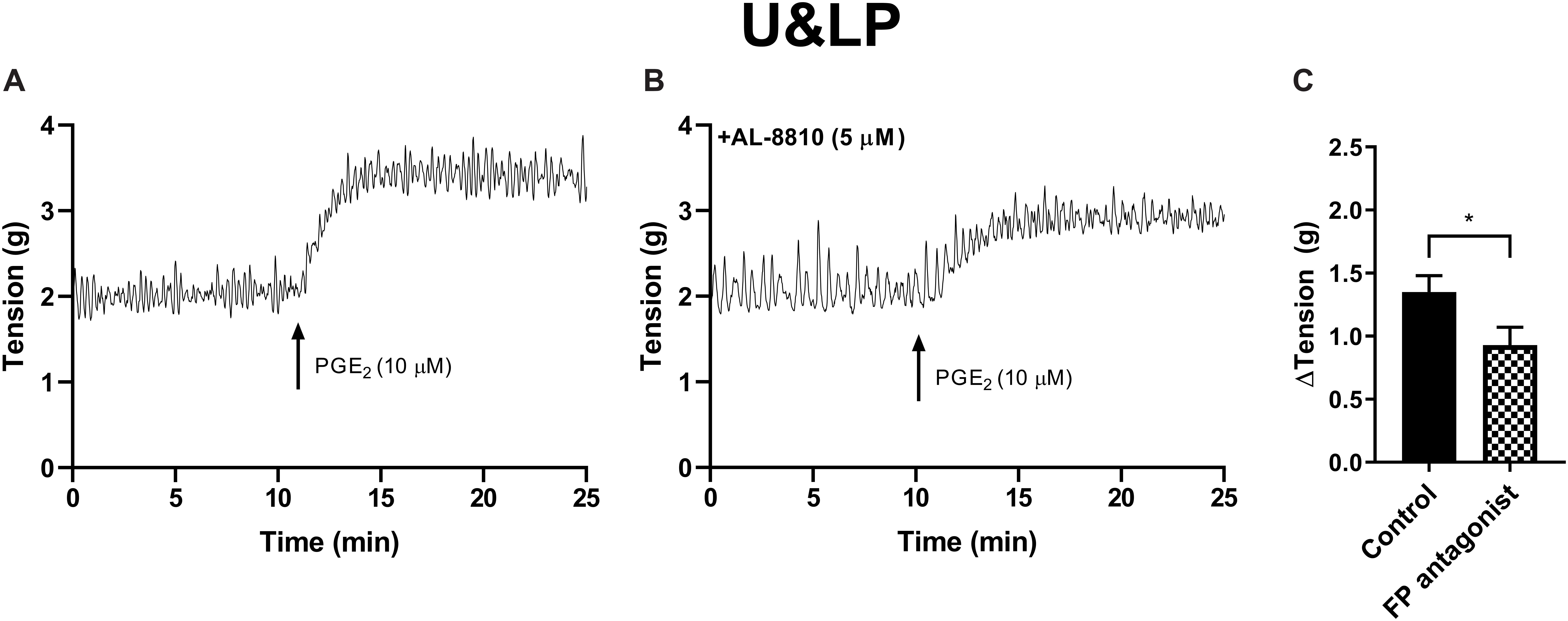
Figure 3. U&LP responses to PGE2 (10 μM) (A) and in the presence of FP receptor antagonist AL-8810 (5 μM) (B). Increases in the baseline tension in response to PGE2 are represented as mean change ± SEM (C). Changes in the baseline tension between control and experimental conditions were evaluated using a paired Student’s two tailed t-test, where *p < 0.05.
Receptor-Selective Antagonists in Detrusor Preparations
Detrusor preparations did not reach a visible peak in response to PGE2 (1 μM) within the experimental timeframe, so each tension recording was completed at set intervals of 10 and 20 min. As such, changes in detrusor tension were measured at 10 and 20 min after the addition of each agonist (Table 5). The responses to prostaglandin E2 were observed in the presence of the selective prostaglandin E2 receptor antagonists: SC-19220 (EP1 antagonist, 10 μM), AH6809 (EP1/EP2 antagonist, 10 μM), L-798106 (EP3 antagonist, 100 nM) and AH23848 (EP4 antagonist, 10 μM). None of the prostaglandin E2 receptor antagonists had any effect on baseline tension in detrusor tissue strips in response to prostaglandin E2 (1 μM).

Table 5. Detrusor responses to prostaglandin E2 (1 μM) at 10 and 20 min after the addition of agonist in the absence and presence of PGE2 receptor antagonists (mean change ± SEM).
The potential for receptor systems, other than prostaglandin E2, to contribute contractile responses to PGE2 were investigated. It was found that the baseline tension in detrusor preparations, in response to prostaglandin E2 (10 μM), was not affected by the presence of the muscarinic receptor antagonist atropine (1 μM), cyclooxygenase (COX) inhibitor indomethacin (5 μM), nitric oxide synthase inhibitor Nω-Nitro-L-arginine (L-NNA, 100 μM) or P2X receptor desensitizing agonist α,β-methylene ATP (αβm-ATP, 10 μM, Table 6). Treatment with the FP receptor antagonist AL-8810 (5 μM) did not affect baseline responses. In the presence of AL-8810 increases to baseline tension mediated by prostaglandin E2 (10 μM) were significantly inhibited both 10 and 20 min after the addition of the agonist (n = 8, p < 0.05 for both, Figure 4). In the absence of any antagonists, contractions to PGE2 increased the tension by 233 ± 60% at 10 min. In the presence of AL-8810 (5 μM) this increase was inhibited with the remaining contraction of 127 ± 46% (n = 8, p < 0.05, Table 6).
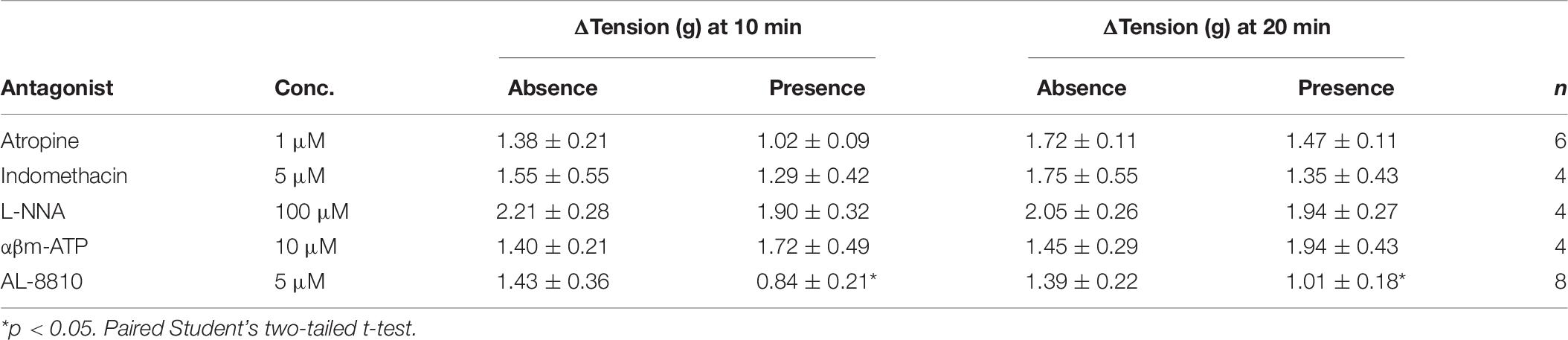
Table 6. Detrusor responses to prostaglandin E2 (10 μM) in the absence and presence of atropine (muscarinic antagonist), indomethacin (cyclooxygenase antagonist), Nω-Nitro-L-arginine (L-NNA, nitric oxide antagonist), α,β-methylene ATP (αβm-ATP, ATP agonist), and AL-8810 (FP antagonist) (mean change ± SEM).
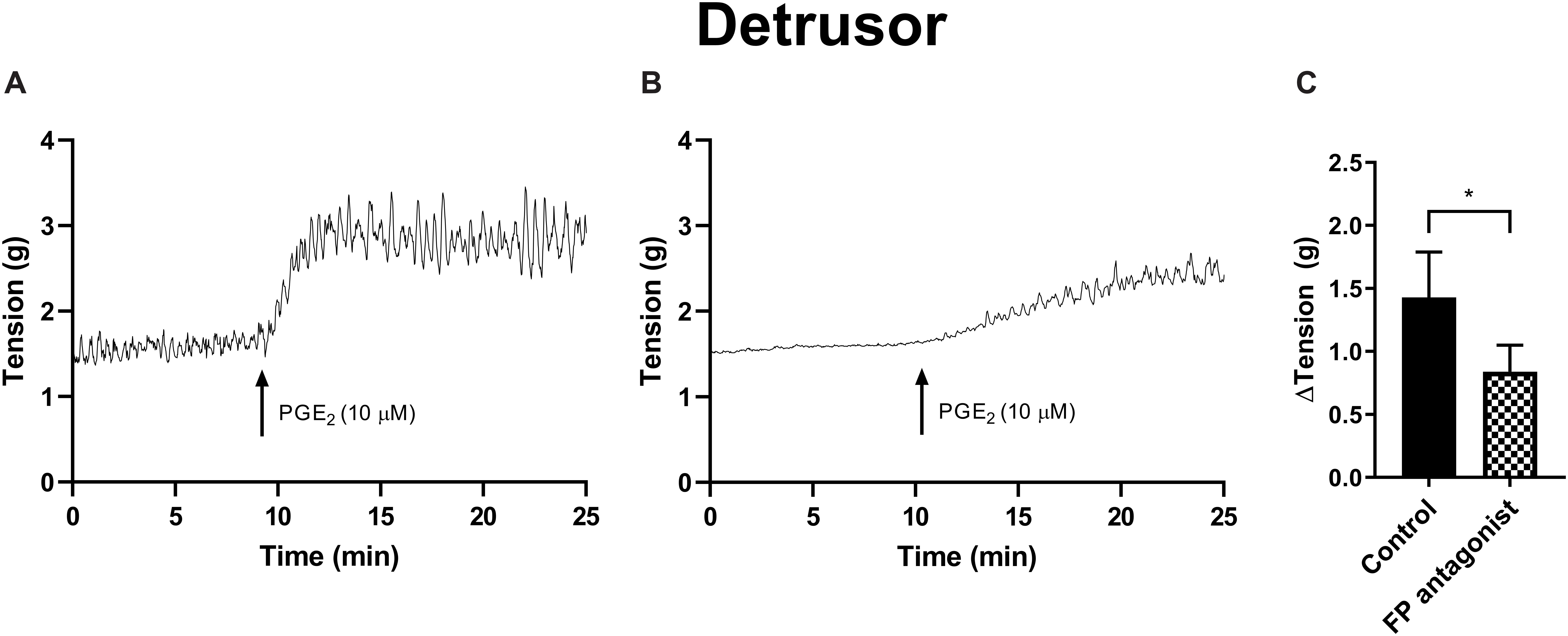
Figure 4. Detrusor responses to PGE2 (10 μM) (A) and in the presence of FP receptor antagonist AL-8810 (5 μM) (B). Increases in the baseline tension in response to PGE2 are represented as mean change ± SEM (C). Changes in the baseline tension between control and experimental conditions were evaluated using a paired Student’s two tailed t-test, where *p < 0.05.
Prostaglandin F2α on U&LP and Detrusor Preparations
In preparations of U&LP, treatment with PGF2α (10 μM) caused a significant increase in the baseline tension of 65 ± 9% (n = 14, p < 0.001). Additionally, the frequency of spontaneous phasic contractions increased by 13 ± 5% (n = 14, p < 0.05) with no changes observed in the amplitude of these contractions. In the presence of AL-8810 (FP antagonist, 5 μM), increases in the baseline tension in response to PGF2α (10 μM) were significantly inhibited (n = 8, p < 0.001, Table 7 and Figure 5). However, the antagonism of FP receptor did not affect the frequency or amplitude of spontaneous phasic contractions.
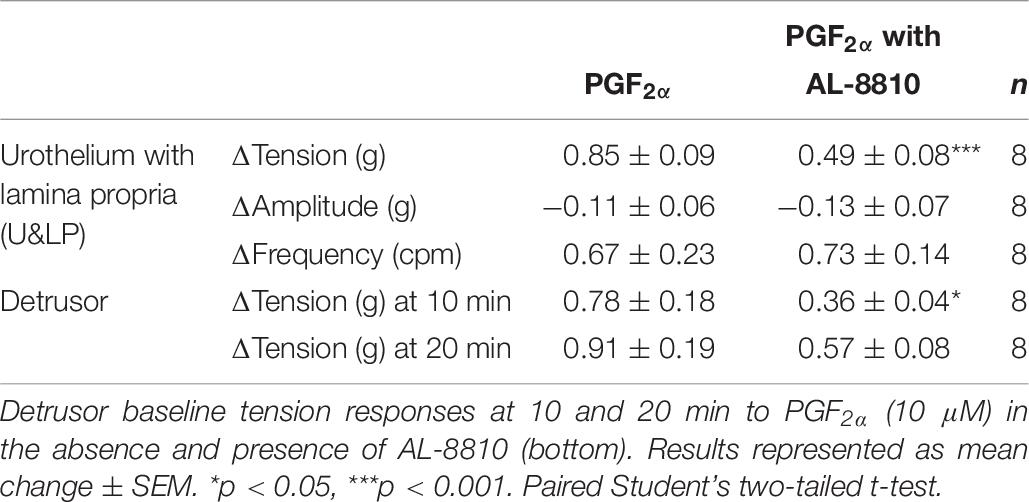
Table 7. U&LP responses to PGF2α (10 μM) in the absence and presence of AL-8810 on the baseline tension and on the frequency and amplitude of phasic contractions (top).
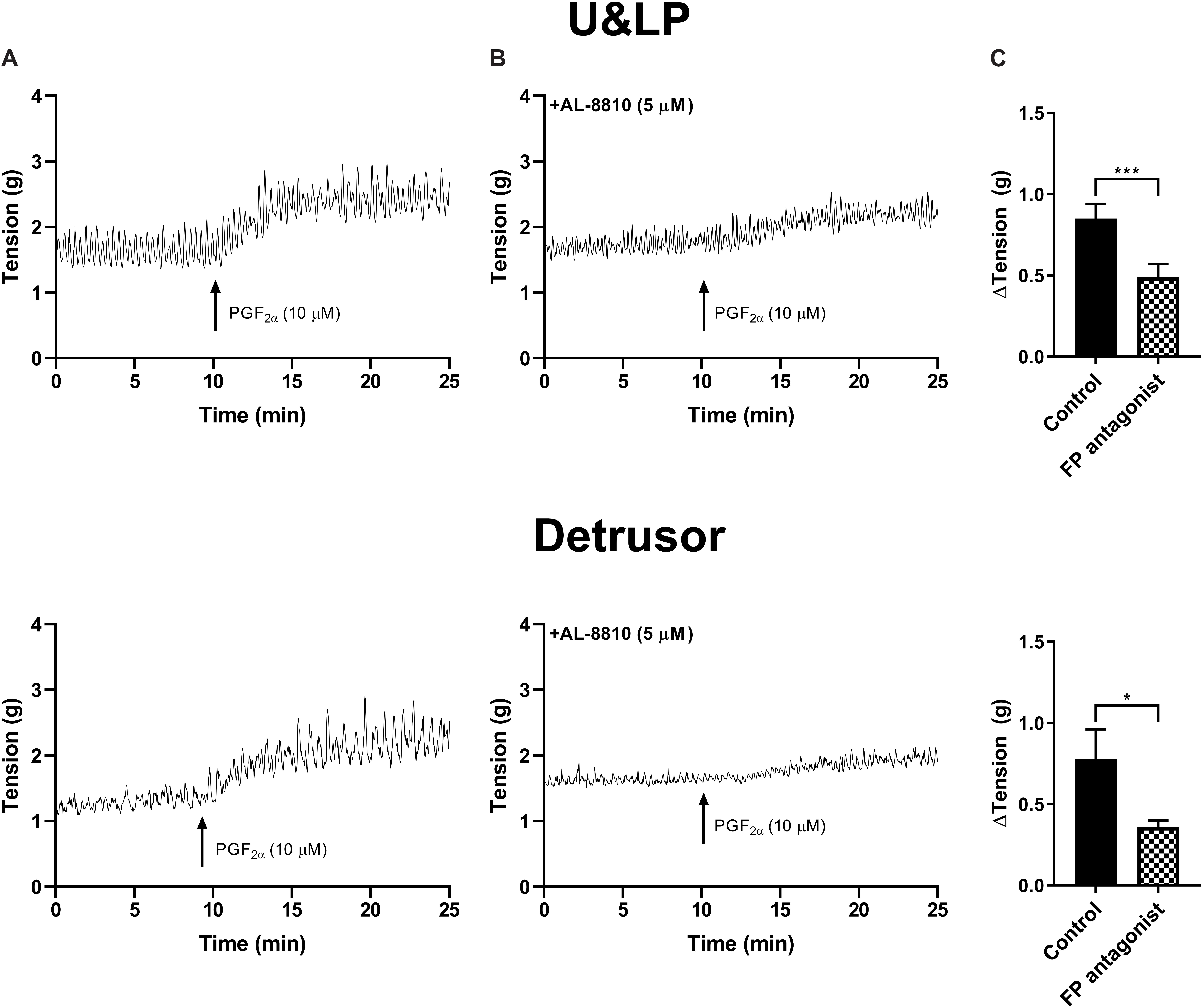
Figure 5. U&LP and detrusor responses to PGF2α (10 μM) (A) and in the presence of FP receptor antagonist AL-8810 (5 μM) (B). Increases in the baseline tension in response to PGF2α are represented as mean change ± SEM (C). Changes in the baseline tension between control and experimental conditions were evaluated using a paired Student’s two tailed t-test, where *p < 0.05, ***p < 0.001.
In detrusor preparations, an increase of 88 ± 10% (n = 12, p < 0.001) was observed in response to PGF2α (10 μM). The presence of FP antagonist AL-8810 (5 μM) significantly inhibited increases in the baseline tension (n = 8, p < 0.05). The inhibition was maintained both 10 and 20 min after the addition of PGF2α (10 μM, Table 7).
Discussion
This study presents prostaglandin E2 as a potential contributor to the overall urinary bladder contractility and showcases the ability of isolated urothelium with lamina propria (also called the bladder mucosa) to respond to this inflammatory mediator. Prostaglandin E2 causes a baseline tension increase in both U&LP and detrusor tissue, as well as influences the frequency and amplitude of U&LP spontaneous phasic contractions. In addition, this study is the first to identify responses to PGE2 in the urothelium with lamina propria when separated from its underlying detrusor layer. The two layers are distinct in both structure and function, with the U&LP comprized of several layers of epithelial cells and an underlying connective tissue layer with muscularis mucosae, while the detrusor is composed primarily of smooth muscle cells. The U&LP not only provides a physical barrier between urine and the bladder wall but is also capable of releasing chemicals like acetylcholine which can influence the contractility of the detrusor (Moro et al., 2011). Furthermore, it is also capable of responding to external signals like noradrenaline (Moro et al., 2013), nitric oxide (Moro et al., 2012), the five primary prostaglandins (Stromberga et al., 2020b), and histamine (Stromberga et al., 2019, 2020a), as well as release ATP (Andersson et al., 2018). It is known that cells found in U&LP can express both EP1 and EP2 receptors (Rahnama’i et al., 2010) indicating that they are capable of responding to PGE2 and eliciting a contractile response. However, the exact mechanisms and the involvement of the different PGE2 receptor subtypes in the contractile responses observed are still unclear.
In this study, increases in the baseline tension in both U&LP and detrusor tissues that were treated with two concentrations of PGE2 (1–10 μM) were observed. The presence of PGE2 increased the frequency of spontaneous contractile activity, which was similar to responses after muscarinic receptor activation (Moro et al., 2011), the target of many first-line pharmaceuticals to treat overactive bladder. In detrusor preparations, which generally do not exhibit spontaneous phasic contractions, these contractions were initiated in most preparations in response to the addition of PGE2. Previous studies have shown that contractions in detrusor smooth muscle in response to acetylcholine and ATP are enhanced by the presence of prostaglandins in both rabbit (Anderson, 1982) and guinea-pig animal models (Nile and Gillespie, 2012). There are complex interactions between these three molecules, as ATP enhances PGE2 release via a P2-purinoreceptor-mediated mechanism (Kasakov and Vlaskovska, 1985) and acetylcholine release has been detected when unstretched preparations were exposed to PGE2 (Nile and Gillespie, 2012). Thereby, interactions between these three mediators can potentially result in the amplification of molecule signaling and an increase in contractile activity. However, the involvement of acetylcholine, ATP and nitric oxide in mediating the increases in both the baseline tension and the frequency of spontaneous phasic contractions were ruled out as the contractile responses were the same when respective receptors were selectively antagonized or desensitized. Therefore, even though previous research has shown that acetylcholine is released when tissue is exposed to PGE2 (Nile and Gillespie, 2012), the inhibition of muscarinic receptors revealed no changes in the magnitude of contractions in response to PGE2. Furthermore, treatment with indomethacin, which inhibits prostaglandin production via cyclooxygenase (COX), did not affect contractile responses.
In order to isolate the role of a specific PGE2 receptor subtype in mediating these changes to baseline tension and the frequency of spontaneous phasic contractions, selective receptor antagonists for EP1–EP4 receptors were used. The antagonists and concentrations selected for this study were based on prior research investigating smooth muscle contractility in rat prostate gland (Tokanovic et al., 2010). When U&LP and detrusor smooth muscle tissues were treated with each individual PGE2 receptor subtype antagonists (EP1–EP4), there were no differences observed in the contractile responses to PGE2 when compared to tissues with no antagonist treatment. Activation of EP1 and EP3 receptor subtypes mediate contractions in smooth muscle, whereas EP2 and EP4 are involved in the relaxatory responses (Woodward et al., 2011). In this study, when both U&LP and detrusor tissues were treated with the highly selective EP1 receptor antagonist SC19220 with a pA2 value of approximately 5.9 (Jones et al., 2009) and EP3 receptor antagonist L798106 with a Ki of 0.3 nM (Juteau et al., 2001) there was no change in the magnitude of contractions to PGE2. However, when U&LP was incubated with the EP1-selective receptor antagonist SC-19220, it took significantly more time to reach peak contraction in response to PGE2 compared to control tissues. Even though the stimulation of EP2 receptors has previously been shown to relax non-vascular urogenital smooth muscle tissue (Coleman et al., 1994; Tokanovic et al., 2010), no changes in contractile response were observed in tissue preparations that were treated with the dual EP1 and EP2 antagonist AH6809 [pA for EP1 approximately 7.3 and pA2 for EP2 approximately 5.7 (Jones et al., 2009)]. The involvement of EP4 receptors in the inhibition of muscle contractions was also ruled out using AH23848, an antagonist for EP4 receptors (pA2 value 5.4) with no activity on other EP receptor subtypes (Coleman et al., 1994). The lack of responses also aligns with previous findings suggesting that the EP4 receptor is involved in vascular smooth muscle relaxation instead of smooth muscle of the urinary bladder (Coleman et al., 1994; Tokanovic et al., 2010). Findings of this study suggest that the contractile responses observed to prostaglandin E2 in both U&LP and detrusor may be mediated by receptors systems other than EP, purinergic and cholinergic.
The ability of AL-8810 to significantly inhibit the contractile response to PGE2 suggests a mechanism of action at the FP receptor. This is most likely a result of the PGE2 chemical undergoing a conversion from PGE2 into PGF2α through 9-ketoreductase activity upon contact with the tissue. The potential for these conversions has been known for some time (Canete Soler et al., 1987; Cheung and Challis, 1989; Abadir and Siragy, 2015). There is evidence to support a partial agonist effect of AL-8810 (Griffin et al., 1999). Although the addition of this antagonist showed no response, or any effect on the baseline activity. The contraction remaining after FP receptor inhibition is unknown, although does not appear to be related to stimulation of the muscarinic, purinergic or nitric oxide receptor systems, nor from the creation of new prostaglandins. One potential mechanism of action could be via a receptor unspecific pathway, such as affecting calcium channels, and this could be considered for future experiments.
A limitation of this study was the use of single-dose applications of PGE2 and PGF2α to examine changes in frequencies and amplitudes of phasic contractions over a 20-minute timeframe. It would be of interest to examine a larger range of concentrations, as well as incorporate alternative agonists to obtain a wider understanding of the prostaglandin responses. Finally, there remains no literature showing FP receptor expression or localization in porcine or any other animal model bladders, and its identification throughout this region would assist in a better understanding of this receptor system. It should also be considered that PGE2 exhibits a similar affinity to PGF2α on the FP receptor (Abramovitz et al., 2000), and as such, there is the potential for an influence of FP receptors in the responses observed. This cross-sensitivity of FP receptors to PGE2 may also present a mechanism underlying the inhibitory effect observed from treatment with AL-8810 and should be further explored in follow-up studies. Future studies could also investigate whether the suppressed responses observed after AL-8810 treatment is due to the actions of PGE2 after a possible conversion to PGF2α.
This study identifies a functional response in both U&LP and detrusor preparations to PGE2, and its ability to be inhibited by an FP receptor antagonist suggests some conversion to PGF2α upon contact with the tissue. The response of direct application of PGF2α which appears to be similar to the PGE2 response also supports this hypothesis. Thereby, the finding of this study supports and further advances prior research, where PGE2 and PGF2α had previously been found to contract isolated detrusor smooth muscle in human preparations (Abrams and Feneley, 1975; Andersson et al., 1977; Palea, 1998).
Conclusion
Prostaglandin E2 and prostaglandin F2α can elicit clear contractile responses in both urinary bladder urothelium with lamina propria, and detrusor smooth muscle preparations. Furthermore, the addition of these prostaglandins in U&LP preparations increases the frequency of spontaneous phasic contractions. In the detrusor, which normally does not exhibit spontaneous contractions, these contractions were initiated in most preparations. The antagonism of all four PGE2 receptor subtypes, cholinergic receptors and purinergic receptors failed to inhibit contractile responses observed to the prostaglandins in either U&LP or detrusor tissues. However, the inhibition of EP1 receptor in U&LP resulted in a significant decrease in the time taken to reach peak contractile responses to PGE2 suggesting partial mediation via the EP1 receptor system. Antagonism of the FP receptor inhibited responses to PGF2α, but also to PGE2, suggesting some conversion of this into PGF2α after contact with the tissue. Overall, contractile responses to both prostaglandin E2 and prostaglandin F2α appear mediated, in part, by the FP receptor in both U&LP and detrusor smooth muscle.
Data Availability Statement
The datasets generated for this study are available on request to the corresponding author.
Ethics Statement
Ethical review and approval was not required for the animal study because bladders were obtained from the local commercial abattoir after slaughter for the routine commercial provision of food. As no animals were bred, harmed, culled, interfered, or interacted with as part of this research project, Animal Ethics Approval was not required for bladder use (reference cited in section “Tissue Preparation”).
Author Contributions
ZS collected the data. All authors were all equally responsible for the study design, data analysis, preparation of manuscript, approved the final manuscript, and are accountable for all aspects of the work. All persons designated as authors qualify for authorship, and all those who qualify for authorship are listed.
Funding
This research was supported by the Australian Bladder Foundation managed by the Continence Foundation of Australia. ZS was supported by an Australian Government Research Training Program Scholarship.
Conflict of Interest
The authors declare that the research was conducted in the absence of any commercial or financial relationships that could be construed as a potential conflict of interest.
Abbreviations
ATP, adenosine triphosphate; OAB, overactive bladder; PGE2, prostaglandin E2; PGF2 α, prostaglandin F2 α; SEM, standard error of the mean; U&LP, lamina propria with urothelium; UAB, underactive bladder; αβ m-ATP, α β− methylene ATP.
References
Abadir, P. M., and Siragy, H. M. (2015). Angiotensin type 1 receptor mediates renal production and conversion of prostaglandins E2 to F2α in conscious diabetic rats. J. Renin. Angiotensin. Aldosterone Syst. 16, 774–779. doi: 10.1177/1470320315592566
Abramovitz, M., Adam, M., Boie, Y., Carriere, M., Denis, D., Godbout, C., et al. (2000). The utilization of recombinant prostanoid receptors to determine the affinities and selectivities of prostaglandins and related analogs. Biochim. Biophys. Acta 1483, 285–293. doi: 10.1016/s1388-1981(99)00164-x
Abrams, P. H., and Feneley, R. C. (1975). The actions of prostaglandins on the smooth muscle of the human urinary tract in vitro. Br. J. Urol. 47, 909–915. doi: 10.1111/j.1464-410x.1975.tb04075.x
Abrams, P. H., Sykes, J. A., Rose, A. J., and Rogers, A. F. (1979). The synthesis and release of prostaglandins by human urinary bladder muscle in vitro. Invest Urol. 16, 346–348.
Anderson, G. F. (1982). Evidence for a prostaglandin link in the purinergic activation of rabbit bladder smooth muscle. J. Pharmacol. Exp. Ther. 220, 347–352.
Andersson, K. E. (2010). Detrusor myocyte activity and afferent signaling. Neurourol. Urodyn. 29, 97–106. doi: 10.1002/nau.20784
Andersson, K. E., Ek, A., and Persson, C. G. (1977). Effects of prostaglandins on the isolated human bladder and urethra. Acta Physiol. Scand. 100, 165–171. doi: 10.1111/j.1748-1716.1977.tb05933.x
Andersson, K. E., Fry, C., Panicker, J., and Rademakers, K. (2018). Which molecular targets do we need to focus on to improve lower urinary tract dysfunction? ICI-RS 2017. Neurourol. Urodyn. 37, S117–S126.
Birder, L. A. (2006). Urinary bladder urothelium: molecular sensors of chemical/thermal/mechanical stimuli. Vascul Pharmacol 45, 221–226. doi: 10.1016/j.vph.2005.08.027
Bultitude, M. I., Hills, N. H., and Shuttleworth, K. E. D. (1976). Clinical and experimental studies on the action of prostaglandins and their synthesis inhibitors on detrusor muscle in vitro and in vivo. Br. J. Urol. 48, 631–637. doi: 10.1111/j.1464-410x.1976.tb06711.x
Canete Soler, R., Lopez Bernal, A., and Turnbull, A. C. (1987). Conversion of prostaglandin E2 to prostaglandin F2 alpha by human myometrium. Horm. Metab. Res. 19, 515–516. doi: 10.1055/s-2007-1011871
Cheng, Y., Mansfield, K., Sandow, S., Sadananda, P., Burcher, E., and Moore, K. (2011). Porcine bladder urothelial, myofibroblast, and detrusor muscle cells: characterization and ATP release. Front. Pharmacol. 2:27 doi: 10.3389/fphar.2011.00027
Cheung, P. Y., and Challis, J. R. (1989). Prostaglandin E2 metabolism in the human fetal membranes. Am. J. Obstet. Gynecol. 161, 1580–1585. doi: 10.1016/0002-9378(89)90930-7
Chuang, Y. C., Tyagi, P., Huang, C. C., Chancellor, M. B., and Yoshimura, N. (2012). Mechanisms and urodynamic effects of a potent and selective EP4 receptor antagonist, MF191, on cyclophosphamide and prostaglandin E2-induced bladder overactivity in rats. BJU Int. 110, 1558–1564. doi: 10.1111/j.1464-410x.2012.11096.x
Clark, J. D., Lin, L. L., Kriz, R. W., Ramesha, C. S., Sultzman, L. A., Lin, A. Y., et al. (1991). A novel arachidonic acid-selective cytosolic PLA2 contains a Ca(2+)-dependent translocation domain with homology to PKC and GAP. Cell 65, 1043–1051. doi: 10.1016/0092-8674(91)90556-e
Coleman, R. A., Smith, W. L., and Narumiya, S. (1994). International Union of Pharmacology classification of prostanoid receptors: properties, distribution, and structure of the receptors and their subtypes. Pharmacol. Rev. 46, 205–229.
D’ancona, C., Haylen, B., Oelke, M., Abranches-Monteiro, L., Arnold, E., Goldman, H., et al. (2019). The international continence society (ICS) report on the terminology for adult male lower urinary tract and pelvic floor symptoms and dysfunction. Neurourol. Urodyn. 38, 433–477. doi: 10.1002/nau.23897
De Groat, W. C., Nadelhaft, I., Milne, R. J., Booth, A. M., Morgan, C., and Thor, K. (1981). Organization of the sacral parasympathetic reflex pathways to the urinary bladder and large intestine. J. Auton. Nerv. Syst. 3, 135–160. doi: 10.1016/0165-1838(81)90059-x
De Jongh, R. (2007). The effects of exogenous prostaglandins and the identification of constitutive cyclooxygenase I and II immunoreactivity in the normal guinea pig bladder. BJU Int. 100, 419–429. doi: 10.1111/j.1464-410x.2007.07011.x
De Jongh, R. (2009). The localization of cyclo-oxygenase immuno-reactivity (COX I-IR) to the urothelium and to interstitial cells in the bladder wall. J. Cell. Mol. Med. 13, 3069–3081. doi: 10.1111/j.1582-4934.2008.00475.x
Ferguson, D. R., Kennedy, I., and Burton, T. J. (1997). ATP is released from rabbit urinary bladder epithelial cells by hydrostatic pressure changes–a possible sensory mechanism? J. Physiol. 505(Pt 2), 503–511. doi: 10.1111/j.1469-7793.1997.503bb.x
Fry, C. H., Sui, G. P., Kanai, A. J., and Wu, C. (2007). The function of suburothelial myofibroblasts in the bladder. Neurourol. Urodyn. 26, 914–919. doi: 10.1002/nau.20483
Fry, C. H., and Vahabi, B. (2016). The role of the mucosa in normal and abnormal bladder function. Basic Clin. Pharmacol. Toxicol. 119(Suppl. 3), 57–62. doi: 10.1111/bcpt.12626
Funk, C. D. (2001). Prostaglandins and leukotrienes: advances in eicosanoid biology. Science 294, 1871–1875. doi: 10.1126/science.294.5548.1871
Griffin, B. W., Klimko, P., Crider, J. Y., and Sharif, N. A. (1999). AL-8810: a novel prostaglandin F2 alpha analog with selective antagonist effects at the prostaglandin F2 alpha (FP) receptor. J. Pharmacol. Exp. Ther. 290, 1278–1284.
Haylen, B. T., De Ridder, D., Freeman, R. M., Swift, S. E., Berghmans, B., Lee, J., et al. (2010). An international urogynecological association (iuga)/international continence society (ICS) joint report on the terminology for female pelvic floor dysfunction. Neurourol. Urodyna. 29, 4–20.
Hegde, S. S., Choppin, A., Bonhaus, D., Briaud, S., Loeb, M., Moy, T. M., et al. (1997). Functional role of M2 and M3 muscarinic receptors in the urinary bladder of rats in vitro and in vivo. Br. J. Pharmacol. 120, 1409–1418.
Jeremy, J. Y., Mikhailidis, D. P., and Dandona, P. (1984). The rat urinary bladder produces prostacyclin as well as other prostaglandins. Prostaglandins Leukot. Med. 16, 235–248.
Jeremy, J. Y., Tsang, V., Mikhailidis, D. P., Rogers, H., Morgan, R. J., and Dandona, P. (1987). Eicosanoid synthesis by human urinary bladder mucosa: pathological implications. Br. J. Urol. 59, 36–39. doi: 10.1111/j.1464-410x.1987.tb04575.x
Jones, R. L., Giembycz, M. A., and Woodward, D. F. (2009). Prostanoid receptor antagonists: development strategies and therapeutic applications. Br. J. Pharmacol. 158, 104–145. doi: 10.1111/j.1476-5381.2009.00317.x
Juteau, H., Gareau, Y., Labelle, M., Sturino, C. F., Sawyer, N., Tremblay, N., et al. (2001). Structure-activity relationship of cinnamic acylsulfonamide analogues on the human EP3 prostanoid receptor. Bioorg. Med. Chem. 9, 1977–1984. doi: 10.1016/s0968-0896(01)00110-9
Kasakov, L. N., and Vlaskovska, M. V. (1985). Profile of prostaglandins generated in the detrusor muscle of rat urinary bladder: effects of adenosine triphosphate and adenosine. Eur. J. Pharmacol. 113, 431–436. doi: 10.1016/0014-2999(85)90092-5
Kim, J. C., Park, E. Y., Seo, S. I., Park, Y. H., and Hwang, T.-K. (2006). Nerve growth factor and prostaglandins in the urine of female patients with overactive bladder. J. Urol. 175, 1773–1776. doi: 10.1016/s0022-5347(05)00992-4
Leslie, C. A., Pavlakis, A. J., Wheeler, J. S., Siroky, M. B., and Krane, R. J. (1984). Release of arachidonate cascade products by the rabbit bladder: neurophysiological significance? J. Urol. 132, 376–379. doi: 10.1016/s0022-5347(17)49631-5
Maggi, C. A. (1988). Prostanoids modulate reflex micturition by acting through capsaicin-sensitive afferents. Eur. J. Pharmacol. 145, 105–112. doi: 10.1016/0014-2999(88)90221-x
Masick, J. M., Levin, R. M., and Hass, M. A. (2001). The effect of partial outlet obstruction on prostaglandin generation in the rabbit urinary bladder. Prostaglandins Other Lipid Mediat. 66, 211–219. doi: 10.1016/s0090-6980(01)00151-4
Masunaga, K., Yoshida, M., Inadome, A., Iwashita, H., Miyamae, K., and Ueda, S. (2006). Prostaglandin E2 release from isolated bladder strips in rats with spinal cord injury. Int. J. Urol. 13, 271–276. doi: 10.1111/j.1442-2042.2006.01274.x
Mccafferty, G. P., Misajet, B. A., Laping, N. J., Edwards, R. M., and Thorneloe, K. S. (2008). Enhanced bladder capacity and reduced prostaglandin E2-mediated bladder hyperactivity in EP3 receptor knockout mice. Am. J. Physiol. Renal Physiol. 295, F507–F514.
Milsom, I., Kaplan, S. A., Coyne, K. S., Sexton, C. C., and Kopp, Z. S. (2012). Effect of bothersome overactive bladder symptoms on health-related quality of life, anxiety, depression, and treatment seeking in the United States: results from EpiLUTS. Urology 80, 90–96. doi: 10.1016/j.urology.2012.04.004
Mitsui, R., Lee, K., Uchiyama, A., Hayakawa, S., Kinoshita, F., Kajioka, S., et al. (2019). Contractile elements and their sympathetic regulations in the pig urinary bladder: a species and regional comparative study. Cell Tissue Res. 379, 373–387. doi: 10.1007/s00441-019-03088-6
Moro, C., Leeds, C., and Chess-Williams, R. (2012). Contractile activity of the bladder urothelium/lamina propria and its regulation by nitric oxide. Eur. J. Pharmacol. 674, 445–449. doi: 10.1016/j.ejphar.2011.11.020
Moro, C., Tajouri, L., and Chess-Williams, R. (2013). Adrenoceptor function and expression in bladder urothelium and lamina propria. Urology 81:211.e1–e7.
Moro, C., Uchiyama, J., and Chess-Williams, R. (2011). Urothelial/lamina propria spontaneous activity and the role of M3 muscarinic receptors in mediating rate responses to stretch and carbachol. Urology 78:1442.e9–e15.
Nile, C. J., De Vente, J., and Gillespie, J. I. (2010). Stretch independent regulation of prostaglandin E2 production within the isolated guinea-pig lamina propria. BJU Int. 105, 540–548. doi: 10.1111/j.1464-410x.2009.08705.x
Nile, C. J., and Gillespie, J. I. (2012). Interactions between cholinergic and prostaglandin signaling elements in the urothelium: role for muscarinic type 2 receptors. Urology 79:240.e17-e23.
Palea, S. (1998). Pharmacological characterization of thromboxane and prostanoid receptors in human isolated urinary bladder. Br. J. Pharmacol. 124, 865–872. doi: 10.1038/sj.bjp.0701903
Park, J. M., Yang, T., Arend, L. J., Schnermann, J. B., Peters, C. A., Freeman, M. R., et al. (1999). Obstruction stimulates COX-2 expression in bladder smooth muscle cells via increased mechanical stretch. Am. J. Physiol. 276, F129–F136.
Parsons, B. A., Drake, M. J., Gammie, A., Fry, C. H., and Vahabi, B. (2012). The validation of a functional, isolated pig bladder model for physiological experimentation. Front. Pharmacol. 3:52.
Ponglowhapan, S., Church, D. B., and Khalid, M. (2010). Expression of prostaglandin E(2) receptor subtypes in the canine lower urinary tract varies according to the gonadal status and gender. Theriogenology 74, 1450–1466. doi: 10.1016/j.theriogenology.2010.06.017
Queensland Government (2016). Using Animals in Science. Available: https://www.business.qld.gov.au/industries/farms-fishing-forestry/agriculture/livestock/animal-welfare/animals-science/activities/dead-animals (accessed October 30, 2019).
Rahnama’i, M. S., Van Koeveringe, G. A., Essers, P. B., De Wachter, S. G., De Vente, J., Van Kerrebroeck, P. E., et al. (2010). Prostaglandin receptor EP1 and EP2 site in guinea pig bladder urothelium and lamina propria. J. Urol. 183, 1241–1247. doi: 10.1016/j.juro.2009.11.004
Reyes, A. A., and Klahr, S. (1990). Bladder contributes to eicosanoids excreted in urine. Am. J. Physiol. 259, F859–F861.
Root, J. A., Davey, D. A., and Af Forselles, K. J. (2015). Prostanoid receptors mediating contraction in rat, macaque and human bladder smooth muscle in vitro. Eur. J. Pharmacol. 769, 274–279. doi: 10.1016/j.ejphar.2015.11.030
Schmauder-Chock, E. A., and Chock, S. P. (1989). Localization of cyclo-oxygenase and prostaglandin E2 in the secretory granule of the mast cell. J. Histochem. Cytochem. 37, 1319–1328. doi: 10.1177/37.9.2504812
Schroder, A., Newgreen, D., and Andersson, K. E. (2004). Detrusor responses to prostaglandin E2 and bladder outlet obstruction in wild-type and Ep1 receptor knockout mice. J. Urol. 172, 1166–1170. doi: 10.1097/01.ju.0000134186.58854.2c
Sigala, S., Mirabella, G., Peroni, A., Pezzotti, G., Simeone, C., Spano, P., et al. (2002). Differential gene expression of cholinergic muscarinic receptor subtypes in male and female normal human urinary bladder. Urology 60, 719–725. doi: 10.1016/s0090-4295(02)01819-8
Stephenson, M. L., and Wing, D. A. (2015). A novel misoprostol delivery system for induction of labor: clinical utility and patient considerations. Drug Des Dev.Ther. 9, 2321–2327.
Stromberga, Z., Chess-Williams, R., and Moro, C. (2019). Histamine modulation of urinary bladder urothelium, lamina propria and detrusor contractile activity via H1 and H2 receptors. Sci. Rep. 9:3899.
Stromberga, Z., Chess-Williams, R., and Moro, C. (2020a). Alterations in histamine responses between juvenile and adult urinary bladder urothelium, lamina propria and detrusor tissues. Sci. Rep. 10:4116.
Stromberga, Z., Chess-Williams, R., and Moro, C. (2020b). The five primary prostaglandins stimulate contractions and phasic activity of the urinary bladder urothelium, lamina propria and detrusor. BMC Urol. 20:48. doi: 10.1186/s12894-020-00619-0
Sunil Kumar, K. S., Shyam, S., and Batakurki, P. (2016). Carboprost versus oxytocin for active management of third stage of labor: a prospective randomized control study. J. Obstetr. Gynaecol. India 66, 229–234. doi: 10.1007/s13224-016-0842-x
Tokanovic, S., White, C. W., Malone, D. T., Exintaris, B., and Ventura, S. (2010). Characterisation of the prostanoid receptor mediating inhibition of smooth muscle contractility in the rat prostate gland. Naunyn Schmiedebergs Arch. Pharmacol. 381, 321–328. doi: 10.1007/s00210-010-0492-y
Wheeler, M. A., Yoon, J. H., Olsson, L. E., and Weiss, R. M. (2001). Cyclooxygenase-2 protein and prostaglandin E(2) production are up-regulated in a rat bladder inflammation model. Eur. J. Pharmacol. 417, 239–248. doi: 10.1016/s0014-2999(01)00911-6
Keywords: inflammatory mediators, prostaglandins, prostaglandin antagonists, urinary bladder, overactive bladder, bladder inflammation, urothelium, mucosa
Citation: Stromberga Z, Chess-Williams R and Moro C (2020) Prostaglandin E2 and F2alpha Modulate Urinary Bladder Urothelium, Lamina Propria and Detrusor Contractility via the FP Receptor. Front. Physiol. 11:705. doi: 10.3389/fphys.2020.00705
Received: 26 November 2019; Accepted: 28 May 2020;
Published: 23 June 2020.
Edited by:
Bellamkonda K. Kishore, University of Utah Health, United StatesReviewed by:
Michael Winder, University of Gothenburg, SwedenWillmann Liang, The Chinese University of Hong Kong, China
Copyright © 2020 Stromberga, Chess-Williams and Moro. This is an open-access article distributed under the terms of the Creative Commons Attribution License (CC BY). The use, distribution or reproduction in other forums is permitted, provided the original author(s) and the copyright owner(s) are credited and that the original publication in this journal is cited, in accordance with accepted academic practice. No use, distribution or reproduction is permitted which does not comply with these terms.
*Correspondence: Christian Moro, cmoro@bond.edu.au