- 1Laboratório de Biomecânica, Centro de Desportos, Universidade Federal de Santa Catarina, Florianópolis, Brazil
- 2Laboratório de Pesquisa do Exercício, Escola de Educação Física, Fisioterapia e Dança, Universidade Federal do Rio Grande do Sul, Porto Alegre, Brazil
Purpose: The aim of this study was to verify whether physiological components [vertical jumps (Squat Jump – SJ and Countermovement Jump – CMJ), eccentric utilization ratio (EUR) of vertical jumps, running economy (RE), metabolic cost (CMET), first and second ventilatory threshold (VT1 and VT2) maximal oxygen uptake (VO2MAX)] can predict maximal endurance running performance.
Methods: Twenty male runners performed maximal vertical jumps, submaximal running at constant speeds, and maximal incremental running test. Before, we measured anthropometric parameters (body mass and height) and registered the training history and volume. SJ and CMJ tests were evaluated prior to running tests. Initially, the oxygen uptake (VO2) was collected at rest in the orthostatic position for 6 min. Soon after, a 10-min warm-up was performed on the treadmill at 10 km⋅h–1, followed by two 5-min treadmill rectangular tests at 12 and 16 km⋅h–1 monitored by a gas analyzer. After that, the runners performed a maximal incremental test, where the VT1, VT2, and VO2MAX were evaluated, as well as the maximum running speed (vVO2MAX). Thus, RE and CMET were calculated with data obtained during rectangular running tests. Multivariate stepwise regression analyses were conducted to measure the relationship between independent variables (height and power of SJ and CMJ, EUR; RE and CMET 12 and 16 km⋅h–1; VT1, VT2, and VO2MAX), as predictors of maximal running performance (vVO2MAX), with significance level at α = 0.05.
Results: We found that VO2MAX and RE at 16 km⋅h–1 predict 81% of performance (vVO2MAX) of endurance runners (p < 0.001).
Conclusion: The main predictors of the maximal incremental running test performance were VO2MAX and RE.
Introduction
Improvements of endurance running performance are based on improvements of the physiological predictors such as the maximal oxygen uptake (VO2MAX), running economy (RE) and metabolic thresholds (McLaughlin et al., 2010). The determinants of the endurance performance model demonstrate how the individual’s VO2MAX determines the upper limit of aerobic metabolism (Bassett and Howley, 2000). Therefore, VO2MAX has an important relationship with endurance running performance (McLaughlin et al., 2010). However, trained runners may have similar VO2MAX values and thus other physiological indexes can contribute for the success of predominantly aerobic events such as RE and lactate threshold (Kipp et al., 2019).
Running speed is then determined by how efficiently the corresponding oxidative adenosine triphosphate turnover at the fractional utilization of VO2MAX is converted to locomotion (i.e., RE) (Joyner and Coyle, 2008). Metabolic effectiveness refers to the utilization of available energy to provide optimal performance, whereas cardiopulmonary efficiency to least work output for the processes related to oxygen transport and utilization (Daniels, 1985; Saunders et al., 2004; Peyre-Tartaruga and Coertjens, 2018). Therefore, RE is an important physiological determinant for the endurance performance (Daniels, 1985; Kipp et al., 2019). Improvements in RE allow athletes to run at a faster velocity for the same oxygen uptake (VO2) and thus achieve superior performances (Hoogkamer et al., 2016, 2017). Accordingly, ∼1% improvements in RE should directly translate to ∼1% faster running 3000 m of running (Hoogkamer et al., 2016).
Another approach used to evaluate the metabolic economy in distance running is the metabolic cost (CMET) for running, which is independent of the speed during indoor tests (Arellano and Kram, 2014). Therefore, the amount of metabolic energy used to run a given distance is the same (Margaria et al., 1963; Arellano and Kram, 2014; Lacour and Bourdin, 2015). In addition, according to di Prampero et al. (1986), runners with higher VO2MAX (direct relation) and lower CMET (inverse relation), could present better performance. However, no study has been found to examine the relationship between CMET and the velocity at VO2MAX (vVO2MAX).
Furthermore, muscle strength or power are other important factor in predicting endurance running performance (Dumke et al., 2010), which can be assessed by performing maximal vertical jumps [squat jump (SJ) and countermovement jump (CMJ), more similar to the motor gesture performed during the race]. Previous research showed that improved strength and power results in better RE and performance (Balsalobre-Fernandez et al., 2016). This may occur probably by the changes in muscle power and tendon stiffness (Kubo et al., 2007). Therefore, the performance of vertical jumps (height and power), could be related to the maximum performance of runners.
Accordingly, relating all these physiological aspects to the maximum running performance can help coaches and runners understand which features can predict performance and decide whether it is important to offer time for training specific system adaptation during regular running training. Our goal was to verify the relationship between physiological parameters [VO2MAX, first and second ventilatory threshold (VT1 and VT2), RE, CMET and height and power of vertical jumps (SJ and CMJ) and eccentric utilization ratio (EUR) of vertical jumps] on prediction of maximal incremental running performance (maximum running speed - vVO2MAX). We hypothesized that the determinants of maximal incremental running performance were VO2MAX and lower RE.
Materials and Methods
Participants
Twenty male recreational runners, with ∼34 ± 8 years of age, participated in this study. Running experience was ∼5.5 years, and training volume was ∼63 ± 32 km/week. Before the selection interview, all procedures were presented to the participants, who signed a consent form to participate in the study that was approved by the local ethics committee (No. 2.437.616), according to the Declaration of Helsinki. Male runners with ages between 18 and 45 years, 2 years of running experience, training volume of at least 30 km/week and reaching a minimum speed of 19 km⋅h–1 in the incremental test were included in the present study. Participants were excluded if (1) they had any musculoskeletal injury of the lower and/or the upper limbs; (2) they had any contra-indications for maximal effort (cardiovascular, musculoskeletal, respiratory, or neurologic diseases); and (3) they had any difficulty in understanding and/or executing of the tests.
Experimental Design
Runners attended to one testing day in the laboratory. Anthropometric, maximal vertical jumps (SJ and CMJ), constant running speed, and maximal incremental running test [VO2 was measured] were performed (Figure 1).
Procedures
Anthropometry
Anthropometry was evaluated according to the International Society for Advancement of Kineanthropometry (Marfell-Jones et al., 2012).
Vertical Jumps (SJ and CMJ)
The runners performed the SJ and CMJ using a jumping mat (Jump System Pro®, CEFISE, Nova Odessa, Brazil), with sample rate of 1000 Hz. Each athlete performed three attempts of the SJ and CMJ with maximal effort. Thirty seconds between attempts were given to each runner. Both vertical jumps were performed according to the recommendations of Bosco et al. (1983). The runners were instructed to jump as high as possible, with their hands on their hips. The variables used in the data analysis were jump height (cm), power output (PO) and PO normalized by the body mass (W⋅kg–1). In addition, the eccentric utilization ratio (EUR) was calculated: EUR jump height [EUR-HJ (CMJ-SJ); (ΔCMJ-SJ); and (CMJ/SJ)] and EUR of peak power output [EUR-PO (CMJ-SJ); (ΔCMJ-SJ); (CMJ/SJ)]. Pre-stretch augmentation can be calculated as a percentage with percentage pre-stretch increase [EUR (ΔCMJ-SJ)]. Additional approach is to amount reactive strength [EUR (CMJ-SJ)], as described in McGuigan et al. (2006).
Running Economy and Metabolic Cost
All the participants underwent familiarization on a treadmill (Super ATL, Inbrasport-Inbramed, Porto Alegre, Brazil). The VO2 was measured breath by breath during the incremental test using an open-circuit indirect calorimetry system (Cosmed, Quark CPET, Rome, Italy). Initially, the VO2 was collected at rest in the orthostatic position for 6 min. Soon after, a 10-min warm-up was performed on the treadmill at 10 km⋅h–1, after which the constant speed running test was performed for 5 min at 12 and 16 km⋅h–1, with a 5-min interval between each test (Saunders et al., 2004). The treadmill velocity was calibrated before tests (Mocap System), by digitizing an adhesive retro-reflective marker on the tread belt as it traveled along the length of the treadmill. In addition, parameters including room temperature and humidity were measured using the same gas analyzer (Cosmed, Quark CPET, Rome, Italy). The VO2 curves from RE tests were analyzed using the software PFT ergo (Cosmed, Quark CPET, Rome, Italy), and the mean VO2 values were calculated and plotted at the last minute of each bout. RE was defined as the relationship between VO2 and the running velocity (Daniels, 1985). The metabolic power (WMET) was considered the difference between the VO2 measured during exercise and the VO2 at rest. Because the unit of measure used was Watts (W), this difference was multiplied by the energy coefficient (20.9 J⋅mL–1) and divided by the time in seconds (60 s). The CMET values relative to the speeds of 12 and 16 km⋅h–1 were calculated by dividing WMET by the speed in m⋅s–1 (di Prampero et al., 1986).
Maximum Incremental Test
The runners were submitted to a maximal incremental test (Figure 1). It started with an initial velocity of 10 km⋅h–1, and 1 km⋅h–1 was added per minute until exhaustion (Bentley et al., 2007). The VO2 obtained during the maximal incremental test was evaluated on the treadmill and followed a similar gas analysis as described above.
The VO2 analysis during the maximal incremental test was performed by visual inspection. VO2 values were plotted to exclude values with four standard deviations above or below the average of the movable windows of the whole curve—average of three breaths in each window (Fernandes et al., 2012). During the maximum incremental test, the vVO2MAX was obtained from the last completed stage, while the VO2MAX was determined as the highest value observed in the last test stage (Bentley et al., 2007). Also, gas exchange data were analyzed to define the VT1 and VT2, as described in Bentley et al. (2007).
Statistical Analysis
Data normality and homogeneity were assessed by the Shapiro-Wilk and Mauchly tests, respectively. Stepwise multiple linear regression method was used to estimate the relative contributions of independent variables [SJ (cm); PO-SJ (W); PO-SJr (W⋅kg–1); CMJ (cm); PO-CMJ (W); PO-CMJr (W⋅kg–1); EUR-HJ (CMJ-SJ); EUR-HJ (ΔCMJ-SJ); EUR-HJ (CMJ/SJ); EUR-PO (CMJ-SJ); EUR-PO (ΔCMJ-SJ); EUR-PO (CMJ/SJ); VO2MAX (ml⋅kg–1⋅min–1); VT2 and VT1 (ml⋅kg–1⋅min–1); RE 12 and 16 km⋅h–1 (ml⋅kg–1⋅min–1); CMET 12 and 16 km⋅h–1 (J⋅kg–1⋅m–1)] on the dependent variable of the performance [vVO2MAX (km⋅h–1)]. Our collinearity diagnostic exploration resulted in variance inflation factors of <2.0 and tolerance of 0.10–0.70, which indicate acceptable levels of multicollinearity of the variables (Dormann et al., 2012). Statistical analysis was performed with SPSS 20.0 for Windows, with a significance level of α = 0.05.
Results
Anthropometric, physiological, training characteristics and performance of runners are summarized in Table 1.
Table 2 shows the contribution of positive VO2MAX and negative RE 16 km⋅h–1 (81%) during incremental running test.
Figure 2 shows the contribution of positive VO2MAX and negative RE 16 km⋅h–1 (81%) during incremental running test.
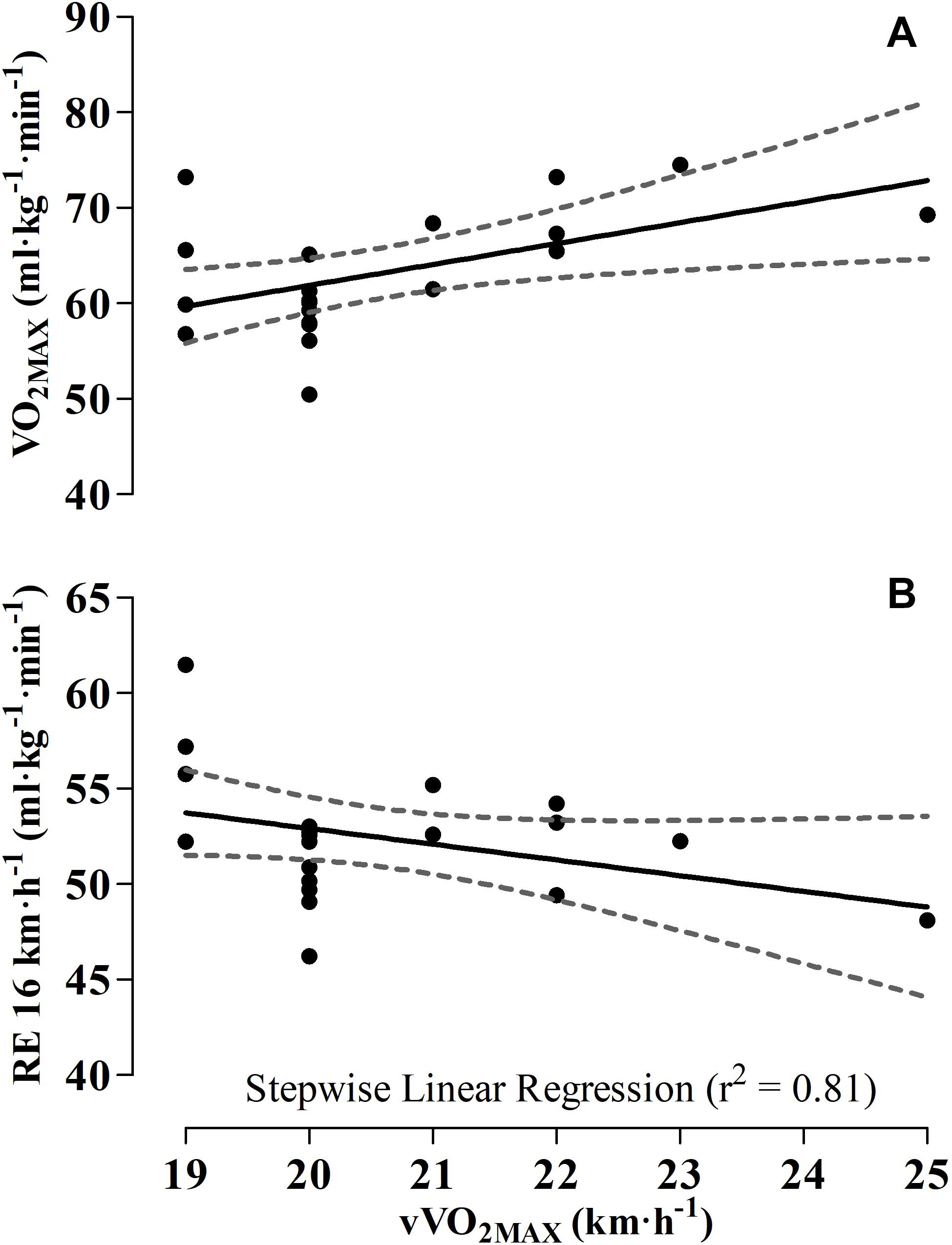
Figure 2. Predicted improvement maximal incremental of running performance (vVO2MAX) with VO2MAX (A) and RE (B).
Discussion
The purpose of this study was to investigate the role of physiological parameters on predicting vVO2MAX in recreational runners. The primary finding of this study is that the main determinants of running performance are VO2MAX and RE at 16 km⋅h–1. It is worth noting that the RE evaluated at speed close to vVO2MAX (16 km⋅h–1) was better related than RE at low speed (12 km⋅h–1). Importantly, this study examined, for the first time, the determinants of vVO2MAX in recreational runners, demonstrating that physiological but not neuromuscular factors were associated with performance in this condition.
Indeed, the VO2MAX and RE at 16 km⋅h–1 (Table 2 and Figure 2) accounted for 81% of the variance in performance of incremental running test (vVO2MAX). Conversely, the CMET, VT1, VT2, SJ and CMJ (height and power output) and EUR (height and power output) did not enter in the regression model to predict vVO2MAX. These results complement what have already been found in the literature as predictors of maximal endurance running performance. These results agree with previous studies, which indicate VO2MAX and RE, as two of the main predictors of running endurance performance (Conley and Krahenbuhl, 1980; Bassett and Howley, 2000; McLaughlin et al., 2010; Kipp et al., 2019). In general, the relationship between VO2MAX and running performance was similar to other studies using well-trained runners, confirming its importance as a physiological variable linked to distance running performance (Costill et al., 1973; Noakes et al., 1990; McLaughlin et al., 2010). According to McLaughlin et al. (2010), VO2MAX explained 81% of the total variance, and RE accounted for an additional 11% of the 16-km endurance running performance.
The running performance is related to better RE (e.g., a lower VO2 for a given running speed) may be worthwhile, especially in endurance events, once it will allow a lower fractional VO2MAX utilization for any submaximal intensity exercise (Daniels, 1985; Barnes and Kilding, 2015). Therefore, runners with the same VO2MAX can present different performances, and this can be explained in part by the RE, as demonstrated here. Results of the current study are in line with previous findings (Noakes et al., 1990; Tartaruga et al., 2012, 2014). For example, Noakes et al. (1990) found a strong correlation between performance and RE (r = 0.76–0.90), without regression test to identify the determination percentage between RE and running performance. Therefore, several physiological and biomechanical factors seem to influence RE in highly trained runners and must be taken into account to enable improvements in RE. These factors include metabolic adaptations within the muscle such as increased mitochondria and oxidative enzymes (Saunders et al., 2004), the ability of the muscles to store and release elastic energy by increasing the stiffness and other key parameters of elastic mechanism (da Rosa et al., 2019), more efficient mechanics leading to less energy wasted on braking forces and high vertical oscillation and stride frequency and stride length (Saunders et al., 2004; Tartaruga et al., 2009, 2012).
However, the multiple linear regression test showed no relationship between the CMET and the performance in a maximal incremental test. In addition to the expected collinearity between RE and CMET, the higher regression coefficient between RE and performance than between CMET and performance is probably due to the role of basal metabolism contributing to this relationship (Saibene and Minetti, 2003). Moreover, training with vertical jumps promotes strength and power and consequently can improve RE and performance (Kubo et al., 2007; Balsalobre-Fernandez et al., 2016). The results of the present study demonstrate that there is no relationship between the neuromuscular outcomes from vertical jumps (SJ and CMJ) and the maximum endurance running performance in recreational runners that did not performed previously strength and power training programs. Conversely, according to Loturco et al. (2015), the performance of vertical jumps (SJ and CMJ) had a strong correlation (r > 0.8) with the sprinting running performance (100 m). Thus, the use of variables from vertical jumps seems to be more suitable for predicting performance in sprints tests, but not for endurance tests. Collectively, the present findings confirm the role of maximal oxygen utilization and metabolic economy on the vVO2MAX (Billat et al., 2000).
Limitations
The main physiological limitation of the present study was that blood lactate was not evaluated during the maximal incremental running performance, that would allow to identify if the lactate threshold also and would determine endurance performance of the runners (Kipp et al., 2019). Another limitation of the present study was the fact that all evaluations were performed in a single day and, therefore, the last test performed (maximal incremental test) may have suffered interference from the a priori tests causing the establishment of processes of muscular fatigue. However, the athletes evaluated were well trained and an interval of 5 min was adopted between each condition, minimizing the effects of muscle fatigue. It is important to highlight that future studies should control for the following variables that were controlled in our study: runners’ age, experience, training volume and competitive level. The control of these variables reinforces the novel approach we used in this study.
Future Perspectives
Our analysis here focused solely on the oxygen uptake during maximal incremental running and vertical jumps (SJ and CMJ) performance. Therefore, future studies can evaluate the prediction of endurance running performance through the use of other physiological (e.g., skeletal muscle respiration), neuromuscular (e.g., muscle activation and size) and biomechanical (e.g., mechanics work and efficient) variables. Besides that, the decisive step will be an actual sport-setting test, such as during a running race (e.g., 3000 m), to identify which physiological, neuromuscular and biomechanical variables determine endurance running performance.
Practical Application
Understanding which variables can be predictors of running performance in a laboratory setting may contribute to athletes, coaches and sport scientists when determining physiological behaviors that may lead to the best performance, despite these predictors may not be the same for the outdoor or sports competition conditions, where other variables physiological and biomechanics may play an important role. These findings suggest that interventions that enhance VO2MAX and RE may increase the runners’ vVO2 and improve their running performance.
Conclusion
In summary, according to the outcomes presented in this study, it can be concluded that maximal aerobic performance prediction depends 81% on VO2MAX and RE at 16 km⋅h–1. However, we suggested that further studies should be carried out with these and other physiological and biomechanical variables to determine performance in ecological conditions (e.g., athletics track tests) or during endurance running competitions.
Data Availability Statement
The raw data supporting the conclusions of this article are available in Supplementary Material.
Ethics Statement
The studies involving human participants were reviewed and approved by Universidade Federal do Rio Grande do Sul (No. 2.437.616). The patients/participants provided their written informed consent to participate in this study.
Author Contributions
FL was responsible for the conception, acquisition of data, analysis and interpretation of data, drafting of the manuscript and revising it critically for important intellectual content, and final approval of the version to be submitted, and was the corresponding author. EM and GF were responsible for the acquisition of data and analysis, drafting of the manuscript and revising it critically for important intellectual content, and final approval of the version to be submitted. EM was responsible for the data analysis, critical revision of the manuscript, and final approval of the version to be submitted. LP-T was responsible for the data analysis and design of the study, study orientation, supervision, critical revision of the manuscript, and final approval of the version to be submitted. All authors contributed to the article and approved the submitted version.
Conflict of Interest
The authors declare that the research was conducted in the absence of any commercial or financial relationships that could be construed as a potential conflict of interest.
Acknowledgments
The authors wish to specially thank the athletes for their participation and cooperation in this study.
Supplementary Material
The Supplementary Material for this article can be found online at: https://www.frontiersin.org/articles/10.3389/fphys.2020.00979/full#supplementary-material
References
Arellano, C. J., and Kram, R. (2014). Partitioning the metabolic cost of human running: a task-by-task approach. Integr. Comp. Biol. 54, 1084–1098. doi: 10.1093/icb/icu033
Balsalobre-Fernandez, C., Santos-Concejero, J., and Grivas, G. V. (2016). Effects of strength training on running economy in highly trained runners: a systematic review with meta-analysis of controlled trials. J. Strength Cond. Res. 30, 2361–2368. doi: 10.1519/jsc.0000000000001316
Barnes, K. R., and Kilding, A. E. (2015). Running economy: measurement, norms, and determining factors. Sports Med. Open 1:8.
Bassett, D. R. Jr., and Howley, E. T. (2000). Limiting factors for maximum oxygen uptake and determinants of endurance performance. Med. Sci. Sports Exerc. 32, 70–84.
Bentley, D. J., Newell, J., and Bishop, D. (2007). Incremental exercise test design and analysis: implications for performance diagnostics in endurance athletes. Sports Med. 37, 575–586. doi: 10.2165/00007256-200737070-00002
Billat, V. L., Slawinski, J., Bocquet, V., Demarle, A., Lafitte, L., Chassaing, P., et al. (2000). Intermittent runs at the velocity associated with maximal oxygen uptake enables subjects to remain at maximal oxygen uptake for a longer time than intense but submaximal runs. Eur. J. Appl. Physiol. 81, 188–196. doi: 10.1007/s004210050029
Bosco, C., Mognoni, P., and Luhtanen, P. (1983). Relationship between isokinetic performance and ballistic movement. Eur. J. Appl. Physiol. Occup. Physiol. 51, 357–364. doi: 10.1007/bf00429072
Conley, D. L., and Krahenbuhl, G. S. (1980). Running economy and distance running performance of highly trained athletes. Med. Sci. Sports Exerc. 12, 357–360. doi: 10.1249/00005768-198012050-00010
Costill, D. L., Thomason, H., and Roberts, E. (1973). Fractional utilization of the aerobic capacity during distance running. Med. Sci. Sports 5, 248–252.
da Rosa, R. G., Oliveira, H. B., Gomenuka, N. A., Masiero, M. P. B., Da Silva, E. S., Zanardi, A. P. J., et al. (2019). Landing-takeoff asymmetries applied to running mechanics: a new perspective for performance. Front. Physiol. 10:415. doi: 10.3389/fphys.2019.00415
Daniels, J. T. (1985). A physiologist’s view of running economy. Med. Sci. Sports Exerc. 17, 332–338.
di Prampero, P. E., Atchou, G., Bruckner, J. C., and Moia, C. (1986). The energetics of endurance running. Eur. J. Appl. Physiol. Occup. Physiol. 55, 259–266. doi: 10.1007/bf02343797
Dormann, C. F., Elith, J., Bacher, S., Buchmann, C., Carl, G., Carré, G., et al. (2012). Collinearity: a review of methods to deal with it and a simulation study evaluating their performance. Ecography 36, 27–46. doi: 10.1111/j.1600-0587.2012.07348.x
Dumke, C. L., Pfaffenroth, C. M., Mcbride, J. M., and Mccauley, G. O. (2010). Relationship between muscle strength, power and stiffness and running economy in trained male runners. Int. J. Sports Physiol. Perform. 5, 249–261. doi: 10.1123/ijspp.5.2.249
Fernandes, R. J., De Jesus, K., Baldari, C., Sousa, A. C., Vilas-Boas, J. P., and Guidetti, L. (2012). Different VO2max time-averaging intervals in swimming. Int. J. Sports Med. 33, 1010–1015. doi: 10.1055/s-0032-1316362
Hoogkamer, W., Kipp, S., Spiering, B. A., and Kram, R. (2016). Altered running economy directly translates to altered distance-running performance. Med. Sci. Sports Exerc. 48, 2175–2180. doi: 10.1249/mss.0000000000001012
Hoogkamer, W., Kram, R., and Arellano, C. J. (2017). How Biomechanical Improvements in Running Economy Could Break the 2-hour Marathon Barrier. Sports Med. 47, 1739–1750. doi: 10.1007/s40279-017-0708-0
Joyner, M. J., and Coyle, E. F. (2008). Endurance exercise performance: the physiology of champions. J. Physiol. 586, 35–44. doi: 10.1113/jphysiol.2007.143834
Kipp, S., Kram, R., and Hoogkamer, W. (2019). Extrapolating metabolic savings in running: implications for performance predictions. Front. Physiol. 10:79. doi: 10.3389/fphys.2019.00079
Kubo, K., Morimoto, M., Komuro, T., Yata, H., Tsunoda, N., Kanehisa, H., et al. (2007). Effects of plyometric and weight training on muscle-tendon complex and jump performance. Med. Sci. Sports Exerc. 39, 1801–1810.
Lacour, J. R., and Bourdin, M. (2015). Factors affecting the energy cost of level running at submaximal speed. Eur. J. Appl. Physiol. 115, 651–673. doi: 10.1007/s00421-015-3115-y
Loturco, I., Pereira, L. A., Cal Abad, C. C., D’angelo, R. A., Fernandes, V., Kitamura, K., et al. (2015). Vertical and Horizontal Jump Tests Are Strongly Associated With Competitive Performance in 100-m Dash Events. J. Strength Cond. Res. 29, 1966–1971.
Marfell-Jones, M. J., Stewart, A. D., and De Ridder, J. H. (2012). International Standards for Anthropometric Assessment. Wellington: ISAK.
Margaria, R., Cerretelli, P., Aghemo, P., and Sassi, G. (1963). Energy cost of running. J. Appl. Physiol. 18, 367–370.
McGuigan, M. R., Doyle, T. L., Newton, M., Edwards, D. J., Nimphius, S., and Newton, R. U. (2006). Eccentric utilization ratio: effect of sport and phase of training. J. Strength Cond. Res. 20, 992–995.
McLaughlin, J. E., Howley, E. T., Bassett, D. R. Jr., Thompson, D. L., and Fitzhugh, E. C. (2010). Test of the classic model for predicting endurance running performance. Med. Sci. Sports Exerc. 42, 991–997. doi: 10.1249/mss.0b013e3181c0669d
Noakes, T. D., Myburgh, K. H., and Schall, R. (1990). Peak treadmill running velocity during the VO2 max test predicts running performance. J. Sports Sci. 8, 35–45. doi: 10.1080/02640419008732129
Peyre-Tartaruga, L. A., and Coertjens, M. (2018). Locomotion as a powerful model to study integrative physiology: efficiency. Economy, and power relationship. Front. Physiol. 9:1789. doi: 10.3389/fphys.2018.01789
Saibene, F., and Minetti, A. E. (2003). Biomechanical and physiological aspects of legged locomotion in humans. Eur. J. Appl. Physiol. 88, 297–316. doi: 10.1007/s00421-002-0654-9
Saunders, P. U., Pyne, D. B., Telford, R. D., and Hawley, J. A. (2004). Factors affecting running economy in trained distance runners. Sports Med. 34, 465–485. doi: 10.2165/00007256-200434070-00005
Tartaruga, M. P., Brisswalter, J., Peyre-Tartaruga, L. A., Avila, A. O., Alberton, C. L., Coertjens, M., et al. (2012). The relationship between running economy and biomechanical variables in distance runners. Res. Q. Exerc. Sport 83, 367–375.
Tartaruga, M. P., Mota, C. B., Peyre-Tartaruga, L. A., and Brisswalter, J. (2014). Scale model on performance prediction in recreational and elite endurance runners. Int. J. Sports Physiol. Perform. 9, 650–655. doi: 10.1123/ijspp.2013-0165
Keywords: runners, incremental test, VO2MAX, running economy, metabolic cost
Citation: Lanferdini FJ, Silva ES, Machado E, Fischer G and Peyré-Tartaruga LA (2020) Physiological Predictors of Maximal Incremental Running Performance. Front. Physiol. 11:979. doi: 10.3389/fphys.2020.00979
Received: 27 June 2020; Accepted: 20 July 2020;
Published: 05 August 2020.
Edited by:
Hassane Zouhal, University of Rennes 2 – Upper Brittany, FranceReviewed by:
Johnny Padulo, University of Milan, ItalyBeat Knechtle, University Hospital Zurich, Switzerland
Copyright © 2020 Lanferdini, Silva, Machado, Fischer and Peyré-Tartaruga. This is an open-access article distributed under the terms of the Creative Commons Attribution License (CC BY). The use, distribution or reproduction in other forums is permitted, provided the original author(s) and the copyright owner(s) are credited and that the original publication in this journal is cited, in accordance with accepted academic practice. No use, distribution or reproduction is permitted which does not comply with these terms.
*Correspondence: Fábio J. Lanferdini, fabiolanferdini@gmail.com