- 1Mekong Region Futures Institute (MERFI), Bangkok, Thailand
- 2School of Life and Environmental Sciences, Deakin University, Melbourne, VIC, Australia
- 3Department of Environmental Conservation and School of Public Policy, University of Massachusetts Amherst, Amherst, MA, United States
Despite significant efforts over many decades, humanity faces daunting challenges in the governance, management and sustainability of natural resources. Perhaps the most obvious is our global inability to collectively act and control or reduce greenhouse gases that are warming the planet. Another example, occurring at finer geographic scales, is the overuse of groundwater aquifers. Institutions—defined in Political Science and Economics as formal and informal rules that guide and incentivize socio-economic activities—are humanity’s general approach toward addressing these and other environmental challenges. Institutional arrangements typically specify whom they apply to, under what circumstances, and what penalty the breaking of the rule involves. Effective institutional design requires the ability to properly incentivize human behavior in the context of socio-economic systems, and establish systems to monitor behavior and sanction when rules are broken. From time to time, technological advances come along that complement institutional designs and improve our ability to incentivize and monitor behavior. We believe that the invention of Blockchain or Distributed Ledger technology—increasingly touted as the beginning of the fourth industrial revolution–could provide new ways to incentivize behavior of resource users, establish innovative monitoring capacity, and help to avoid corrupt governmental behavior. In this Perspective article, we summarize Proof-of-Stake Blockchain technology and provide two examples—deforestation and groundwater management—to describe how this new revolution could provide new solutions for the sustainable management of natural resources at local to global scales.
Introduction
Commons scholar Elinor Ostrom and others understood that advancing environmental sustainability requires improved diagnostic capacity (North, 1990; Ostrom, et al., 1993; Ostrom, 2005; Young, 2018) as well as an evolutionary understanding of institutional dynamics (Williamson, 2000; Aoki, 2001; Bromley, 2006). In parallel, it is also well understood that new technological developments often create opportunities where new technology and institutional designs can intermix to advance sustainable natural resource management. Consider, for example, the innovations in water market trading created by the technological advances of the Internet and World Wide Web (Bjornlund, 2003).
Scholars predict that blockchain technology will have transformative implications on the prevailing socio-economic system, including various aspects of governance (Atzori, 2015; Schwab, 2016; Allen, et al., 2017), shifting boundary constraints for solutions developed in the fields of institutional economics, political science, and sustainability. In other words, blockchain technology provides mechanisms to alter the effectiveness of institutional arrangements concerned with the sustainable natural resource management at local, regional and global scales—the core argument in this Perspective article.
Blockchain fundamentals
Our perspective is focused on how blockchain technology in combination with new institutional arrangements can be used for good in the context of environmental sustainability. Readers undoubtedly recognize Blockchain’s three interlinked components:
1) a peer-to-peer network of computers (nodes), that contribute processing resources, where each node participates with a unique public key and executes specific individual transactions (bundled in “blocks”) with a corresponding private key (Nakamoto, 2008);
2) a decentralized public ledger database of the unalterable transactions or “blocks” that is monitored by all in the network and is not centrally controlled (Pazaitisa et al., 2017); and,
3) a consensus algorithm (also known as a protocol) that defines how groups of nodes confirm transactions, which provides the essential security framework for the ledger (Karaindrou, 2017).
Readers will also understand that the invention of blockchain allowed cryptocurrencies like Bitcoin to be created through a software-enabled consensus process called “mining” that generates a digital coin or token. Individual or decentralized computers can mine Bitcoins, but it requires the network, through the distributed ledger, to approve this new coin or “token”.
Energy consumption problem connected to “Proof of Work” mining (CBECI, 2022) has led to a shift to the alternative consensus approach called “Proof of Stake” (PoS)1, which is an important part of the Blockchain for Good idea we propose below. The PoS approach can involve pre-minted tokens, which means that the total supply of all tokens is created when the blockchain starts, and in that case, no major energy demands are required (Howson, 2019).
Blockchain participant nodes in PoS applications are -- referred to as “validators”, not miners—need to establish a stake in the system by holding some number of tokens in their node’s ‘wallet’ (Saleh, 2021). PoS systems assign block validation tasks to nodes based on the proportion of stake they have in their wallets. Upon receiving their assignment, actors in a node validate their assigned block transactions, and after posting their block to the public ledger, receive a transaction fee in the form of tokens or some percentage of a token added to their wallet for their efforts.
Improved sustainability through PoS blockchain-based incentives, monitoring and decentralized design
For effectively applying blockchain for environmental sustainability, three dimensions are at the core of merging the technological innovation of blockchains and the design of institutional arrangements:
1) The ability to directly incentivise the behaviour of resource users;
2) The utilisation of innovative monitoring capacity to establish an effective reward/penalty system and improve compliance; and,
3) The mitigation of centralised power and related incentives that promote behaviour inconsistent with sustainable development objectives (coercion and corruption) through a decentralized design.
Incentives. Blockchain solutions can provide directly payments to resource users for socially desirable behaviour, such as planting trees or using less water. The blockchain would analyse relevant monitoring data (see below) and automatically transfer payments to the individual behaving sustainably. Most other incentive mechanisms in the sustainability context have indirect effects, such as REDD through government agencies distributing funds, rendering the incentive as perceived by the individual often meaningless. Various emerging blockchain projects aim to incentivise particular behaviour. For instance, emerging social media platforms like Steemit and Sapien use blockchain-based technology to encourage the production of high-quality content on social media. This incentive unfolds as readers ‘like’ certain posts and each ‘like’ triggers a transfer of the platform specific token. As aforementioned, some coins/tokens can directly be spent for consumption (as accepted by merchants), or be converted into fiat currencies and transferred to traditional bank accounts. Consequently, the blockchain-based incentive converts into real economic incentives. Automotive companies like Daimler (the parent company for Mercedes Benz) provide another example. They are experimenting with blockchain-based cryptocurrency mobiCOINS to incentivize drivers to operate their automobiles in more environmentally sensitive ways. These projects and others demonstrate the potential utility of blockchain-based cryptocurrency systems to provide individuals or households with a payment for a desired behaviour. In the context of natural resource or ecosystem management, unsustainable outcomes emerge due to a lack of effective incentives (Costanza et al., 1997; Smajgl et al., 2015a). If, for instance, prices for a particular cash crop increase, the pressure on remaining conservation forests and wetlands also increases. External costs are difficult to introduce to this calculation (Kandulu et al., 2014; Smajgl et al., 2015b). As we will describe below, the advance of blockchain technologies provide new opportunities to incentivize behaviour toward activities that help to sustain natural resources.
Monitoring is a critical aspect for ensuring compliance in institutional arrangements. Many such arrangements fail to achieve sustainable development objectives because of the lack of monitoring at the relevant scale (Ostrom, 2009). Recent advances in remote sensing technologies combined with pattern recognition software to distinguish different forest types provide an effective monitoring mechanism of, for example, forest/canopy cover to audit the outcomes of actual forest related behaviour (Cord et al., 2017; Campos et al., 2018). Such data and algorithms can be integrated in blockchain designs to develop incentives based on actual observation. Such a blockchain integrated monitoring solution has several advantages over traditional governance approaches, including that the link between behavioural changes on the ground (e.g. reforestation) and the confirmation of payments is fully automated, which causes in traditional approaches substantial (or even prohibitive) overheads in government agencies.
Decentralization. The provision of economic incentives through centralised power structures without effective monitoring and enforcement has the tendency to involve coercion and corruption, which leads to unsustainable outcomes (Meppem, 2000; Ewers and Smith, 2007). Scholars argue that the decentralisation of economic incentives can circumvent power related risks (Hughes, 2017; Kshetri, 2017). The main decentralisation advantage of blockchain technology would be that payments for incentivised behaviour would be automatically transferred to the relevant individual to reward the desired change (based on aforementioned monitoring) without involving any middle-people responsible for validating behavioural changes and making payments. The latter can be the basis for corruption and coercion. There are already examples of blockchain based technologies that are providing decentralized services and markets (Swan, 2015; Allen et al., 2017).
The effective combination of these three elements—incentives, monitoring and decentralisation—is a necessary prerequisite for blockchain mechanisms to contribute toward the sustainable management of natural resources. In the next section, we provide an example to in the context of reducing global deforestation to clarify this vision. Similar solutions for sustainability-focused blockchains include the Regen Network, first published in 2017 and has since been implemented with various partners, including The Nature Conservancy, and the Yale OpenLab Project (Open Climate Platform), which was developed to track the global carbon budget and monitors how country actions compare to national reduction targets.
A blockchain-based approach to incentivize forest conservation and reforestation
In this Section we present a blockchain-based approach that presents land managers with incentivising payments to create new or maintain existing forests (Smajgl, 2016; Smajgl and Damen 2020). Deforestation is a key driver for the decline in global greenhouse gas mitigation potential (Smajgl and Bohensky, 2012). Several global efforts have aimed at stabilising the extent of forests as carbon sinks or even reversing the trend in forest loss, including the UN/World Bank coordinated REDD and the REDD + programs (Angelsen, 2008). Numerous observations and evaluations highlighted that REDD involves very high transaction costs, that are due to incentives, monitoring and a centralised, multi-level governance mechanism (Angelsen, 2008; Olson and Bishop, 2009).
As described in the previous sections, blockchain technology with a Proof of Stake (POS) design offers a decentralized governance mechanism coupled with new approaches to monitoring and incentivizing both monitoring staff and land managers. Figure 1 depicts our proposed blockchain-based institutional and technological solution, which incorporates: 1) incentives for positive land management behavior—involving pre-minted Global Forest Coins (GFC); 2) a validation mechanism to monitor behavior using remote sensing data and analysis, employing a Proof-of-Stake consensus algorithm; and 3) a decentralized governance system via a GFC blockchain ledger. The initial blockchain technical infrastructure would need to be established with the three components described earlier: 1) two or more network nodes; 2) the GFC ledger with the consensus Proof of Stake algorithm; and 3) the digital GFC currency.
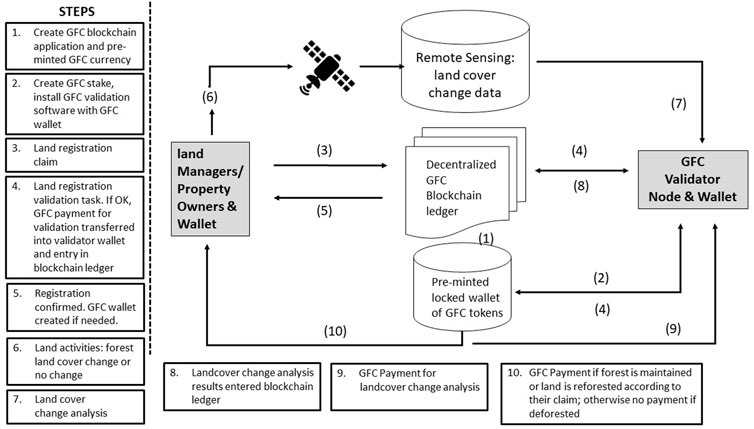
FIGURE 1. A conceptualisation of links in blockchain-based incentive mechanism for reforestation and avoided deforestation following a Proof of Stake approach.
The incentive would be established through payments made to land managers for changing land cover to forest or for maintaining forest. GFC tokens are issued and put into circulation when paid to farmers in response to their forestry initiatives. Automated payments would be made through the blockchain triggered by validating monitoring data. On an annual basis the land manager would receive GFC tokens that he/she can convert into fiat currency. The amount paid depends on the amount of carbon sequestered, which is estimated by the monitoring approach integrated into the blockchain solution. Several existing remotes sensing technologies provide monitoring solutions that robustly convert area of forest into sequestered carbon (Tubiello et al., 2021). The farmer gets paid the annual difference in forest growth as an estimate of sequestered carbon. In a year when the forest of a participating land owner declines, for instance after logging or a forest fire, no payments are made. Annual payments recommence with regrowth. The incentive scheme could require land managers to buy back carbon credit in case forest is lost to manage moral hazard. However, in areas where fire risks prevail land managers would perceive a high risk to participate in a scheme that includes such a buy-back clause. Also, in case of logging, which is typically part of sustainable forest management, land managers might be asked to buy back GFC. However, if timber was converted into building material, furniture and mulch, carbon storage would be at least partly maintained and regrowing forest would involve accelerated carbon sequestration. Consequently, moral hazard issues would need to be managed well without diminishing the incentive to participate in the GFC mechanism. The below approach is not assuming that the GFC is a stable coin but rather that the token/coin price can change depending on market supply and demand, if more forest is legislated for global carbon sequestration.
Governments and international organizations would buy GFC from land managers. First, the price would be based on what the buyer perceives as an effective incentive. But the more funds are made available to buy GFC the higher the demand, which would increase the price per token/coin. The latter would become an important driver if private sector actors participate in this market to achieve a zero-carbon target.
The launch would require some initial investment by an NGO or some government agencies to create the initial GFC blockchain application -- the blockchain ledger system, the GFC pre-minted currency and client software that would include GFC wallet technology (Figure 1, Step 1). This would establish the GFC currency, which will be transferred into an integrated core locked wallet that will be used for payments later for both participating landowners and validator nodes (described below). At this stage organizations interested in becoming validator nodes of the blockchain ledger could invest in the GFC currency to establish their GFC digital wallets and as a result their Proof of Stake in the system (Figure 1, Step 2).
Once the initial blockchain technical infrastructure and pre-minted GFC currency are established (e.g., ledger), land managers or property owners who want to participate and earn GFC through forest conservation would register their land in a specifically developed client software and make a quantifiable forest land cover commitment. This would be submitted through software as a request by the land manager to participate in the GFC blockchain program as well as an initial 1-year proposed commitment (e.g., to not deforest the parcel) (Figure 1, Step 3). This would initiate a proof of ownership check by decentralized GFC network validator nodes in their particular country with access to property records to avoid individuals establishing a claim for somebody else’s land, as well as an initial baseline assessment of the property for forest cover and their proposed commitment by these same validation network nodes. In more developed countries the property ownership records will be relatively easy to connect with and utilize. In other lesser developed countries, this will be a more challenging validation system to implement. But regardless, validation needs to be done by one or more validation nodes in the country where the parcel resides, and once the validation is completed, the initial transaction for the parcel is added to the ledger by the validator, and in return, the validator node is paid GFC for their efforts (Figure 1, Step 4), and the land manager or property owner is sent a registration confirmation (Figure 1, Step 5).
Over a period of time, 1 year perhaps, land managers would undertake their regular land-related activities (Figure 1, Step 6) that would, ideally, be consistent with their commitment made in Step 3, such as not to deforest or to reforest their parcel. After a year, the initial commitment entry in the GFC blockchain ledger would be monitored utilizing remote sensing satellite land cover data (e.g. http://www.openforis.org or https://www.usgs.gov/special-topics/lcmap), again, by a participating monitoring/validator node in the country where the parcel resides (Figure 1, Step 7).
The results of this analysis would be entered by these network nodes into the GFC ledger (Figure 1, Step 8). The software used by the monitoring\validator network nodes would also ensure that the network node is being paid for the transaction validation services, as would the forest land manager or property owner if the analysis provided evidence that they were meeting their original commitment (Figure 1, Steps 9 and 10). The payment would be per ha for the previous period of a year (or some other reasonable time frame), and could consider the previous vegetation state as remote sensing data is continuously improving. Such a mechanism would generate regular GFC income for the land owners and would improve forests’ competitiveness against cash crops. During a reforestation phase, payments per ha might be higher than during a maintenance state when additional carbon sequestration declines. The mechanism could be modified to provide higher rewards for establishing mixed species than monoculture to improve biodiversity values. The land manager would be inclined to compare per ha returns from forests with other crops, which combined with non-pecuniary motivations could make the conservation of forests competitive.
The conceptual blockchain-based approach minimizes the risk for coercion and corruption because of the decentralized design and minimizes transaction costs. However, the utilization of a token as incentive would require a market for land owners to turn their earned GFC token rewards into fiat currency. Computationally, this step could be facilitated through the same software application as the initial step for registration or through one of the many existing cryptocurrencies. However, cryptocurrencies exchanges are subject to high volatility (Tapscott and Tapscott, 2016). It is unlikely that risk-averse land owners will replace existing crops with forests if income prospects are highly uncertain. Equally, extremely high incentives could introduce substantial risks for food security. Land owners that produced rice, vegetables, or other produce relevant for local and regional food security might be incentivized to replace food crops by trees to increase farm revenue. This could trigger a surge in food prices as food crops would start competing with high forest income. This scenario could severely affect food security if effectively causing land use change at a global scale. Third parties—Governments or international organizations—might provide the necessary interventions to stabilize GFC token prices to provide effective incentives for the desired land use change while minimizing food security risks.
The GFC decentralized token-based mechanism would allow Governments and International Organizations, and advocacy groups to increase the incentive by buying GFC to increase the price. This allows government and non-government agencies to establish a minimum GFC value to ensure effective incentives for land owners on the ground. Further demand for GFC might come from donations, citizens, but also from ESG (environmental, social, and governance) requirements or offsets by firms. If companies, individuals, or (local or central) governments want to achieve zero emission targets by offsetting their own carbon emissions they might want to buy GFC as their carbon credit, which would be valid for a year. This establishes robust demand, increasing GCF value and would allow landowners to increase their income from forests and accelerate conservation incentives on the ground.
Deforestation could be targeted by regulatory instruments and require land owners to buy a certain amount of GFC tokens for a multi-year period and deposit them in a locked wallet. From a global perspective, this would increase the price and therefore the incentive to establish forests somewhere else, which would result in a stabilization or even increase of global forest cover.
Extending this blockchain approach to other natural resource management contexts—a groundwater example
We have conceptualized similar PoS blockchain-based incentive models in other important natural resource contexts, such as Groundwater Management and Greenhouse Gas Emissions. Due to space limitations, we will only briefly mention the former here.
In groundwater management, the blockchain mechanism would require the creation and introduction of a payment token—let’s call it the Regional Aquifer Coin or RAC—for a specific aquifer. Farmers adjacent to this aquifer would be offered to participate in this incentive scheme.
After creation of the RAC pre-minted tokens and development of the technical blockchain infrastructure and client software, and the establishment of PoS validation nodes, registration of individual farms is made through a software application and the validation of ownership through the network the farm would be listed—a block created in the RAC ledger—for the relevant monitoring. Farming activities continue and tools such as FAO’s AquaCrop provide essential high-resolution technology to track crop growth. Even easier would be a system that is based on actual well monitoring and rainfall data (e.g. http://www.marvi.org.in/mywell-app). This network of RAC monitoring\validating nodes would execute a water utilization algorithm that compares average crop growth, the actual rainfall and possible surface water subtraction to indicate if the farm used ground water or not. Depending on the crop planted the amount of water used could be estimated. This value would be compared with the level of sustainable groundwater use based on aquifer recharge for the past one or 2 years (Ward and Dillon, 2012; Maheshwari et al., 2014). Network validators would record the results of the analysis in the RAC blockchain ledger—or this could be done automatically by software—and RAC tokens would be generated by the reporting software. Farmers that use less groundwater than calculated for a sustainable amount per ha receive a number of RAC tokens per megalitre, and network validators would receive RAC payment for their analysis efforts. In the case of a drought, the sustainable use level would decline and more RAC tokens would be provided for lower or no use. In time of abundant water fewer RAC tokens would be provided.
Discussion
Many scholars have investigated institutional mechanisms to contribute to the sustainable management of natural resources. Blockchain technology offers opportunities to craft instruments for sustainability-focused institutional arrangements. The combination of decentralised ledgers and state-of-the-art monitoring (e.g., remote sensing) allows circumventing centralised solutions, associated with high transaction costs and often failing to introduce effective incentives for the actual resource user (Schomers and Matzdorf, 2013). This paper conceptualises blockchain solutions that could improve sustainability for forest management and groundwater use. These solutions demonstrate how the new era of token-economics—and the ability to improve incentive structures and monitoring through a decentralized method of implementation—could effectively change the behaviour of resource users and, in some application areas, at a global scale. The creation of ‘complementary’ currencies supporting sustainable development is empirically well tested (Sahakian 2014).
However, these technologies and the institutional arrangements they would be embedded in could have substantial side effects and raise questions needing further analysis. For example, first, as for many other institutional arrangements, there is always a risk for resource users misusing the newly introduced economic incentives and lead to less sustainable outcomes. Therefore, it is pertinent to test the institutional arrangements that would involve blockchain technology—for example through the implementation of social simulation models, which allow for the modelling of individual behaviour (Gilbert, 2008; An, 2012). Furthermore, small-scale pilots and experiments can provide further insights into the practicality of the Global Forest Coin blockchain and how incentives affect behaviour and decision making on the ground. Such small-scale solutions would allow communities to implement their own DAO which can be small and local.
In sum, blockchain technology offers new effective mechanisms for improving institutional arrangements for sustainable natural resource management and justifies increasing attention by the scientific and public policy community. Currently, blockchain developments are largely driven by private sector interests. In this Perspective, we hope we have demonstrated that blockchain has real potential for the public good.
Data availability statement
The original contributions presented in the study are included in the article/supplementary material, further inquiries can be directed to the corresponding author.
Author contributions
AS was the initiator of the ideas here however both authors collaborated on developing the ideas and co-writing the manuscript.
Conflict of interest
The authors declare that the research was conducted in the absence of any commercial or financial relationships that could be construed as a potential conflict of interest.
Publisher’s note
All claims expressed in this article are solely those of the authors and do not necessarily represent those of their affiliated organizations, or those of the publisher, the editors and the reviewers. Any product that may be evaluated in this article, or claim that may be made by its manufacturer, is not guaranteed or endorsed by the publisher.
Footnotes
1We should note that while PoW and PoS are the two main options there are also hybrids and other alternatives emerging (Bach et al., 2018). For instance, PoW principles could be integrated without Bitcoin’s deflationary algorithm, which would allow reducing energy use to the level of PoS concepts.
References
Allen, D. W. E., Berg, C., Lane, A. M., and Potts, J. (2017). The economics of crypto-democracy. SSRN J., 2973050. doi:10.2139/ssrn.2973050
An, L. (2012). Modeling human decisions in coupled human and natural systems: Review of agent-based models. Ecol. Model. 229, 25–36. doi:10.1016/j.ecolmodel.2011.07.010
Angelsen, A. (2008). Moving ahead with REDD: Issues, options and implications. Bogor Regency, Indonesia: CIFOR.
Atzori, M. (2015). Blockchain technology and decentralized governance: Is the state still necessary? Rochester, NY: SSRN.
Bach, L. M., Mihaljevic, B., and Zagar, M. (2018). “Comparative analysis of blockchain consensus algorithms,” in Proceedings of the 2018 41st International Convention on Information and Communication Technology, Electronics and Microelectronics (MIPRO), Opatija, Croatia, 21-25 May 2018, 1545–1550. doi:10.23919/MIPRO.2018.8400278
Bjornlund, H. (2003). Efficient water market mechanisms to cope with water scarcity. Int. J. Water Resour. Dev. 19, 553–567. doi:10.1080/0790062032000161364
Bromley, D. (2006). Sufficient reason: Volitional pragmatism and the meaning of economic institutions. Princeton, New Jersey, United States: Princeton University Press.
Campos, I., Gonzalez-Gomez, L., Villodre, J., Gonzalez-Piqueras, J., Suyker, A. E., and Calera, A. (2018). Remote sensing-based crop biomass with water or light-driven crop growth models in wheat commercial fields. Field Crops Res. 216, 175–188. doi:10.1016/j.fcr.2017.11.025
CBECI (2022). Cambring Bitcoin energy consumption index. Available at: https://ccaf.io/cbeci/index/comparisons (Accessed June 4, 2022).
Cord, A. F., Brauman, K. A., Chaplin-Kramer, R., Huth, A., Ziv, G., and Seppelt, R. (2017). Priorities to advance monitoring of ecosystem services using earth observation. Trends Ecol. Evol. 32, 416–428. doi:10.1016/j.tree.2017.03.003
Costanza, R., d'Arge, R., de Groot, R., Farber, S., Grasso, M., Hannon, B., et al. (1997). The value of the world’s ecosystem services and natural capital. Nature 387, 253–260. doi:10.1038/387253a0
Ewers, R. M., and Smith, R. J. (2007). Choice of index determines the relationship between corruption and environmental sustainability. Ecol. Soc. 12, resp2. doi:10.5751/es-01950-1201r02
Gilbert, N. (2008). Agent-based models. Thousand Oaks, California, United States: SAGE Publications.
Howson, P. (2019). Tackling climate change with blockchain. Nat. Clim. Chang. 9, 644–645. doi:10.1038/s41558-019-0567-9
Hughes, K. (2017). Blockchain, the greater good, and human and civil rights. Metaphilosophy 48, 654–665. doi:10.1111/meta.12271
Kandulu, J. M., Connor, J. D., and MacDonald, D. H. (2014). Ecosystem services in urban water investment. J. Environ. Manag. 145, 43–53. doi:10.1016/j.jenvman.2014.05.024
Karaindrou, E. (2017). Distributed ledger technology and the future of payment services. doi:10.2139/ssrn.3078523
Kshetri, N. (2017). Will blockchain emerge as a tool to break the poverty chain in the Global South? Third World Q. 38, 1710–1732. doi:10.1080/01436597.2017.1298438
Maheshwari, B., Varua, M., Ward, J., Packham, R., Chinnasamy, P., Dashora, Y., et al. (2014). The role of transdisciplinary approach and community participation in village scale groundwater management: Insights from Gujarat and Rajasthan, India. Water 6, 3386–3408. doi:10.3390/w6113386
Meppem, T. (2000). The discursive community: Evolving institutional structures for planning sustainability. Ecol. Econ. 34, 47–61. doi:10.1016/s0921-8009(00)00151-8
North, D. C. (1990). Institutions, institutional Change and economic performance. Cambridge, United Kingdom: Cambridge University Press.
Olsen, N., and Bishop, J. (2009). The financial costs of REDD: Evidence from Brazil and Indonesia. Gland Switzerland: IUCN.
Ostrom, E. (2009). A general framework for analyzing sustainability of social-ecological systems. Science 325, 419–422. doi:10.1126/science.1172133
Ostrom, E., Gardner, R. H., and Walker, J. (1993). Rules, games, and common-pool resources. Ann Arbor, Michigan, United States: The University of Michigan Press.
Ostrom, E. (2005). Understanding institutional diversity. Princeton, New Jersey, United States: Princeton University Press.
Pazaitisa, P., De Filippib, P., and Kostakisa, V. (2017). Blockchain and value systems in the sharing economy: The illustrative case of Backfeed. Technol. Forecast. Soc. Change 125, 105–115. doi:10.1016/j.techfore.2017.05.025
Sahakian, M. (2014). Complementary currencies: What opportunities for sustainable consumption in times of crisis and beyond? Sustain. Sci. Pract. Policy 10 (1), 4–13. doi:10.1080/15487733.2014.11908121
Saleh, F. (2021). Blockchain without waste: Proof-of-Stake. Rev. Financial Stud. 34, 1156–1190. doi:10.1093/rfs/hhaa075
Schomers, S., and Matzdorf, B. (2013). Payments for ecosystem services: A review and comparison of developing and industrialized countries. Ecosyst. Serv. 6, 16–30. doi:10.1016/j.ecoser.2013.01.002
Smajgl, A., and Bohensky, E. (2012). When households stop logging ‐ evidence for household adaptation from East Kalimantan. Forest Policy Econ. 20, 58–65. doi:10.1016/j.forpol.2012.01.013
Smajgl, A., and Damen, B. (2020). Blockchain solutions to unlock climate finance for increased ambition in the agriculture and landuse sectors under the Paris Agreement. Bangkok: FAO & MERFI. Avaiable at: https://www.merfi.org/_files/ugd/8dc2cd_df917e49c8db421b97921bbdd958ad97.pdf.
Smajgl, A. (2017). The Global Forest Coin: Institutional arrangements for sustainability and the transformative potential of blockchain technology. Bangkok: MERFI. Revised working paper November 2017. Available at: https://www.merfi.org/_files/ugd/8dc2cd_4bf8f065aa1249f5bd7ffd2800674891.pdf.
Smajgl, A., Toan, T. Q., Nhan, D. K., Ward, J., Trung, N. H., Tri, L. Q., et al. (2015b). Responding to rising sea levels in the Mekong Delta. Nat. Clim. Chang. 5, 167–174. doi:10.1038/nclimate2469
Smajgl, A., Xu, J., Egan, S., Yi, Z. F., Ward, J., and Su, Y. (2015a). Assessing the effectiveness of payments for ecosystem services for diversifying rubber in Yunnan, China. Environ. Model. Softw. 69, 187–195. doi:10.1016/j.envsoft.2015.03.014
Swan, M. (2015). Blockchain: Blueprint for a new economy. Sebastopol, California, United States: O’Reilly.
Tapscott, D., and Tapscott, A. (2016). Blockchain revolution: How the technology behind Bitcoin is changing money, business, and the world. New York, United States: Penguin Publishing Group.
Tubiello, F. N., Conchedda, G., Wanner, N., Federici, S., Rossi, S., and Grassi, G. (2021). Carbon emissions and removals from forests: New estimates, 1990–2020. Earth Syst. Sci. Data 13, 1681–1691. doi:10.5194/essd-13-1681-2021
Ward, J., and Dillon, P. (2012). Principles to coordinate managed aquifer recharge with natural resource management policies in Australia. Hydrogeol. J. 20, 943–956. doi:10.1007/s10040-012-0865-z
Williamson, O. (2000). The new institutional economics: Taking stock, looking ahead. J. Econ. Literature 38, 595–613. doi:10.1257/jel.38.3.595
Keywords: blockchain, sustainability, institutions, incentives, deforestation
Citation: Smajgl A and Schweik CM (2022) Advancing sustainability with blockchain-based incentives and institutions. Front. Blockchain 5:963766. doi: 10.3389/fbloc.2022.963766
Received: 07 June 2022; Accepted: 07 October 2022;
Published: 21 October 2022.
Edited by:
Leanne Ussher, Wolfram Blockchain Labs, United StatesReviewed by:
Will Masters, Ogallala Life, United StatesCopyright © 2022 Smajgl and Schweik. This is an open-access article distributed under the terms of the Creative Commons Attribution License (CC BY). The use, distribution or reproduction in other forums is permitted, provided the original author(s) and the copyright owner(s) are credited and that the original publication in this journal is cited, in accordance with accepted academic practice. No use, distribution or reproduction is permitted which does not comply with these terms.
*Correspondence: Alex Smajgl, YWxleC5zbWFqZ2xAbWVyZmkub3Jn