- Department of Engineering and Geology (INGEO), University “G. D'Annunzio” of Chieti-Pescara, Pescara, Italy
Seismic vulnerability assessment of urban centers is a challenging issue that needs to be faced accurately for the earthquake risk of large territorial areas. The selection of suitable methods is a crucial aspect that must be treated according to different evaluation processes, depending on the size of the problem and on the available calculation capacities. A possible strategy consists in analyzing large stocks of buildings, so to include in the analyses all those structural parameters that characterize their response and to involve the variability of the considered features. This would require a high computational effort that should be addressed to the investigation of the response of a large number of models. For this reason, simplified procedures based on engineeristic judgements, are commonly considered a viable way to be undertaken in order to predict damage scenarios. Alternatively, the attention could be focused on a limited number of buildings that are judged to be representative of the whole stock. In this case, more sophisticated analyses could be carried out and the obtained results could be extended to the whole urban center. Based on this premise, this paper presents the results obtained through the application of two different seismic vulnerability methodologies on the historic center of Campotosto, in Italy, which was hit by the last 2016 Central Italy earthquake. The first is an empirical method, applied considering a large stock of 130 buildings, which was calibrated by the authors after the 2009 L'Aquila earthquake for historical centers that are similar to the one studied in this paper. The latter, is a method based on analytical formulations dealt with by the Vulnus software, developed at the University of Padua in Italy, which was used for evaluating the seismic vulnerability of an aggregate building, which has been considered representative of the historic center. The final aim is to compare, also in the light of the damage provoked by the 2016 earthquake, the observed post-seismic scenarios, expressed in terms of fragility curves, derived from the two applied methodologies, in order to prove their reliability and to stress the possible issues related to their implementation at different scales.
Introduction
The seismic activity that hit the central area of Italy in 2016 stresses, once again, the fragility of those territories characterized by the presence of small medieval historic centers made of poor masonry structures (Fiorentino et al., 2018; Sorrentino et al., 2018). For this reason, their seismic vulnerability assessment is a very timely topic that needs to be faced urgently.
Seismic vulnerability evaluation is a process that can be implemented at different levels. The selection of a proper method is a crucial issue that depends on the accuracy of the targeted results, as well as on the sustainability of the analyses that have to be carried out owing to the scale to which the evaluation itself is addressed (Formisano and Marzo, 2017).
When the goal is to quantitatively detect the vulnerability at the building level, traditional methods of structural analysis, based on the use of sophisticate Finite Element (Brando et al., 2015) or numerical (Clementi et al., 2017; Portioli and Cascini, 2017; Cascini et al., 2018) models, properly calibrated on the basis of tests (Krstevska et al., 2010; Tashkov et al., 2010; Anastasopoulos et al., 2018), can be used. This often entails a careful knowledge of the construction details and a significant effort devoted to capture the most relevant behaviors of the structures during an earthquake.
Conversely, when the objective is to examine a population of buildings and to establish priorities of interventions for mitigating the seismic risk of the whole urban center they form, rapid methods based on engineering judgements are often the most viable way (Vicente et al., 2011; Ferreira et al., 2013). In this case, empirical methods can be applied for assessing the potential damage (expressed in terms of percentages of buildings that would experience certain limit states) under different earthquake intensities. The engineering judgements should be given for those structural characteristics that contribute positively or negatively to the buildings response, also in view of the observations carried out after earthquakes of the past on buildings that are similar to the ones of the studied stock (Brando et al., 2017b;Sorrentino et al., 2019).
A compromise strategy is to select, among the buildings of the urban centers, few typologies that are representative of the whole population under examination and to carry out analyses of intermediate complexity that are more manageable. For example, for masonry buildings, kinematic analyses (Criber et al., 2015) are often implemented, looking at those out-of-plane mechanisms that are more dangerous for the building stability. These types of analyses are particularly indicated when aggregates buildings are analyzed. In fact, for these types of constructions (Formisano, 2017a,b), numerical FEM model would result too much heavy and would need a large number of information on the structural details. Moreover, within the kinematic analyses, the interaction forces between the buildings in the same aggregate can be taken into account too.
On the basis of this premises, this paper deals with the seismic vulnerability assessment of the historic center of Campotosto, in Abruzzi region. To this purpose, two methodologies are applied at different scales. The first is an empirical method developed by the Authors after the 2009 L'Aquila earthquake and validated on historic centers that are similar to the one dealt with in this paper. The latter consists in the application of kinematic analyses on an aggregate building that has been judged to be representative of the whole historic center. To this aim the Vulnus software, developed at the University of Padua in Italy, has been used.
The final aim is to compare the fragility curves derived from the two applied methodologies, in order to prove their reliability and to stress the possible issues related to their implementation at different scales.
The paper is organized as follows. In section The Historic Center of Campotosto, a brief description of the historic center of Campotosto is given. In section The methodologies Applied for the Seismic Vulnerability Assessment, the considered procedures that have been implemented at the “urban” and at the “aggregate” scales are presented. Finally, in section The Seismic Vulnerability of the Historic Center of Campotosto, the results obtained by applying the two methodologies are presented and the corresponding fragility curves are compared.
It must be pointed out that the results reported in the paper are the base for a more comprehensive study that has to be developed in the future accounting for larger stocks of buildings that have to be analyzed through the here presented procedures. Moreover, some issues that are only briefly mentioned in this paper, such as the one related to the site effects due to the soil typology, are worthy of being deepening, as they could influence significantly the proposed outcomes.
The Historic Center of Campotosto
General
Campotosto is a town of about 730 inhabitants in the province of L'Aquila, located at 1,420 m on the sea level. It is part of the so-called “Amiternina” mountain community and gives its name to a lake, in the hearth of the homonymous natural park.
The urban articulation of Campotosto is characterized by a main core in the top and most ancient part of the town, which had its spatial development, during the centuries that followed its foundation (XIII century), until the lake. The urban organization is articulated around a series of minor roads arranged like a comb, which has in the main street, Via Castello, its rib (Figure 1A).
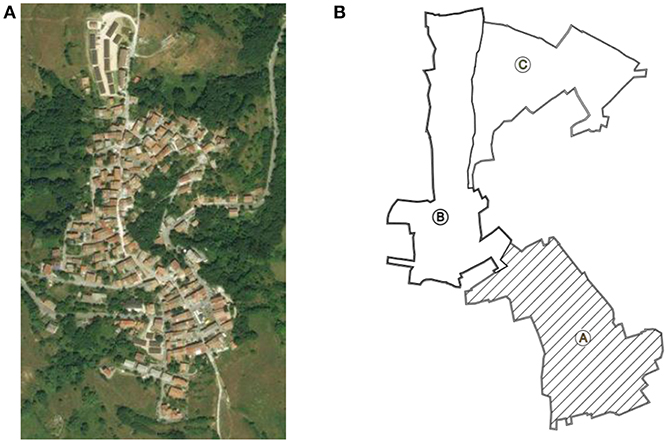
Figure 1. Historic center of Campotosto: (A) Aerial photo (source: Google Earth) and (B) Territorial subdivision in areas according to the reconstruction plan of Campotosto drafted after 2009 L'Aquila earthquake (Ponzi et al., 2013).
The historical center was divided in three areas (Figure 1B). The first is located on the top of the hill and is labeled, in Figure 1B, with the letter C. The second mainly extends in the central area of Campotosto, along the western side of an embankment (Area B). The third (Area A) is situated closed to the lake.
This formal division was used to optimize the survey actions and the subsequent data elaboration. It was proposed in the reconstruction plan according to an historical analysis (Ponzi et al., 2013), and was accepted for carrying out the analyses presented in this paper, because the areas present different lithological features that will not dealt here, but that the authors are deepening for future more comprehensive analyses. However, the buildings of the three area present similar features and can be seen as a unique vulnerability class.
Historical and Recent Seismicity
The territory of Campotosto was characterized in the past by a relevant earthquake activity, which found in the last 2016 seismic sequence, culminated in the most destructive shaking for Campotosto of January 2017, one of the most tragic episodes.
The main historical earthquakes occurred in 1639 (Mw 6.2), in 1646 (Mw 5.9) and in 1703 (Mw 6.9). They caused severe damage and implied several reconstruction processes that shaped the historic center up to the current aspect. Indeed, the original medieval plants of several buildings were completely demolished and replaced by more modern masonry buildings.
This historic center was also affected by the 2009 L'Aquila earthquake. The main shock of this earthquake was followed by two significant aftershocks of magnitudes 5.3 Mw (7 April) and 5.1 Mw (9 April), that provoked a severe state of damage, in particular the latter.
Finally, the last 2016 Central Italy earthquake affected almost the 70% of the buildings, provoking several collapses, in particular in the zone A of Figure 1B, near the lake.
The damage survey presented in this paper was carried out after the seismic event occurred on the 18th of January 2017, with epicenter in Montereale (L'Aquila). The accelerogram of this earthquake, recorded by the station of Mascioni, 19 km far from the epicenter and 14 km from Campotosto, is shown in Figure 2A. The 5% damped elastic spectrum shown in Figure 2B shows a maximum spectral acceleration of 0.72 g for short period buildings such as the ones studied in this paper.
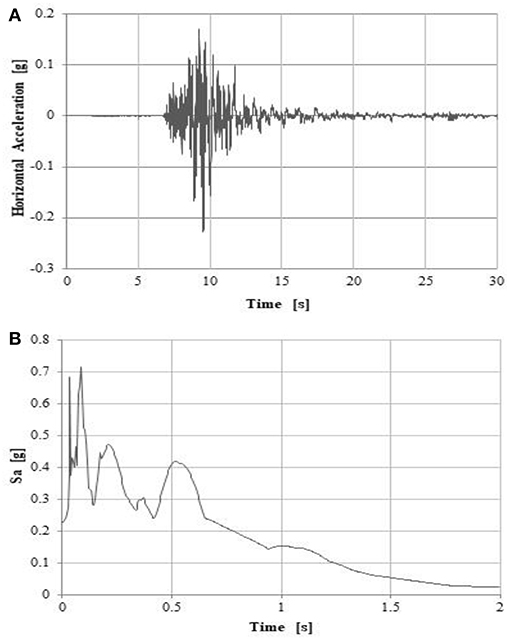
Figure 2. (A) The accelerogram recorded by the station of Mascioni (18th of January 2017). (B) The corresponding 5% damped elastic spectrum.
Recent geological studies carried out in Campotosto confirm that the medium-high seismic hazard of the Campotosto basin is due to the presence of an active fault, the Monte Gorzano–Campotosto fault, also known as Monti della Laga fault, whit an extent of almost 30 km.
As mentioned before, this hazard can be exacerbated by the presence of side effects. In fact, as it is shown in Figure 3, extracted from a recent investigation carried out for achieving a third-level microzonation of the area (D'Onofrio and Tatoni, 2017), Campotosto is located at the top of a small ridge consisting of a flyschoid or turbiditic rock.
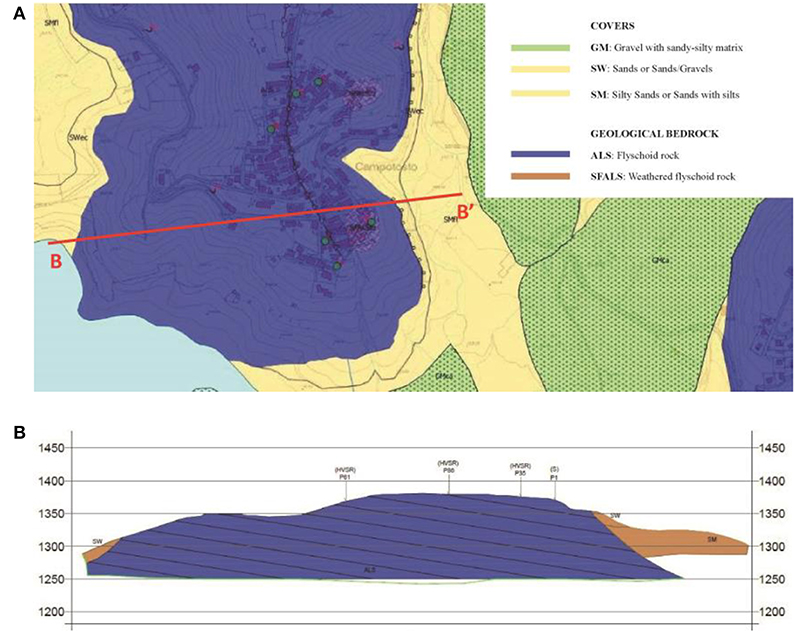
Figure 3. Morphological and geological outlines: (A) Geological map of the historic center of Campotosto and (B) Geological section of the most critic area of the historic center (D'Onofrio and Tatoni, 2017).
This rock is highly weathered in the upper portion leading to possible stratigraphic amplification phenomena, in addition to the site effects related to the topographical configuration described in section General. Gravelly and sandy covers can be found on the flanks of the ridge. Thus, amplification factors of 1.9, 2.0 are possible, as shown in the microzonation map in Figure 4.
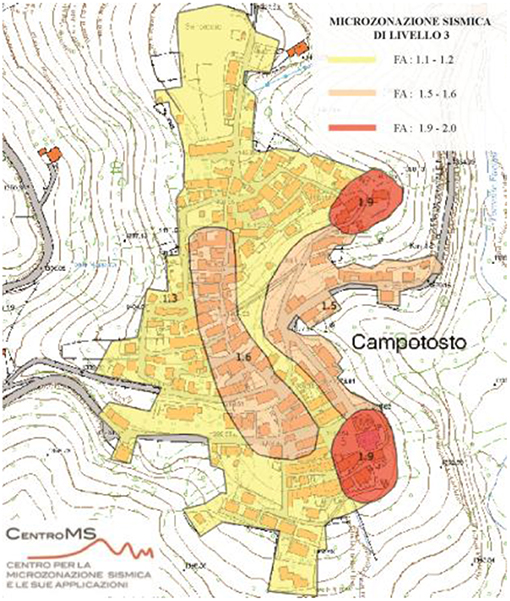
Figure 4. Soil amplification factors detected by third level microzonation studies (D'Onofrio and Tatoni, 2017).
Main Features and Fragilities of Buildings
As mentioned before, the reconstructions that were carried out on the historic center of Campotosto, after the several earthquakes of the past, have led to the loss of the original urban configuration, as well as of the original building plant. However, the current layout of the built environment appears to be very uniform, except for few cases of reinforced concrete buildings, with recurrent typologies that can be seen as a unique vulnerability class.
The settlement consists of stone masonry buildings that mostly are undergrounded for one or more stories, at least on one side, where, at the top of the underground floors, on one of the perimeter edges, the main entrances overlook on minor roads. In this way, buildings form a series of “terraces” laid on the sloped terrain, so to accompany the orographic configuration of the site.
Focusing the attention on the masonry buildings, mainly organized on 2 or 3 stories above the ground, they are made of sandstone—the typical stone of L'Aquila province, where several mines are still present- with extremely varied size and assembled with weak and thin layers of lime mortars, as shown in Figure 5.
Observing the damaged buildings it has been possible, after the earthquake, to identify the characteristics of the three-leaves masonry wall sections, which have a thickness of about 80 cm and have inner core composed of poor filling material (Figure 6A). This kind of masonry is widely used in Abruzzi region and it results extremely dangerous for the stability of the wall when the internal core is degraded and there are no transversal connections between the two outer leaves.
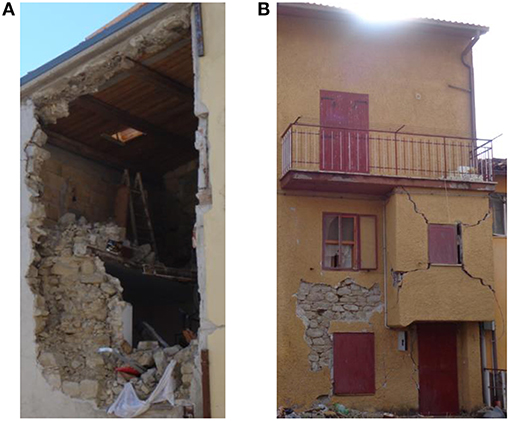
Figure 6. Structural features: (A) Three-leaf masonry walls. (B) Example of a building negatively affected by improper additions.
Also, it has been observed that the walls are scarcely connected each other, as well as that the connection between the orthogonal walls is basically absent or not effective. These lacks were responsible of several out-of-plane mechanisms that were observed after both the 2009 and the 2016 seismic events. Roofing system are mainly made of timber elements.
Moreover, several buildings and aggregates show signs of transformation over time that worsened their original structural behavior, such as additions in plan (Figure 6B) and elevation, new or enlarged openings and use of materials that are not homogeneous with respect to the original ones, such as reinforced concrete used for horizontal slabs, ring beam, lintels and roofs.
Only the buildings renovated in more recent times are characterized by walls of good quality and are equipped with anti-seismic provisions as tie rods.
The Methodologies Applied for the Seismic Vulnerability Assessment
The Method Used at the Urban Scale
The seismic vulnerability at large scale of Campotosto was evaluated through the application of an empirical method that was calibrated in order to reproduce the damage observed on the masonry structures of the minor historic centers of Abruzzi after the 2009 L'Aquila earthquake, as it is fully explained in Brando et al. (2017a).
It is a predictive method based on the fundamental hypothesis that, for each seismic intensity I, the probability p[k|I] of attaining a certain limit state, evaluated according to the five damage levels of the EMS-98 macroseismic scale (Grünthal, 1998), can be interpreted by the binomial probability distribution given in Equation (1).
where k is an integer score, ranging between 0 and 5, corresponding to the damage grade Dk that the earthquake may potentially provoke:
• D0: no structural damage (k = 0);
• D1: negligible damage. Slight cracks on the walls, fall of small pieces of plaster, fall of tiles k = 1);
• D2: moderate damage. Cracks in many walls, fall of large pieces of plaster, partial collapse of the chimney (k = 2);
• D3: substantial damage. Large cracks in the walls, failure of non-structural elements, activation of out-of-plane mechanisms (k = 3);
• D4: serious damage. Serious cracks, development of out-of-plane mechanisms with partial collapses that interest the horizontal structures and the walls (k = 4);
• D5: collapse. Notable collapses affecting more than 50% of the structure (k = 5).
μD is the mean of the expected damage grades, according to Equation (2).
where n is the number of buildings of the analyzed stock.
In Figure 7, some example of damage grades observed after the January 2017 earthquake are shown. As it is possible to observe, buildings having more or less the same types of plants presented different types of damage because of the different number and position of opening (see for example the different behavior of the buildings that experienced damage grades D1, D2, and D3), the different position in the aggregate (see the building that experienced a damage grade D5) and, also, because of other factors discussed previously, such as site effects.
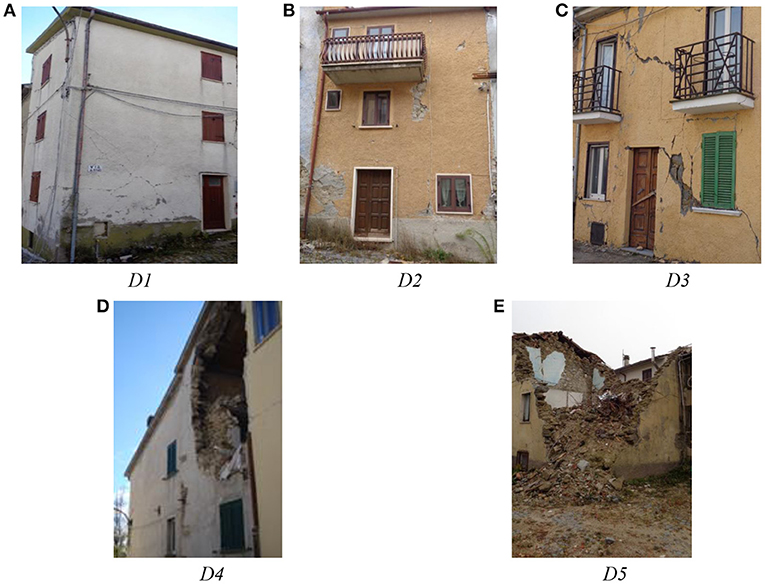
Figure 7. Examples of the observed damage grades in Campotosto after the 2016 seismic event. (A) Negligible damage; (B) Moderate damage; (C) Substantial damage; (D) Serious damage; (E) Collapse.
On the other hand, the hypothesis related to the reliability of the binomial probability function was proved to be well-founded also in the light of the damage observed in the studied historic center after the 2016 Cental Italy Earthquake. As it is shown in Figure 8A, where the observed frequencies of the several damage grades are represented in terms of Damage Probability Matrix (DPM), the binomial probability function well approximate the damage grade D1, D3, and D4, even if the damage grade D2 is overestimated and D5 is underestimated. As for these discrepancies, some ongoing studies, that the authors are carrying out by relating the spatial damage distribution (Figure 8B) to the soil conditions, are proving that the excessive number of buildings experiencing a damage level D5, which lead to an overestimation of buildings that, instead, would be characterized by a damage level D2, collapsed because of some site effects, concentrated in the area A of Figure 1A, closed to the lake. This aspect is surely to be deepened in the future.
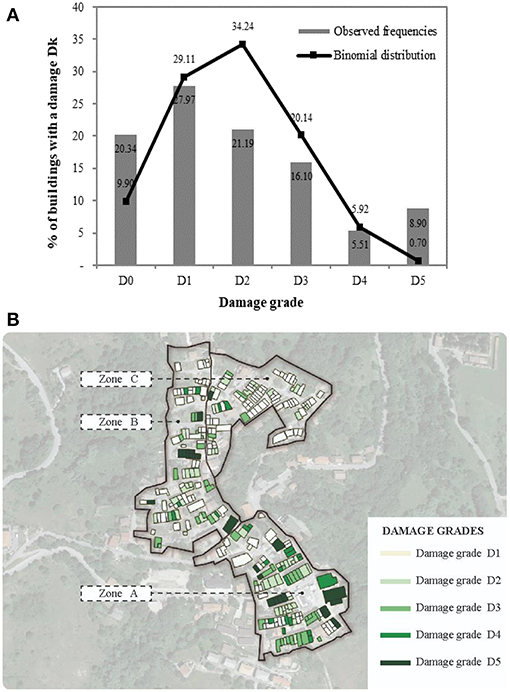
Figure 8. Observed damage scenario. (A) Damage Probability Matrix and Binomial Distribution for the historic center of Campotosto. (B) GIS representation of the Damage spatial distribution (on a Google Earth Map).
It must be finally pinpoint that the discrepancies corresponding to the damage level D0 are quite expectable, as they are likely due to the ambiguities that usually characterize the pre-existing and the seismic induced damage.
The reliability of the binomial distribution for representing damage scenarios means that any type of predictive model has to target to provide, for each earthquake intensity, an estimation of the mean damage to be considered in Equation (1).
The method that has been considered in the present study, which was calibrated in the light of the damage scenarios observed on similar historic centers after the 2009 L'Aquila earthquake, is based on the evaluation, for each building of the historic center, of the structural features that characterize the 14 potential vulnerability sources Pm (with m = 1:14) listed in Table 1.
Each of these features are then judged through the application of two scores vmf and vmp. The first, named “fragility score,” is higher as the structural features makes the vulnerability sources Pm more severe. On the contrary, vmp, named “protection score,” represents a judgment on the type and the effectiveness of the possible anti-seismic devices applied in order to contrast the development of the vulnerability source “Pm.”
Details on how to assign the scores to each vulnerability sources, are given in Rapone et al. (2018). According to the methodology, the two scores have been used, for each building of the stock, to find a vulnerability index iv, given in Equation (3):
where ρm factor, also reported in Table 1, accounts for the influence that the source of vulnerability Pm has on the overall stability of the structure.
All the vulnerability indices calculated according to Equation (3) are then used in order to find the mean index i, according to Equation (4).
is therefore used to calculate the vulnerability factor V of Equation (5):
In this equation, the factors a, b, c, d have been calibrated on the basis of the observations carried out after the 2009 L'Aquila earthquake, as reported in Brando et al. (2017a).
The vulnerability factor V is then used in order to assess the mean damage by applying Equation (6):
where I is the expected macro seismic intensity, expressed in the Mercalli-Cancani-Sieberg scale (MCS), while Q is a coefficient that takes into account the ductility that characterizes the type of analyzed buildings, which, conventionally, also considering studies of the past [see for example (Lagomarsino and Giovinazzi, 2006)], has been imposed to be equal to 2.3.
The Method Used at the Aggregate Scale
Alternatively to the empirical method dealt with in Section The Method Used at the Urban Scale, the vulnerability assessment of the urban center has been analyzed focusing the attention on one aggregate of Campotosto. It was selected in order to be representative of the great part of the buildings forming the stock analyzed with the empirical method dealt with in the previous Section, according to the masonry texture, the number of stories, the number of buildings forming the aggregate itself.
The Vulnus software (Bernardini et al., 2009), developed at the University of Padua in Italy, has been used in order to draw the fragility curve related to the attainment of a condition of severe damage/collapse, namely that fragility curve giving back, consistently with the damage grades provided by the EMS98 macroseismic scale, the probability of attaining a damage grade higher than D3.
In its latest version, the software allows to give an evaluation of the vulnerability of the aggregate by properly combining three indices, named I1, I2, and I3, which can be computed for the single structural units.
I1 is the ratio between the sum of the walls in-plane shear strengths, computed along the weakest direction of the building, and the weight of the building itself. In other words, I1 accounts for the in-plane shear strength of the walls, which are expressed according the well-known Turnsek-Cacovic formulation (Turnsek and Cacovic, 1971), properly modified in order to account for potential sources of non-regularity in plan and/or in elevation.
I2 is the ratio between the acceleration able to provoke the most critical out-of-plane mechanism and the acceleration of gravity. In order to compute this index, the software considers several mechanisms that can be triggered on the perimeter walls
I3 is the weighted sum of the scores of the partial vulnerability factors applied in the second level GNDT form (GNDT, 1994) and not involved in the evaluation of I1 and I2 (only seven of the 11 factors of the GNDT form are taken into account). It adjusts the evaluation based on the I1 and I2 indices and accounts for those sources of vulnerability or of mitigation which are not directly included in the calculations described previously.
The Seismic Vulnerability of the Historic Center of Campotosto
Results Obtained by Applying the Empirical Method
Following an inspection of the typological and structural features of about 130 buildings belonging to the historical center of Campotosto (equally distributed in the three areas shown in Figure 1B), the related fragility (νmf) and protection (νmp) scores have been assigned.
In this way, it has been possible to identify and to classify the most influential fragilities (Figure 9A) and mitigation measures (Figure 9B) that characterize the analyzed buildings.
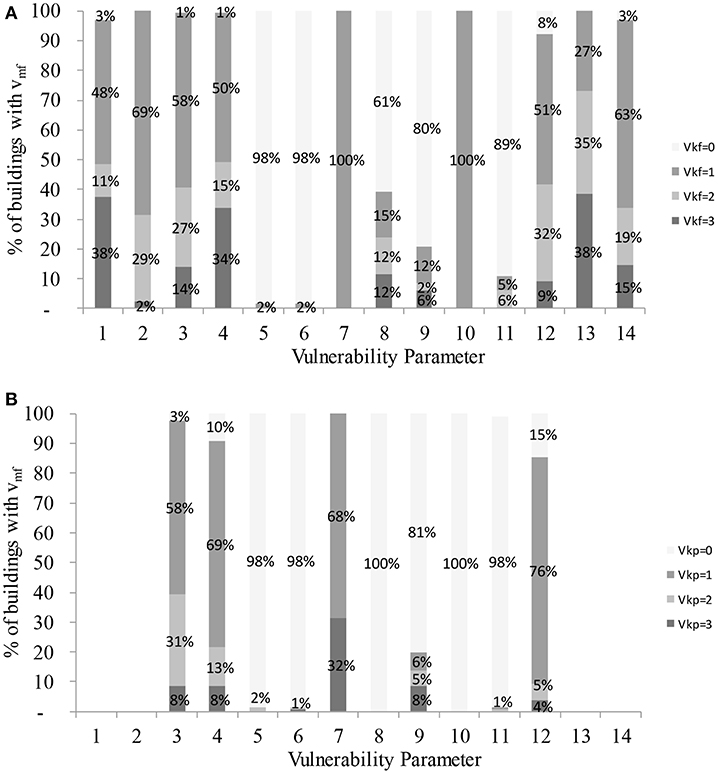
Figure 9. Distribution of the (A) fragility and (B) protection scores for the buildings of Campotosto.
Also, possible interventions to be implemented for pursuing a risk mitigation have been preliminarily determined. As it can be observed in Figure 9A, the fragilities related to the potential trigger of the out-of-plane (Vulnerability source n° 3) and in-plane mechanisms (Vulnerability source n° 4) are particularly relevant. Moreover, a low percentage of buildings with effective anti-seismic preventive measures have been observed, as it is shown in Figure 9B.
Then, the scores have been used in order to apply the methodology presented in section 3.1, for obtaining an evaluation of the mean damage μD values that have to be expected for each earthquake intensity (Figure 10A). These mean values have been therefore used in order to forecast the damage probability matrices, shown in Figure 10B, corresponding to the binomial distribution expressed in Equation (1).
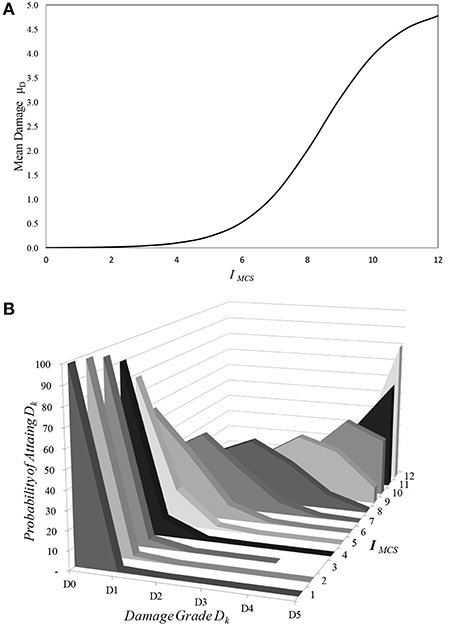
Figure 10. (A) Expected mean Damage (μD) vs. Earthquake Intensity (IMCS) and (B) the related Damage Probability Matrices.
To demonstrate the accuracy of the vulnerability index methodology and its validity in predicting the vulnerability of the considered stock of buildings, the binomial function obtained from the expected mean damage μD for an earthquake intensity IMCS = VIII, that one recorded after the seismic event of 2017, has been superimposed to the binomial distribution obtained through the observed mean μD.
As it is possible to observe in Figure 11, the two distribution perfectly match each other, this proving the reliability of the proposed empirical method.
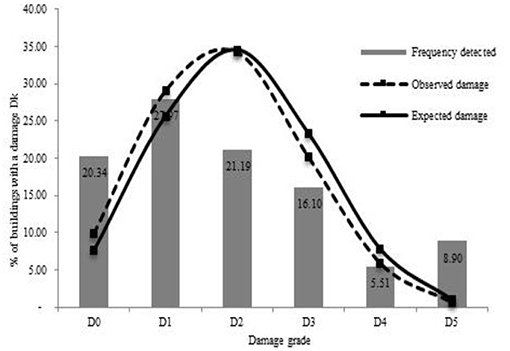
Figure 11. Comparison between the observed frequencies and the binomial distributions evaluated according to the observed and the predicted mean damage.
By cumulating the probabilities shown in Figure 10B, the fragility curves for representing the expected damage scenarios for several earthquake intensities, which give the probability of exceeding the several damage grades, have been obtained. These are shown in Figure 12.
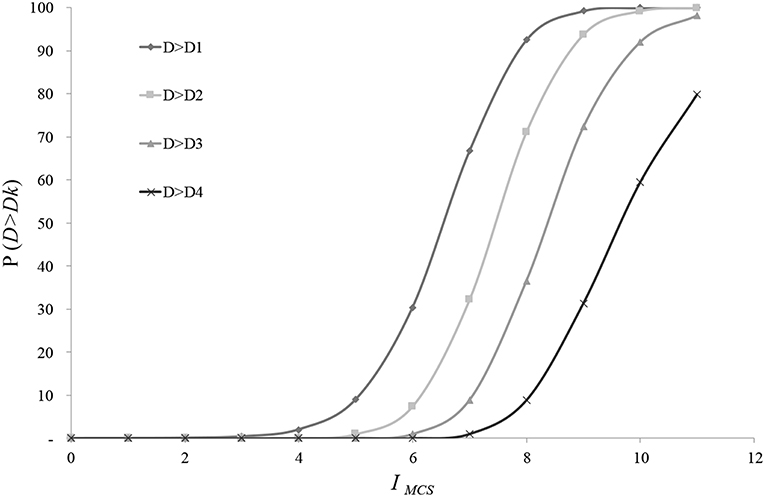
Figure 12. Comparison between the observed frequencies and the binomial distributions evaluated according to the observed and the predicted mean damage.
Results by Applying the VULNUS Methodology
The aggregate considered as representative of the built environment of Campotosto is located in the area A shown in Figure 1B. The plan of the building and its cadastral identification are depicted in Figure 13, whereas in Figure 14 the front and the back are shown. The aggregate develops mainly along one longitudinal axis with a length of about 24 meters and a width of about 6 meters. It consists of 3-storeis buildings with an average height of 7.4 meters.
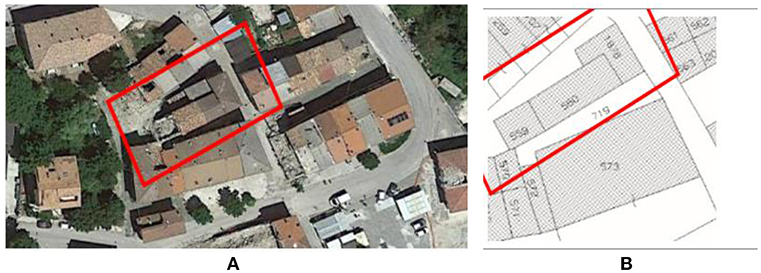
Figure 13. The considered aggregate. (A) Satellite view (Source: Google Earth) and (B) Cadastral map.
Given the impossibility of obtaining detailed historical documents, hypotheses concerning the development of the aggregate during the centuries have been done, mainly based on the analysis of the opening misalignments in the façade. Four structural units have been therefore detected (Figure 15).
Vertical walls are made of rubble stones kept together by thin layer of air lime mortar and are organized according to a three leaf layout, with a total thickness of about 60 cm. According to the Italian Provisions “Circolare”1, this type o masonry is characterized (for a knowledge level LC1) by a compressive strength of fm=2,0 MPa, a shear stress, in absence of axial stress, of 0,035 MPa, elastic normal and tangential moduli of 1,020 MPa and 340 MPa, respectively. The weight per unit of volume is 20 kN/m3.
Floors and roofs are made of timber elements.
A first evaluation of the I1 and I2 indices has been carried out for the maximum ground acceleration recorded at the Mascioni acceleration station, the closest among the ones belonging to the Italian Accelerometric network [RAN (Paolucci et al., 2011)], of 0.279g. This acceleration is very close to the ground acceleration given by the Italian Technical Standards for Construction of 2018 (NTC 2018)2, for a soil type A and a probability of exceedance of 10% in 50 years (0.258g).
The obtained results are given in Figure 16 for the four structural units. Broadly speaking, all the structural units present in both the in-plane and the out-of-plane directions safety factors lower than 1, with a minimum of 0.243 for the out of plane mechanism of the Building 2. This result was actually confirmed by analyzing the damage provoked by the earthquake occurred in January 2017. In fact, several cracks and some triggered out-of-plane mechanisms were observed, with a general state of damage that in the EMS-98 scale can be classified with a grade D3.
It is interesting to note that the I2 indices are lower than I1, this stressing the higher sensitivity of the aggregate to experience out-of-plane mechanisms.
Once that all the information required for the definition of the I3 index have been imputed in the software, fragility curves have been generated. In particular, the software combines the three indices I1, I2, and I3 for different ground accelerations, and gives the probability of attaining a state of severe damage/collapse, or rather, consistently with the EMS-98 macro seismic scale, the probability of attaining a damage level D4 or D5 (namely the probability of exceeding a damage level D3).
In Figure 17, the obtained fragility curves are shown. In the same figure, two other fragility curves, named “Lower Bound” and “Upper Bound,” are represented. They can be considered as a lower and an upper bound of the fragility and are obtained by Vulnus through a proper modification of the curve “Mean,” once that uncertainties, related to those parameters that cannot determined accurately, on the indices I1, I2, and I3 are properly accounted for. Also, the curves corresponding to the vulnerability classes “A” (curve “EMS98 LOW”) and “B” (curve “EMS98 UP”), according to the macroseismic scale EMS-98 are reported. It is possible to observe that, considering the mean curve, the buildings of the aggregate have to be assimilated to a vulnerability class “A.”
Conclusion
In this paper, the vulnerability assessment of the historic center of Campotosto, in the district of L'Aquila (Italy), has been dealt with.
The investigated historic center was hit by the 2016 Central Italy earthquake and reported several damage that have been represented by means of a Damage Probability Matrix, as well through a GIS map.
The vulnerability evaluation has been performed through the application of two simplified methods.
The first is an empirical method calibrated by the authors after the 2009 L'Aquila earthquake, based on observations carried out on similar historical centers. It has been applied on 130 buildings of the historic center of Campotosto, for which the main structural features have been identified.
The latter, is a methodology based on the use of the Vulnus software, developed at the University of Padua. It has been applied on a clustered building formed by four structural units, which has been considered as representative of the whole built environment.
The main conclusions of the study can be summarized as follows:
• The reconnaissance activity carried out after the 2016 Central Italy earthquake proved that the damage distribution can be satisfyingly interpreted by a binomial probability function, which entails that the proposed empirical method for the vulnerability evaluation can be applied.
• The considered clustered building is characterized by a fragility curve for severe damage (damage grade higher than D3) that well fit the fragility curve given by the EMS-98 macroseismic scale for a Vulnerability Class A.
• The fragility curve given by the analytical method based on the Vulnus evaluation is higher than the one provided by the empirical method [given by transforming the IMCS intensity in the ground acceleration according to Margottini et al. (1987)], as it is shown in Figure 18. However, the registered scatters are quite acceptable (about 15%) for ground acceleration higher than 0.4 g.
• The high scatters for ground accelerations that are lower than 0.4 g can be justified by the fact that a unique cluster buildings is not sufficient to well esteem the vulnerability. For this reason, in the future, evaluations about the minimum number of aggregated buildings to be considered for the assessment have to be carried out.
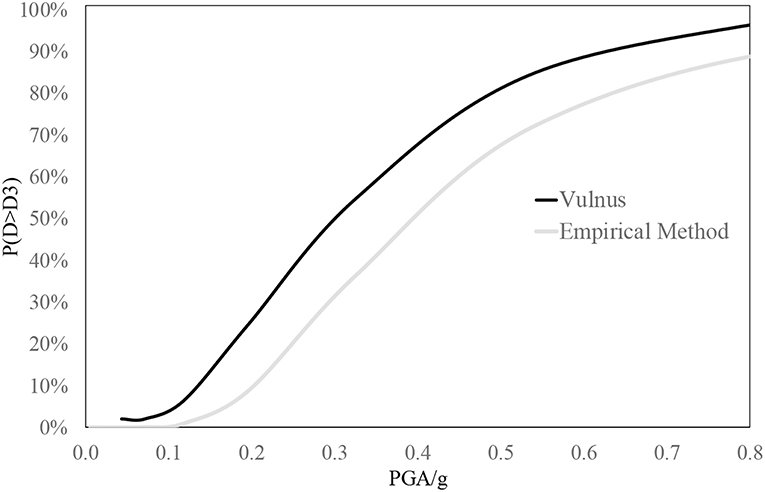
Figure 18. Comparison between the fragility curves given by Vulnus and by the empirical method for a damage grade higher than D3.
It must be pointed out that, apart from the limited number of clustered buildings considered for the evaluation, other issues that have been only briefly mentioned in the paper, such as the one related to the site effects due to the soil typology, are worthy of being deepening, as they could influence significantly the proposed outcomes.
Data Availability
The datasets generated for this study are available on request to the corresponding author.
Author Contributions
All authors listed have made a substantial, direct and intellectual contribution to the work, and approved it for publication.
Conflict of Interest Statement
The authors declare that the research was conducted in the absence of any commercial or financial relationships that could be construed as a potential conflict of interest.
Acknowledgments
The study presented in this paper has been carried out in the framing of the ReLUIS Research Project, funded by the Italian Civil Protection.
The authors gratefully acknowledge the support provided by Dr. Marco Munari for the use of the Vulnus software.
Footnotes
1. ^Circolare n. 7 21/01/2019. Istruzioni per l'applicazione delle “Norme tecniche per le costruzioni, di cui al D.M. 17/01/2018” [Italian].
2. ^D.M. 17/01/2018N. NTC 2018. Norme tecniche per le costruzioni [Italian].
References
Anastasopoulos, D., De Smedt, M., Vandewalle, L., De Roeck, G., and Reynders, E. P. B. (2018). Damage identification using modal strains identified from operational fiber-optic Bragg grating data. Struct. Health Monitor. 17, 1441–1459. doi: 10.1177/1475921717744480
Bernardini, A., Gori, R., Modena, C., Valluzzi, M. R, Benincà, G., Barbetta, E., et al. (2009). Vulnus Vb 4.0: Procedura Automatica per Analisi di Vulnerabilità Sismica di Edifici in Muratura. Available online at: http://hdl.handle.net/11577/3150148
Brando, G., Criber, E., and De Matteis, G. (2015). The effects of L'aquila earthquake on the St. Gemma church in Goriano Sicoli: part II—fem analysis. Bull. Earthquake Eng. 13, 3733–3748. doi: 10.1007/s10518-015-9793-3
Brando, G., De Matteis, G., and Spacone, E. (2017a). Predictive model for the seismic vulnerability assessment of small historic centers: application to the inner Abruzzi Region in Italy. Eng. Struct. 153, 81–96. doi: 10.1016/j.engstruct.2017.10.013
Brando, G., Rapone, D., Spacone, E., O'Banion, M. S., Olsen, M. J., Barbosa, A. R., et al. (2017b). Damage reconnaissance of unreinforced masonry bearing wall buildings after the 2015 Gorkha, Nepal, Earthquake. Earthquake Spectra 33(Special issue 1), S243–S273. doi: 10.1193/010817EQS009M
Cascini, L., Gagliardo, R., and Portioli, F. (2018). LiABlock,_3D: a software tool for collapse mechanism analysis of historic masonry structures. Int. J. Archit. Herit. doi: 10.1080/15583058.2018.1509155. [Epub ahead of print].
Clementi, F., Pierdicca, A., Formisano, A., Catinari, F., and Lenci, S. (2017). Numerical model upgrading of a historical masonry building damaged during the 2016 Italian earthquakes: the case study of the Podestà palace in Montelupone (Italy). J. Civil Struct. Health Monitor. 7, 703–717. doi: 10.1007/s13349-017-0253-4
Criber, E., Brando, G., and De Matteis, G. (2015). The effects of L'Aquila earthquake on the St. Gemma church in Goriano Sicoli: part I—damage survey and kinematic analysis. Bull. Earthquake Eng. 13, 3713–3732. doi: 10.1007/s10518-015-9792-4
D'Onofrio, K., and Tatoni, S. (2017). Third Level Micro-zonation of the Municipality of Campotosto (Italian). Available online at: https://www.comune.campotosto.aq.it/microzonazione-sismica/
Ferreira, T. M., Vicente, R., Mendes da Silva, J. A. R., Varum, H., and Costa, A. (2013). and Seismic vulnerability assessment of historical urban centers: case study of the old city center in Seixal Portugal. Bull. Earthquake Eng. 11, 1753–1773. doi: 10.1007/s10518-013-9447-2
Fiorentino, G., Forte, A., Pagano, E., Sabetta, F., Baggio, C., Lavorato, D., et al. (2018). Damage patterns in the town of Amatrice after August 24th 2016 Central Italy earthquakes. Bull. Earthquake Eng. 16, 1399–1423. doi: 10.1007/s10518-017-0254-z
Formisano, A. (2017a). Local- and global-scale seismic analyses of historical masonry compounds in San Pio delle Camere (L'Aquila, Italy). Nat. Hazards 86, 465–487. doi: 10.1007/s11069-016-2694-1
Formisano, A. (2017b). Theoretical and numerical seismic analysis of masonry building aggregates: case studies in San Pio Delle Camere (L'Aquila, Italy). J. Earthquake Eng. 21, 227–245. doi: 10.1080/13632469.2016.1172376
Formisano, A., and Marzo, A. (2017). Simplified and refined methods for seismic vulnerability assessment and retrofitting of an Italian cultural heritage masonry building. Comput. Struct. 180, 13–26. doi: 10.1016/j.compstruc.2016.07.005
GNDT (1994). Scheda di Vulnerabilità di 2° Livello (Muratura). Available online at: https://emidius.mi.ingv.it/GNDT2/Pubblicazioni/Lsu_96/vol_1/schede.pdf
Grünthal, G. (1998). Ed European Macroseismic Scale 1998. Luxembourg: Cahiers du Center Europèen de Gèodynamique et de Seismologie. Conseil de l'Europe.
Krstevska, L., Tashkov, L., Naumovski, N., Florio, G., Formisano, A., Fornaro, A., et al. (2010). “In-situ experimental testing of four historical buildings damaged during the 2009 L'Aquila earthquake,” in COST ACTION C26: Urban Habitat Constructions Under Catastrophic Events - Proceedings of the Final Conference, ed Mazzolani (London: Taylor & Francis Group), 427–432.
Lagomarsino, S., and Giovinazzi, S. (2006). Macroseismic and mechanical models for the vulnerability and damage assessment of current buildings. Bull. Earthquake Eng. 4, 415–443. doi: 10.1007/s10518-006-9024-z
Margottini, C., Molin, D., Narcisi, B., and Serva, L. (1987). “Intensity vs. acceleration: Italian data,” in Proceedings of the Workshop on Historical Seismicity of Central-Eastern Mediterranean Region (Roma: ENEA-IAEA), 213–226.
Paolucci, R., Pacor, F., Puglia, R., Ameri, G., Cauzzi, C., and Massa, M. (2011). “Record processing in ITACA, the new Italian strong motion database,” in Earthquake Data in Engineering Seismology, Geotech- nical, Geological and Earthquake Engineering Series, Vol. 14, eds S. Akkar, P. Gulkan, and T. Van Eck (Berlin: Springer Science + Business Media B.V.), 99–113.
Ponzi, A., Tempesta, G., Di Napoli, E., Colasante, P., Limiti, F., Limiti, P., et al. (2013). Reconstruction Plan of the di Campotosto Municipality Following the Seismic Event of the 6th of April 2009 (Italian). Available online at: https://www.comune.campotosto.aq.it/vecchio/index.php?option=com_content&view=article&id=79&Itemid=200
Portioli, F., and Cascini, L. (2017). Large displacement analysis of dry-jointed masonry structures subjected to settlements using rigid block modelling. Eng. Struct. 148, 485–496. doi: 10.1016/j.engstruct.2017.06.073
Rapone, D., Brando, G., Spacone, E., and De Matteis, G. (2018). Seismic vulnerability assessment of historic centers: description of a predictive method and application to the case study of Scanno (Abruzzi, Italy). Int. J. Archit. Herit. 12, 1171–1195. doi: 10.1080/15583058.2018.1503373
Sorrentino, L., Cattari, S., da Porto, F., Magenes, G., and Penna, A. (2018). Seismic behaviour of ordinary masonry buildings during the 2016 central Italy earthquakes. Bull. Earthquake Eng. 33, 1–25. doi: 10.1007/s10518-018-0370-4
Sorrentino, L., Doria, M., Tassi, V., and Liotta, M. (2019). Performance of a far-field historical church during the 2016-2017 central Italy earthquakes. J. Performance Constructed Facil. 33:04019016. doi: 10.1061/(ASCE)CF.1943-5509.0001273
Tashkov, L., Krstevska, L., Naumovski, N., De Matteis, G., and Brando, G. (2010). “Ambient vibration tests on three religious buildings in Goriano Sicoli damaged during the 2009 L'Aquila earthquake,” in COST ACTION C26: Urban Habitat Constructions Under Catastrophic Events - Proceedings of the Final Conference (London), 433–438.
Turnsek, V., and Cacovic, F. (1971). “Some experimental results on the strength of brick masonry walls,” in Proceedings of 2nd International Brick Masonry Conference (Stoke on Trent).
Keywords: historic centers, aggregate buildings, seismic vulnerability assessment, damage scenarios, masonry buildings, empirical methods, analytical methods, 2016 Central Italy earthquake
Citation: Cocco G, D'Aloisio A, Spacone E and Brando G (2019) Seismic Vulnerability of Buildings in Historic Centers: From the “Urban” to the “Aggregate” Scale. Front. Built Environ. 5:78. doi: 10.3389/fbuil.2019.00078
Received: 11 March 2019; Accepted: 22 May 2019;
Published: 11 June 2019.
Edited by:
Antonio Formisano, University of Naples Federico II, ItalyReviewed by:
Francesco Fabbrocino, Pegaso University, ItalyLiborio Cavaleri, University of Palermo, Italy
Copyright © 2019 Cocco, D'Aloisio, Spacone and Brando. This is an open-access article distributed under the terms of the Creative Commons Attribution License (CC BY). The use, distribution or reproduction in other forums is permitted, provided the original author(s) and the copyright owner(s) are credited and that the original publication in this journal is cited, in accordance with accepted academic practice. No use, distribution or reproduction is permitted which does not comply with these terms.
*Correspondence: Giuseppe Brando, Z2l1c2VwcGUuYnJhbmRvQHVuaWNoLml0