Tropical Cyclone Impacts on Cities: A Case of Hong Kong
- Guy Carpenter Asia-Pacific Climate Impact Centre, School of Energy and Environment, City University of Hong Kong, Hong Kong, China
The Pearl River Delta region in South China is known as a significant hotspot of tropical cyclone (TC) risk, which calls for an in-depth evaluation of TC damages and its determinants to support mitigation of associated impacts (e.g., loss of lives and infrastructure, and economic damages). Hence, in the context of TCs and cities, this study examines the historical impacts of TCs in Hong Kong and evaluates the association between economic losses and different TC hazards/parameters (i.e., wind, rainfall, and storm surge). An analysis of the reported TC-caused damages during 1988–2018 suggests a strong positive relationship between the normalized economic damages (Damage/GDP) and TC rainfall (p < 0.001, R2 = 0.43). This relationship for TC wind (explained by the power dissipation index—PDI) is positively statistically significant (>90% confidence) but insignificant for TC-induced storm surge. These results ascertain that among all three TC hazards, rainfall is the most significant factor for economic losses in Hong Kong followed by the PDI. However, it is further found that TC rainfall has significantly decreased (p < 0.01), while PDI has increased (93% confidence) during 1988–2018. Hence, it is obvious that the increasing economic losses in Hong Kong cannot be associated with TC rainfall intensification as it has decreased while the damages are still increasing. The increasing PDI, population rise over the past few decades and growing number of buildings in Hong Kong to accommodate this population are likely the major reasons behind these increasing damages. However, further in-depth evaluations are recommended in this regard to improve our understanding regarding tropical cyclones and cities. This study further calls for such evaluations in other coastal cities to identify the city-specific significant determinants of TC-related damages in the context of supporting damage mitigation in the future.
Introduction
Regardless of the debate on the increase in the frequency or intensity of natural hazards worldwide, the damages caused by them have potentially increased in different regions of the world (Neumayer and Barthel, 2011; Elsevier, 2017; Munich Re, 2017). Among these natural hazards, tropical cyclones (TCs), because of their strong wind, heavy rainfall, and high storm surge, are one of the most destructive hazards in terms of economic damages and impacts on socio-ecological systems particularly in the coastal regions of the most active TC basins such as the Western North Pacific and North Atlantic (Weinkle et al., 2018; Pugatch, 2019; Lee et al., 2020; Sajjad et al., 2020b). However, different regions (i.e., highly urbanized coastal cities, sub-urban areas, and rural areas) are not equally sensitive to the three hazards of TCs (i.e., wind, rainfall, and storm surge) due to specific environmental settings, and the threats of these hazards are spatially dynamic (Boyd et al., 2017; Hsiang et al., 2017). For example, in some coastal areas, TC wind is a major concern than the storm surge and vice versa. Therefore, understanding TC-related impacts along with the relationship between the damages and TC-induced hazards (i.e., wind, rainfall, and storm surge) is a critical concern in the context of future risk reduction.
Coastal cities, especially those being the hubs of economic activities and hence generally having a higher population density, bear the brunt of these impacts. Given the climate change influence on global TC activity, these impacts could multiply rapidly in the future (Gettelman et al., 2018; Marsooli et al., 2019; Lee et al., 2020; Liu and Chan, 2020). In order to manage (mitigation and reduction) the worsening impacts of these weather systems and increase future vigilance, it is essential to assess area-specific higher spatial-resolution trends of TC-related damages and to estimate the significance/sensitivity of different TC-related hazards (i.e., wind, rainfall, and storm surge) to damages. While the former is an operative way of assessing the effectiveness of different efforts made to reduce the impacts and, thus, has high policy relevance, the latter is helpful in designing strategies and action plans for the future. Furthermore, assessing the relationship between economic losses and different TC-induced hazards can progressively identify the driving forces behind the damages, making it easy for risk planners and decision-makers to come up with appropriate counter measures.
Along the China coast, the Pearl River Delta region, situated in the most active global TC-basin (i.e., the Western North Pacific), has been found to be a hotspot of TC risk (Wen et al., 2018; Sajjad and Chan, 2019; Sajjad et al., 2020a). This situation necessitates the evaluation of TC-related damages, economic losses, and its different determinants to articulate operational strategies for future vigilance and TC-related cost reduction. This need is particularly imperative for coastal cities due to rapid urbanization-induced huge capital investments, population growth, and infrastructure development. While many studies have been dedicated to assess the TC activity along the China coastline (e.g., Lin and Chan, 2015; Li et al., 2017; Liu and Chan, 2020), few, if any, have assessed trends in the economic losses caused by TCs in urban regions and its relationship with different TC-induced hazards (i.e., wind, rainfall, and storm surge). Therefore, in this study, we analyze the TC-related impacts and its relationship with the aforementioned TC hazards, if any, using Hong Kong as a case study. For this purpose, TC-caused impacts (i.e., vessels damaged/sunk and person missing/died) and economic losses data are used and a standard least squares regression model is proposed to identify the significant driving factors of the damages in Hong Kong. While the results from trend analysis will help to explore the state of impacts and economic losses through time, the identification of the most significant TC-induced hazard toward these impacts will be particularly useful in TC risk reduction.
Materials and Methods
The data for this study are compiled from websites of the Hong Kong Observatory (HKO),1 the Census and Statistics Department2 and the Buildings Department3 of the Hong Kong Special Administrative Region of China, and the China Meteorological Administration (CMA).4 The data on economic damages caused by each individual TCs during 1988–2018 are retrieved from the annual reports of specific years available at the HKO website. Based on the information available for Hong Kong, we complied three types of datasets to analyze the trends in TC-related impacts, including the number of vessels damaged/sunk due to TCs (1961–2018), number of people dead/went missing (1961–2018), and total economic losses due to TCs (1988–2018). To examine the long-term trends (upward or downward) in these impacts in Hong Kong (i.e., vessels damaged/sunk, people missing/dead, and economic losses), we estimate the linear correlation of all three series of impacts with time, individually.
For TC-related damages, many researchers have found that the significant increasing trends for actual losses from natural hazards become insignificant after “normalization” (Neumayer and Barthel, 2011; Barthel and Neumayer, 2012; Martinez, 2018; Weinkle et al., 2018). This situation implies that recent larger reported damages due to landfalling TCs might not only be related to more intense TCs but also the increasing development in coastal regions, which could have resulted in increased exposure. There are typically several approaches adopted by different researchers to normalize the economic losses (e.g., Pielke and Landsea, 1998; Pielke et al., 2003; Neumayer and Barthel, 2011; Weinkle et al., 2018; Grinsted et al., 2019). In this study, we follow Neumayer and Barthel (2011) and normalize the damages by dividing the losses of each year with that year’s gross domestic product (GDP). It is noted that as the GDP refers to the monetary value of all the goods produced and services provided, it is used as a proxy of wealth for a specific year, but not as a full representation of the total wealth in a city. For instance, rather than representing the total wealth of a city, GDP describes the changes in socio-economic aspects resulting in higher or lower market values for a specific year. This method is relatively robust and does not necessitate a base-year as required in other conventional approaches (Neumayer and Barthel, 2011).
To determine the relationship between normalized economic damages (NDam.) and a TC hazard, we propose the regression model as:
where the dependent variable is the natural-log of the normalized economic damage, and φ is the independent variable represented by any TC parameter [i.e., wind (km/h), rainfall (mm), and storm surge (m)] of TC i, and μi the random error. The purpose of using the log-transformed variables is to convert the data to near-normal distribution as it reduces the skewness of the distribution from 5.6 to −0.5.
In order to determine the most significant hazard/parameter for the economic damages, the above model can be modified to account for all the TC hazards as:
where μi and i are the same as above. In Eq. 2, PDI is the power dissipation index (unit: 105 knots3 in this study) estimated as the cube of the maximum sustained wind. This index is introduced by Emanuel (2005) to represent the wind-related destructiveness of a landfalling TC, which characterizes the TC wind hazard, and has been adopted by many researchers (e.g., Liu and Chan, 2017; Sajjad et al., 2019). The PDI for TC i is calculated as:
where Vmax represents the maximum sustained wind speed of TC i.
The wind observation at the HKO station is used to calculate the PDI. The rainfall (mm) is the total rainfall during the passage of a TC observed at the Airport station in Hong Kong. Storm surge hazard is represented by the maximum storm surge in meters (above astronomical tide) at the Quarry Bay station in Hong Kong. Although it would be ideal to use the data of these parameters from the same station, we were unable to find the data for the same period for the study area. Using the only available data at the same station would have reduced the number of observations significantly, which could have compromised the regression model. Therefore, for the sake of reliability, we use the data from the available stations to have a longer time period series of the observations and to best fit the regression model.
Results
Tropical Cyclone-Related Impacts on Hong Kong
The trends in three different types of impacts from TCs (i.e., damage to vessels, persons dead/missing due to TCs, and total economic damages) are analyzed (Figure 1). While the impacts of TCs on vessels have been fluctuating throughout the time period (Figure 1A), the number of vessels sunk/damaged due to TCs during 1960–2019 shows a statistically significant increase (99% confidence, solid line in Figure 1A). On the other hand, a significant decrease in the number of people died/missing due to TCs over the past half century is observed (99% confidence, Figure 1B).
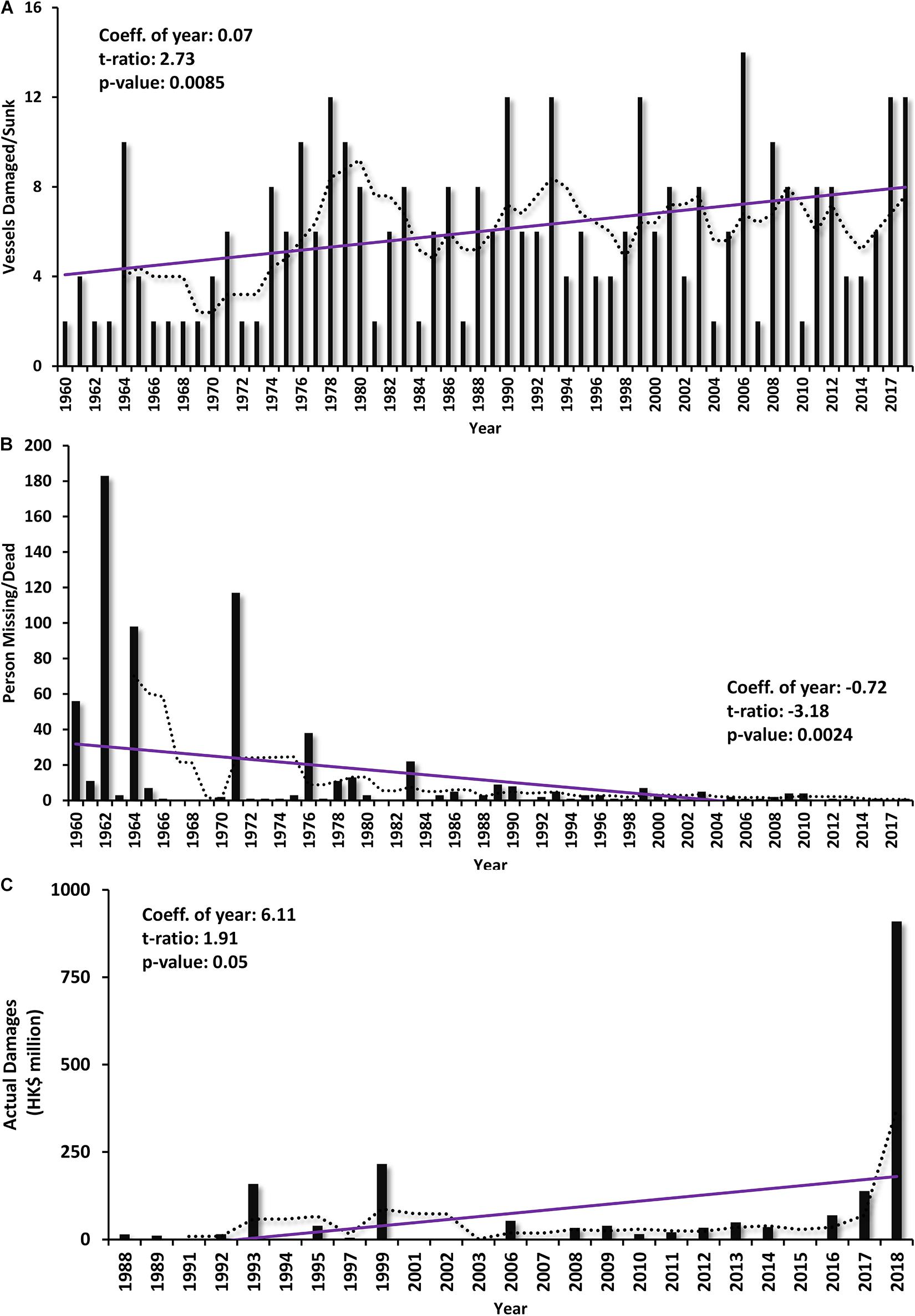
Figure 1. Time series of TC-related impacts in Hong Kong. (A) number of vessels (sunk/damaged), (B) number of persons died/missing, and (C) actual observed TC-related damages (millions HK$). The solid purple lines show the long-term trend (correlation between the series and time). The dotted black line is the 5-year moving average.
The total economic damages due to TCs in Hong Kong shows an overall increasing trend during 1988–2018, which is statistically significant at p = 0.05 (Figure 1C). This increasing trend is continuous, much obvious during the recent decade (i.e., after 2005). The largest damages in Hong Kong to date are caused by Super Typhoon Mangkut in 2018 (HK$ ∼910 million, or close to US$ 120 million5) before normalization, which remains the largest even after normalizing with the GDP for the year 2018 (i.e., normalized value = 0.02). Based on these data, Hong Kong has experienced approximately HK$ 30 million TC-related economic losses each year on the average during 1988–2018. The average annual losses before 2005 were approximately HK$ 15 million and increased to approximately an average of HK$ 48.2 million after 2005. This reflects a significant increase in the TC-related economic losses in Hong Kong over the past decades.
Normalized Damages and Its Relationship With Different TC-Induced Hazards
Although the actual observed damages caused by TCs in Hong Kong show an overall upward trend during 1988–2018 (see Figure 1C), this trend becomes statistically insignificant (p = 0.35) after normalizing the total damages by the GDP of the corresponding year (Figure 2). However, even the trend is insignificant, one cannot neglect the fact that the damages have increased and been more frequent, particularly in the recent decade (i.e., after 2005).
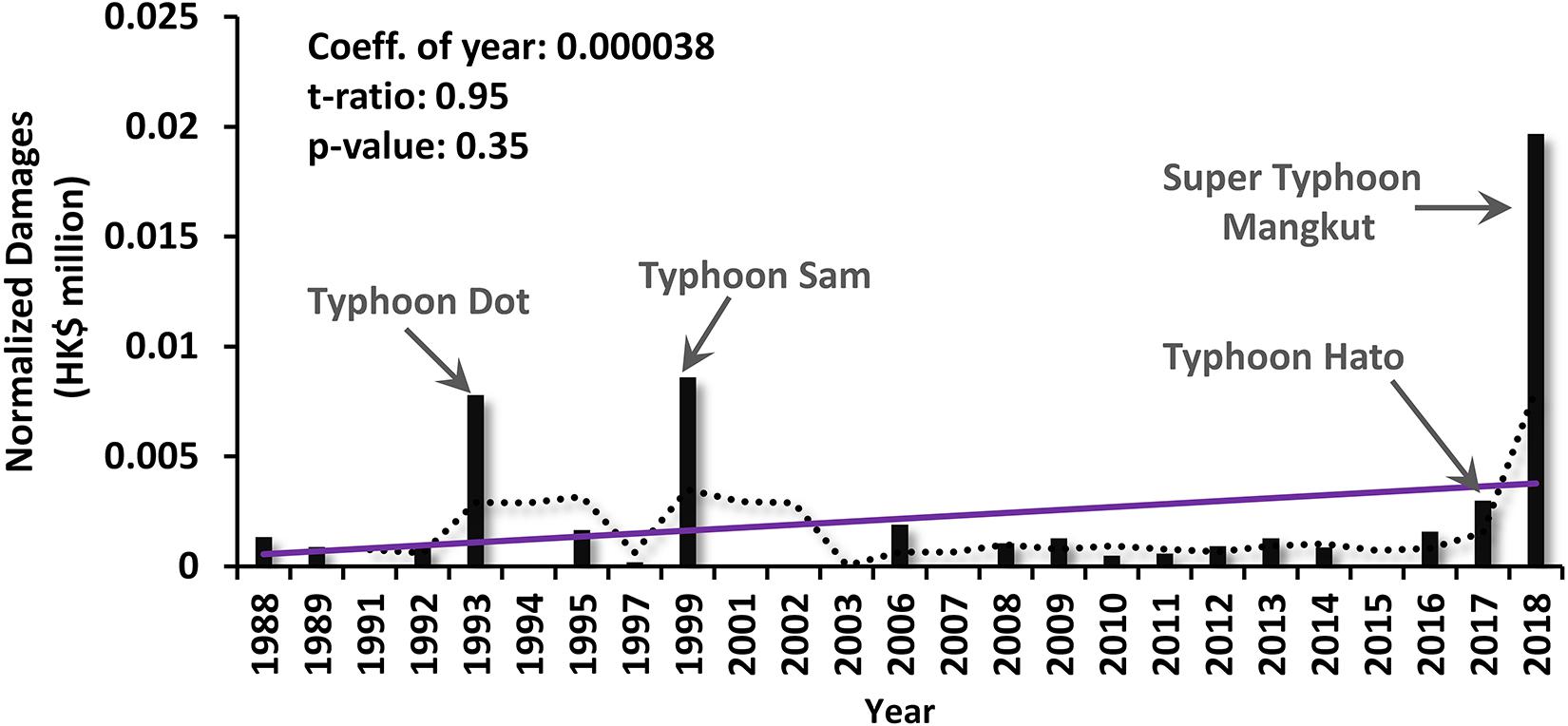
Figure 2. Normalized time series of TC-related damages (NDam.) in Hong Kong. The solid purple line shows the long-term trend (correlation between the series and time). The dotted black line is the 5-year moving average.
The Standard Least Squares regression (S-LSR) between NDam. and the individual TC-induced hazards reveals a statistically significant relationship (95% confidence, Table 1) between the damages and all the parameters (i.e., PDI, rainfall, and storm surge). The goodness-of-fit values (R-squared values) for these individual models (0.22, 0.26, and 0.2 for wind, rainfall, and storm surge, respectively) suggest that the relationship involving rainfall is the strongest.
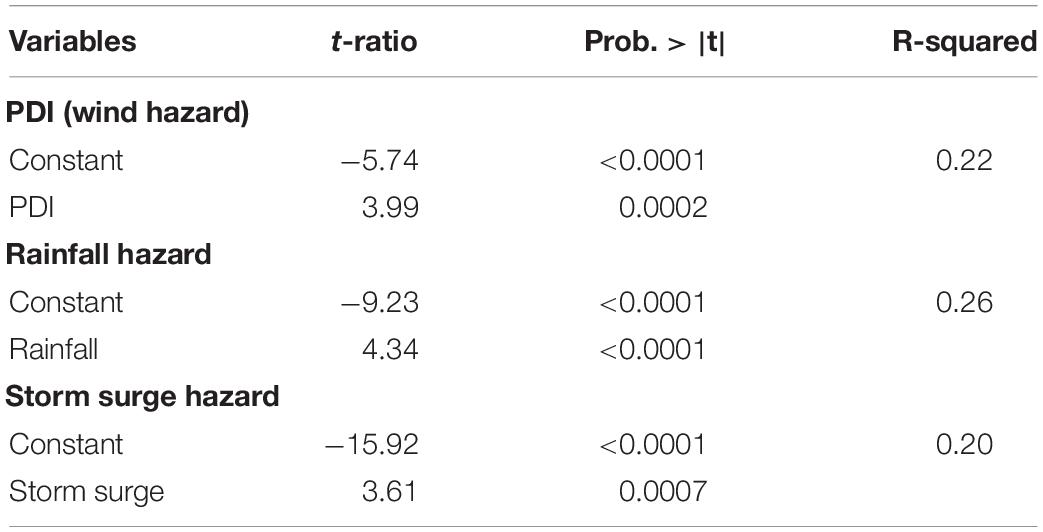
Table 1. Individual Standard Least Squares regression (S-LSR) models to explore the relationship between normalized damages (NDam.) caused by TCs in Hong Kong and different TC parameters (i.e., PDI, rainfall, and storm surge).
We further model the relationship between normalized damages (NDam.) and TC-induced hazards using a multivariate S-LSR model using the log-transformed NDam. as the dependent variable. This multivariate model performs better than the individual models (Table 2), with the goodness-of-fit for the multivariate model being much higher (explaining 43% variance in the data) than the individual models. The estimate value (β-coefficient) for wind is the largest (3.29) followed by rainfall (1.46) and storm surge (0.8), which indicates that TC-related damages in Hong Kong are relatively more sensitive to wind. However, the significance of rainfall is higher than wind (t-value being the larger), which ascertains that rainfall parameter of TCs is the most relevant in the case of Hong Kong when it comes to economic damages, followed by PDI. Whereas, TC storm surge has the least effect on TC-related economic damages in Hong Kong.
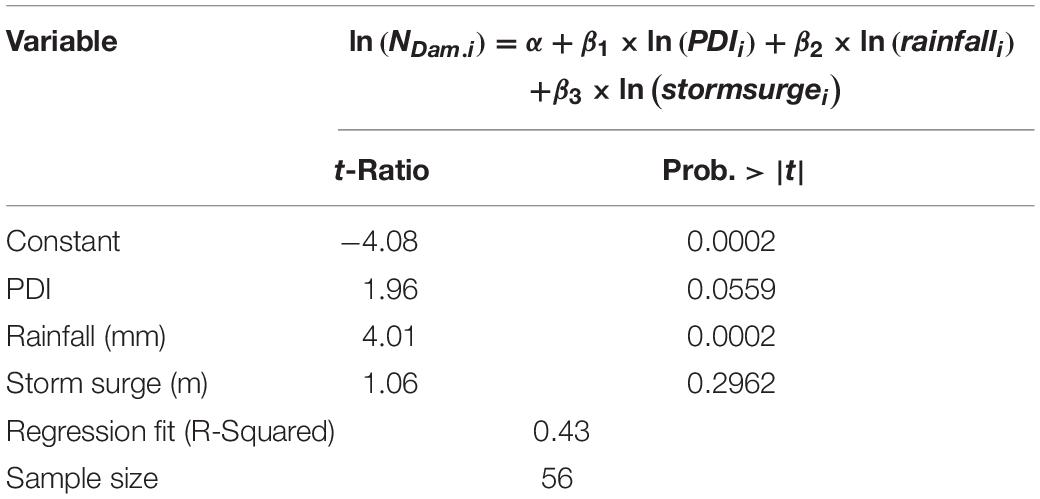
Table 2. Regression results based on multivariate S-LSR for TC-related damages and different TC parameters (i.e., wind, rainfall, and storm surge).
TC Characteristics in Hong Kong
In view of the above, we analyze TC characteristics in Hong Kong to investigate whether the increase in damages is due to change in the frequency of TCs affecting Hong Kong and in associated wind strength, rainfall amount, and/or storm surge height.
Frequency Analysis
On the average, approximately 16 TCs come within 600 km radius of Hong Kong annually (the area of Hong Kong jurisdiction described in the Tropical Cyclone Annual Publications from the HKO) (Figure 3A). However, there is an overall statistically significant decreasing trend (p < 0.05) during 1960–2018. Based on the Mann-Kendall monotonic trend estimation, there is a decrease of ∼2 TCs (Test Z-value = -1.8, significant at 90%) between 1961 and 2018. On the other hand, around six TCs (averaged over 1960–2018) affect Hong Kong each year (TCs which required the issuing of the Tropical Cyclone Warning Signal from the HKO, Figure 3B). The time series for the same period shows an overall decreasing trend of TCs affecting Hong Kong although it is not statistically significant (p = 0.23 > 0.05). A closer examination of the TC frequency below-/above-average number of TCs during 1960–2018 suggests a multi-decadal variation (Figure 3C), with relatively more above-average TCs (No. of TCs > 6) during 1960–1990 but more below-average TCs for most of the years after 1990 (No. of TCs < 6).
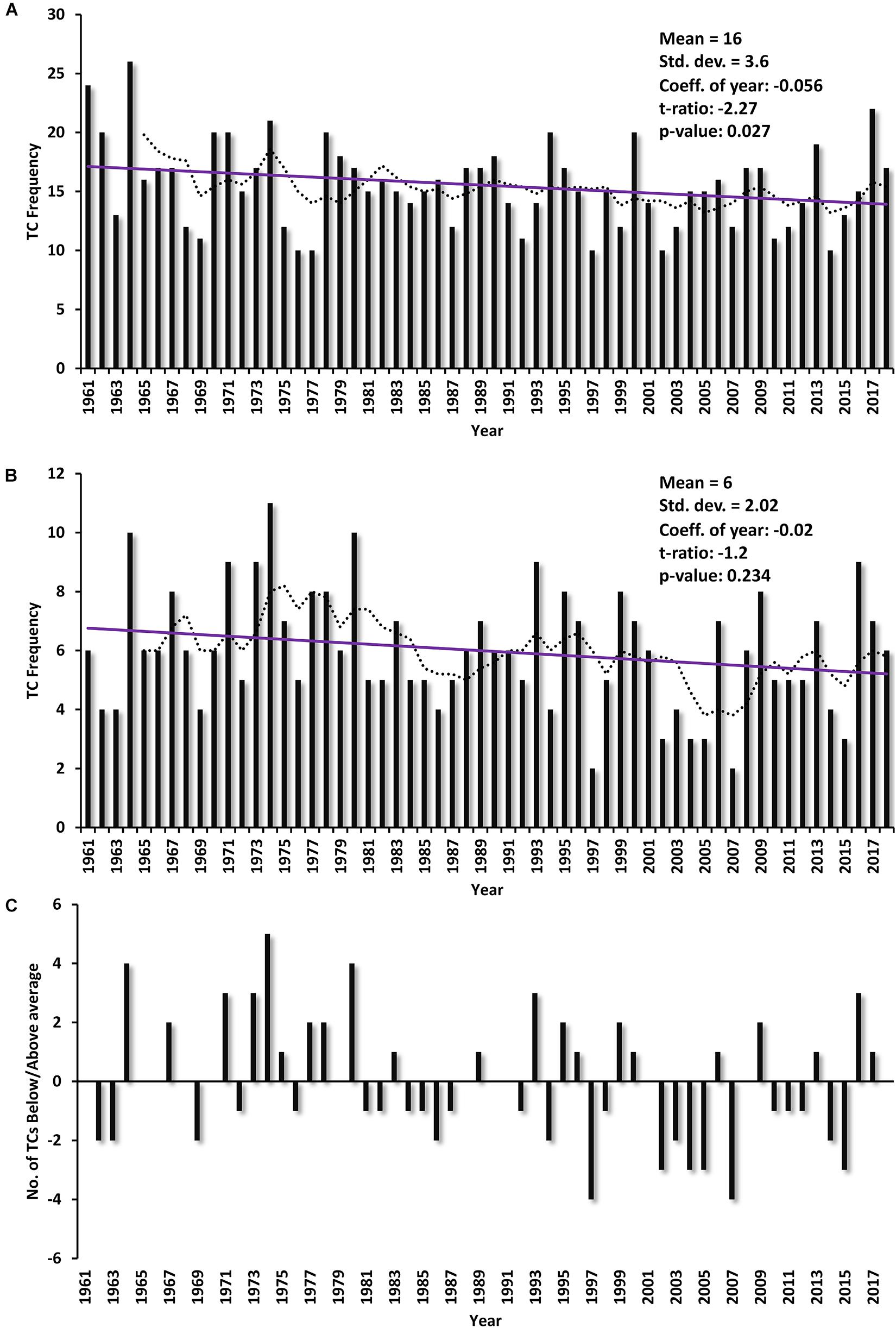
Figure 3. (A) Total number of TCs within the jurisdiction of Hong Kong (600 km radius as described in the Tropical Cyclone Annual Publications: www.hko.gov.hk). (B) Number of TCs affecting Hong Kong, and (C) number of TCs above or below overall average. The solid purple lines in (A,B) show the long-term trend (correlation between the series and time) at a confidence interval of 95% (p = 0.05) and the dotted black lines are the 5-year moving averages. All the data are for the period 1960–2018.
Temporal Variation of TC-Related Wind, Rainfall, and Storm Surge
Among all three TC-induced hazards, PDI and storm surge show an increasing trend (Figures 4A,C). However, this increasing trend is only significant for PDI (93% confidence), reflecting that the TC affecting Hong Kong during 1988–2018 have become more destructive in the context of TC-induced wind hazard. On the contrary, rainfall has a statistically significant decreasing trend (p = 0.039). The 5-year moving average for the rainfall also shows a decadal behavior of TC-induced rainfall in Hong Kong, which in recent years has experienced an increase. This recent increase in the rainfall might be the potential reason behind increasing TC-related damages, recently, as rainfall is the most significant hazard to explain the damages (Tables 1, 2).
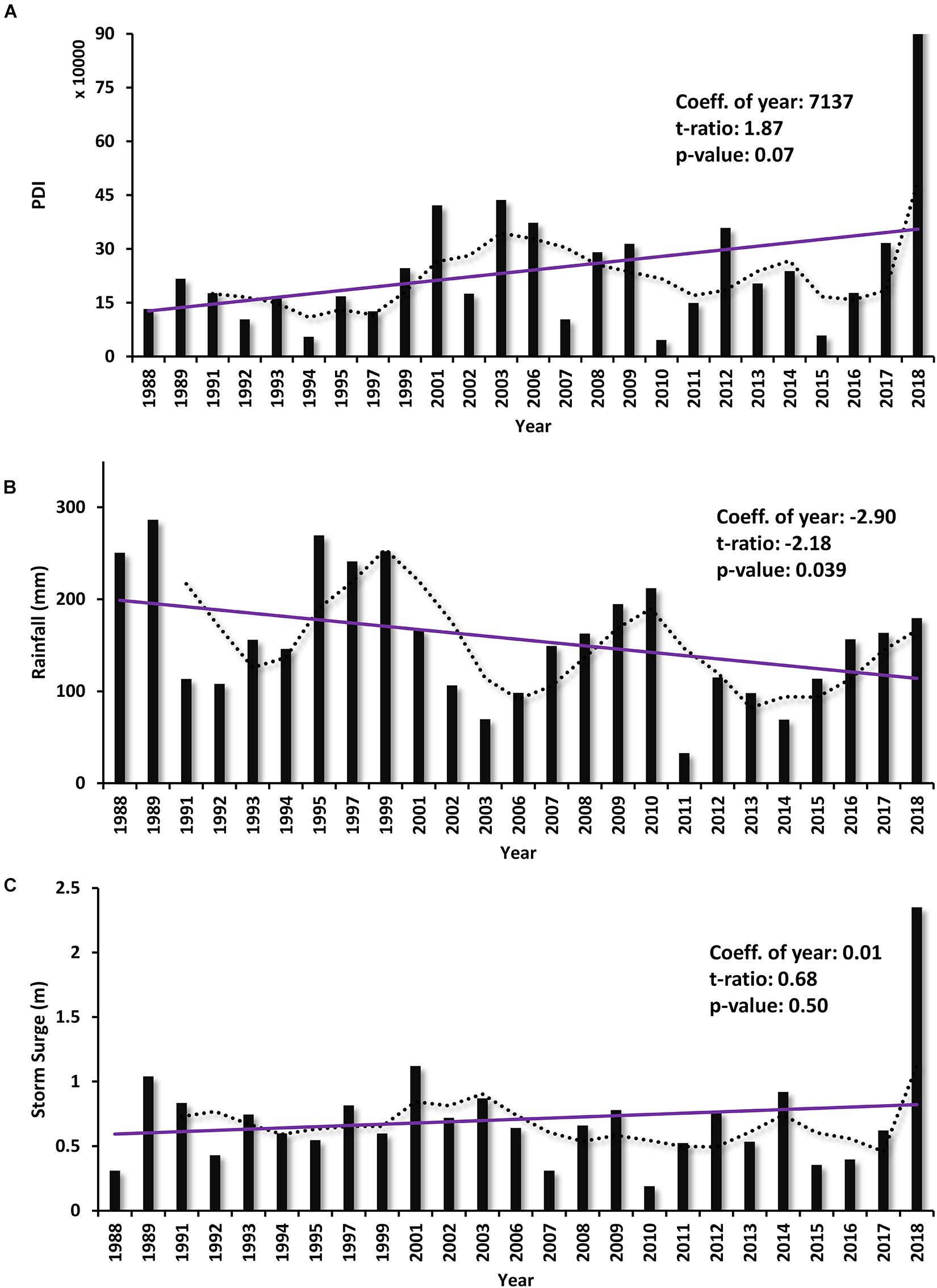
Figure 4. Time series of different TC-induced hazards (A) PDI, (B) rainfall, and (C) storm surge associated with TCs affecting Hong Kong. The solid purple lines show the long-term trend (correlation between the series and time) at a confidence interval of 95% (p = 0.05) and the dotted black lines are the 5-year moving averages.
Discussion
Reducing economic damages is a key indicator of progress toward disaster risk reduction and the mitigation of hazards impacts as described by the United Nations Sustainable Development Goals framework (Clark and Wu, 2016; Prajal et al., 2017; Peduzzi, 2019). In this context, an investigation of trends in damages can help progressively to assess the sustainable development. Considering the large and significant contribution of landfalling TCs to disaster losses, our study not only provides the first assessment on the long-term trends in TC-related impacts in Hong Kong but goes a step-ahead to identify the relationship of these impacts with different TC-induced hazards (i.e., wind, rainfall, and storm surge). It is important to note that through there is a significant increase in the TC-related non-normalized damages (95% confidence), this trend becomes insignificant after normalizing the damages with the corresponding years GDP. This finding is in line with the existing literature such as Neumayer and Barthel (2011).
It is notable that despite the decrease in TC frequency and rainfall in Hong Kong (Figures 3, 4B) the economic damages has increased (Figures 1C, 2). These increasing damages in Hong Kong can be explained by the increase in PDI (Figure 4A) to some extent. However, the significance of PDI is much lower than TC rainfall (Table 2). Another likely reason for this increasing damages could be an increase in the city’s population and the number of buildings (Figure 5), which have grown significantly (99% confidence) in recent decades resulting in higher exposure to landfalling TCs.
The increasing number of damaged/sunk vessels (99% confidence) are significantly related to PDI (t-Ratio = 3.53, p = < 0.01, R-squared = 0.35) and storm surge (t-Ratio = 3.22, p = < 0.01, R-squared = 0.31) based on the analysis of the relationship individual TC-induced hazards and the impacts on vessels. On the other hand, the multivariate S-LSR between damaged/sunk vessels (dependent variable) and the TC hazards (i.e., wind, rainfall, and storm surge) reveals that the impacts of TCs on vessels are positively significantly related to PDI only (t-Ratio = 2.86, p = < 0.01, R-squared = 0.55). However, one cannot neglect that a potential reason behind this increasing trend of impacts on vessels could be due to the increase in total number of vessels in Hong Kong as the city is an economic hub with one of the highest tourism activity in the world.
Similarly, a probable reason behind the continuous and recent (after 2005) increasing TC-related damages (Figure 1C) could be the growing annual TC frequency since 2008 and the annual maximum landfalling intensity (MLI) since 2012 (Liu and Chan, 2020) in South China. This increase in MLI is related to rapid intensification of TCs over South China Sea. Yet, it is still difficult to say for sure if the increase in TC-related damages in Hong Kong is due to this phenomenon as the significant TC hazard for economic losses in Hong Kong is found to be rainfall (Table 2). TC rainfall is likely to intensify in a warmer climate and with a possible reduction in the translational speed of landfalling TCs, cities that are the most sensitive to rainfall (i.e., Hong Kong) are expected to experience higher TC rainfalls, and consequently a larger threat of flooding, particularly in the most active TC basins and the most developed coastlines in the world (Geert-Jan-Van et al., 2017; Kossin, 2018; Trenberth, 2018; Lai et al., 2020).
As coastal development continues, cities should thus expect to be at greater risks to landfalling TCs in the future under the global environmental change. Implementation of robust, yet sustainable, risk mitigation policies under an uncertain future climate requires governments and societies to seek solutions to be more resilient. The Chinese coastline is dominated by hard engineered defenses (i.e., seawalls) since 1970, and Pearl River Delta region is no exception. Liu et al. (2019) conducted an analysis to evaluate the potential of hard engineered defenses vs. nature-based solutions (i.e., coastal wetlands) to mitigate the TC-related economic damages along Mainland China’s coast. They concluded that while the hard defenses in China provide 3.18 million per km on average for TC-related protection, coastal wetlands outweigh these hard defenses as they are more cost-effective. Similarly, adopting natural solutions to risk mitigation has been recognized as a progressive way-out globally (Temmerman et al., 2013; Sajjad et al., 2018; Mabon, 2019). Hence, adopting nature-based urban development or hybrid approaches (integrated natural and engineered) in Hong Kong should be explored and considered to cope with the increasing damages from TCs rather engineering-based defenses.
Regardless of the influence of climate change on TC frequencies and intensities in the future, the priority of communities should remain focused on addressing vulnerabilities and exposures to landfalling TCs. Thus, not only TC-/hazard-characteristics but development-induced societal changes in coastal regions particularly urbanization and related phenomenon (i.e., increase in urban population and building, economic activities, and businesses) needs to be considered when modeling future impacts and designing action plans, policies, and risk reduction strategies (Bakkensen and Larson, 2014; Aerts et al., 2018; Sajjad et al., 2020b).
Conclusion
In recent years, estimations of economic losses and impacts from landfalling TCs often focus on more intense and frequent TC events while neglecting the influences of societal changes. These societal changes (e.g., increasing socioeconomic development and capital investments) in coastal cities typically result in an increased exposure, and should be considered in evaluating the TC-related impacts in addition to the identification of signals in the changes in TC frequency, destructive potential, rainfall, and storm surge. The present study provides observational evidence on the trends in the historical damages and impacts caused by tropical cyclones (TCs) in Hong Kong to support this approach. Furthermore, a regression model is proposed to identify the relationship between the observed historical economic damages and different TC-induced hazards (i.e., wind, rainfall, and storm surge), if any. This regression model also helps to identify the most significant TC-induced hazard to explain economic damages in Hong Kong, providing a progressive opportunity to take appropriate actions.
The results show that while there is a significant increasing trend in the actual observed economic damages in Hong Kong during 1988–2018, this trend becomes insignificant after normalizing the damages with GDP of the corresponding year. Furthermore, among all three TC-induced hazards (i.e., wind, rainfall, and storm surge), wind and rainfall are statistically significantly (confidence 94 and 99.99%, respectively) related to economic damages in Hong Kong. On the other hand, TC rainfall has a significant decreasing trend (95% confidence). This decreasing trend in the most significant TC hazard to cause economic losses leads to the conclusion that rather than the changing TC characteristics, societal changes might have caused the recent increasing observed damages in Hong Kong, which should not be overlooked. The observed increasing economic losses might also be linked to an increase in the PDI of landfalling TCs though the increasing trend in the PDI is insignificant at p = 0.05. Similarly, though there is a positive relation between the storm surge and economic losses, this relationship is insignificant. To the best of our knowledge, this study is the first of its kind to provide a comprehensive assessment of TC-related damages in Hong Kong and put forth a discussion regarding the need for a city-specific identification of significant TC-induced hazard[s] to cause damages. Such identification could help corrective decision-making and designing appropriate measures more effectively considering local situations, which is often overlooked by the coarser national-scale studies. The approach adopted (i.e., regression model) in this study is equally important for other cities in Mainland China and beyond.
The authors do acknowledge the presence of several limitations in this study. Firstly, only 43% of the variance of the total TC-related damages is explained by the TC-induced hazards. A possible reason behind could be the larger variance (9.9) of ln(Damage/GDP), which is a relatively large variance for a variable that is log-transformed—as discussed in Liu et al. (2019). Additionally, due to the constraint of data availability regarding the TC-related historical damages, the sample size is relatively small (n = 56). A larger sample size might provide better result. Similarly, we use GDP to normalize the economic damages in this study, which is a proxy of the actual economic wealth of a city. Therefore, GDP might have understated the actual wealth status of Hong Kong. However, as the purpose here is to normalize the damages, GDP serves the purpose well and has been used in various studies globally (Neumayer and Barthel, 2011; Weinkle et al., 2018; Liu et al., 2019). Lastly, for the sake of proposing the model and highlighting the need of such studies, we only focus on Hong Kong in this study. However, it is of great importance and will be an interesting future research topic to conduct similar studies for other coastal cities to explore how different urban areas are more sensitive to different TC-induced hazards.
Data Availability Statement
All the data used in this study are available free of cost and the links to these data resources are provided within the article.
Author Contributions
MS proposed the idea, collected necessary data, performed data analysis (methods and visualization), and prepared the initial draft. JC was responsible to supervise the overall study and acquired the funding. MS and JC contributed toward discussions, editing, revision, and final manuscript preparation. Both authors contributed to the article and approved the submitted version.
Funding
This work was partly supported by a Research Studentship from City University of Hong Kong (000618) and the Research Grant Council of the Hong Kong Special Administration Region of China Grant E-CityU101/16.
Conflict of Interest
The authors declare that the research was conducted in the absence of any commercial or financial relationships that could be construed as a potential conflict of interest.
The handling editor declared a past co-authorship with one of the authors MS.
Footnotes
- ^ www.hko.gov.hk/
- ^ www.censtatd.gov.hk
- ^ www.bd.gov.hk/
- ^ http://www.cma.gov.cn/
- ^ Hereafter, all monetary data are in HK$. Readers can convert this to US$ using the approximate exchange rate of 1 US$ = 7.75 HK$ as of June 2020.
References
Aerts, J. C. J. H., Botzen, W. J., Clarke, K. C., Cutter, S. L., Hall, J. W., Merz, B., et al. (2018). Integrating human behaviour dynamics into flood disaster risk assessment. Nat. Clim. Chang. 8, 193–199. doi: 10.1038/s41558-018-008581
Bakkensen, L. A., and Larson, W. (2014). Population matters when modeling hurricane fatalities. Proc. Natl. Acad. Sci. 111, E5331–E5332. doi: 10.1073/pnas.1417030111
Barthel, F., and Neumayer, E. (2012). A trend analysis of normalized insured damage from natural disasters. Clim. Change 113, 215–237. doi: 10.1007/s10584-011-0331332
Boyd, E., James, R. A., Jones, R. G., Young, H. R., and Otto, F. E. L. (2017). A typology of loss and damage perspectives. Nat. Clim. Chang 7:723. doi: 10.1038/nclimate3389
Clark, H., and Wu, H. (2016). The sustainable development goals: 17 goals to transform our world. New York: UNDP, 36–54.
Emanuel, K. (2005). Increasing destructiveness of tropical cyclones over the past 30 years. Nature 436, 686–688. doi: 10.1038/nature03906
Geert-Jan-Van, O., Karin, van der, W., Antonia, S., Roop, S., Julie, A., et al. (2017). Attribution of extreme rainfall from Hurricane Harvey, August 2017. Environ. Res. Lett 12:124009. doi: 10.1088/1748-9326/aa9ef2
Gettelman, A., Bresch, D. N., Chen, C. C., Truesdale, J. E., and Bacmeister, J. T. (2018). Projections of future tropical cyclone damage with a high-resolution global climate model. Clim. Change 146, 575–585. doi: 10.1007/s10584-017-19021907
Grinsted, A., Ditlevsen, P., and Christensen, J. H. (2019). Normalized US hurricane damage estimates using area of total destruction, 1900-2018. Proc. Natl. Acad. Sci. U. S. A. 116, 23942–23946. doi: 10.1073/pnas.1912277116
Hsiang, S., Kopp, R., Jina, A., Rising, J., Delgado, M., Mohan, S., et al. (2017). Estimating economic damage from climate change in the United States. Science 356, 1362–1369. doi: 10.1126/science.aal4369
Kossin, J. P. (2018). A global slowdown of tropical-cyclone translation speed. Nature 558, 104–107. doi: 10.1038/s41586-018-0158-3
Lai, Y., Li, J., Gu, X., David, Y., Kong, D., and Yew, T. (2020). Greater flood risks in response to slowdown of tropical cyclones over the coast of China. Proc. Natl. Acad. Sci 117:14751. doi: 10.1073/pnas.1918987117
Lee, T.-C., Knutson, T. R., Nakaegawa, T., Ying, M., and Cha, E. J. (2020). Third Assessment on Impacts of Climate Change on Tropical Cyclones in the Typhoon Committee Region-Part I: Observed Changes, Detection and Attribution. Trop. Cyclone Res. Rev. 9, 1–22. doi: 10.1016/j.tcrr.2020.03.001
Li, R. C. Y., Zhou, W., Shun, C. M., and Lee, T. C. (2017). Change in destructiveness of landfalling tropical cyclones over China in recent decades. J. Clim. 30, 3367–3379. doi: 10.1175/JCLI-D-16-0258.1
Lin, I. I., and Chan, J. C. L. (2015). Recent decrease in typhoon destructive potential and global warming implications. Nat. Commun 6:7182. doi: 10.1038/ncomms8182
Liu, K. S., and Chan, J. C. L. (2017). Variations in the power dissipation index in the East Asia region. Clim. Dyn. 48, 1963–1985. doi: 10.1007/s00382-016-31853185
Liu, K. S., and Chan, J. C. L. (2020). Recent increase in extreme intensity of tropical cyclones making landfall in South China. Clim. Dyn. 55, 1059–1074. doi: 10.1007/s00382-020-053115315
Liu, X., Wang, Y., Costanza, R., Kubiszewski, I., Xu, N., Gao, Z., et al. (2019). Is China’s coastal engineered defences valuable for storm protection? Sci. Total Environ. 657, 103–107. doi: 10.1016/j.scitotenv.2018.11.409
Mabon, L. (2019). Enhancing post-disaster resilience by ‘building back greener’: Evaluating the contribution of nature-based solutions to recovery planning in Futaba County, Fukushima Prefecture, Japan. Landsc. Urban Plan. 187, 105–118. doi: 10.1016/j.landurbplan.2019.03.013
Marsooli, R., Lin, N., Emanuel, K., and Feng, K. (2019). Climate change exacerbates hurricane flood hazards along US Atlantic and Gulf Coasts in spatially varying patterns. Nat. Commun. 10:3785. doi: 10.1038/s41467-019-11755-z
Martinez, A. B. (2018). Improving normalized hurricane damages. Nat. Sustain 3:519 doi: 10.1038/s41893-020-0550555
Munich Re (2017). A stormy year: TOPICS Geo Natural catastrophes 2017. Available online at: https://www.munichre.com/site/touch-publications/get/documents_E711248208/mr/assetpool.shared/Documents/5_Touch/_Publications/TOPICS_GEO_2017-en.pdf doi: 10.1038/s41893-020-0551-4 (accessed April 26, 2020).
Neumayer, E., and Barthel, F. (2011). Normalizing economic loss from natural disasters: A global analysis. Glob. Environ. Chang. 21, 13–24. doi: 10.1016/j.gloenvcha.2010.10.004
Peduzzi, P. (2019). The Disaster Risk, Global Change, and Sustainability Nexus. Sustainability 11:957. doi: 10.3390/su11040957
Pielke, R. A., and Landsea, C. W. (1998). Normalized hurricane damages in the United States: 1925-95. Weather Forecast 13, 621–631. doi: 10.1175/1520-04341998013
Pielke, R. A., Rubiera, J., Landsea, C., Fernández, M. L., and Klein, R. (2003). Hurricane vulnerability in Latin America and the Caribbean: Normalized damage and loss potentials. Nat. Hazards Rev. 4, 101–114. doi: 10.1061/(ASCE)1527-698820034:3(101)
Prajal, P., Luís, C., Diego, R., and Wolfgang, L. (2017). A Systematic Study of Sustainable Development Goal (SDG) Interactions. Earth’s Futur 5, 1169–1179. doi: 10.1002/2017EF000632
Pugatch, T. (2019). Tropical storms and mortality under climate change. World Dev. 117, 172–182. doi: 10.1016/j.worlddev.2019.01.009
Sajjad, M., and Chan, J. C. L. (2019). Risk assessment for the sustainability of coastal communities: A preliminary study. Sci. Total Environ. 671, 339–350. doi: 10.1016/j.scitotenv.2019.03.326
Sajjad, M., Chan, J. C. L., and Kanwal, S. (2020a). Integrating spatial statistics tools for coastal risk management: A case-study of typhoon risk in mainland China. Ocean Coast. Manag. 184:105018. doi: 10.1016/j.ocecoaman.2019.105018
Sajjad, M., Lin, N., and Chan, J. C. L. (2020b). Spatial heterogeneities of current and future hurricane flood risk along the U.S. Atlantic and Gulf coasts. Sci. Total Environ. 713:136704. doi: 10.1016/j.scitotenv.2020.136704
Sajjad, M., Li, Y., Li, Y., Chan, J. C. L., and Khalid, S. (2019). Integrating Typhoon Destructive Potential and Social-Ecological Systems Toward Resilient Coastal Communities. Earth’s Futur. 7, 805–818. doi: 10.1029/2019EF001226
Sajjad, M., Li, Y., Tang, Z., Cao, L., and Liu, X. (2018). Assessing Hazard Vulnerability, Habitat Conservation, and Restoration for the Enhancement of Mainland China’s Coastal Resilience. Earth’s Futur. 6, 326–338. doi: 10.1002/2017EF000676
Temmerman, S., Meire, P., Bouma, T. J., Herman, P. M. J., Ysebaert, T., and De Vriend, H. J. (2013). Ecosystem-based coastal defence in the face of global change. Nature 504, 79–83. doi: 10.1038/nature12859
Trenberth, K. E. (2018). Hurricane Harvey Links to Ocean Heat Content and Climate Change Adaptation Earth’s Future. Earth’s Futur. 0, 1–15. doi: 10.1029/2018EF000825
Weinkle, J., Landsea, C., Collins, D., Musulin, R., Crompton, R. P., Klotzbach, P. J., et al. (2018). Normalized hurricane damage in the continental United States. Nat. Sustain 1, 1900–2017. doi: 10.1038/s41893-018-0165162
Keywords: economic damage, cities, typhoon impacts, risk reduction, coastal development
Citation: Sajjad M and Chan JCL (2020) Tropical Cyclone Impacts on Cities: A Case of Hong Kong. Front. Built Environ. 6:575534. doi: 10.3389/fbuil.2020.575534
Received: 23 June 2020; Accepted: 10 September 2020;
Published: 25 September 2020.
Edited by:
Ning Lin, Princeton University, United StatesReviewed by:
Swamy Selvi Rajan, Structural Engineering Research Centre (CSIR), IndiaLixiao Li, Shenzhen University, China
Copyright © 2020 Sajjad and Chan. This is an open-access article distributed under the terms of the Creative Commons Attribution License (CC BY). The use, distribution or reproduction in other forums is permitted, provided the original author(s) and the copyright owner(s) are credited and that the original publication in this journal is cited, in accordance with accepted academic practice. No use, distribution or reproduction is permitted which does not comply with these terms.
*Correspondence: Muhammad Sajjad, mah.sajjad@hotmail.com