- 1School of Engineering, University of Birmingham, Birmingham, United Kingdom
- 2Institute of Transportation Studies, University of California Berkeley, Berkeley, CA, United States
Introduction
In the past several years, global warming has caused essential issues that all sectors must respond immediately. The Paris Agreement has turned into a critical framework provoking public and private sectors worldwide (Dimitrov, 2016; Pye et al., 2017). One approach to solving this problem involves the use of green energies and reducing CO2 emitted from all sectors.
Regarding the transportation sectors, it emitted CO2 above one-fourth of the global emission. A well-known problem with over emission is that some countries have inadequate public transportation and non-environmental policies. The low-fare service and high accessibility on public transit are major strategies to reduce emission from a private car (Krishnan et al., 2015; De Andrade and D’Agosto, 2016). These schemes eventually promote a long-term shift from self-vehicle to public transportation services. The United Kingdom government has been concerned with the global warming issue and provided new strategies to reduce CO2 emission in all sectors, such as launching new public transportation (Kaewunruen et al., 2018; Logan et al., 2020). Additionally, the United Kingdom’s railway network is considered the lowest CO2 emission per passenger over other public services. Regarding the global climate policies, the United Kingdom government has still intended to cut off the railway’s emission by replacing it with alternative fuels and changing the current diesel engine system toward the decarbonization concept, mainly reducing the emission from the operational process.
Even though the CO2 emission from the railway’s life cycle predominantly comes from the railway infrastructure, the United Kingdom government and rail sectors exceptionally focus on reducing those emissions from the operational stage. In this research, the authors believe that only promoting strategies to reduce CO2 in the operational process cannot bring the United Kingdom government to reach its targets by 2050. In contrast, the government should also consider other effective and practical strategies.
In order to understand the amount of CO2 emission from the railway network, the research deeply examines the entire life cycle analysis (LCA) through the high-speed rail (HSR)’s infrastructure. This research aims to provide future strategies and policies to cut the CO2 off the railway network. Furthermore, the decarbonization concepts and practical approaches to the railway system have been stated in this study.
The Decarbonization Concept in the Railway System
The Paris Agreement has been launched as a global environmental policy. It targets to keep global temperature below 1.5°C compared with the pre-industrial era (Dimitrov, 2016; Streck et al., 2016). The agreement has been involved in the transportation industry, especially on railway and HSR networks. Implementing environmental concepts becomes a challenging issue for researchers and engineers in the railway industry. The International Union of Railways (UIC)’s report states that transportation shared 24.7% of CO2 emission or 8 billion tCO2. The railway sector, well-known as the lowest CO2 emitter, has produced 26.64 million tCO2 across the European countries (Korea Ministry of Land, 2008; Kaewunruen et al., 2016; UIC, 2017). Also, many attempts to reduce global emission have been applied to the railway industry. There were several collaborations among railway industries, operators, policymakers, and other related sections to respond to governmental policies.
Based on the United Kingdom’s targets to achieve net-zero by 2050 and reduce 80% of greenhouse gases (GHGs) emissions relative to 1990 levels (GOVUK, 2019; ORR, 2019). The principal of the net-zero refers to the balance between the emitted GHGs and their amount in the atmosphere. The discharged and taken out GHGs can be equivalent by using high technologies such as carbon capture and carbon storage (Bonsu, 2020). In fact, the GHGs are mainly composed of CO2; hence, the amount of emission is commonly measured in the CO2 unit. Moreover, the government launches the net-zero plan to produce low carbon industries across the United Kingdom. Nevertheless, the strategies are unable to bring the United Kingdom to reach its targets due to a lack of reducing CO2 emissions in other sections such as infrastructural emission.
Department for Transport (DfT)’s report (2020) reveals that the decarbonization plan decreases 43% of emissions while increasing the country’s economy by 75%. It illustrates significantly advanced progress over other sectors. The Rail Safety and Standards Board (RSSB) states that the United Kingdom’s rail system has a high potential to provide net-zero carbon by 2050 (ORR, 2019; LSE, 2020). The statement confirms the United Kingdom’s rail network in response to European’s policy toward a decarbonizing framework. Following the global guidelines, the United Kingdom’s government has proposed a vision to remove all diesel trains from the network by 2040. According to the United Kingdom railway network, it has been widely linked across the country in a total of 16,209 km of distance (Stittle, 2004; Network Rail, 2020). The railway takes 2% market share of public transportation and 10% of passenger mile traveled in Great Britain. The transport sector’s emission shows at 28%, which the rail sector shared 3% of total transport’s emission (Power et al., 2016; ORR, 2019; RSSB, 2019). The rail network is expected to use electrical and other renewable sources as alternative energy. Moreover, an effective plan to reduce CO2 from the network is adopted in all related rail activities, that is, using zero-carbon self-powered vehicles, increasing energy efficiency, and reducing pollution emissions.
The decarbonization concept refers to the termination of CO2 emission from fossil fuel (RSSB, 2019). The government plans to operate an entire network without CO2 emission by 2050. All diesel trains will not be allowed in the United Kingdom’s rail network, and the existing engines need to be replaced with an electric system. Moreover, the government is encountering unsupported infrastructural issue because only 42% of the United Kingdom’s network is an electrical track (RSSB, 2019). Alternative energies have been applied to the railway network, such as hydrogen (H2) fuel cell, battery, solar energy, and biomass. Some research compares an engine performance between the existing diesel trains and electric trains. The outcomes state that the fuel cell and the electrification systems do not significantly improve the train’s performance. In contrast, the H2 train has higher efficiency than other low-carbon vehicles, but it is the most expensive option (Anandarajah et al., 2013). The electric hybrid vehicle is highly recommended as it is able to reduce the fuel consumption by more than 20%. As a result, the GHG emission can be reduced up to 15–30% (Cheli et al., 2006).
In this day, the United Kingdom’s HSR network, namely, “HS2,” has been continually constructed. The HS2 project strictly follows the government’s net-zero targets and decarbonization concept since the beginning of the construction phase (HS2, 2021). The HSR service has limited the lower speed at 250 km/h, while the conventional rail’s speed at 200 km/h. The HS2 project is expected to be the most sustainable railway network in the world, reducing CO2 emission than cars and planes. Regarding the future developments toward the rail network, the decarbonization vision should be turned into practice. The government must consider the cost of electrical track and power engines replacement.
The Life Cycle Analysis of High-Speed Rail’s Infrastructure
The LCA, a tool to identify environmental impacts along the life cycle, is applied to this research. This assessment clearly shows the CO2 emission in each life cycle stage that leads to sustainable development. To overcome this problem, some approaches have also been made with the decarbonization of the railway. The outcomes have precisely estimated the CO2 emission from each LCA stage; moreover, the insight provides practical recommendations to reduce the railway system’s emission.
The LCA of HSR’s infrastructure consists of manufacturing, operation, maintenance, and demolition (Kaewunruen et al., 2019). The manufacturing stage comprises rail track construction that the CO2 is emitted during its construction and logistic processes. The three characteristics of the rail infrastructure include ballasted track, bridge, and tunnel. The material requirements on each characteristic are dissimilar, caused by the unequal amount of their emissions, as shown in Table 1.
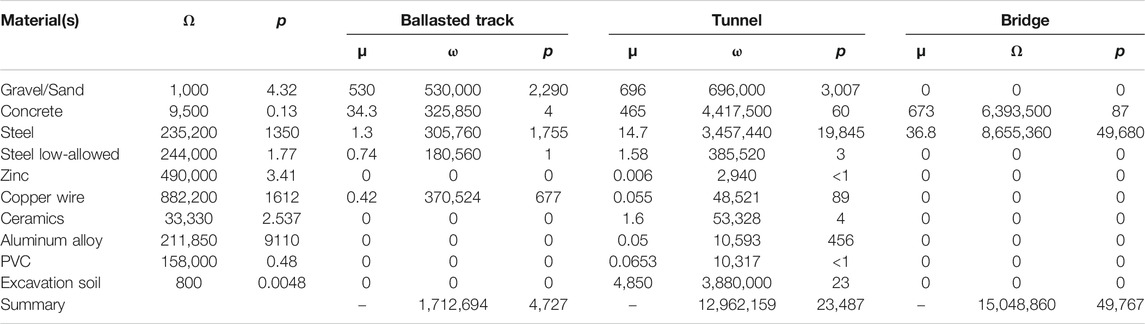
TABLE 1. The summary of material used (µ: kg), embodied energy (ω: kJ/kg), and emission (p;kgCO2/km) in 1°km of ballasted track, tunnel, and bridge (adopted from the Department for Business, Energy and Industry Strategies, 2020; Global source, 2020).
The research compares ballasted track, tunnel, and bridge in terms of embodied energy requirement and CO2 emission. The results clearly show that the ballasted track, which consumes 1,712,694 kJ and emits 4,727 kg CO2, is the lowest emitter. The CO2 emission from the ballasted track is lower than that in rail’s tunnel and bridge five and ten times, respectively.
The maintenance and operational stages are necessary to maintain the safety conditions of rail infrastructure. The operational and maintenance stages are typically the longest phase in the lifecycle. Moreover, these stages include the standard operation plan, emergency operation, and related activities (Kaewunruen et al., 2019; Li and Zhang, 2020). This research examines LCA with an assumption of significant operation and maintenance that are allocated every 5 years. And, the material requirements are estimated at 15% on the amount of initial construction. The timeframe of infrastructure’s LCA is placed at 70 years. As a result, the total emission of these stages is 9,927.56 kgCO2/km.
Last, the demolition stage is assigned at the final step and aimed at recycling non-using parts. All of the nonrecyclable materials with the toxic chemical are cleaned up. Then, they are transferred to the shredding process and landfill. On the other hand, recyclable parts such as steel, concrete are sent to cleaning, crushing and shredding processes. The small pieces of materials are shifted to the melting process for reforming to the new products. The rail infrastructure composed the recyclable and nonrecyclable parts. In this study, the ballasted track, which is the majority, is analyzed toward the demolition stage (Network Rail, 2020; Rungskunroch et al., 2021). As a result, the calculation shows that the final stage emits 0.248 kgCO2/km.
In conclusion, the LCA’s result reveals 14,655.22 kgCO2/km. The fractions of emission in manufacturing, operation and maintenance, and demolition are shared at 32.25, 6, and 0.001%, respectively. It can conclude that a significant amount of CO2 emission comes from operation and maintenance.
Discussions and Recommendations
Following the national energy consumption statistics, the total GHG emission is 351.1 million tonnes (Mt), containing 280.88 MtCO2 (Department for Business, Energy and Industrial Strategy, 2020). The transportation sector denotes 28% of total emission across the United Kingdom, whereas the railway network shares only 2% of CO2 emission of that sector (RSSB, 2019). It can be estimated that CO2 emission from the United Kingdom rail network is 15.72 MtCO2 or 970.40 tCO2/km per annual. The Office of Rail and Road (ORR) reports that the United Kingdom’s CO2 emission for passenger train shows at 36.6 gCO2 per passenger kilometer (pkm), whereas 25.3 gCO2 per tonne-kilometer (tkm) from freight train emission (ORR, 2019).
Since eliminating diesel train is not enough response to the government’s targets, the implications of these findings are discussed as the solutions to tackle the CO2 emission from the United Kingdom railway network as follows:
Railway Infrastructure Construction and Maintenance
Various policies have been announced without concerns for the improvement of railway structure. The LCA’s infrastructure results show that 14,655.22 kgCO2/km is emitted in the 70 years of timeframe. It can also be increased to 72,811.52 and 154,284.07 kgCO2/km on constructing a rail’s tunnel and bridge, respectively. Most of the CO2 emission occurs at the operational and maintenance stages. Moreover, the volume of emissions can be increased when the track requires remarkable maintenance, that is, severe accident, natural disaster, and vandalism. Hence, the emissions by infrastructural activities should not be neglected. Future research should consider the negative effects of CO2 during infrastructural construction and maintenance carefully (Kaewunruen et al., 2014; Kaewunruen et al., 2015, Kaewunruen et al., 2016).
Replacement of Diesel Engine
RSSB reports that 80% of the traveling distance uses electric trains, and the rest diesel trains. The United Kingdom government has faced political issues of buying the new freight train because none of the current models meets the United Kingdom’s net zero-emission targets (Stittle, 2004; Kaewunruen and Lee, 2017). Therefore, the existing freight trains, which are high-level of CO2 emission, must be continually serviced. This research highly recommends replacing diesel engines with an H2 fuel cell or biofuel. These alternative sources offer lower CO2 emission and reduce energy consumption than the diesel model.
Installation of Electric Track Along With the United Kingdom Rail Network
42% of the entire network is an electric track. It means that only those numbers of tracks can push the government’s targets forward. Some researchers offer critical development on the environmental perspectives by installing electric tracks (Marin et al., 2010; Kirkwood et al., 2016; Krezo et al., 2018; Chovančíková and Dvořák, 2019). This study also recommends replacing the standard track with the electric track for long-term sustainability goals. Future research on electric track might provide cost details and efficiency about direct current (DC) and alternate current (AC) networks. As found, the DC network shows tangible benefits in terms of power control and noise nuisance (Alnuman, Gladwin and Foster, 2018), while the AC network offers low heat loss.
Conclusion
Regarding the Paris Agreement, the United Kingdom government responds to that by planning to make zero-emission across the United Kingdom by 2050. Leading strategies push forward governmental regulation by removing diesel trains out of the network by 2040. Nevertheless, the emission of CO2 in the railway network occurs from various sections. Therefore, the LCA is applied to rail infrastructure to precisely describe the CO2 emission on each lifecycle stage. As shown, the CO2 predominantly emits from operation and maintenance stages. In this study, both LCA stages have emitted approximately 60% of the total emission in a whole life cycle. It brings the total emission of infrastructure at least 14,655.22 kg CO2/km.
Conversely, the CO2 emission in the railway operational stage is 36.6°g CO2/pkm annually, which exceedingly depends on the volume of a passenger. The research’s broad implication is that the railway system’s reduction of CO2 emission should be widely considered along with the rail network, especially on the railway infrastructure. Our study provides three realistic key developments covering infrastructure, electric rail track, and vehicle’s engine power. By following these guidelines, the United Kingdom government’s target to be net-zero carbon is more achievable.
Author Contributions
PR carried out data analytics and visualization. PR and SK developed the concept and methodology for the investigation. All authors contributed in the writing of the opinion paper.
Conflict of Interest
The authors declare that the research was conducted in the absence of any commercial or financial relationships that could be construed as a potential conflict of interest.
Acknowledgments
The first author gratefully acknowledges the Royal Thai Government for the PhD scholarship at the University of Birmingham, United Kingdom, and the RISEN funding for one year at the University of California, Berkeley. The second author acknowledges the Australian Academy of Science (AAS) and the Japan Society for the Promotion of Sciences (JSPS) for the JSPS Invitation Fellowship for Research (long-term), Grant No. JSPS-L15701, at the Railway Technical Research Institute (RTRI) and the University of Tokyo, Japan. The authors are sincerely grateful to the European Commission for the financial sponsorship of the H2020-RISEN Project No. 691135 “RISEN: Rail Infrastructure Systems Engineering Network,” which enables a global research network that tackles the grand challenge of railway infrastructure resilience and advanced sensing in extreme environments (www.risen2rail.eu).
References
Alnuman, H., Gladwin, D., and Foster, M. (2018). Electrical Modelling of a DC Railway System with Multiple Trains. Energies 11, 3211. doi:10.3390/en11113211
Anandarajah, G., McDowall, W., and Ekins, P. (2013). Decarbonising Road Transport with Hydrogen and Electricity: Long Term Global Technology Learning Scenarios. Int. J. Hydrogen Energ. 38, 3419–3432. doi:10.1016/j.ijhydene.2012.12.110
Andrade, C., and D’Agosto, M. (2016). The role of rail transit systems in reducing energy and carbon dioxide emissions: The case of the city of Rio de Janeiro. Sustainability 8 (2), 150. doi:10.3390/su8020150
Bonsu, N. O. (2020). Towards a Circular and Low-Carbon Economy: Insights from the Transitioning to Electric Vehicles and Net Zero Economy. J. Clean. Prod. 256, 120659. doi:10.1016/j.jclepro.2020.120659
Cheli, R., et al. (2006). “Rail-car Hybrid Trains to Reduce Fuel Consumption and Emissions,” in 7th World Congress on Railway Research, 2006 June 4–6. Montreal, Canada.
Chovančíková, N., and Dvořák, Z. (2019). Effect of a Power Failure on Rail Transport. Transp. Res. Procedia 40, 1289–1296. doi:10.1016/j.trpro.2019.07.179
Department for Business, Energy and Industrial Strategy (2020). 2019 UK Greenhouse Gas Emission, Provisional Figure. Available at: https://assets.publishing.service.gov.uk/government/uploads/system/uploads/attachment_data/file/932122/decarbonising-transport-setting-the-challenge.pdf (Accessed on December 03, 2020).
Dimitrov, R. S. (2016). The Paris Agreement on Climate Change: Behind Closed Doors. Glob. Environ. Polit. 16, 1–11. doi:10.1162/GLEP_a_00361
Global source (2020). Products. Available at: https://www.globalsources.com/manufacturers/Concrete-Mixer.html (Accessed on: April 28, 2020).
GOV.UK (2019). UK Becomes First Major Economy to Pass Net Zero Emission Law. Available at: https://www.gov.uk/government/news/uk-becomes-first-major-economy-to-pass-net-zero-emissions-law (Accessed on: February 1, 2021).
HS2 (2021). Sustainability. Available at: https://www.hs2.org.uk/building-hs2/hs2-and-the-natural-environment/environment-sustainability/ (Accessed on: March 16, 2021).
Kaewunruen, S., Remennikov, A. M., and Murray, M. H. (2014). Introducing a new limit states design concept to railway concrete sleepers: an Australian experience. Front. Mater. 1, 8. doi:10.3389/fmats.2014.00008
Kaewunruen, S., Sussman, J. M., and Einstein, H. H. (2015). Strategic framework to achieve carbon-efficient construction and maintenance of railway infrastructure systems. Front. Environ. Sci. 3, 6. doi:10.3389/fenvs.2015.00006
Kaewunruen, S., Sussman, J. M., and Matsumoto, A. (2016). Grand Challenges in Transportation and Transit Systems. Front. Built Environ. 2, 4. doi:10.3389/fbuil.2016.00004
Kaewunruen, S., and Lee, C. K. (2017). Sustainability Challenges in Managing End-of-Life Rolling Stocks. Front. Built Environ. 3, 10. doi:10.3389/fbuil.2017.00010
Kaewunruen, S., Rungskunroch, P., and Jennings, D. V. (2019). A Through-Life Evaluation of End-Of-Life Rolling Stocks Considering Asset Recycling, Energy Recovering, and Financial Benefit. J. Clean. Prod. 212, 1008–1024. doi:10.1016/j.jclepro.2018.11.271
Kaewunruen, S., Rungskunroch, P., and Welsh, J. (2018). A Digital-Twin Evaluation of Net Zero Energy Building for Existing Buildings. Sustainability 11 (1), 159. doi:10.3390/su11010159
Kirkwood, L., Giuntini, L., Shehab, E., and Baguley, P. (2016). Development of a Whole Life Cycle Cost Model for Electrification Options on the UK Rail System. Proced. CIRP 47, 1–5. doi:10.1016/j.procir.2016.03.067
Korea Ministry of Land (2008). Infrastructure and Transport, Korea Agency for Infrastructure Technology Advancement. Building Mater. Environ. Inf. DB Final Rep. Available at: http://contents.archives.go.kr/next/search/showDetailPopup.do?rc_code=1310377&rc_r?le_no= 201103732500&rc_ritem_no= (Accessed on December 02, 2020).
Krishnan, V., Kastrouni, E., Pyrialakou, V. D., Gkritza, K., and McCalley, J. D. (2015). An Optimization Model of Energy and Transportation Systems: Assessing the High-Speed Rail Impacts in the United States. Transportation Res. C: Emerging Tech. 54, 131–156. doi:10.1016/j.trc.2015.03.007
Krezo, S., Mirza, O., Kaewunruen, S., and Sussman, J. M. (2018). Evaluation of CO2 Emissions from Railway Resurfacing Maintenance Activities, Transportation Research Part D: Transport and Environment 65, 458–465. doi:10.1016/j.trd.2018.09.019
Li, L., and Zhang, X. (2020). Integrated Optimization of Railway Freight Operation Planning and Pricing Based on Carbon Emission Reduction Policies. J. Clean. Prod. 263, 121316. doi:10.1016/j.jclepro.2020.121316
Logan, K. G., Nelson, J. D., McLellan, B. C., and Hastings, A. (2020). Electric and Hydrogen Rail: Potential Contribution to Net Zero in the UK. Transp. Res. D: Transp. Environ. 87, 102523. doi:10.1016/j.trd.2020.102523
LSE (2020). What Is “Decarbonisation” of the Power Sector? Why Do We Need to Decarbonise the Power Sector in the UK? Available at: https://www.lse.ac.uk/granthaminstitute/explainers/what-is-decarbonisation-of-the-power-sector-why-do-we-need-to-decarbonise-the-power-sector-in-the-uk/ (Accessed on November 30, 2020).
Marin, G. D., Naterer, G. F., and Gabriel, K. (2010). Rail Transportation by Hydrogen vs. Electrification - Case Study for Ontario, Canada, II: Energy Supply and Distribution. Int. J. Hydrogen Energ. 35, 6097–6107. doi:10.1016/j.ijhydene.2010.03.095
Network Rail (2020). Track. Available at: https://www.networkrail.co.uk/running-the-railway/looking-after-the-railway/track/ (Accessed on December 03, 2020).
ORR (2019). Rail Emissions 2018-19 Annual Statistical Release. Available at: https://dataportal.orr.gov.uk/media/1550/rail-emissions-2018-19.pdf (Accessed on December 03, 2020).
Power, C., Mian, J., Spink, T., Abbott, S., and Edwards, M. (2016). Development of an Evidence-Based Geotechnical Asset Management Policy for Network Rail, Great Britain. Procedia Eng. 143, 726–733. doi:10.1016/j.proeng.2016.06.112
Pye, S., Li, F. G. N., Price, J., and Fais, B. (2017). Achieving Net-Zero Emissions through the Reframing of UK National Targets in the Post-Paris Agreement Era. Nat. Energ. 2, 17024. doi:10.1038/nenergy.2017.24
RSSB (2019). Decarbonisation – Our Final Report to the Rail Minister. Available at: https://www.rssb.co.uk/Research-and-Technology/Sustainability/Decarbonisation/Decarbonisation-our-final-report-to-the-Rail-Minister (Accessed on December 02, 2020).
Rungskunroch, P., Jack, A., and Kaewunruen, S. (2021). Benchmarking on Railway Safety Performance Using Bayesian Inference, Decision tree and Petri-net Techniques Based on Long-term Accidental Data Sets, Reliability Engineering & System Safety 213, 107684. doi:10.1016/j.ress.2021.107684
Stittle, J. (2004). Accounting for UK Rail Freight Track Charges: Privatisation, Politics and the Pursuit of Private Sector Vested Interests. Account. Forum 28, 403–425. doi:10.1016/j.accfor.2004.05.002
Keywords: decarbonisation, life cycle assessment, rail infrastructure, CO2 emission, railway system
Citation: Rungskunroch P, Shen Z-J and Kaewunruen S (2021) Getting It Right on the Policy Prioritization for Rail Decarbonization: Evidence From Whole-Life CO2e Emissions of Railway Systems. Front. Built Environ. 7:638507. doi: 10.3389/fbuil.2021.638507
Received: 06 December 2020; Accepted: 09 April 2021;
Published: 29 April 2021.
Edited by:
Eduardo Cabrita Fortunato, National Laboratory for Civil Engineering, PortugalReviewed by:
Serdar Dindar, Izmir Kâtip Çelebi University, TurkeyCheul Kyu Lee, Korea Railroad Research Institute, South Korea
John Riley Edwards, University of Illinois at Urbana-Champaign, United States
Copyright © 2021 Rungskunroch, Shen and Kaewunruen. This is an open-access article distributed under the terms of the Creative Commons Attribution License (CC BY). The use, distribution or reproduction in other forums is permitted, provided the original author(s) and the copyright owner(s) are credited and that the original publication in this journal is cited, in accordance with accepted academic practice. No use, distribution or reproduction is permitted which does not comply with these terms.
*Correspondence: Panrawee Rungskunroch, ci5wYW5yYXdlZUBiZXJrZWxleS5lZHU=; Zuo-Jun Shen, c2hlbkBpZW9yLmJlcmtlbGV5LmVkdQ==; Sakdirat Kaewunruen, cy5rYWV3dW5ydWVuQGJoYW0uYWMudWs=