- Division of Sustainable Development, College of Science and Engineering, Hamad Bin Khalifa University, Doha, Qatar
The implementation of sustainable solutions for maintaining indoor air quality has become a particular concern to the building community. Research on green technologies for indoor air has highlighted the potential of active botanical biofiltration (ABB) systems, where the air is circulated through the plant root zone as well as the growing medium for maximum phytoremediation effect. ABB has been found beneficial for pollutant removal along with the potential for increasing humidity and air cooling. Assessment in laboratory condition revealed the removal efficiency of ABB systems ranged from 54 to 85% for total suspended particulate matters where gaseous pollutants such as formaldehyde and toluene removal efficiencies were 90% and over 33%, respectively, in real environment. Moreover, the esthetic value of ABB acts as an added benefit for positive mental effects. However, very limited data is available to date that demonstrates the pollutant removal efficiency of ABB systems in realistic indoor environments, and the mechanisms behind this emerging technology are still poorly understood. The purpose of this mini review study is to present a quantitative assessment of the recent advancement of ABB systems and indoor air quality. Finally, the limitations of ABB systems and research gaps are highlighted for future improvement.
Introduction
According to the Environmental Protection Agency, indoor air pollution is considered one of the top five environmental health risks. Moreover, 2.7% of the global burden of disease has been linked to indoor air pollution in a report published by the World Health Organization (WHO) (WHO Global Health Risks, 2009; Mannan and Al-Ghamdi, 2021). Commonly listed health impacts of indoor air pollutants include asthma, headache, nausea, fatigue, eye irritation, reduced lung function, cough, and lung cancer (Park et al., 2001; Swanson, 2001; USEPA, 2003; Dorothy Shimer and Phillips, 2005; Fisk et al., 2007). To minimize indoor air pollutants concentration below the threshold levels in different indoor environments, several methods have been adopted so far, such as source reduction, dilution, and the use of air cleaning devices. Portable air cleaning devices, also known as air purifiers or sanitizers, and HVAC and other duct-mounted air cleaning devices are two types of general air cleaning devices available that commonly use fibrous air filters, electrostatic precipitators, and ionizers to remove particles from indoor air (USEPA, 2018).
Along with the advancement of mechanical devices for indoor air pollution control, in the long run, building professionals are showing great interest in indoor plant-based air purification systems for several potential mechanisms of leaf surface, stomata, and plant roots. These include adsorption and absorption capacity of gaseous air pollutants and particulate material (PM); degradation capacity of gaseous air pollutants; CO2 removal and O2 supply; increase in humidity; and reduction in bioaerosols (Newman and Reynolds, 2004; Orwell et al., 2004; Kohlrausch et al., 2006; Liu et al., 2007; Llewellyn and Dixon, 2011). However, the limitations of the potted plant and green wall approaches initiated the research for more advanced green wall–based air purification systems, which allow the intimate contact of polluted air with the microorganisms in the rhizosphere zone of the plants to maximize the phytoremediation process by creating an airflow through mechanical devices. This approach was defined as active botanical biofiltration (ABB) by Pettit et al. (2018a) where Liewellyn and Dixon (2011) stated the system as the botanical indoor air biofilter (BIAB) (Llewellyn and Dixon, 2011; Pettit et al., 2018a). Moreover, researchers used several other nomenclatures to refer this same system such as an active living wall (ALW) or active green wall (AGW) system when they applied this technology to the existing living wall or green wall systems (here living wall and green wall both indicate the similar vertical greening system) (Irga et al., 2017a; Pettit et al., 2019a). So far, research has been carried out dealing with different aspects of ABB to reduce overall air pollutants, such as the impact of plant quantity and type; temperature and airflow; media substrates; plant nutrition and irrigation; and lighting. However, very limited data is available to date that demonstrates the pollutant removal efficiency of ABB or ALW systems in realistic indoor environments, and the mechanisms behind this emerging technology are still poorly understood for specific areas. Figure 1 represents the schematic of BIAB and ALW systems.
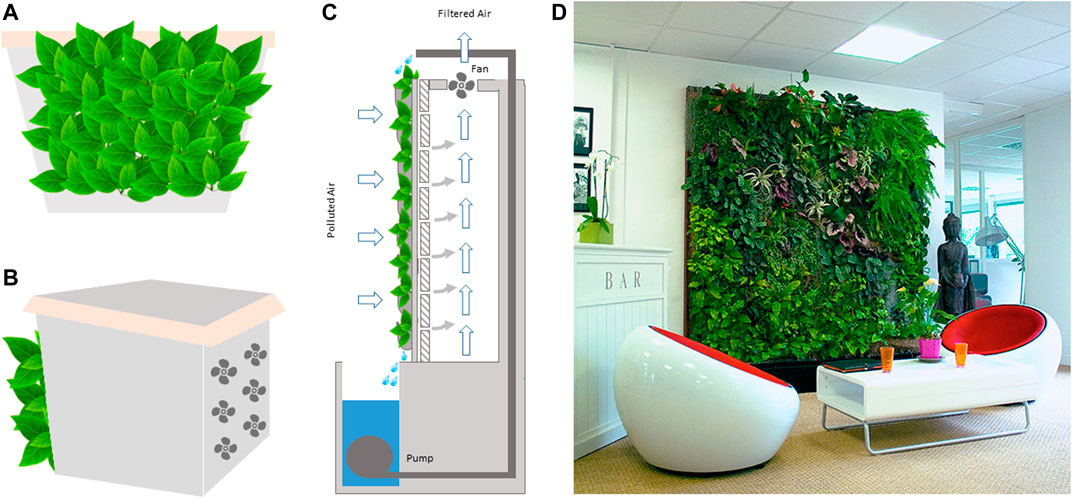
FIGURE 1. Schematic of BIAB and ALW systems. Section (A, B) represents the front and side view of BIAB system where section (C) presents the cross-sectional view of ALW system (D) represents how an indoor living wall system would look like. (Ibrahim et al., 2018; Mannan and Al-Ghamdi, 2020).
The purpose of this short review study is to present a quantitative assessment of the recent advancement of ABB systems, especially ALW systems, for improved indoor air quality (IAQ). To achieve this, the authors have conducted a literature search for peer-reviewed articles with a special focus on the application of ABB or ALW systems for indoor spaces for a period of the last 10 years. The literature search included mainly peer-reviewed journals along with conference papers and other scientific reports. The databases that have been searched mostly for relevant publications are Science Direct, Springer Link, and Wiley Online Library. The article’s selection was based on several criteria, including the construction of the systems, the efficiency of pollutant removal, system assessment condition, and impact of the ABB or ALW systems on other indoor environmental quality. Finally, the limitations of these systems and research gaps are highlighted for future improvement. The selected articles have been classified and presented in this review in two categories, mainly the air filtration systems in laboratory conditions and realistic indoor environments. Based on these two classifications, the application of ABB or ALW systems have been carefully evaluated and described in the following section. Hence, this mini review aims to:
- Review the recent advancement of ABB systems to identify the indoor pollutant removal efficiency in quantitative manner
- Review the construction strategy of the ABB or ALW systems along with the condition of the experiment environment (e.g., size of the room) to benefit the system’s future development
- Highlight the research gaps and future outlook
Active Botanical Biofiltration for Better Indoor Air
This section reviews the application of the ABB system for indoor spaces from different perspectives to present the most recent developments, challenges, and opportunities of ABB for better IAQ. Table 1 summarized the reviewed studies based on the construction and operation condition and pollutant removal capacity. Here, all the terms related to ABB systems (e.g., the terms ABB/ALW/AGW) have been used based on how they have defined in each specific research studies, although having the same mechanism for air filtration.
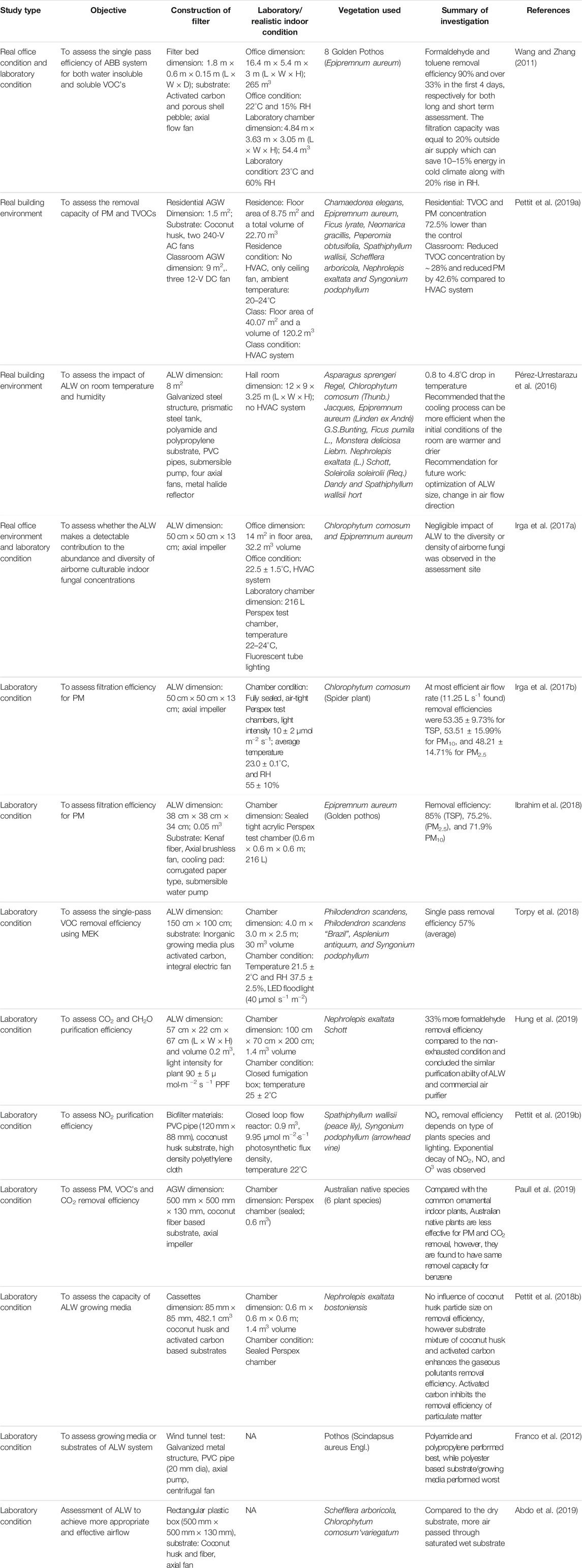
TABLE 1. Summary of ALW systems. Analysis of the ALW/ABB studies performed in real and laboratory environment based on construction and operation conditions and pollutant removal capacity.
Active Botanical Biofiltration in Realistic Conditions
The investigation of an ABB prototype has been performed for both soluble and insoluble volatile organic compounds (VOCs) and was developed based on three basic principles: the degradation of VOCs through plant root microorganisms, pollutant adsorption through activated carbon, and pollutant absorption by water (Wang and Zhang, 2011). This prototype was assessed in a typical office environment as well as in a full-scale stainless-steel test chamber. 5–32 vol% was found as the optimum water content range in the root bed for maximum VOC removal. Experimentation in real office conditions for 300 days indicated satisfactory single-pass removal efficiency for formaldehyde and toluene (90% and over 33%, respectively, for the first four days). Moreover, this ABB system successfully reduced the indoor air temperature by 0.5°C in the realistic environment, while the reduction of temperature was 1°C in laboratory conditions. The relative humidity (RH) increase for realistic and laboratory conditions was 17.7 and 9–13%, respectively. Furthermore, a reduction of 20% of the supply of outdoor air can be achieved using the ABB system and thus can save energy required by the building given that the concentrations of toluene and formaldehyde dictated the standard ventilation rate for this case. However, different climate zones should be investigated (including hot and cold) to justify the applicability of indoor ABB systems along with the energy-saving potential. Two pilot-scale AGWs were tested in both residential housing and a classroom to assess the removal capacity of both total volatile organic compounds (TVOCs) and PM (Pettit et al., 2019a). Analysis of the investigated data confirmed the reduction of TVOC and PM concentrations to a level that the classroom’s current HVAC system could not achieve in normal conditions. In the residential space, a 72.5% lower TVOC concentration was found compared to the control system when the AGW system was applied; the same occurred for residential PM. Although significant improvement was observed here, this study highlights the need for long-term experimentation and empirical validation assuring AGW systems’ efficiency in improving IAQ.
Apart from pollutant removal capacity, a prototypic ALW was tested in realistic conditions to observe the impact of ALW on indoor temperature and humidity (Pérez-Urrestarazu et al., 2016). Acting as natural evaporative coolers, ALW systems can reduce indoor temperature as well as increase humidity, thus lowering the cooling energy requirements in buildings (Rodgers et al., 2013). Analysis of the sensor results revealed temperature drops ranging from 0.8 to 4.8°C for different positions around the ALW. The differences in the impact of the ALW on different positions in the assessment hall indicate the requirement of further investigation into topics such as ALW size optimization and airflow direction. Moreover, the effects on indoor temperature and humidity should also be investigated during normal weekdays when the assessment location experiences normal activities. The contribution of an ALW to indoor fungal spread was assessed in both a realistic environment and laboratory conditions in Sydney, Australia (Irga et al., 2017a). Analysis of the testing data concluded the ALW system had a negligible impact on airborne fungal concentration; however, this study emphasizes the importance of long-term assessment to realize the potential of fungal growth in indoor spaces.
Active Botanical Biofiltration in Laboratory Conditions
The efficiency of ABB in the removal of PM was assessed exclusively in a static chamber where general observation recorded that PM removal efficiency increased with an increase in airflow rate (Irga et al., 2017b). Experiments were conducted at five different airflow rates to assess the single-pass efficiency of the ABB system for the filtration of total suspended particles (TSPs), PM2.5, and PM10. However, airflow rate over 11.25 L s-1 reduced the filtration efficiency during the experiment with the botanical system, whereas systems without the botanical part did not show the same characteristics. Approximately 53, 54, and 48% removal efficiencies were achieved for TSPs, PM10, and PM2.5, respectively. Based on the collected data and further analysis, this study estimates that for four occupants in an office environment, 1 m2 of an ABB system could sufficiently supply the required ventilation. Further assessment of this system in situ is marked as the next step so that in the near future, the ABB system could operate as a standalone system or an integral part of an HVAC unit to provide standard ventilation for indoor spaces. A similar investigation was conducted where the single-pass filtration efficiency of a BIAB system was assessed for PM levels (TSPs, PM2.5, and PM10) (Ibrahim et al., 2018). Compared to the available systems on the market, this BIAB system added an evaporative cooling pad to enhance the principle of PM removal efficiency through absorption. With this advancement in BIAB design, this system successfully removed 85% of TSPs, 71.9% of PM10, and 75.2% of PM2.5. Nonetheless, the long-term efficiency of BIAB systems, along with the simultaneous removal of CO2 and VOCs with PM, are still to be investigated extensively.
For methyl ethyl ketone, the filtration efficiency of an AGW was investigated in a 30 m3 chamber representative of a realistic room (Torpy et al., 2018). A combination of inorganic growing media and activated carbon was applied for the reduction of indoor VOCs, which showed a 57% VOC reduction on average for single-pass removal efficiency. Recommendations are made to further investigate plant type, growing medium, and moisture content to enhance VOC removal efficiency. CO2 and formaldehyde purification with the assistance of an ALW was assessed, and thereafter, test results were compared with the pollutant removal efficiency of two commercial air filters (Hung et al., 2019). The fumigation tank experiment indicated a similar air cleaning capacity for the ALW as for the selected two commercially available portable air cleaners; the ALW and the two commercial air cleaners are also economically comparable. A closed-loop flow reactor assessment was performed to check the ability of AGW systems to reduce NO2 in green buildings (Pettit et al., 2019b). Both O3 and NO were also monitored in this study for two specific vegetation systems and two lighting conditions. In ambient conditions, this system demonstrated exponential decay for all the targeted air pollutants; however, the study indicates a requirement for in situ long-term assessment. The use of native Australian plants and their efficiencies in an ALW system was assessed; the performance of native and ornamental plants was compared (Paull et al., 2019). Single-pass removal efficiency revealed the less effective nature of the native Australian plants for the removal of PM and CO2 when compared with the common ornamental plants. Further comparison indicated a similar ability to remove benzene in both types of plants.
Investigation about growing media (granulated activated carbon and coconut coir) in a functional green wall was conducted for a range of indoor air pollutants, especially for PM and VOCs, as the growing media or substrates not only act as a support system for vegetation but also promote primary pollutant removal mechanisms (Pettit et al., 2018b). Although coconut coir’s particle size was found to have no influence on pollutant removal efficiency, the increased removal efficiency was observed when activated carbon was mixed with coconut coir substrates, particularly for gaseous air pollutants. Oppositely, the growing media activated carbon was found to have a negative impact on PM removal efficiency. Equal concentrations of both coconut husk and activated carbon showed the best VOC removal conditions. This study strongly suggests selecting the growing media based on target indoor air pollutants along with assessing different vegetation conditions when using those substrates in future studies. Likewise, three different synthetic substrates (polyamide-polypropylene, polyester, and polyurethane) were assessed by open-circuit wind tunnel from four different perspectives, namely saturation efficiency, water volume retained, water consumption, and pressure drop (Franco et al., 2012). Considering all the given parameters, polyamide-polypropylene was found to be the best option, as it exhibited low water consumption tendency, average water retention capacity, and high saturation efficiency.
An airflow assessment for an ALW system was performed where both unplanted and planted modules were investigated in wet and dry conditions (Abdo et al., 2019). The preliminary observation indicated a substantially increased airflow rate through the saturated wet substrate compared with the dry substrate. This study recommends performing computational fluid dynamics analysis and large eddy simulation, which can verify the effectiveness of ALW system modification. The impact of four different vegetation systems on an ALW system was monitored, which highlighted the influence of plant species on specific VOCs’ removal processes in different percentages and recommended assessing the impact of mixed plants in future studies (Irga et al., 2019).
Conclusion and Future Directions
As the quality of buildings’ indoor air contributes to human health problems on a large scale, the implementation of sustainable solutions for maintaining IAQ has become a particular concern to the building community. ABB has been found beneficial for pollutant removal along with the potential for increasing humidity and air cooling. It has also been well studied that these systems do not promote fungal spread if kept in a well-monitored condition (Fleck et al., 2020). Moreover, the esthetic value of ABB acts as an added benefit for positive mental effects.
Although ABB systems have been assessed from different perspectives, investigations still need to be performed in real indoor environments to acquire conclusive results in the long run. Most studies thereby highlight the requirement for in situ experimentation for an extended time. Moreover, the optimization of the systems and advanced simulation analysis have been indicated as future work in this research field. As many studies have been performed that focus on specific pollutants, more investigations are required that focus on combining all the common indoor pollutants and observing how the advanced botanical system works in such conditions. Area- and weather-specific investigation can also benefit the development of ABB systems.
Although most of the studies performed in both realistic condition and laboratory condition have specified the ABB or ALW system dimensions (such as length, width) with the dimension of the indoor space or static chamber where the experiments have been performed, however, still now no clear equation or relationship has been drawn for optimum size of ABB or ALW system for any specific indoor space. Hence, simulation studies can be implemented as future studies to find such equations/relations. The temperature was set to 22–25°C in most cases for the experimental procedure; thereby, it is still unknown how the ABB or ALW systems will perform inside buildings where the temperature reaches far more than this specific temperature range during the pick summer season or opposite in the winter.
Along with the performance assessment of the ABB systems, it is also needed to evaluate the economic viability of these systems. Hung et al. (2019) has compared the ALW system with two commercially available air filters systems both from pollutant removal efficiency and economic perspective, however, most of the studies have excluded the economic factor. Moreover, with the progress of ABB systems, it is necessary to assess the overall sustainability of the entire system, including the construction, operation, and disposal phase. Though there are several studies that focused on the sustainability performance of LW systems (Feng and Hewage, 2014; Oquendo-Di Cosola et al., 2020), however, this is still rare for ALW systems.
It is very important to mention that direct comparison between systems for indoor air pollutant removal efficiency is difficult and not straightforward due to the specific conditions under which the tests were conducted as well as the nature of the pollutants themselves (Pettit et al., 2019c).
Author Contributions
Conceptualization, MM and SA-G; data curation, MM; formal analysis, MM; and SA-G; project administration, SA-G; supervision, SA-G; writing–original draft, MM; writing–review and editing, SA-G All authors have read and agreed to the final version of the manuscript.
Funding
This research was supported by a scholarship (210004673) from Hamad Bin Khalifa University (HBKU), a member of Qatar Foundation (QF). Any opinions, findings, and conclusions or recommendations expressed in this material are those of the author(s) and do not necessarily reflect the views of the HBKU or QF. Open Access funding provided by the Qatar National Library.
Conflict of Interest
The authors declare that the research was conducted in the absence of any commercial or financial relationships that could be construed as a potential conflict of interest.
References
Abdo, P., Huynh, B. P., Irga, P. J., and Torpy, F. R. (2019). Evaluation of Air Flow through an Active green wall Biofilter. Urban For. Urban Green. 41, 75–84. doi:10.1016/j.ufug.2019.03.013
Dorothy Shimer, P. L. J., and Phillips, T. J. (2005). Indoor Air Pollution in California. State of California: California Environmental Protection Agency, 363.
Feng, H., and Hewage, K. (2014). Lifecycle Assessment of Living walls: Air Purification and Energy Performance. J. Clean. Prod. 69, 91–99. doi:10.1016/j.jclepro.2014.01.041
Fisk, W. J., Lei-Gomez, Q., and Mendell, M. J. (2007). Meta-analyses of the Associations of Respiratory Health Effects with Dampness and Mold in Homes. Indoor Air 17, 284–296. doi:10.1111/j.1600-0668.2007.00475.x
Fleck, R., Gill, R. L., Pettit, T., Irga, P. J., Williams, N. L. R., Seymour, J. R., et al. (2020). Characterisation of Fungal and Bacterial Dynamics in an Active green wall Used for Indoor Air Pollutant Removal. Building Environ. 179, 106987. doi:10.1016/j.buildenv.2020.106987
Franco, A., Fernández-Cañero, R., Pérez-Urrestarazu, L., and Valera, D. L. (2012). Wind Tunnel Analysis of Artificial Substrates Used in Active Living walls for Indoor Environment Conditioning in Mediterranean Buildings. Building Environ. 51, 370–378. doi:10.1016/j.buildenv.2011.12.004
Hung, Y.-H., Chen, J.-K., Yeh, D.-M., and Tseng, T.-I. (2019). Active Living wall Modules - CO2 and CH2O Purification. IOP Conf. Ser. Mater. Sci. Eng. 609, 032022. doi:10.1088/1757-899X/609/3/032022
Ibrahim, I. Z., Chong, W.-T., and Yusoff, S. (2018). The Design of the Botanical Indoor Air Biofilter System for the Atmospheric Particle Removal. MATEC Web Conf. 192, 02035. doi:10.1051/matecconf/201819202035
Irga, P. J., Abdo, P., Zavattaro, M., and Torpy, F. R. (2017). An Assessment of the Potential Fungal Bioaerosol Production from an Active Living wall. Building Environ. 111, 140–146. doi:10.1016/j.buildenv.2016.11.004
Irga, P. J., Paull, N. J., Abdo, P., and Torpy, F. R. (2017). An Assessment of the Atmospheric Particle Removal Efficiency of an In-Room Botanical Biofilter System. Building Environ. 115, 281–290. doi:10.1016/j.buildenv.2017.01.035
Irga, P. J., Pettit, T., Irga, R. F., Paull, N. J., Douglas, A. N. J., and Torpy, F. R. (2019). Does Plant Species Selection in Functional Active green walls Influence VOC Phytoremediation Efficiency? Environ. Sci. Pollut. Res. 26, 12851–12858. doi:10.1007/s11356-019-04719-9
Kohlrausch, F., Prucker, D., Kölher, L., and Röber, R. (2006). Influence of Irradiance of Foliage Plants on Transpiration and Air Humidity. Acta Hortic. 711, 219–224. doi:10.17660/actahortic.2006.711.28
Liu, Y.-J., Mu, Y.-J., Zhu, Y.-G., Ding, H., and Crystal Arens, N. (2007). Which Ornamental Plant Species Effectively Remove Benzene from Indoor Air? Atmos. Environ. 41, 650–654. doi:10.1016/j.atmosenv.2006.08.001
Llewellyn, D., and Dixon, M. (2011). Can Plants Really Improve Indoor Air Quality? Compr. Biotechnol., 9, 331–338. doi:10.1016/B978-0-444-64046-8.00228-7
Mannan, M., and Al-Ghamdi, S. G. (2021). Indoor Air Quality in Buildings: A Comprehensive Review on the Factors Influencing Air Pollution in Residential and Commercial Structure. Ijerph 18, 3276. doi:10.3390/ijerph18063276
Mannan, M., and Al-Ghamdi, S. G. (2020). Life Cycle Embodied Energy Analysis of Indoor Active Living wall System. Energ. Rep. 6, 391–395. doi:10.1016/j.egyr.2020.11.180
Newman, L. A., and Reynolds, C. M. (2004). Phytodegradation of Organic Compounds. Curr. Opin. Biotechnol. 15, 225–230. doi:10.1016/j.copbio.2004.04.006
Oquendo-Di Cosola, V., Olivieri, F., Ruiz-García, L., and Bacenetti, J. (2020). An Environmental Life Cycle Assessment of Living Wall Systems. J. Environ. Manage. 254, 109743. doi:10.1016/j.jenvman.2019.109743
Orwell, R. L., Wood, R. L., Tarran, J., Torpy, F., and Burchett, M. D. (2004). Removal of Benzene by the Indoor Plant/Substrate Microcosm and Implications for Air Quality. Water Air Soil Pollut. 157, 193–207. doi:10.1023/B:WATE.0000038896.55713.5b
Park, J.-H., Gold, D. R., Spiegelman, D. L., Burge, H. A., and Milton, D. K. (2001). House Dust Endotoxin and Wheeze in the First Year of Life. Am. J. Respir. Crit. Care Med. 163, 322–328. doi:10.1164/ajrccm.163.2.2002088
Paull, N. J., Irga, P. J., and Torpy, F. R. (2019). Active Botanical Biofiltration of Air Pollutants Using Australian Native Plants. Air Qual. Atmos. Health 12, 1427–1439. doi:10.1007/s11869-019-00758-w
Pérez-Urrestarazu, L., Fernández-Cañero, R., Franco, A., and Egea, G. (2016). Influence of an Active Living wall on Indoor Temperature and Humidity Conditions. Ecol. Eng. 90, 120–124. doi:10.1016/j.ecoleng.2016.01.050
Pettit, T., Bettes, M., Chapman, A. R., Hoch, L. M., James, N. D., Irga, P. J., et al. (2019c). The Botanical Biofiltration of VOCs with Active Airflow: Is Removal Efficiency Related to Chemical Properties? Atmos. Environ. 214, 116839. doi:10.1016/j.atmosenv.2019.116839
Pettit, T., Irga, P. J., Surawski, N. C., and Torpy, F. R. (2019b). An Assessment of the Suitability of Active green walls for NO2 Reduction in green Buildings Using a Closed-Loop Flow Reactor. Atmosphere 10, 801. doi:10.3390/ATMOS10120801
Pettit, T., Irga, P. J., and Torpy, F. R. (2018b). Functional green wall Development for Increasing Air Pollutant Phytoremediation: Substrate Development with Coconut Coir and Activated Carbon. J. Hazard. Mater. 360, 594–603. doi:10.1016/j.jhazmat.2018.08.048
Pettit, T., Irga, P. J., and Torpy, F. R. (2019a). The In Situ Pilot-Scale Phytoremediation of Airborne VOCs and Particulate Matter with an Active green wall. Air Qual. Atmos. Health 12, 33–44. doi:10.1007/s11869-018-0628-7
Pettit, T., Irga, P. J., and Torpy, F. R. (2018a). Towards Practical Indoor Air Phytoremediation: A Review. Chemosphere 208, 960–974. doi:10.1016/j.chemosphere.2018.06.048
Rodgers, K., Handy, R., and Hutzel, W. (2013). Indoor Air Quality (IAQ) Improvements Using Biofiltration in a Highly Efficient Residential home. J. Green. Build. 8, 22–27. doi:10.3992/jgb.8.1.22
Swanson, M. C. (2001). Clearing the Air: Asthma and Indoor Air Exposures. Ann. Allergy Asthma Immunol. 87, 80. doi:10.1016/s1081-1206(10)62329-0
Torpy, F., Clements, N., Pollinger, M., Dengel, A., Mulvihill, I., He, C., et al. (2018). Testing the Single-Pass VOC Removal Efficiency of an Active green wall Using Methyl Ethyl Ketone (MEK). Air Qual. Atmos. Health 11, 163–170. doi:10.1007/s11869-017-0518-4
USEPA (2003). EPA Assessment of Risks from Radon in Homes. Washington, DC: United States Environmental Protection Agency (US EPA).
USEPA (2018). Residential Air Cleaners: A Technical Summary. Washington, DC: United States Environmental Protection Agency (US EPA), 74. Available at: www.epa.gov/iaq.
Wang, Z., and Zhang, J. S. (2011). Characterization and Performance Evaluation of a Full-Scale Activated Carbon-Based Dynamic Botanical Air Filtration System for Improving Indoor Air Quality. Building Environ. 46, 758–768. doi:10.1016/j.buildenv.2010.10.008
Keywords: active living wall, indoor air quality, air filters, building environment, biofiltration
Citation: Mannan M and Al-Ghamdi SG (2021) Active Botanical Biofiltration in Built Environment to Maintain Indoor Air Quality. Front. Built Environ. 7:672102. doi: 10.3389/fbuil.2021.672102
Received: 25 February 2021; Accepted: 10 June 2021;
Published: 30 June 2021.
Edited by:
Hasim Altan, Arkin University of Creative Arts and Design (ARUCAD), CyprusReviewed by:
Zhonghua Gou, Griffith University, AustraliaRui Pitarma, Instituto Politecnico da Guarda, Portugal
Copyright © 2021 Mannan and Al-Ghamdi. This is an open-access article distributed under the terms of the Creative Commons Attribution License (CC BY). The use, distribution or reproduction in other forums is permitted, provided the original author(s) and the copyright owner(s) are credited and that the original publication in this journal is cited, in accordance with accepted academic practice. No use, distribution or reproduction is permitted which does not comply with these terms.
*Correspondence: Sami G. Al-Ghamdi, c2FsZ2hhbWRpQGhia3UuZWR1LnFh