- 1Faculty of Technology, Research Center WANDER, Satakunta University of Applied Sciences, Pori, Finland
- 2Turku School of Economics, University of Turku, Pori, Finland
The COVID-19 pandemic has shown that actions related to infection prevention and control (IPC) need to be made more efficient, especially in indoor public spaces. Many standalone technologies and solutions are available to increase the hygiene levels of indoor environments. However, it is not clear how these technologies and solutions can be combined and adapted to building processes such that they cover the entire indoor environment and life cycle of a building—from its design to its use and maintenance. The construction industry faces challenges in this regard because many actors are involved, and interactions at multiple levels can hinder the implementation of innovations. Therefore, the aim of this article is to establish a framework for IPC within built environments by introducing a new indoor hygiene concept (IHC). It provides a tool for implementing necessary IPC actions during a building’s life cycle to construct or renovate hygienic indoor environments. The IHC is based on the idea that all the elements of an indoor environment need to be considered to create a hygienic building. In addition, hygiene objectives need to be set at an early stage of the construction process and monitored throughout all the phases of a building’s life cycle. This comprehensive approach enables designers, engineers, and other actors involved in different stages of a building’s life cycle to see their roles in the IPC of shared public spaces. Adopting this approach can result in fewer infection transmissions via indoor environments and, in turn, cost benefits for society.
Introduction
The COVID-19 pandemic has changed societies and peoples’ lives in numerous ways. It is evident that diseases transmitted from animals to humans will increase in the future (Kenyon, 2020). In addition, multidrug-resistant bacteria emerged presenting global health risks in the 2000s (Hernando-Amado et al., 2019). Resistant bacteria are not only threats in hospitals but have also been detected in public transportation, airports, and nurseries (Cassidy et al., 2020). The treatment of severe infections caused by viruses or resistant bacteria and fungi is expensive, and the outcome is often insufficient. For example, the treatment of COVID-19 remains challenging, and the development of effective drugs is still in progress (Boytz et al., 2022; Mehraeen et al., 2022; Polivka et al., 2022). Therefore, more focus on preventing the transmission of infections is required.
Since people in the developed world spend most of their time indoors, these environments contain major factors for decreasing infection transmission, especially public spaces that many people spend time in or pass through. Various technologies and solutions have been developed that individually increase hygiene and decrease infection transmission in built environments. For example, the antimicrobial effect of copper was exploited early in human history, although the concept of microbes had not yet been discovered. Copper is now utilized in many antimicrobial applications, including antimicrobial coatings (AMCs). Numerous studies on the effects of antimicrobial solutions have shown that they decrease the levels of microorganisms (Inkinen et al., 2017a; Colin et al., 2018). Further, a reduction in infections has been found in a few studies mainly concerning antimicrobial copper (Salgado et al., 2013; Lazary et al., 2014; Sifri et al., 2016; von Dessauer et al., 2016; Marcus et al., 2017; Zerbib et al., 2020). However, applying single antimicrobial technologies in indoor environments has limited potential for infection prevention and control (IPC) (Weber et al., 2017; Albarqouni et al., 2020). IPC means here all actions made to prevent transmission of infections, also in other than health care settings.
The economic effects of infections transmitted indoors can be extremely high. For example, costs related to COVID-19 have been estimated to be over $16 trillion in the US alone (Cutler and Summers, 2020). In terms of social and mental wellbeing and long-term complications, the true costs are difficult to quantify (Salari et al., 2020; Nalbandian et al., 2021). In addition, the overall economic costs of infections caused by microorganisms that do not pose the risk of epidemics can be high due to healthcare costs and sick leaves. The cost of controlling infections in societies may be less than bearing them. For example, it has been estimated that the investments in ventilation necessary to address airborne infections would cause less than a 1% increase in the construction costs of a typical building (Morawska et al., 2021).
Building’s life cycle, starting from needs assessment, planning, and design stages and continuing to commissioning and use, involves many actors, including architects, designers, engineers, and cleaning and maintenance personnel. Due to the multiple levels and many actors involved, creating a hygienic indoor environment to control the spread of infections is not easy. In addition, the project-based construction industry is strongly dependent on competitive tendering which makes the implementation of innovative solutions challenging (Dubois and Gadde 2002; Aouad et al., 2010; Bygballe and Ingemansson, 2014; Hemström et al., 2017).
To decrease the transmission of infections indoors, we suggest a new conceptual approach to designing, building, furnishing, and renovating public buildings and their indoor environments, which we call the indoor hygiene concept (IHC). Instead of performing individual hygiene-improving actions in an operational building, IHC consolidates all aspects of hygiene enhancement in built environments during the building’s whole life cycle for a comprehensive framework. This holistic approach can empower architects, designers, engineers, and other actors involved in different stages of a building’s life cycle to see their roles in the joint goal of preventing infections from spreading in the post-pandemic era.
Developing the concept
The IHC was developed in a long-term multidisciplinary cooperation that was started in 2012 by Satakunta University of Applied Sciences. Since then, we have contributed to many surveys, reports, and publications concerning indoor hygiene improvements (Mäkinen et al., 2013; Latva et al., 2016; Ahonen et al., 2017; Inkinen et al., 2017a; Inkinen et al., 2017b; Dunne et al., 2018; Inkinen et al., 2019; Rosenberg et al., 2019; Dunne et al., 2020; Pietsch et al., 2020; Blomberg et al., 2022).
Figure 1 presents the timeline for the co-creation process of the IHC. In the initial stage, the focus was on developing and testing a set of hygiene-improving technologies, products, and services at Living Labs. In the next stage, the focus shifted from individual technologies toward co-creating ideas for hygienic indoor environments via joint workshops. At this point, qualitative research methods were utilized to collect data from companies and other key stakeholders. It became evident that the most important barrier to implementing innovative indoor hygiene solutions in the construction industry was the lack of a definition of indoor hygiene. This guided the next stage of the process, in which network actors concentrated on making indoor hygiene “visible” in construction. The network management activities developed by this multisector network to target new market creation have been reported by Aarikka-Stenroos et al. (2017).
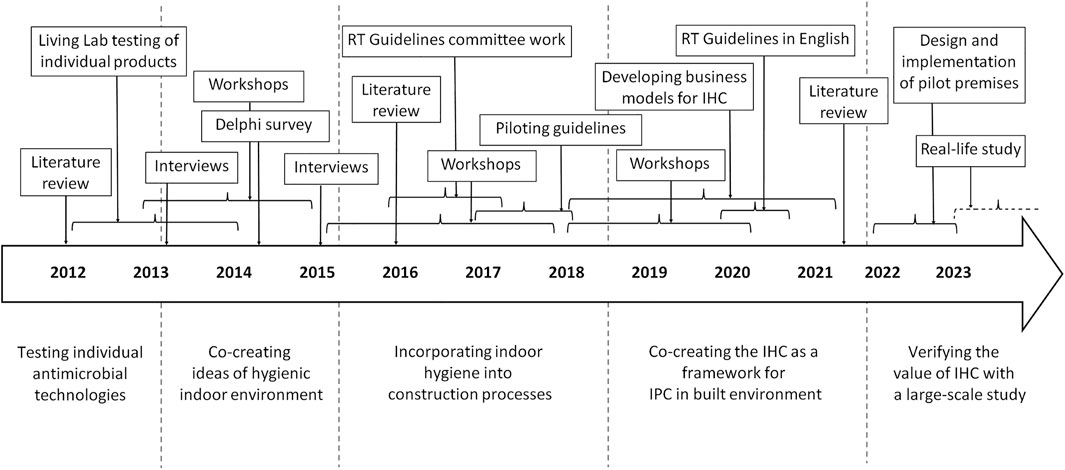
FIGURE 1. Timeline of the process resulting in the introduction of the IHC. For example, data collection surveys, workshops, interviews, RT Guidelines committee work, and Living Labs with microbiological sampling and informal sick-day follow-ups were involved.
To overcome the challenges of innovation implementation caused by the typical features of the project-based construction industry—that is, fragmentation and a strong reliance on competitive tendering (Dubois and Gadde 2002; Aouad et al., 2010; Bygballe and Ingemansson, 2014)—we created and published the National Indoor Hygiene Guidelines (RT Guidelines) together with the Finnish Building Information Foundation RTS sr (Finnish Building Information Foundation RTS sr, 2021a; Finnish Building Information Foundation RTS sr, 2021b; Finnish Building Information Foundation RTS sr, 2021c). The main goals of the Indoor Hygiene Guidelines were 1) to make IPC “visible” to different actors in the construction industry, 2) to create a definition for hygiene in built environments, 3) to establish qualitative criteria for tendering processes, and 4) to address the critical points of a building’s construction process from a hygiene perspective. The RT Guidelines are attached to the construction regulations of Finland and serve practitioners, such as builders, designers, contractors, and building officials as well as property management professionals and property owners.
Previous research has called for better collaboration and communication between the actors in the construction industry to overcome innovation barriers (Hemström et al., 2017). The IHC introduced in this paper provides a framework for infection prevention in built environments and addresses the issue on a general level, making the concept applicable to different regulatory contexts worldwide. It provides a tool for different actors involved in a building’s life cycle for implementing necessary IPC actions to create hygienic indoor environments.
Components of the indoor hygiene concept
According to IHC principles, it is necessary to consider all the significant factors involved in the creation of a hygienic indoor environment. These include touchless and antimicrobial technologies and solutions as well as design, construction, and cleaning and maintenance practices that aim to attain and maintain the desired hygiene level. The components of the IHC are presented in Figure 2.
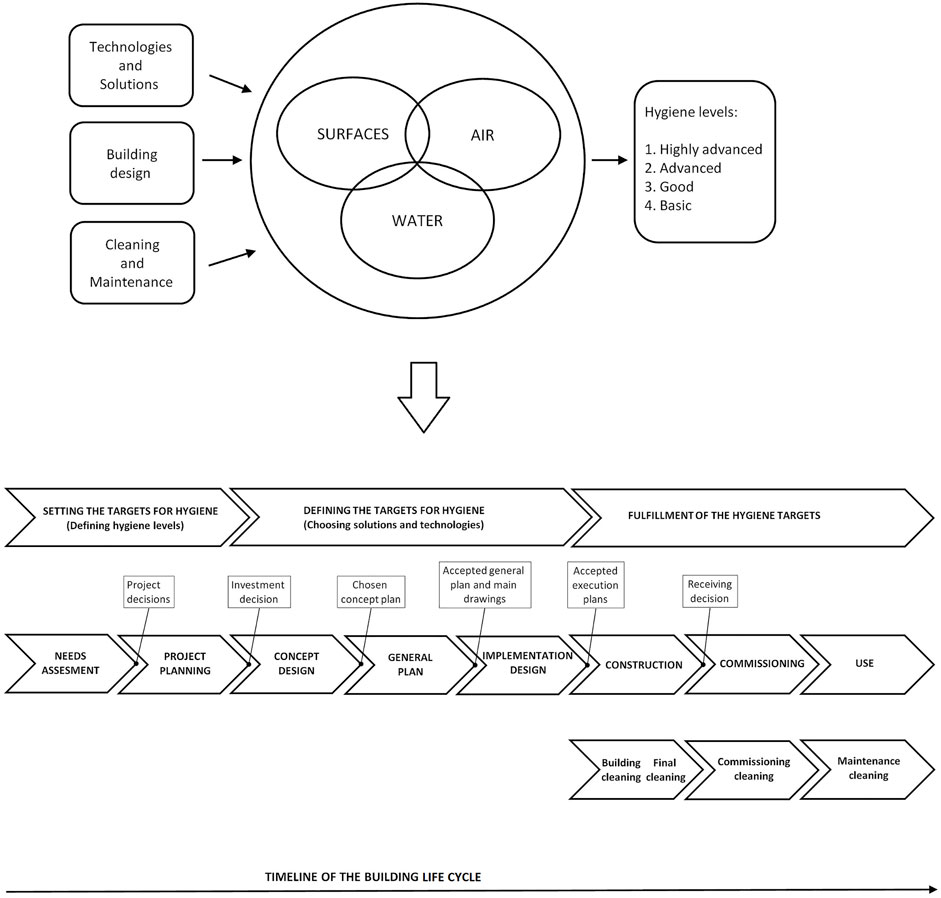
FIGURE 2. The idea of IHC. All the critical components that affect the hygiene of an indoor environment need to be considered to reach the required hygiene level. The IHC should be implemented throughout a building’s life cycle. The points of transition between the phases of a construction project are critical for maintaining the targets of the IHC (timeline adapted from Finnish Building Information Foundation RTS sr, 2021b and Finnish Building Information Foundation RTS sr, 2021c).
An indoor environment consists of three elements—surfaces, air, and water supply—which all must be considered for IPC because of the different habitats of microorganisms and different routes of infection transmission. Contaminated surfaces evidently contribute to the transmission of healthcare-associated infections (Otter et al., 2013). Many bacteria, including antibiotic-resistant microbes, viruses, and pathogenic yeast, can stay viable for long durations on different surfaces, thus making indirect contact infection a significant risk (Cook et al., 2016; Kampf et al., 2020). The hygiene levels of indoor surfaces can be enhanced using, for example, AMCs. AMCs include materials that incorporate silver or copper, which are toxic to a wide range of microbes, and light-activated materials that can degrade organic compounds, including microbes, via generated reactive oxygen species (Birkett et al., 2022). Using AMCs on critical, frequently touched surfaces can stop them from functioning as microbial reservoirs and reduce the risk of onward transmission (Dancer, 2014). AMCs should be carefully considered before applying to avoid problems arising from, e.g., antimicrobial resistance and environmental burdens (Ahonen et al., 2017; Rosenberg et al., 2019; Cassidy et al., 2020; Pietsch et al., 2020).
In addition to surfaces, indoor air can mediate infections via respiratory droplets and aerosols originating from human carriers (Zhang R. et al., 2020; Tang et al., 2021). For example, SARS-CoV-2, the cause of COVID-19, can remain viable and infectious in aerosols for several hours (van Doremalen et al., 2020). To diminish the risk of airborne transmission, the ventilation rate can be increased and special ventilation strategies applied (Cao et al., 2014; Morawska et al., 2021; Izadyar and Miller, 2022). Various air purification and disinfection strategies are available, such as filtration, electrostatic precipitation, and ultraviolet irradiation (Morawska et al., 2020; Zhang J. et al., 2020; Feng et al., 2021).
Certain pathogens can find their way into the human body through building water systems. Biofilms in water systems can form reservoirs for harmful microorganisms that are difficult to destroy. For example, building water systems are the primary sources of Legionnaires’ disease, severe pneumonia caused by the bacterium Legionella pneumophila, and cases have been on the rise in recent years (McCoy and Rosenblatt, 2015). The risk of waterborne infections increases in green buildings with different water- and energy-saving strategies. Waterborne infection transmission can be controlled by, e.g., adjusting water temperature, age, and flow (Leslie et al., 2021).
Existing hygiene-enhancing solutions mainly target separate parts of an indoor environment. However, concentrating on only one individual indoor element does not result in as high a level of hygiene as concentrating on all three elements (i.e., surfaces, air, and water supply). If only one antimicrobial solution is applied, such as antimicrobial door handles or touch-free faucets, the IPC impact will be relatively low compared to implementing several carefully chosen antimicrobial technologies or solutions for all indoor elements.
The construction industry is characterized by specific complexity factors due to the presence of multiple actors and interdependencies. It can be depicted as a loosely coupled system consisting of manufacturing (materials, equipment, etc.) and service (engineering design, consulting, etc.) elements (Dubois and Gadde 2002). Thus, the implementation of a hygienic indoor environment has been challenging. It is not yet possible for a building developer to acquire a turnkey solution for a “hygienic building” from a single operator. A solution needs to be compiled from several fragments, combining technologies, materials, and designs, obtained from different operators in the value network. In addition, building developers often have neither the knowledge nor the time to collect all the necessary individual parts. Likewise, planners and designers may not be familiar with all possible technologies, solutions, and products to enhance indoor hygiene. To overcome these challenges, it is recommended that each construction or renovation project involve a nominated hygiene-dedicated expert who can help set hygiene targets and monitor their fulfillment throughout the project.
Hygiene requirements may differ significantly between different buildings based on each building’s intended use. It is not reasonable to target a completely microbe-free environment, as an indoor environment harbors abundant microbiota that might have positive or negative effects on the occupants (Cassidy et al., 2020). When the users of a building have low immunity, the risks posed by harmful microorganisms are significant. Examples of such buildings are hospitals, nursing homes, and kindergartens.
According to the IHC principles, the hygiene levels of indoor environments should be classified as highly advanced, advanced, good, and basic (Finnish Building Information Foundation RTS sr, 2021a). The hygiene-influencing procedures and solutions applied during the design and construction processes as well as use and maintenance vary according to the building’s hygiene level. Notably, a building may contain indoor spaces with different hygiene levels, and these indoor spaces can be grouped into zones based on their purpose and the user groups involved.
Implementing the indoor hygiene concept
The IHC’s main objective is to increase indoor hygiene levels throughout the life cycle of a building. Hygiene-related objectives should be determined at a very early stage of a construction or renovation project. Figure 2 shows how the IHC is implemented throughout a building’s life cycle. The implementation of IHC should start in the needs assessment phase and continue through the design, construction, furnishing, and use phases (Finnish Building Information Foundation RTS sr, 2021b). Furthermore, after commissioning, maintenance and cleaning are important for maintaining the desired indoor hygiene level in a building or a certain indoor environment (Dancer and Kramer, 2019; Finnish Building Information Foundation RTS sr, 2021c).
Building design decisions are extremely important because they lay the foundation for a hygienic building by, for instance, affecting the movement of people several years into the future. During the design and construction phases, hygiene-related choices are made in architectural, internal facility, premise plumbing system, and HVAC design and when preparing the building’s maintenance guidelines (Dietz et al., 2020; Udomiaye et al., 2020; Megahed and Ghoneim, 2021; Amran et al., 2022; Udomiaye et al., 2022). Thus, the actors involved in every stage of a building’s life cycle need to be aware of how they can contribute to creating hygienic buildings. For example, with respect to architecture, compact, clear, and easy-to-clean structures should be pursued. During spatial planning, dirty and clean areas should be structurally separated to decrease cross-contamination. Adequate spacing is required to support social distancing when needed. Furthermore, the furnishing, equipment, and devices chosen should promote ease of cleaning and maintain high hygiene levels, e.g., through the use of antimicrobial and antifouling materials (Finnish Building Information Foundation RTS sr, 2021b). The overall design should support maintaining proper hand hygiene (Clancy et al., 2021).
The transition points between the different phases of a construction project are of critical importance. At these points, there is a clear risk of forgetting the set objectives (Figure 2). The transition from one phase to another in a construction project is challenging with any innovative solution, and the solution might be discontinued due to cost issues or because the benefits are not known to all actors. The cost estimates may be reviewed in light of the project phases, there may be a change in the planning staff, or the client may make significant decisions regarding the project. The role of the hygiene-dedicated expert is important at these transition points. If the implementation of hygiene objectives is not monitored, the investment costs would be given priority, causing the qualitative objectives related to hygiene to not be achieved.
Due to its connections to spatial planning and architectural engineering, the IHC is most effective in new constructions, but it can also be applied to existing buildings by introducing antimicrobial or touchless solutions on critical surfaces, utilizing suitable technologies for air purification and in water systems, and following maintenance and cleaning protocols.
The construction sector is generally quite strictly regulated. However, regulations are mainly present in the form of national legislation and supporting guidelines. Building developers may even have additional guidelines to follow. Several rating and level systems for the different factors of a building’s indoor environment are available. For example, in Finland, indoor air is evaluated using classification models for the indoor environment, and materials are evaluated according to the emissions classifications of building materials (Ahola et al., 2019). However, the Finnish national building code hardly provides instructions for constructing hygienic buildings. For example, ventilation instructions mostly focus on temperature, humidity, and CO2 levels, but no instructions exist on how to avoid airborne infection transmission. As an exception, certain buildings, such as hospitals, have their own detailed rating systems for indoor spaces that need to be followed. In Finland, the National Indoor Hygiene RT Guidelines were formulated within the IHC creation process to design, construct, and implement hygienic indoor environments throughout a building’s life cycle.
International green (ecological) building certification programs, such as LEED (Leadership in Energy and Environmental Design) and BREEAM (Building Research Establishment Environmental Assessment Method), have been widely adopted to improve indoor environmental quality and building sustainability (Zang et al., 2022). Recently, new certification programs, including WELL and Fitwel, have aimed to put human wellbeing at the center of building design (Licina and Yildrim, 2021). These programs have tried to adapt to the pandemic (Tleuken et al., 2021). However, certifications for hygienic buildings are not yet available. Various construction objectives are sometimes inconsistent with each other. Seeking high hygiene may not be compatible with the objectives of saving energy and water. For example, the high ventilation rate necessary for efficient IPC requires more energy. Furthermore, regularly flushing faucets with hot water and avoiding stagnation causes energy and water consumption. Increasing the understanding of potential hygiene risks in green buildings can help minimize them. Compromises in building design are often needed. When seeking satisfying solutions, it is recommended that the building’s purpose and different indoor spaces are considered.
Green building certification systems are mostly designed to evaluate the sustainability of buildings in a specific region. Sustainability variations may occur due to climate factors, geographical features, and government policies. This also applies to hygienic buildings. For example, different ventilation strategies are required for different climates. Therefore, developing universal instructions for hygienic buildings is challenging.
Conclusion
For humans, indoor environments are the most essential living environments and can significantly impact infection transmission. In addition to direct microbial transmission between humans, infections may be indirectly transmitted via surfaces, indoor air, and water supply. Thus, attention needs to be paid to enhancing hygiene, especially in public buildings where infections spread easily due to high turnovers of people and in buildings accommodating people with low immunity.
A building should be designed and operated according to its purpose so that the infection risk remains within an acceptable, defined hygiene level. To reach this goal, all actors in the building process must share the same understanding of the necessary hygiene level. The IHC provides a tool for different actors in the construction process to manage IPC issues in built environments from the need assessment stage to use and maintenance. All the significant factors related to indoor air, surfaces, and water supply that affect hygiene levels in indoor environments need to be considered. Hygiene-related decisions and actions should be carried out throughout a building’s life cycle. Preventing the spread of infections in built work environments enhances the safety of both the people working in the facilities and those passing through public spaces as customers or visitors. The pandemic challenged both the physical and mental wellbeing of employees in various spaces, especially in healthcare. Enhanced hygiene in the work environment can reduce people’s stress by assuring safety.
Hygiene in indoor environments and the effects of antimicrobial technologies have traditionally been studied by analyzing the burden of microorganisms on targets. Only a few studies have been published on the effects of antimicrobial solutions on a number of infections. Thus, a thorough and large-scale study is required to clarify how a hygienic indoor environment can reduce morbidity. We are currently determining suitable pilot premises to carry out this kind of study. Further, a cost–benefit analysis is required to demonstrate that investments in hygienic buildings would cost less than the treatment of infections and the loss of productivity due to sick leaves.
Data availability statement
The original contributions presented in the study are included in the article/supplementary material, further inquiries can be directed to the corresponding author.
Author contributions
NS prepared the manuscript. RM contributed to writing and editing the text and funding acquisition. MA contributed to supervising the writing process, editing the text, and project administration. TM contributed to writing and editing the text. AP-H contributed to editing the text. ML contributed to supervising the writing process, editing the text, and funding acquisition.
Funding
This study was funded by the Ministry of Education and Culture in Finland and Satakunta University of Applied Sciences. It is a part of the project HEAL: Healthier life with comprehensive indoor hygiene concept. The grant number of Ministry of Culture and Education is: OKM/119/523/2021.
Acknowledgments
We thank the funders and participants of the following projects for facilitating the development of the comprehensive IHC: RYM-SHOK: Finnish iENV project (user-centric indoor environment), 2011–2015, Tekes (currently Business Finland); HYGTECH: Solutions to manage hygiene in indoor environment, 4/2012–10/2014, Tekes ERDF (currently Business Finland); HYGLI: Hygiene into business—HygNess, 11/2014–12/2017, Regional Council of Satakunta ERDF; SIHI: Innovation environment for indoor hygiene, 9/2018–4/2019, Regional Council of Satakunta AIKO; AMiCI: CA COST Action CA15114 Anti-microbial coating innovations to prevent infectious diseases, 4/2016–4/2020, EU COST; IHMEC: Opening indoor hygiene SME’s exports to Middle East construction markets, CB664, 3/2018–4/2021, Interreg Central Baltic ERDF; CIG-15114: ePlatform for a “test bed” tool across EU for antimicrobial coating solutions in health care entering to the market, 5/2020–10/2021, EU COST; TERVA: Health Ahoy!—Healthy and hygienic ships, 3/2021–08/2023, Regional Council of Satakunta ERDF; HEAL: Healthier life with comprehensive indoor hygiene concept, 1/2022 onwards, The Ministry of Education and Culture, Finland.
Conflict of interest
The authors declare that the research was conducted in the absence of any commercial or financial relationships that could be construed as a potential conflict of interest.
Publisher’s note
All claims expressed in this article are solely those of the authors and do not necessarily represent those of their affiliated organizations, or those of the publisher, the editors and the reviewers. Any product that may be evaluated in this article, or claim that may be made by its manufacturer, is not guaranteed or endorsed by the publisher.
References
Aarikka-Stenroos, L., Jaakkola, E., Harrison, D., and Mäkitalo-Keinonen, T. (2017). How to manage innovation processes in extensive networks: A longitudinal study. Ind. Mark. Manag. 67, 88–105. doi:10.1016/j.indmarman.2017.09.014
Ahola, M., Säteri, J., and Sariola, L. (2019). Revised Finnish classification of indoor climate 2018. E3S Web Conf. 111, 02017. doi:10.1051/e3sconf/201911102017
Ahonen, M., Kahru, A., Ivask, A., Kasemets, K., Kõljalg, S., Mantecca, P., et al. (2017). Proactive approach for safe use of antimicrobial coatings in healthcare settings: Opinion of the COST action network AMiCI. Int. J. Environ. Res. Public Health 14, 366. doi:10.3390/ijerph14040366
Albarqouni, L., Byambasuren, O., Clark, J., Scott, A. M., Looke, D., and Glasziou, P. (2020). Does copper treatment of commonly touched surfaces reduce healthcare-acquired infections? A systematic review and meta-analysis. J. Hosp. Infect. 106, 765–773. doi:10.1016/j.jhin.2020.09.005
Amran, M., Makul, N., Fediuk, R., Borovkov, A., Ali, M., and Zeyad, A. M. (2022). A review on building design as a biomedical system for preventing COVID-19 pandemic. Buildings 12, 582. doi:10.3390/buildings12050582
Aouad, G., Ozorhon, B., and Abbott, C. (2010). Facilitating innovation in construction: Directions and implications for research and policy. Const. Innov. 10, 374–394. doi:10.1108/14714171011083551
Birkett, M., Dover, L., Lukose, C. C., Zia, A. W., Tambuwala, M. M., and Serrano-Aroca, Á. (2022). Recent advances in metal-based antimicrobial coatings for high-touch surfaces. Int. J. Mol. Sci. 23, 1162. doi:10.3390/ijms23031162
Blomberg, E., Herting, G., Rajarao, G. K., Mehtiö, T., Uusinoka, M., Ahonen, M., et al. (2022). Weathering and antimicrobial properties of laminate and powder coatings containing silver phosphate glass used as high-touch surfaces. Sustainability 14, 7102. doi:10.3390/su14127102
Boytz, R., Slabicki, M., Ramaswamy, S., Patten, J. J., Zou, C., Meng, C., et al. (2022). Anti-SARS-CoV-2 activity of targeted kinase inhibitors: Repurposing clinically available drugs for COVID-19 therapy. J. Med. Virol. 2022, 1–10. doi:10.1002/jmv.28157
Bygballe, L., and Ingemansson, M. (2014). The logic of innovation in construction. Ind. Mark. Manag. 43, 512–524. doi:10.1016/j.indmarman.2013.12.019
Cao, G., Sirén, K., and Kilpeläinen, S. (2014). Modelling and experimental study of performance of the protected occupied zone ventilation. Energy Build. 68, 515–531. doi:10.1016/j.enbuild.2013.10.008
Cassidy, S. S., Sanders, D. J., Wade, J., Parkin, I. P., Carmalt, C. J., Smith, A. M., et al. (2020). Antimicrobial surfaces: A need for stewardship? PLoS Pathog. 16, e1008880. doi:10.1371/journal.ppat.1008880
Clancy, C., Delungahawatta, T., and Dunne, C. P. (2021). Hand-hygiene-related clinical trials reported between 2014 and 2020: A comprehensive systematic review. J. Hosp. Infect. 111, 6–26. doi:10.1016/j.jhin.2021.03.007
Colin, M., Klingelschmitt, F., Charpentier, E., Josse, J., Kanagaratnam, L., De Champs, C., et al. (2018). Copper alloy touch surfaces in healthcare facilities: An effective solution to prevent bacterial spreading. Materials 11, 2479. doi:10.3390/ma11122479
Cook, N., Knight, A., and Richards, G. P. (2016). Persistence and elimination of human norovirus in food and on food contact surfaces: A critical review. J. Food Prot. 79, 1273–1294. doi:10.4315/0362-028X.JFP-15-570
Cutler, D. M., and Summers, L. H. (2020). The COVID-19 pandemic and the $16 trillion virus. JAMA 324, 1495–1496. doi:10.1001/jama.2020.19759
Dancer, S. J. (2014). Controlling hospital-acquired infection: Focus on the role of the environment and new technologies for decontamination. Clin. Microbiol. Rev. 27, 665–690. doi:10.1128/CMR.00020-14
Dancer, S. J., and Kramer, A. (2019). Four steps to clean hospitals: LOOK, PLAN, CLEAN and DRY. J. Hosp. Infect. 103, e1–e8. doi:10.1016/j.jhin.2018.12.015
Dietz, L., Horve, P. F., Coil, D. A., Fretz, M., Eisen, J. A., and Vand Den Wymelenberg, K. (2020). 2019 novel coronavirus (COVID-19) pandemic: Built environment considerations to reduce transmission. mSystems 5, e002455-20. doi:10.1128/mSystems.00245-20
Dubois, A., and Gadde, L. E. (2002). The construction industry as a loosely coupled system: Implications for productivity and innovation. Constr. Manag. Econ. 20 (7), 621–631. doi:10.1080/01446190210163543
Dunne, C. P., Askew, P. D., Papadopoulos, T., Gouveia, I. C., Ahonen, M., Modic, M., et al. (2020). Antimicrobial coating innovations to prevent infectious disease: A consensus view from the AMiCl COST action. J. Hosp. Infect. 105, 116–118. doi:10.1016/j.jhin.2020.04.006
Dunne, S. S., Ahonen, M., Modic, M., Crijns, F. R. L., Keinänen-Toivola, M. M., Meinke, R., et al. (2018). Specialized cleaning associated with antimicrobial coatings for reduction of hospital acquired infection. Opinion of the COST Action Network AMiCI (CA15114). J. Hosp. Infect. 99, 250–255. doi:10.1016/j.jhin.2018.03.006
Feng, Z., Cao, S. J., Wang, J., Kumar, P., and Haghighat, F. (2021). Indoor airborne disinfection with electrostatic disinfector (ESD): Numerical simulations of ESD performance and reduction of computing time. Build. Environ. 200, 1–12. doi:10.1016/j.buildenv.2021.107956
Finnish Building Information Foundation RTS sr (2021c). Hygiene in indoor spaces. Cleaning and maintenance. RT 103193 en. 1–11. Available at: https://www.rakennustieto.fi/en (Accessed June 13, 2022).
Finnish Building Information Foundation RTS sr (2021a). Hygiene in indoor spaces. General principles. RT 103191 en. 1–9. Available at: https://www.rakennustieto.fi/en (Accessed June 13, 2022).
Finnish Building Information Foundation RTS sr (2021b). Hygiene in indoor spaces. Spatial planning. RT 103192 en. 1–10. Available at: https://www.rakennustieto.fi/en (Accessed June 13, 2022).
Hemström, K., Mahapatra, K., and Gustavsson, L. (2017). Architects’ perception of the innovativeness of the Swedish construction industry. Constr. Innov. 17 (2), 244–260. doi:10.1108/CI-06-2015-0038
Hernando-Amado, S., Coque, T. M., Baquero, F., and Martínez, J. L. (2019). Defining and combating antibiotic resistance from one health and global health perspectives. Nat. Microbiol. 4, 1432–1442. doi:10.1038/s41564-019-0503-9
Inkinen, J., Ahonen, M., Iakovleva, E., Karppinen, P., Mielonen, E., Mäkinen, R., et al. (2019). Contamination detection by optical measurements in a real-life environment: A hospital case study. J. Biophot. 13, e2019600699. doi:10.1002/jbio.201960069
Inkinen, J., Jayaprakash, B., Ahonen, M., Pitkänen, T., Mäkinen, R., Pursiainen, A., et al. (2017b). Bacterial community changes in copper and PEX drinking water pipeline biofilms under extra disinfection and magnetic water treatment. J. Appl. Microbiol. 124, 611–624. doi:10.1111/jam.13662
Inkinen, J., Mäkinen, R., Keinänen-Toivola, M. M., Nordström, K., and Ahonen, M. (2017a). Copper as an antibacterial material in different facilities. Lett. Appl. Microbiol. 64, 19–26. doi:10.1111/lam.12680
Izadyar, N., and Miller, W. (2022). Ventilation strategies and design impacts on indoor airborne transmission: A review. Build. Environ. 218, 109158. doi:10.1016/j.buildenv.2022.109158
Kampf, G., Todt, D., Pfander, S., and Steinmann, E. (2020). Persistence of coronaviruses on inanimate surfaces and their inactivation with biocidal agents. J. Hosp. Infect. 104, 246–251. doi:10.1016/j.jhin.2020.01.022
Kenyon, C. (2020). Emergence of zoonoses such as COVID-19 reveals the need for health sciences to embrace an explicit eco-social conceptual framework of health and disease. Epidemics 33, 100410–100415. doi:10.1016/j.epidem.2020.100410
Latva, M., Inkinen, J., Rämö, J., Kaunisto, T., Mäkinen, R., Ahonen, M., et al. (2016). Studies on the magnetic water treatment in new pilot scale drinking water system and in old existing real-life water system. J. Water Process Eng. 9, 215–224. doi:10.1016/j.jwpe.2016.01.009
Lazary, A., Weinberg, I., Vatine, J. J., Jefidoff, A., Bardenstein, R., Borkow, G., et al. (2014). Reduction of healthcare-associated infections in a long term care brain injury ward by replacing regular linens with biocidal copper oxide impregnated linens. Int. J. Infect. Dis. 24, 23–29. doi:10.1016/j.ijid.2014.01.022
Leslie, E., Hinds, J., and Hai, F. I. (2021). Causes, factors and control measures of opportunistic premise plumbing pathogens – A critical review. Appl. Sci. (Basel). 11, 4474. doi:10.3390/app11104474
Licina, D., and Yildrim, S. (2021). Occupant satisfaction with indoor environmental quality, sick building syndrome (SBS) symptoms, and self-reported productivity before and after relocation into WELL-certified office buildings. Build. Environ. 204, 108183. doi:10.1016/j.buildenv.2021.108183
Mäkinen, R., Miettinen, I. T., Pitkänen, T., Kusnetsov, J., Pursiainen, A., Kovanen, S., et al. (2013). Manual faucets induce more biofilms than electronic faucets. Can. J. Microbiol. 59, 407–412. doi:10.1139/cjm-2013-0131
Marcus, E. L., Yosef, H., Borkow, G., Caine, Y., Sasson, A., and Moses, A. E. (2017). Reduction of health care–associated infection indicators by copper oxide–impregnated textiles: Crossover, double-blind controlled study in chronic ventilator-dependent patients. Am. J. Infect. Control 45, 401–403. doi:10.1016/j.ajic.2016.11.022
McCoy, W. F., and Rosenblatt, A. A. (2015). HACCP-Based programs for preventing disease and injury from premise plumbing: A building consensus. Pathogens 4, 513–528. doi:10.3390/pathogens4030513
Megahed, N. A., and Ghoneim, E. M. (2021). Indoor air quality: Rethinking rules of building design strategies in post-pandemic architecture. Environ. Res. 193, 110471–110479. doi:10.1016/j.envres.2020.110471
Mehraeen, E., Najafi, Z., Hayati, B., Javaherian, M., Rahimi, S., Dadras, O., et al. (2022). Current treatments and therapeutic options for COVID-19 patients: ASystematic review. Infect. Disord. Drug Targets. 22 (1), 62–73. doi:10.2174/1871526521666210726150435
Morawska, L., Allen, J., Bahnfleth, W., Bluyssen, P. M., Boerstra, A., Buonanno, G., et al. (2021). A paradigm shift to combat indoor respiratory infection. Science 372, 689–691. doi:10.1126/science.abg2025
Morawska, L., Tang, J. W., Bahnfleth, W., Bluyssen, P. M., Boerstra, A., Buonnonno, G., et al. (2020). How can airborne transmission of COVID-19 indoors be minimised? Environ. Int. 142, 105832–105837. doi:10.1016/j.envint.2020.105832
Nalbandian, A., Sehgal, K., Gupta, A., Madhavan, M. V., McGroder, C., Stevens, J. S., et al. (2021). Post-acute COVID-19 syndrome. Nat. Med. 27, 601–615. doi:10.1038/s41591-021-01283-z
Otter, J. A., Yezli, S., Salkeld, J. A. G., and French, G. L. (2013). Evidence that contaminated surfaces contribute to the transmission of hospital pathogens and an overview of strategies to address contaminated surfaces in hospital settings. Am. J. Infect. Control 41, 6–11. doi:10.1016/j.ajic.2012.12.004
Pietsch, F., O’Neill, A. J., Ivask, A., Jenssen, H., Inkinen, J., Kahru, A., et al. (2020). Selection of resistance by antimicrobial coatings in the healthcare setting. J. Hosp. Infect. 106, 115–125. doi:10.1016/j.jhin.2020.06.006
Polivka, L., Gajdacsi, J., Fazekas, L., Sebok, S., Barczi, E., Hidvegi, E., et al. (2022). Long-term survival benefit of male and multimorbid COVID-19 patients with 5-day remdesivir treatment. J. Glob. Health 12, 05031. doi:10.7189/jogh.12.05031
Rosenberg, M., Ilić, K., Juganson, K., Ivask, A., Ahonen, M., Vrček, I. V., et al. (2019). Potential ecotoxicological effects of antimicrobial surface coatings: A literature survey backed up by analysis of market reports. PeerJ 7, e6315. doi:10.7717/peerj.6315
Salari, N., Hosseinian-Far, A., Jalali, R., Vaisi-Raygani, A., Rasoulpoor, S., Mohammadi, M., et al. (2020). Prevalence of stress, anxiety, depression among the general population during the COVID-19 pandemic: A systematic review and meta-analysis. Glob. Health 16, 57. doi:10.1186/s12992-020-00589-w
Salgado, C. D., Sepkowitz, K. A., John, J. F., Cantey, J. R., Attaway, H. H., Freeman, K. D., et al. (2013). Copper surfaces reduce the rate of healthcare-acquired infections in the intensive care unit. Infect. Control Hosp. Epidemiol. 34, 479–486. doi:10.1086/670207
Sifri, C. D., Burke, G. H., and Enfield, K. B. (2016). Reduced health care-associated infections in an acute care community hospital using a combination of self-disinfecting copper-impregnated composite hard surfaces and linens. Am. J. Infect. Control 44, 1565–1571. doi:10.1016/j.ajic.2016.07.007
Tang, J. W., Bahnfleth, W. P., Bluyssen, P. M., Buonanno, G., Jimenez, J. L., Kurnitski, J., et al. (2021). Dismantling myths on the airborne transmission of severe acute respiratory syndrome coronavirus-2 (SARS-CoV-2). J. Hosp. Infect. 110, 89–96. doi:10.1016/j.jhin.2020.12.022
Tleuken, A., Tokazhanov, G., Guney, M., Turkyilmaz, A., and Karaca, F. (2021). Readiness assessment of green building certification systems for residential buildings during pandemics. Sustainability 13, 460. doi:10.3390/su13020460
Udomiaye, E., Osondu, E. D., and Kalu, K. C. (2020). Architectural design strategies for infection prevention and control (IPC) in health-care facilities: Towards curbing the spread of covid-19. J. Environ. Health Sci. Eng. 18, 1699–1707. doi:10.1007/s40201-020-00580-y
Udomiaye, E., Ukpong, E., Kalu, K. C., Odum, C., and Okon, I. U. (2022). A review of sustainable design strategies for infection prevention and control (IPC) in public buildings. IOP Conf. Ser. Earth Environ. Sci. 1054, 012015. doi:10.1088/1755-1315/1054/1/012015
van Doremalen, N., Lloyd-Smith, J. O., and Munster, V. J. (2020). Aerosol and surface stability of HCoV-19 (SARS-CoV-2) compared to SARS-CoV-1. N. Engl. J. Med. 382, 16. doi:10.1101/2020.03.09.20033217
von Dessauer, B., Navarrete, M. S., Benadof, D., Benavente, C., and Schmidt, M. G. (2016). Potential effectiveness of copper surfaces in reducing health care-associated infection rates in a pediatric intensive and intermediate care unit: A nonrandomized controlled trial. Am. J. Infect. Control 44, e133–e139. doi:10.1016/j.ajic.2016.03.053
Weber, D. J., Otter, J. A., and Rutala, W. A. (2017). Can copper-coated surfaces prevent healthcare-associated infections? Infect. Control Hosp. Epidemiol. 38, 772–776. doi:10.1017/ice.2017.90
Zang, J., Royapoor, M., Acharya, K., Jonczyk, J., and Werner, D. (2022). Performance gaps of sustainability features in green award-winning University buildings. Build. Environ. 207, 108417. doi:10.1016/j.buildenv.2021.108417
Zerbib, S., Vallet, L., Muggeo, A., de Champs, C., Lefebvre, A., Jolly, D., et al. (2020). Copper for the prevention of outbreaks of health care-associated infections in a long-term care facility for older adults. J. Am. Med. Dir. Assoc. 21, 68–71.e1. doi:10.1016/j.jamda.2019.02.003
Zhang, J., Huntley, D., Fox, A., Gerhardt, B., Vatine, A., and Cherne, J. (2020). Study of viral filtration performance of residential HVAC filters. ASHRAE J. 62, 26–32.
Keywords: indoor environment, indoor hygiene, infection prevention, construction process, building life cycle
Citation: Salonen N, Mäkinen R, Ahonen M, Mäkitalo T, Pelto-Huikko A and Latva M (2022) A comprehensive indoor hygiene concept for infection prevention and control within built environments. Front. Built Environ. 8:1075009. doi: 10.3389/fbuil.2022.1075009
Received: 20 October 2022; Accepted: 15 November 2022;
Published: 25 November 2022.
Edited by:
Hasim Altan, Arkin University of Creative Arts and Design (ARUCAD), CyprusReviewed by:
Arianna Brambilla, The University of Sydney, AustraliaCopyright © 2022 Salonen, Mäkinen, Ahonen, Mäkitalo, Pelto-Huikko and Latva. This is an open-access article distributed under the terms of the Creative Commons Attribution License (CC BY). The use, distribution or reproduction in other forums is permitted, provided the original author(s) and the copyright owner(s) are credited and that the original publication in this journal is cited, in accordance with accepted academic practice. No use, distribution or reproduction is permitted which does not comply with these terms.
*Correspondence: Noora Salonen, bm9vcmEuc2Fsb25lbkBzYW1rLmZp
†Present address: Tiina Mäkitalo, RoboAI Research and Development Center, Satakunta University of Applied Sciences, Pori, Finland