- 1Université de Tunis El Manar, Ecole Nationale d’Ingénieurs de Tunis, LR14ES03 Laboratoire d’Ingénierie Géotechnique et Géorisque, Tunis, Tunisia
- 2Université de Tunis, Ecole Nationale Supérieure d’Ingénieurs de Tunis, Département de Génie Civil, Tunis, Tunisia
This article first introduces a new method of characterization of expansive clays after oedometer test results performed on intact saturated clay specimens. On the presumed expansive clays specimens, oedometer tests are preceded by the free swelling test from which the swelling pressure is measured. From current oedometer test results carried out on expansive and non-expansive clays, the ratio of compression index (Cc) by the swelling index (Cs) was determined. A threshold value of the ratio Cc/Cs was identified to distinguish between expansive clay specimens and non-expansive clay specimens. Second, a novel mitigation method of the swelling phenomena was validated by performing oedometer tests on expansive clay specimens preceding the measurement of swelling pressure. Oedometer tests performed on a 53% thickness clay specimen overlaid by a 47% thickness of sand showed a significant reduction of the swelling pressure compared to that measured on a full expansive clay specimen. The mitigation solution reduced the swelling effect by placing a compacted granular layer as an interface between the expansive clay and the foundation. This solution has been adopted and approved in forthcoming a lightweight building construction at the Faculty of Sciences of Tunis City.
1 Introduction
Expansive soils typically clays are a problematic soil category, which often poses serious pathology, especially when lightweight constructions are built on it. Hence, studying the behaviour of foundations on expansive soils is a theme of high interest, in particular for many African countries (Kalantari, 2012; Nelson et al., 2015; Sridharan and Prakash, 2016).
The swelling phenomenon attracted several investigators, first, to explain how it can occur (Sridharan and Prakash, 2016). Then, which methods are suitable for measuring the swelling pressure. In this regard, several contributions have been published (Hussain and Dash, 2011; Plaisted and Zornberg, 2011; Gueddouda et al., 2013; Aniculaesi and Lungu, 2019).
The main issue is to find out how to mitigate the swelling phenomenon to build foundations, retaining structures in contact with expansive soils. Chemical treatment of expansive clays and improvement solutions, like using granular material, were proposed (Gueddouda et al., 2013; Fattah et al., 2017, etc.). To prevent the occurrence of the swelling phenomenon, the peripheral drainage trench revealed a suitable method to control water evacuation away from structural elements in contact with these problematic clays.
In North of Tunisia, several projects are in progress where expansive clays exist from the ground surface and extend to deep layers. In the three last decades, the repair of several constructions was affected by extreme damage attributed to the swelling phenomena (Bouassida et al., 2006). Hence, the Tunisian Ministry of Equipment, building and infrastructures have made it a priority of special attention when designing foundations on expansive clays. Such a decision needs a research program that aims to characterize expansive clays suitably and to formulate countermeasures and construction methods to help civil engineers by conducting safe designs and for the execution of foundations on this problematic type of soil.
This article focuses on how the swelling phenomenon is triggered in expansive clays. There is, then, a brief bibliographic review of the damage occasioned by expansive soils, with proposed methods for their classification and methods of mitigation of the swelling phenomena.
First, the article suggests a new approach for characterizing expansive soils from oedometer test results and, second, the mitigation of swelling phenomenon by using a granular material, as an interface tested in a laboratory, for reducing the swelling pressure on foundations.
Previous methods of classification of expansive soils have focused on the use of plasticity parameters and the free-swell test result. The gap between those existing approaches appears in the absence of comparison between those methods. Non-consideration of the current oedometer parameters can be thought of as a minor limitation that can be helpful.
The merit of the suggested approach is to consider the soil parameters of current use, as determined by the oedometer tests, without consideration of the free-swell test result. Therefore, the suggested method of classification is applicable both for expansive and non-expansive soils. The interpretation of the results and main findings are addressed in the final section.
2 Problem Statement
2.1 Swelling Mechanism
The swelling phenomenon of soil occurs progressively and depends on the soil saturation and its clay structure (Medjnoun et al., 2014). The volume of expansive soils changes according to their moisture content (Figure 1). When adding water to expansive clays, their molecules are drawn into the space between the clay particles (Figure 2). As water is absorbed, the clay particles move apart, increasing the swelling pressure (Elarabi, 2010).
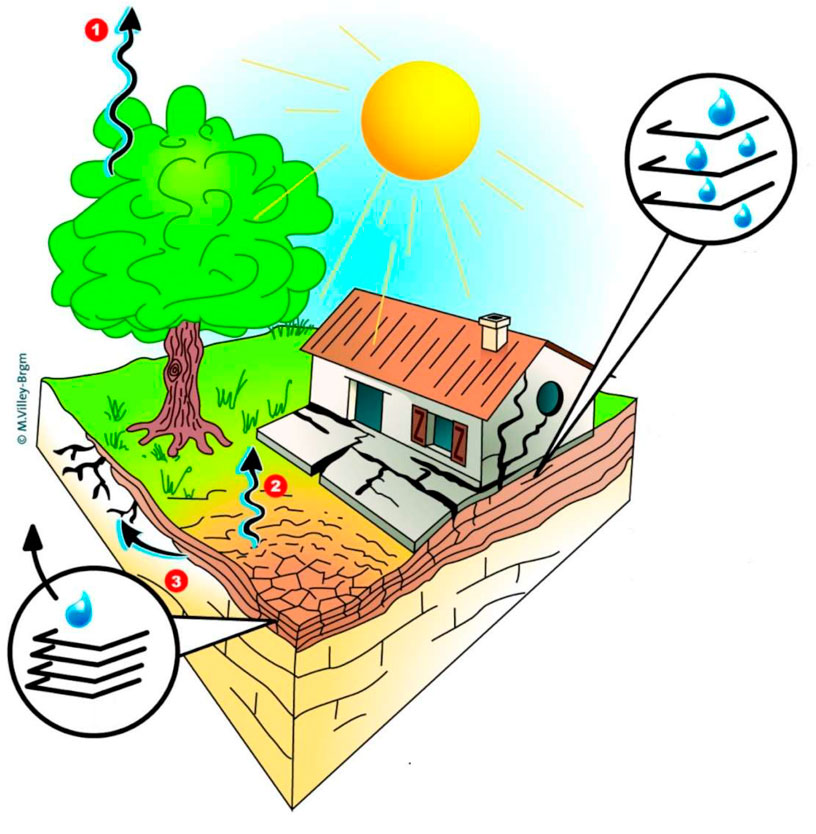
FIGURE 1. Illustration of shrink-swell clay, (1) evapotranspiration, (2) evaporation and (3) root absorption (Villey, 2010).
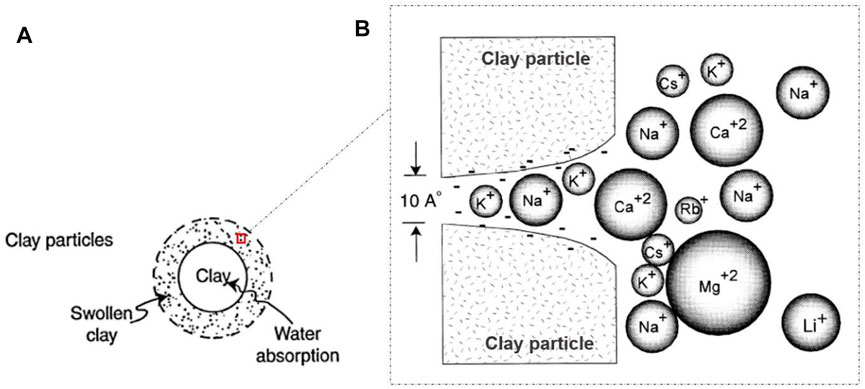
FIGURE 2. Schemes of (A) expansion of a clay particle and (B) insertion of cations with their relative dimensions (Civan, 2016).
The swelling phenomenon in expansive clays is complex and arises from an electrochemical process. The latter affects the distribution of internal stresses between the soil particles (Kehew, 1995). Clay particles are plate-shaped, having a negative charge on their surface in presence of the interstitial water solution and water molecules. The molecules of water are polar, and are attracted to the surface of the clay particles. This interaction is modelled by two types of forces: Van der Waals surface forces and the adsorption forces between clay minerals and water molecules. Due to the externally applied stress and the capillary tension in the soil water, an equilibrium can be reached in the internal electrochemical system (Sahin, 2011).
The second factor overcoming the swelling behaviour is the migration of cations. The latter are generally transferred from a higher to a lower concentration in a solution to ensure that the ions are evenly distributed. As ions are retained by clay particles in expansive soils (Figure 2B), there is a movement of water from low ionic concentration areas to high ionic concentration areas inside the clay. This water movement generates pressure and, consequently, induces the swelling of the clay (Sahin, 2011).
To better illustrate this process, exchangeable cations are attracted to the surface of the negatively charged clay particles. Figure 2B shows the effect of cation size on cation migration into an interparticle. The space between the clay particles is smaller than the dimensions of some cations. When cations migrate, the interlayer is shifted because of the weak liaisons between the clay particles (Figure 2B). The volume increases (Figure 2A), then followed by the swelling of the clay. This phenomenon is reversible when the water content decreases due to evaporation, evapotranspiration and absorption by the roots (Figure 1). Meanwhile, there is a high probability of cracks appearance in the soil. For instance, the soil swells during the high humidity season with induced deformation in a retaining wall. Whilst, in the warm season, the expansive soil shrinks, then fissures appear (Figures 3A,B) which will be filled by dust or also by plants growing in it. Figure 4 illustrates the occurrence of soil cracking in an expansive clay. Among the clay minerals, the smectite group is responsible for soil’s high swelling properties, in particular montmorillonite (Chen, 1988). This is due to the weakness of layer liaisons. The swelling and shrinkage behaviour of clay is a very dangerous phenomenon that leads to several kinds of damage and huge repair costs. Hence, finding methods to characterize this category of soil is crucial. As for mitigation, adequate methods to prevent the swell effect should be formulated.
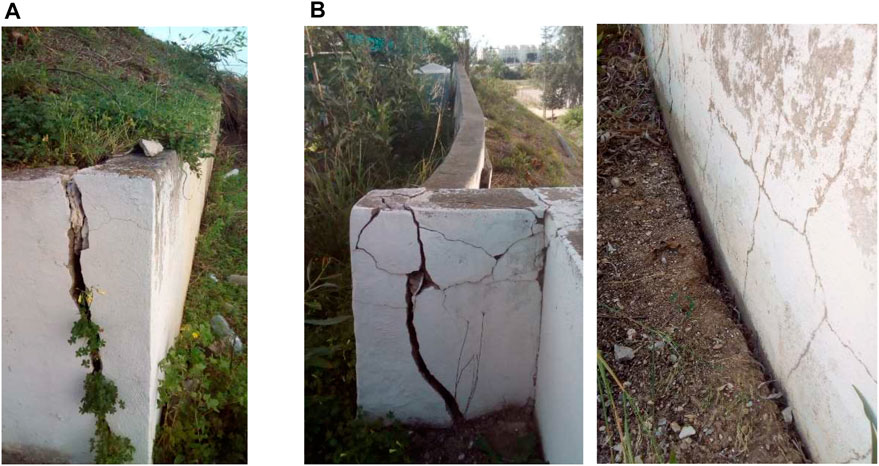
FIGURE 3. Damages in retaining walls at the National Engineering School of Tunis (Manigniavy, 2021). (A). reinforced concrete wall subjected to active pressures induced by expansive clays. (B). Unreinforced concrete wall subjected to active pressures induced by expansive clays.
3 Background
3.1 Damages Caused by Expansive Soils
3.1.1 Structural Damages
Expansive soils are among the top natural hazards and phenomena such as earthquakes, hurricanes, floods and tornadoes (Chen, 1975). Three (03) main factors control the triggering of swelling phenomenon and related damages on structures (Holtz and Kovacs, 1981): the presence of montmorillonite, with natural water content close to the soil’s plastic limit, and a water source near a potential expansive soil. The existence of swelling soils can lead to slope instability, a differential heave buckling of pavement, a differential settlement of roads or railways, retaining walls’ buckling (Figures 3A,B), tunnels collapsing, and damages to retaining walls. Destruction of hydraulic structures such as buried pipelines, drains, sewage systems and irrigation systems are very dangerous and can be fatal in certain conditions. Without precautions, canals can be destroyed by foundations built on expansive clays (Elarabi, 2010). This could be risky for users.
3.1.2 Cost Damages
Annually, billions of dollars are spent worldwide in repairing the severe damage that currently affects constructions as a result of the swell phenomenon. The most reported types of damages are cracking of pavements, basement walls, floors, foundations and hydraulic structures. The cost of repairing these damages is quite high (Osman and Charlie, 1983; Nuhfer, 1994; Azam et al., 2013). According to Mostafiz et al. (2021), the high cost associated with maintenance and repairing is comparable to other sources of damage (subsidence, inadmissible settlement, etc). To date, many countries have not yet made financial assessments of the losses related to expansive soil problems.
3.2 Characterization of Expansive Soils
Boscardin and Cording (1989) proposed a classification based on structural damages, more precisely on the width of cracks. According to Sridharan and Prakash (2016), two characterization types are distinguished. The first type of characterization considers the knowledge of expansive soils’ mineralogy, such as X-ray diffraction analysis (Athmania et al., 2010) or differential thermal analysis, dye adsorption, chemical analysis and scanning electron microscopy. The second type of characterization uses data obtained from an inferential test either by direct or by indirect methods. There is a strong correlation between swelling characteristics and basic soil parameters (Kim et al., 2013). Several researchers have found ways to classify expansive soils by indirect methods: Atterberg limits tests (Holtz and Gibbs, 1956; IS:1498, 1970; Chen, 1975), activity method (Skempton, 1953; Seed et al., 1962; Sridharan and Prakash, 2016) and the clay fraction method (Holtz and Gibbs, 1956; Chen, 1965; Holtz et al., 2011). The characterization is referred to direct methods rather use data from the oedometer swell test, free swell tests and suction measurement. According to Holtz and Gibbs (1956), the conventional oedometer swell test is the most useful and reliable assessment of the swell potential.
3.3 Methods of Swell Mitigation
Expansive clays have become a worldwide concern in the field of geotechnical engineering because of the problems they cause in several countries. To take full and optimal advantage of the ideal Smart Cities, improvements are required to mitigate or adapt to this type of soil. To reduce the swelling pressure, there are many methods proposed such as the use of piles (Kay, 1990; Al-Busoda et al., 2017), chemical treatments (Lahmadi et al., 2013; Mahamedi and Khemissa, 2013) and soil mixture (Gueddouda et al., 2013; Fattah et al., 2017; Tiwari et al., 2019).
Furthermore, soil replacement, use of strong enough structures and structure isolation from the swelling clay are among the three most commonly used techniques (Kalantari, 2012).
4 New Approach
4.1 Characterization of Expansive Soils Using Cc/Cs Ratio
The proposed characterization method is based on the Cc/Cs ratio by using data collected from four (04) case histories and selected ones from others countries (Algeria and United States). Table 1 details those data as determined from oedometer tests preceded by the measurement of the swell pressure. Table 2 gives a first classification showing the difference between expansive and non-expansive soils. Compression index (Cc) and the swelling index (Cs) are determined from an oedometer test during which the tested soil specimen is permanently submerged, hence full saturation condition applies for the tested soil specimen during loading and unloading steps. One can interpret this ratio as an indicator of volume variation quantifying the degree of the soil compression (Cc) with respect to the soil swell (Cs). Performing an oedometer test, those two indices can be determined for any saturated soil either expansive or non-expansive. From collected data, Figure 5 plots the variation of the swell pressure vs Cc/Cs ratio. From this figure, it is seen that data of non-expansive soils, characterised by a swell pressure lower or equal to nearly 50 kPa belong to the side where Cc/Cs ratio is greater than 10. Also, it is noted when Cc/Cs ratio exceeds 15, the swell pressure is almost zero. In turn, when Cc/Cs ratio is lower than 8 one can identify expansive soils for which the swell pressure is in the range of 75–400 kPa. Data comprises Tunisian and two non-Tunisian soils. This method of characterization is in accordance with Chen (1988) who stated the swell potential is low when the swell pressure is lower than 50 kPa.
The plasticity chart in Figure 6 reveals that data collected for non-swelling clays are located in the low plasticity zone.
Therefore, the classification method herein presented is in good agreement with several methods for the characterization of expansive soils (Table 3).
Figures 7A,B show the difference between the respective oedometer curves of a non-expansive soil and an expansive soil, however without having any notice about the measurement of the free swell. During the unloading phase, comparison between Figures 7A,B indicate that the increase in thickness of the expansive soil specimen is not only attributed to the release of vertical stress, the second fact is attributed to the swell induced by the infiltrated water within the soil specimen.
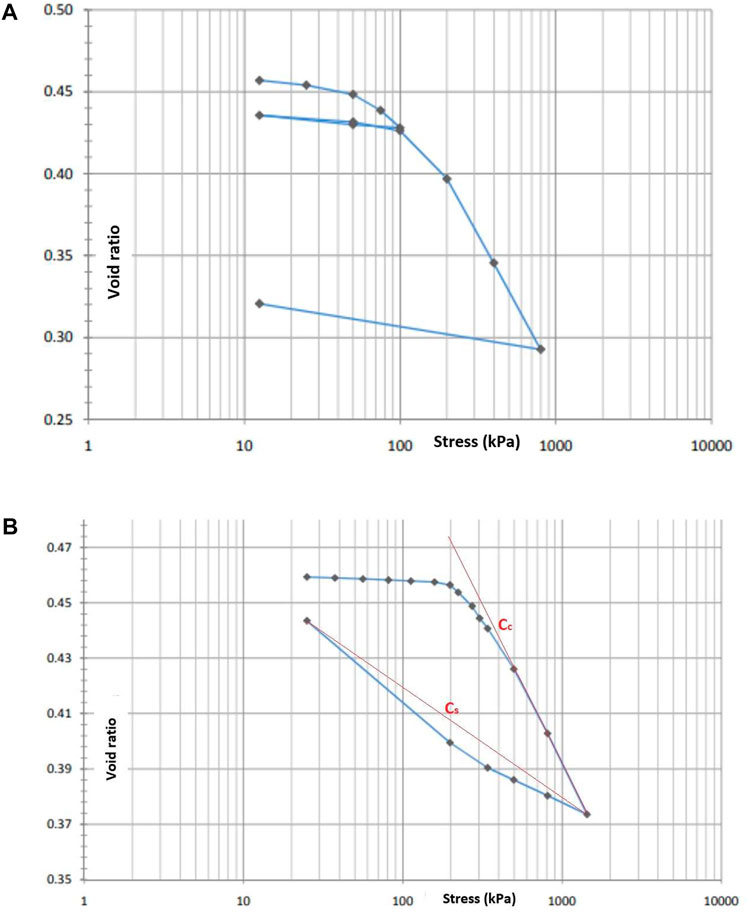
FIGURE 7. (A) Oedometer curve of a Tunis El Manar University’s non-expansive soil (SC2-EI21 sample) with Cc/Cs = 19.2. (B) Oedometer curve of a Tunis El Manar University’s expansive soil (SC1-EI14 sample) with Cc/Cs = 4.2.
4.2 Use of a Granular Layer to Mitigate the Swelling Phenomenon
Mitigation of the swelling phenomena can be attained by using a granular layer separation between the foundation and the swelling clay. This layer plays, first, the role of an absorber of the swelling pressure. Second, the drainage role is facilitated by the high permeability of the granular material. This design, as shown in Figure 8A, was chosen because the load distribution (swelling pressure of soil) on the foundation is different to that on a granular layer, which is non-continuous loading (Figure 8B). This is because the contact between the granular material particles can be a point, line or surface.
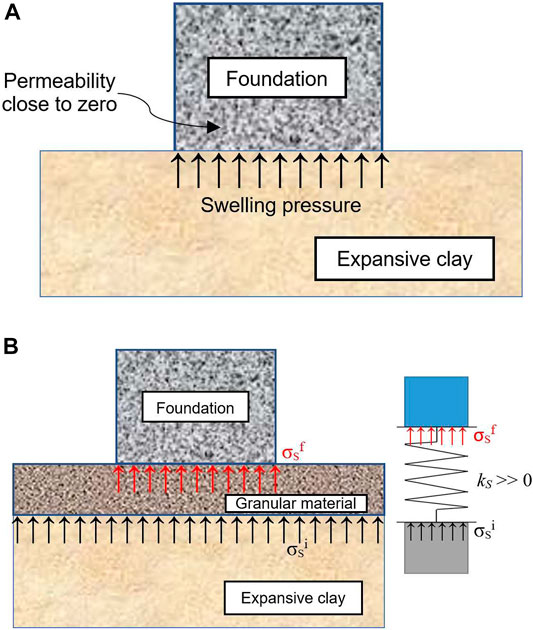
FIGURE 8. (A) Swelling pressure applied to a foundation in contact with an expansive clay layer. (B) Load distribution by adding a granular layer σSf < σSi.
4.2.1 Oedometer Test
To analyze the effect of the granular for mitigating the swelling pressure, two oedometer tests were performed on a pure clay specimen. As shown in Figure 7B, the compression index Cc and the swelling index Cs are determined from the oedometer curve.
In this study, two distinct oedometer tests are proposed:
⁃ only with expansive clay sample (Figure 9A)
⁃ with expansive clays sample plus a granular layer (Figure 9B)
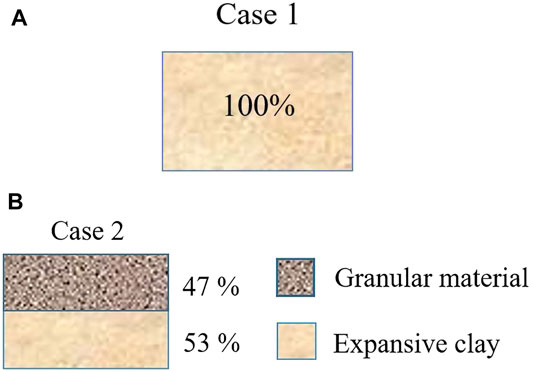
FIGURE 9. (A) An expansive clay sample. (B) A composite sample: 53% of expansive clay and 47% of granular material.
Finding the optimum efficiency and thickness of the granular layer to reduce the swelling pressure is the objective.
Two cored samples, noted S1 and S2 (Figure 10; Table 4), have been extracted at the site of the National Engineering School of Tunis at a depth of 0.6 m (Figures 3A,B).
Case 1: ( Only S1 expansive clay)
An intact sample from the S1 core, of water content 15.9%, is placed in the oedometer ring as shown in Figure 11. The unique load on the sample is the mass of the porous stone which is converted to a pressure of 0.25 kPa. The oedometer cell is filled with water to ensure the soil saturation and continuous circulation of the water. A displacement sensor enables the recording of the evolution of swelling pressure (Figure 12). The temperature in the distilled water in the lab was 24°C.
Case 2: ( Sand + S1)
This case, illustrated in Figures 13A,B, 1 cm of the oedometer ring thickness is filled with expansive soil S1 and 0.9 cm remaining with compacted sand (ρSand = 1.806 g/cm3; wiSand = 5%; 1 mm < D ≤ 1.250 mm). Oedometer swell test is applied to Case 2. In this experiment, the clay layer covering the bottom 1 cm of the ring thickness, under porous stone and sand layer, is loaded with a total of 0.43 kPa. Indeed, the mass of sand layer and porous stone is 167.2 g and the oedometer ring diameter is 7 cm. A filter paper is used to separate the sand layer and the expansive clay layer.
4.2.2 Experimental Results and Interpretations
4.2.2.1 Identification Test Results
The extracted specimen comes from the National Engineering School of Tunis site, nearby the retaining wall shown in Figures 3A,B. According to the classification by Chen (1975), the physical parameters of this sample, given in Table 4, indicate that it has a medium swell potential.
By referring to Sridharan and Prakash (2016), this specimen has low activity and a low probability to swell. Activity, noted Ac, is calculated as the ratio between the plasticity index and the percentage of particles of dimensions less or equal to 2 µm. Therefore, based on those two classification methods the swelling characterization is not confirmed.
Given the swelling behaviour of this sample, an oedometer swell test was performed and discussed hereafter.
4.2.2.2 Comparison of the Results for the Two Case Studies
Expansive clay sample S1 grain size distribution shows that the dimensions of 91% of the particles is inferior to 80 μm. After observed results, the specimen swelling is noticed (Figures 14A,B). The two cases do not have the same swelling rate or the same behaviour. The compacted sand layer acts as a shock absorber to reduce the swelling pressure effect of the soil.
Case 1: For the first hour and a half, it is clear that case one’s curve, shown in Figure 14A, keeps the swelling at a medium rate, approximately 38.4 nm/s. After 01 h 38 min from the beginning of the free swell test, the applied load to the specimen is increased to the observed swelling. Each time, the swelling evolution attains a plateau corresponding to the end of swelling. The oedometer cell is filled to ensure saturation and to compensate for the amount of absorbed water. The soil swelling continued until 0.54 mm upward displacement after 24 h, subject to 11.85 kPa vertical stress (Figure 15A). Then, the swelling stabilized during 02 h 30 min. Then, the specimen settlement started.
Case 2: Upward vertical displacement vs time displayed in Figure 14B shows a different evolution as recorded for the specimen case 1 (Figure 14A). During time, the swelling rate decreases; in fact, in the intervals time (0; 2000 s); (2000; 4,500 s); (4,500; 5,000 s); (5,000; 6,000 s), the swelling rates are equal to 22.4 nm/s, 38.5 nm/s, 17.1 nm/s, 36 nm/s and 15 nm/s, respectively. An explanation of such behaviour might be the friction resistance induced between the sand particles. Five hours after the beginning of the test, the upward vertical displacement equals 0.22 mm (Figure 15B). Then, the specimen stabilized at this deformation level.
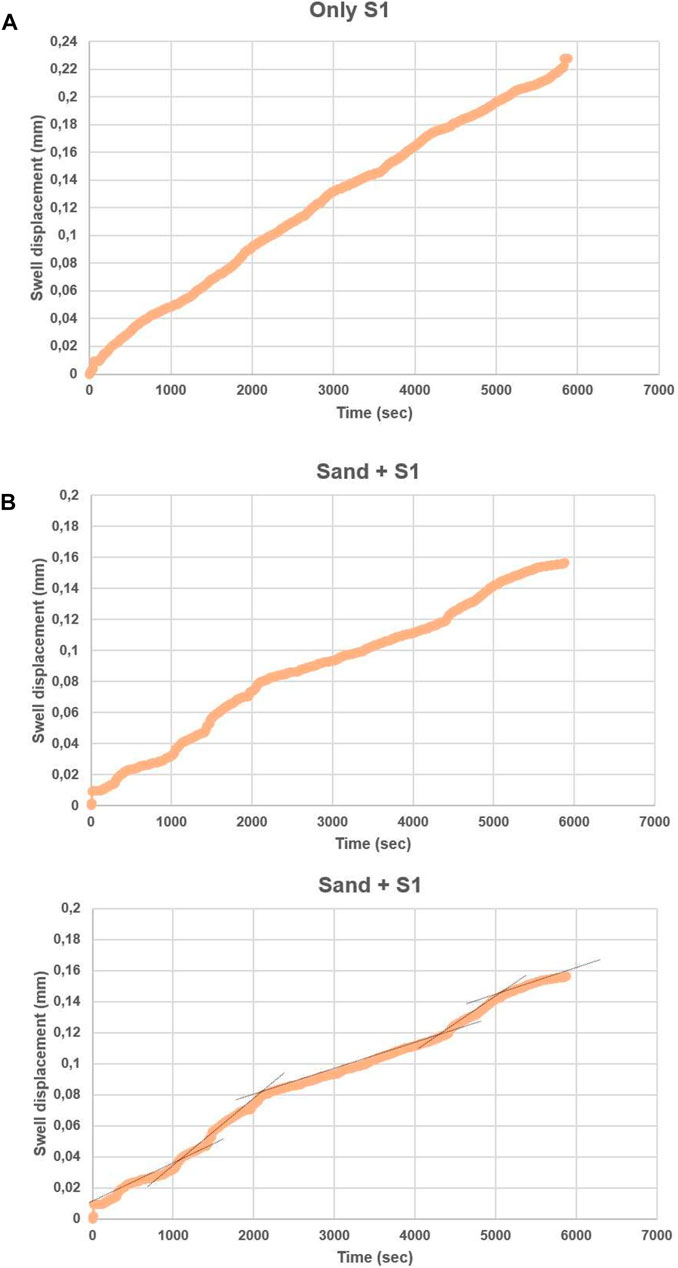
FIGURE 14. (A) Free swell—Time curve of case 1 (only expansive clay) under 0.25 kPa. (B) Free swell—Time curve of case 2 (47% Sand +47% S1) under 0.25 kPa.
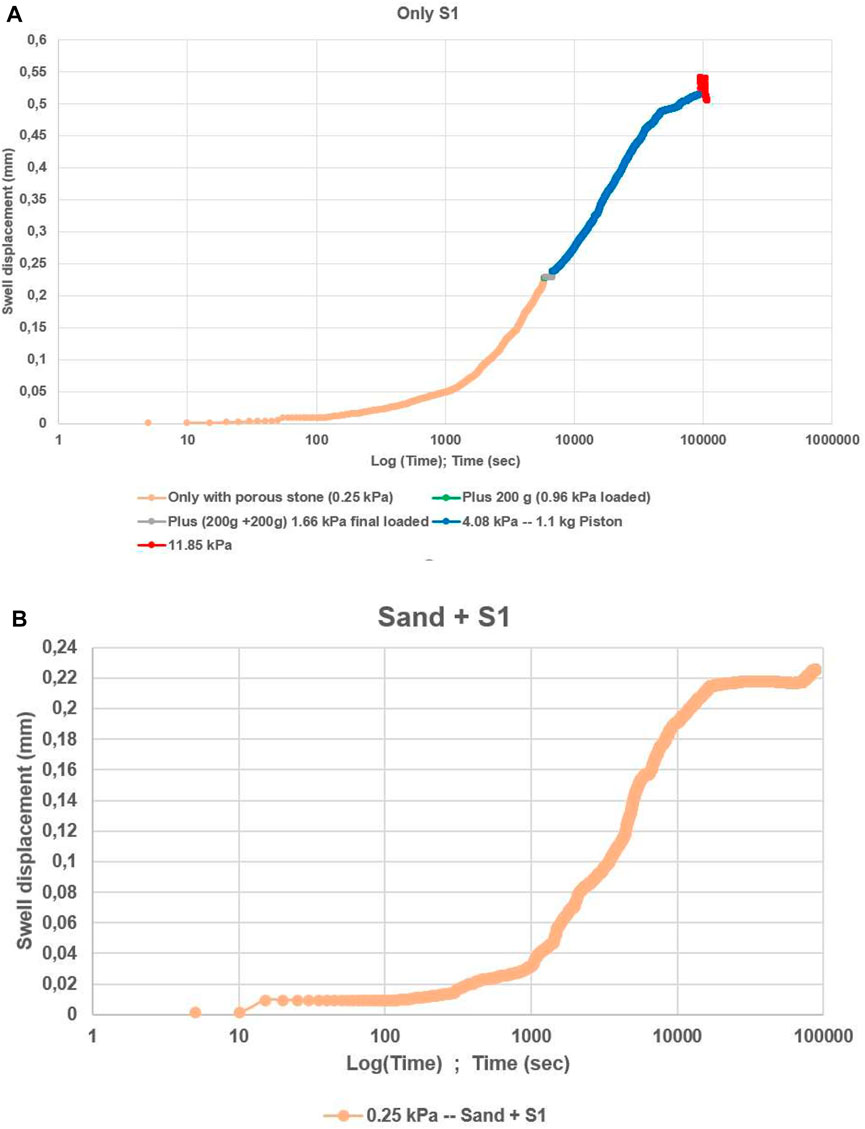
FIGURE 15. (A) Swelling evolution of an expansive clay (Case 1) under different loads. (B) Swelling evolution of Case 2 specimen (47% Sand +53% S1) under 0.25 kPa.
Table 5 illustrates the vertical displacements and the final stress levels for both cases. A reduction in deformation of 21.4% is observed, then, follows the decrease in the swelling pressure compared to the tested specimen case 1.
Deformation in case 1: ε(1) = Δl(1) / l(1) = 0.54 / 19 = 0.028
Deformation in case 2 : ε(2) = Δl(2) / l(2) = 0.22 / 10 = 0.022, which approximates 78.6% of ε(1).
5 Conclusion
This paper addressed the study of the classification and mitigation of expansive soils. Based on oedometer curves, when the Cc/Cs ratio exceeds 10, the soil is concluded to be either non-swelling or its swelling potential is negligible. Whilst when the Cc/Cs<10, one should consider calculating the swelling pressure. Compiled data of about Thirty-one (31) specimens mostly collected from four Tunisian sites and two sites (Algeria and United States), revealed that soils with Cc/Cs ≤ 8; their swelling pressure exceeds 50 kPa.
The present study also showed, after experimental laboratory investigation, that the use of a granular material layer (sand) as an interface between the foundation and an expansive clay layer reduces the swelling of tested specimen, in oedometer condition, to 21.4%, for a HG/HS ratio equal to 0.9 (HG: the thickness of the granular layer and HS: the thickness of an expansive soil).
The novelty of the present study relies on adopting a different analysis, as compared to existing methods, based on oedometer data, especially the compression and swelling indices. Throughout the ratio Cc/Cs value, one can perceive if the tested soil is expansive or non-expansive, without the need for the measurement of the swell pressure in the free swell phase. Such an indicator is easily determined from an existing test and of great help in the practical assessment of expansive soils.
The validation of the suggested method of classification needs, first, the collection of a big number of oedometer data, for expansive and non-expansive soils from different case histories. Second, the implementation of a scaled test model might lead to a better assessment of the use of a granular layer to mitigate the swelling effect.
In the future, this research aims to collect more data to confirm the first findings on the characterization of expansive clays and to further investigate the performance of using granular material as a separation zone between foundations and expansive soil to mitigate the swelling phenomenon.
Data Availability Statement
The original contributions presented in the study are included in the article/Supplementary Material, further inquiries can be directed to the corresponding author.
Author Contributions
BM: principal investigator; S.A. Manigniavy: PhD fellow D. Azaiez: Ph D fellow Y. Bouassida: co supervisor of PhD fellow
Conflict of Interest
The authors declare that the research was conducted in the absence of any commercial or financial relationships that could be construed as a potential conflict of interest.
Publisher’s Note
All claims expressed in this article are solely those of the authors and do not necessarily represent those of their affiliated organizations, or those of the publisher, the editors, and the reviewers. Any product that may be evaluated in this article, or claim that may be made by its manufacturer, is not guaranteed or endorsed by the publisher.
Abbreviations
Cc:Compression index; Cs: Swelling index; ρ: unit mass; σ: stress; σf: final applied stress level; σs: swelling pressure; ε :deformation; l: thickness of the expansive clay; Δl: upward swelling displacement; HG: thickness of granular material layer; HS: thickness of expansive clay layer; D: dimension; ENIT: National Engineering School of Tunis; UTM: University of Tunis El Manar.
References
Al-Busoda, B. S., Abid Awn, S. H., and Obaid, H. (2017). Numerical Modeling of Retaining Wall Resting on Expansive Soil. Geotechnical Eng. J. SEAGS AGSSEA.
Aniculaesi, M., and Lungu, I. (2019). Evaluation of the Swelling Pressure for Expansive Soils. IOP Conf. Ser. Mater. Sci. Eng. 586, 012012. doi:10.1088/1757-899x/586/1/012012
Sridharan, A., and Prakash, K. (2016). Expansive Soil Characterisation: an Appraisal. Inael 1 (1), 29–33. doi:10.1007/s41403-016-0001-9
Athmania, D., Benaissa, A., Hammadi, A., and Bouassida, M. (2010). Clay and Marl Formation Susceptibility in Mila Province, Algeria. Geotech Geol. Eng. 28 (6), 805–813. doi:10.1007/s10706-010-9341-5
Azam, S., Ito, M., and Chowdhury, R. (2013). “Engineering Properties of an Expansive Soil,” in Proceeding of the 18th International Conference on Soil Mechanics and Geotechnical Engineering, Paris, 199–202.
Boscardin, M. D., and Cording, E. J. (1989). Building Response to Excavation‐Induced Settlement. J. Geotech. Engrg. 115 (1), 1–21. doi:10.1061/(asce)0733-9410(1989)115:1(1)
Bouassida, M., Ben Ouezdou, M., and Trabelsi, H. (2006). Cas pathologiques de deux bâtiments à l’ENIT. Ann. de l’équipement N°26, 53–66.
Bowels, J. E. (1988). Foundation Analysis and Design. New York City: McGraw-Hills Inc., U.S.A., 312–316.
Chen, F. H. (1975). Foundations on Expansive Soils. New York: Elsevier Scientific Publishing Company Inc.
Chen, F. H. (1965). “The Use of Piers to Prevent the Uplifting of Lightly Loaded Structure Founded on Expansive Soil,” in Concluding Proceedings Engineering Effects of Moisture Change in Soils, International Research and Engineering Conference on Expansive Clay Soils. A&M, Texas, 152–171.
Civan, F. (2016). Mineral Sensitivity of Petroleum-Bearing Formations**After Civan, 1999a, 2001c SPE, Parts Reprinted by Permission of the Society of Petroleum Engineers from SPE 52134 and SPE 67293. Reservoir Formation Damage 2016, 77–125. doi:10.1016/b978-0-12-801898-9.00005-9
Elarabi, H. (2010). “Damage Mechanism of Expansive Soils.” in Proceeding of the 2nd International Conference on Geotechnical Engineering ICGE’10, Tunisia, 125–131.
Fattah, M. Y., Salim, N. M., and Irshayyid, E. J. (2017). Influence of Soil Suction on Swelling Pressure of Bentonite-Sand Mixtures. Eur. J. Environ. Civil Eng. 2017, 1–15. doi:10.1080/19648189.2017.1320236
Federal Highway Administration Research and Technology (1999). A Quarter Century of Geotechnical Research - Chapter 4: Soil and Rock Behavior. Washington, DC, USA: U.S. Department of Transportation.
Gueddouda, M. K., Goual, I., Lamara, M., and Goual, S. (2013). “Amélioration des propriétés physico-mécaniques des argiles gonflantes stabilisées par ajout de sable de dune,” in 3ème Conférence Maghrébine en Ingénierie Géotechnique CMIG’13, Alger, 221–226.
Holtz, R. D., and Kovacs, W. D. (1981). Introduction to Geotechnical Engineering. Hoboken, NJ, USA: Prentice-Hall, 590.
Holtz, R. D., Kovacs, W. D., and Sheahan, T. C. (2011). An Introduction to Geotechnical Engineering. 2nd Edition. ISBN-10: 0132496348.
Holtz, W. G., and Gibbs, H. J. (1956). Engineering Properties of Expansive Clays. T. Am. Soc. Civ. Eng. 121, 641–663. doi:10.1061/taceat.0007325
Hussain, M., and Dash, S. K. (2011). “Swelling Behavior of Soils,” in Proceedings of the Golden Jubilee Indian Geotechnical Conference, Kochi, 229–232. Paper No. D-366.
Indian Standard IS:1498 (1970). (reaffirmed 1987). Indian Standard Classification and Identification of Soils for General Engineering Purposes. New Delhi: BIS.
Kalantari, B. (2012). Foundation on Expansive Soils: A Review. Res. J. Appl. Sci. Eng. Technol. 4 (18), 3231–3237.
Kehew, E. A. (1995). Geology for Engineers and Environmental Scientists. 2nd Edition. New Jersey: Prentice Hall Englewood Cliffs.
Kim, H-K., Shin, H., and Kang, Y-T. (2013). “Experimental and Numerical Study on Swelling Potential Estimation of Expansive Clay Mixtures,” in Proceedings of the 3rd International Conference on Geotechnical Engineering ICGE’13, Tunisia, 281–288.
Lahmadi, A., Titoum, M., and Bakir, N. (2013). “Chemical Treatment of Expansive Soil by Different saline Solutions,” in Proceedings of the 3rd International Conference on Geotechnical Engineering ICGE’13, Tunisia, 341–349.
Mahamedi, A., and Khemissa, M. (2013). “Cement and Lime Stabilization of Compacted Expansive Clay,” in Proceedings of the 3rd International Conference on Geotechnical Engineering ICGE’13, Tunisia, 369–377.
Manigniavy, S. A. (2021). Characterization of Expansive Clays. Master Thesis. Tunis, Tunisia: National Engineering School of Tunis, University of Tunis El Manar.
Medjnoun, A., Bahar, R., and Khiatine, M. (2014). Caractérisation et estimation du gonflement des argiles algériennes, cas des argiles de Médéa. MATEC Web of Conferences 11, 03004. doi:10.1051/matecconf/20141103004
Medjnoun, A., and Bahar, R. (2016). Shrinking-swelling of clay under the Effect of Hydric Cycles. Innov. Infrastruct. Solut. 1 (1). doi:10.1007/s41062-016-0043-6
Mostafiz, R. B., Friedland, C. J., Rohli, R. V., Bushra, N., and Held, C. L. (2021). Property Risk Assessment for Expansive Soils in Louisiana. Front. Built Environ. 7, 754761. doi:10.3389/fbuil.2021.754761
Nelson, J. D., Chao, K. C., Overton, D. D., and Nelson, E. J. (2015). Foundation Engineering for Expansive Soils. Hoboken, New Jersey: John Wiley & Sons. 978-1-118-41799-7.
Osman, M. A., and Charlie, W. A. (1983). Expansive Soil in Sudan, BBRI Current Papers. Khartoum, Sudan: No. CP.3/83. Building and Road Research Institute. University of Khartoum.
Plaisted, M. D., and Zornberg, J. G. (2011). “Testing of Expansive Clays in a Centrifuge Permeameter,” in Pan-Am CGS Geotechnical Conference.
Sahin, H. (2011). Characterization of Expansive Soil for Retaining wall Design. Master's thesis (College Station, Texas: Texas A&M University, USA).
Seed, H. B., Woodward, R. J., and Lundgren, R. (1962). Prediction of Swelling Potential for Compacted Clays. J. Soil Mech. Found. Div. 88 (3), 53–87. doi:10.1061/jsfeaq.0000431
Skempton, A. W. (1953). “The Colloidal Activity of Clays,” in Proceedings of the 3rd International Conference on Soil Mechanics and Foundation Engineering, Switzerland, 57–61.
Snethen, D. R., Johnson, L. D., and Patrick, D. M. (1977). “An Evaluation of Expedient of Methodology for Identification of Potentially Expansive Soils,” in Soils and Pavements Lab, US Army Eng. Water Way Exp. Station, Vicksburg, MS. Report No. FHWA-RE-77-94, NTIS PB-289-164.
Tiwari, N., Saytam, N., and Patva, J. (2019). “Experimental Study on the Swelling Behavior of Expansive Soil Reinforced with Coir Geotextile,” in Proceedings of the Indian Geotechnical Conference 2019: IGC – 2019, Volume 4. doi:10.1007/978-981-33-6564_11
Villey, M. (2010). Cartographie de l'aléa retrait-gonflement des argiles dans le département de l'Ille-et-Vilaine. BRGM/RP-58120-FR, page 17.
Keywords: expansive clay, characterization, granular material, swelling pressure, mitigation, oedometer test, compression index, swelling index
Citation: Bouassida M, Manigniavy SA, Azaiez D and Bouassida Y (2022) New Approach for Characterization and Mitigation of the Swelling Phenomenon. Front. Built Environ. 8:836277. doi: 10.3389/fbuil.2022.836277
Received: 15 December 2021; Accepted: 15 February 2022;
Published: 24 March 2022.
Edited by:
Wan-Huan Zhou, University of Macau, Macao SAR, ChinaCopyright © 2022 Bouassida, Manigniavy, Azaiez and Bouassida. This is an open-access article distributed under the terms of the Creative Commons Attribution License (CC BY). The use, distribution or reproduction in other forums is permitted, provided the original author(s) and the copyright owner(s) are credited and that the original publication in this journal is cited, in accordance with accepted academic practice. No use, distribution or reproduction is permitted which does not comply with these terms.
*Correspondence: Mounir Bouassida, mounir.bouassida@enit.utm.tn