- 1Epicentre Research Group, Department of Civil, Environmental and Geomatic Engineering, University College London (UCL), London, United Kingdom
- 2Department of Earthquake Engineering, Bogazici University, Istanbul, Turkey
- 3Department of Civil, Maritime and Environmental Engineering, Southampton University, Southampton, United Kingdom
- 4Civil and Environmental Engineering Department, Imperial College London, London, United Kingdom
- 5ARUP, Ankara, Turkey
- 6Department of Civil Engineering, University of Patras, Patras, Greece
- 7Independent Researcher, Samos, Greece
- 8Department of Civil Engineering, Earthquake Engineering Research Centre (EERC), Middle East Technical University, Ankara, Turkey
- 9Department of Civil Engineering, University of Greenwich, London, United Kingdom
- 10Mott Macdonald, London, United Kingdom
- 11Department of Architecture, Izmir University of Economics, Izmir, Turkey
- 12Seismological Laboratory, Faculty of Geology and Geoenvironment, National Kapodistrian University of Athens, Athens, Greece
- 13Atkins, London, United Kingdom
- 14Department of Earth Sciences, University of Cambridge, Cambridge, United Kingdom
- 15Department of Architecture, Canakkale Onsekiz Mart University, Çanakkale, Turkey
- 16School of Earth and Environmental Sciences, Cardiff University, Cardiff, United Kingdom
- 17Arup, London, United Kingdom
- 18School of Environmental Sciences, University of East Anglia, Norwich, United Kingdom
- 19Department of Civil Engineering, Newcastle University, Newcastle, United Kingdom
- 20School of Computing, Engineering and Built Environment, Glasgow Caledonian University, Glasgow, United Kingdom
On 30 October 2020, an earthquake of Mw 6.9 hit the Aegean coasts of Turkey and Greece. The epicentre was some 14 km northeast of Avlakia on Samos Island, and 25 km southwest of Seferihisar, Turkey, triggering also a tsunami. The event has been followed by >4,000 aftershocks up to Mw 5.2 The Earthquake Engineering Field Investigation Team (EEFIT) has immediately gathered a team to conduct a hybrid reconnaissance study, bringing together remote and field investigation techniques. The mission took place between 16 November and 17 December, inclusive of three sets of field study carried out by the field crews for building damage assessment in the affected areas in Turkey and Greece under the coordination of the remote team. The mission also aimed to assess the viability of alternative data sources for an appraisal of the future viability of hybrid missions. This paper summarises the mission setup and findings, and discusses the benefits of and difficulties encountered during this hybrid reconnaissance activity.
1 Introduction
On 30 October 2020 at 1:51 p.m. Eastern European time (11:51 UTC), an earthquake of Mw 6.9 (KOERI, 2020; NOA, 2020) hit the Aegean coasts of Turkey and Greece. The epicentre was some 14 km northeast of the Greek town Avlakia on Samos Island, and 25 km southwest of Seferihisar, Turkey, at a 10–21 km reported depth. The reported intensity of the event was as high as VIII MMI (USGS, 2020). The event has been followed by >4,000 aftershocks up to Mw 5.2. The event also triggered a tsunami which affected the coastline from Alaçatı to Gümüldür in Turkey, and from Karlovasi and Vathy in northern Samos with wave heights reaching up to 1.9 m (Dogan et al., 2021). The LastQuake App developed by the European-Mediterranean Seismological Centre (EMSC) suggests that the event was felt from as far as Croatia and Romania and north Macedonia (Contreras et al., 2022).
The fault plane solutions and previous field observations point out a pure north-dipping normal faulting motion (Akinci et al., 2021; Kiratzi et al., 2021). The surface projection of the fault plane crosses just the North of the island of Samos. The strong ground vibrations from the mainshock triggered the seismic stations of both the Greek and Turkish networks operated by the Ministry of Interior Disaster and Emergency Management Presidency (AFAD), Boğaziçi University’s Kandilli Observatory and Earthquake Research Institute (KOERI), National Observatory of Athens Seismic Network (NOA) and ITSAK Strong Ground Motion Network. The mainshock was only recorded by two stations in the east of Samos Island, which are the closest to the earthquake epicentre. As expected, the highest peak ground acceleration (PGA) was measured in NS component of one of these stations at about 0.24 g, which is slightly smaller than the expected PGA value of the Greece zonation map (2003) for a 475-years return period. In Turkish territories, while a station in Gümüldür produced a maximum PGA (≈0.21 g), the softest soil station in İzmir Basin-the most damaged area—recorded 0.15 g, which is below the expected PGAs of the recent Turkish earthquake hazard map (2019) for the design basis earthquake.
The most immediate direct economic losses due to the event were officially estimated as ₺415 M and €81 M for Turkey [based on the payout figures reported by the DASK (2021), which are rather conservative compared to the reported insured losses by Doğan (2020), in the range of ₺1.8B] and Greece [based on figures reported at Governmental Gazette 5293/1 December 2020, which are similarly much lower than the estimated €120 M when the expenditure reported by samos24 (2021) are accounted for]. For more details see Aktas et al. (2021), respectively. The building and infrastructure damage concentrated in two districts of Izmir, Bayraklı and Bornova, located around 70 km away from the epicentre of the event and built on alluvial sediments and active normal faults [Izmir Fault (IF) and Karşıyaka Fault Zone (KFZ)]. This area suffered from 13 building collapses and around 200 heavy damage cases (TMoEUCC, 2020). Out of the total of 119 causalities, 116 took place in these districts, while one lost their life in Seferihisar due to the tsunami. In Samos, the event caused two casualties, due to the collapse of an abandoned traditional masonry building. That neither Turkey nor Greece had specific protocols during an ongoing pandemic made the response and recovery process following the earthquake challenging.
The Earthquake Engineering Field Investigation Team (EEFIT) of the United Kingdom Institute of Structural Engineers (IStructE) decided on 2 November 2020 to deploy a mission for reasons including 1) the dramatic damage levels in Turkey, accompanied by a high death toll; 2) the tsunami affecting the area; and 3) the then ongoing COVID-19 pandemic affecting the impact of the event and subsequent recovery efforts. On 5 November a team leader was appointed, and the call for expression of interest was issued. The team was finalised by 13 November and started the mission work on the 16th, during the second national lockdown in England.
It is due to the pandemic and the lockdown that the team adopted an unconventional strategy and coordinated the mission remotely. The EEFIT mission to the 30 October 2020 Aegean Earthquake and Tsunami had the following specific objectives pertinent to its special circumstances:
- Combine field and remote survey strategies for a hybrid mission for damage assessment in buildings, critical infrastructure and geotechnical structures.
- Recruit, train and deploy local field crews on the damage assessment tools developed during the Learning from Earthquakes (LfE) project, namely the LfE Mobile App.
- Investigate the extent to which other data sources can be used for remote reconnaissance in support of fieldwork.
- Conduct a systematic and comparative analysis of the impact of the earthquake and tsunami over a wide geographic area.
- Develop an understanding as to how the LfE Mobile App can be tailored to address the specificities of this event.
- Develop an understanding of how the lockdown restrictions and additional pressures induced by the ongoing pandemic affected the response and recovery operations.
The mission lasted approximately 1 month roughly until the 17th December, when the mission lecture took place (accessible from: https://www.istructe.org/resources/case-study/2020-eefit-aegean-earthquake-mission/). During that time, the team gathered information on the event and its impact on buildings and infrastructure from initial reports by other research groups and news items, scanned social media (Contreras et al., 2022) interviewed 12 key stakeholders in Turkish and Greek governmental and non-governmental organisations, launched a public survey to gauge people’s response to and perception of the event, remotely coordinated three sets of field work in Turkey and Greece, ran a mission blog, identified other suitable data sources for damage assessment, and completed remote (secondary) assessment of all damage data collected. The details of all this work can be seen in the mission report (Aktas et al., 2021), which was published in May 2021. The mission timeline is shown in Figure 1.
This paper aims to summarise the main findings of the mission, as well as the key lessons learnt from this experimental reconnaissance strategy with specific emphasis on the 30 October 2020 event, in order to draw conclusions regarding the feasibility of similar approaches in future disasters.
2 Systematic Field Data Collection
For the aim of damage data collection, the field crews used a specialised tool developed by UCL researchers and academics as part of the Learning from Earthquake project (2017–2022, EP/P025641/1). Details regarding this tool as well as the process of recruiting and training the teams to be deployed in the field and the routes they should follow were determined are presented below. The main challenges regarding the data quality as well as how they were addressed are presented with an overall summary of the collected data.
2.1 LfE Mobile App and EEFIT Spatial Data Infrastructure (SDI)
The LfE Mobile App has been developed in response to two needs arising from previous reconnaissance experiences: the need to have consistent data across different missions and the need to have complete data within a mission and within a data collection campaign. The data completeness relates to the information needed to identify a given building as belonging to a typology of known vulnerability, the classification of its state of damage according to agreed damage scales and patterns, and sufficient associated visual evidence (photos, etc.). The LfE Mobile App, available to use both online and offline, is based on a workflow which follows a multi-tiered assessment rationale, dependent on the amount of time the user can spend on site. The data collected is commensurate to this time and gets hierarchically organized so that there is no repetition, whilst guaranteeing that an increasingly detailed level of information is gathered in each successive tier.
The LfE Mobile App has been developed by Putrino, D’Ayala and Ellul, as a customised application of the off-the-shelf DeviceMagic (DM) platform, a form builder that allows to create customisable forms for data collection.
The LfE Mobile App was conceived to work in tandem with a bespoke Spatial Data Infrastructure (SDI) system, also under development within the LfE project, which allows to integrate and reconcile the data collected with the LfE Mobile App with data coming from other sources used during the mission. The SDI is planned to have a web-interface for mission management, data upload and data download, a metadata extractor to reconcile metadata such as coordinates, and a mapper that allows for the display of all the reconciled data as web-maps. More information on the rationale that led to the development of these tools as well as a detailed description of their technical specifications can be found in EEFIT’s upcoming Data Collection Guidelines and Tools. The LfE App can also be used as a stand-alone tool for data collection, as data can be retrieved from the Device Magic Dashboard and sent to a destination of choice.
The LfE Mobile App allows field crews to record a wide range of information on a given building. This includes hazards which affect the building, details on its structural and non-structural characteristics and descriptions of the observed damage. The app is designed to be flexible and, through six different options, allows the surveyor to spend from a minimum of 3–5 min to quickly assess the building externally while in a car, to up to 25 min on-foot, for a detailed and internal assessment of the building. The user chooses how far they wish to carry on with the damage assessment primarily depending on the level of access they have to the property, time limitations and other practical considerations. The user can record necessary information through a combination of drop-down menus, free-text entries, photographic evidence and geotagging. In case of a remotely coordinated or hybrid mission, the recorded information is saved through the SDI and can be accessed by the remote team as the data comes in. This allows to review the data live and provide advice to the on-site teams, on quality and completeness of the collected data, or review and agree specific strategies for data collection.
The first version of the LfE Mobile App was tested during the 2019 EEFIT Albania Earthquake on site mission (Andonov et al., 2020) and the 2020 EEFIT Zagreb Earthquake remote mission, the first of its kind (So et al., 2020; Verrucci et al., 2021). Prior to its use for the 2020 Aegean reconnaissance mission, the app was updated and relaunched as LfE Mobile App Version 2.0, to streamline data capture and include new items specific to the examined event. In particular, the 2020 Aegean event was a complex earthquake and tsunami event, therefore the forms were tailored to easily record building characteristics associated with tsunami vulnerability and be able to record the tsunami damage based on the scale used by Rossetto et al. (2019) for the Sulawesi Earthquake and Tsunami in 2018. Moreover, the hybrid timber-masonry buildings, commonly found in the affected areas, were added in the drop-down menus of the primary structural system so that the teams could easily record them.
2.2 Recruitment and Training of the Field Crews
The travel-restrictions due to the COVID-19 pandemic meant that the United Kingdom researchers could not travel to the affected areas and the only way to collect field data was to recruit local engineers through official or personal networks. In Turkey, two MSc students on earthquake engineering were recruited through contacts with the Earthquake Engineering Research Centre (EERC) at Middle East Technical University Civil Engineering Department. Similarly, a final year student of structural engineering and a practicing structural engineer were recruited through contacts with the University of Patras and community groups in Samos, respectively.
The recruited field crews were extensively trained for on the EEFIT’s Mobile App and ran pilot sessions before the commencement of the field work. Risk assessment protocols were developed to ensure the safety of the field teams. These protocols included detailed guidance against risks associated to working in potentially structurally unstable settings as well as against COVID-19 infection risk. In light of these, the field crews were instructed against entering any building, irrespective of how structurally safe it might appear.
2.3 Itinerary Planning
The aim of the reconnaissance mission was to combine information from several sources. This meant that the fieldwork itinerary was decided based on all relevant information which the EEFIT team could access. In what follows, the rationales that shaped the itineraries followed in Turkey and Greece are detailed.
2.3.1 Turkey Itinerary
In Turkey, the Turkish Ministry of Environment and Urbanization (recently renamed as Ministry of Environment, Urbanization and Climate Change, TMoEUCC) started their extensive damage assessment surveys in the direct aftermath of the event. This activity involved a thorough external and internal examination of the buildings, to categorise damage levels. The data collected was made available along with the photographs depicting damage for each building through an online database (TMoEUCC, 2020). The database allowed search based on district and neighbourhood names, or specific address information and damage levels. It therefore allowed for the total number of surveyed buildings in each district to be understood. Another database, the Izmir Metropolitan Municipality’s GIS Database (Izmir Metropolitan Municipality, 2016), which reports the total number of building and the surface area for each district within Izmir, was used to estimate the building density in each district. This information was used in conjunction with the numbers of surveyed buildings from TMoEUCC database in order to calculate the level of coverage by the TMoEUCC damage assessment survey in each given district. The results are depicted in. It can be seen that the ministry prioritized districts with highest levels of damage with Bornova and Bayraklı, associated with the highest coverage rates (approximately 80%) followed by the Karşıyaka district with a coverage rate of 60%.
With the aim of developing a database complementary to the ministry’s efforts in Izmir city while minimising duplication, and extending our understanding of damage levels and distribution to beyond Izmir, the 5 days itinerary of the field crew in Turkey was planned as follows:
(Day 1) Kuşadası, which is one of the nearest-to-epicentre locations in Turkey and had not been surveyed by the TMoEUCC; (Day 2) Sığacık and Akarca, where tsunami damage was reported but not systematically assessed; (Day 3) Güzelbahçe, Narlıdere, Balçova, Karabağlar and Buca, which are districts in the southern edge of Izmir Bay, covered very little by the TMoEUCC; (Day 4) Konak, which is the densest district in Izmir (Figure 2A) with a high number of public and monumental structures and a diverse building stock, covered little by the TMoEUCC and (Day 5) Çiğli, home to Izmir Atatürk Organised Industrial Area - the largest in the whole region. The crew spent also some time in Bayraklı and Bornova on this last day, to sample a few buildings from these localities.
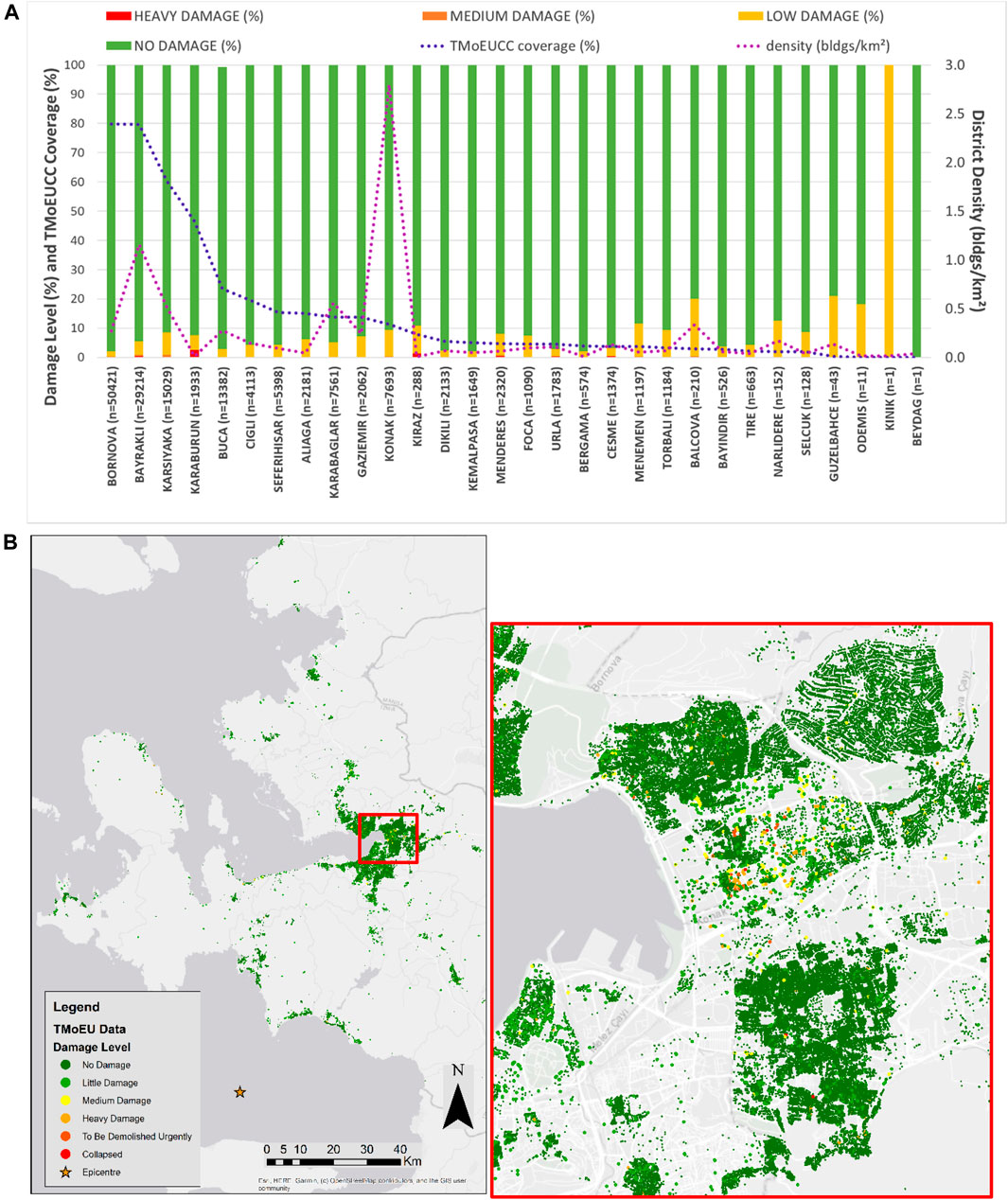
FIGURE 2. (A) District-by-district damage assessment outcome and coverages by TMoEUCC as of end of November 2020. Please note that the damage categories reported here are the ministry’s own (B) Distribution of damage levels from the TMoEUCC database. The image on the right shows the Bayraklı and Bornova area. All this information was used to delineate the mission itinerary to come up with a database complementary to TMoEUCC’s while minimising duplication.
2.3.2 Samos Itinerary
In Samos also, large-scale usability assessments of the building inventory were conducted by the authorities in the direct aftermath of the earthquake. However, the results were not available in the same level of detail as in case of Turkey, and could not be used as part of this reconnaissance study. For this reason, along with the travel and time constraints experienced by the team members in Samos, the focus was mainly on the two main towns (i.e., Karlovasi and Samos city). These regions were of particular interest due to their location as they were also impacted by the tsunami wave. Moreover, a significant part of their historical centre was closed due to heavy earthquake damages. The field crew also visited other villages in the northern part to account for the variability in structural types and maintenance conditions. Those villages are Konteika, Kontakeika, and Kokkari, where serious damage was reported (Kokkari was also impacted by tsunami). Finally, to perform an investigation on the areas that were not severely impacted by the event, the site team also visited the Koumeika village in the central part of the island. Due to lack of time, the rest of the regions were covered through remote assessment using alternative sources of information (see Section 3), in order to develop a general overview on the response of the building stock.
2.4 Implementation and Coordination of the Fieldwork
The field crews were instructed to cover a good cross-section of structural systems, age and occupancy. The affected areas consist of very diverse buildings with different uses, including mid/high-rise RC, industrial precast RC, historic monumental and other large-scale public buildings, various traditional typologies, among others, which we wished to capture through fieldwork. The field crews were also instructed not to focus on damage. Critical to the success of the field work is the collection of an adequate and unbiased sample of buildings which can reflect a reliable picture of the impact of the 2020 Aegean earthquake and tsunami event to the building inventory of the affected area. To address this, the field crews were also instructed to select one or more representative streets of each area they targeted and to survey either every single building in this street or, if they were pressed for time, those at a given interval (e.g., every second or third building).
The daily coordination of the fieldwork was achieved through a morning brief between the team leader and the field crews, and an evening briefing between the whole of remote team and the field crews.
Overall, the field crews collected data from a total of 520 buildings (305 from Turkey and 215 from Samos) as depicted in Figure 3. It can also be noted that the majority of the surveyed buildings were exposed to the earthquake only and the rest to both earthquake and tsunami.
As mentioned before, the LfE mobile app allows a multi-tiered assessment, which depends mainly on the amount of time the user can spend on site. A breakdown of the level of depth of assessments is shown in Table 1. The workflow was designed to collect information starting from what is absolutely indispensable to the mission (such as location and EMS98 damage grade) all the way to valuable but hard/time-consuming-to-collect data. As seen, 70% of all assessments were done at Tier 0 or Tier 1 level, 14% at Tier two and another 15% at Tier three and Tier 4. Importantly, the set of information that differentiate the Tier two from the deeper assessment levels, especially information on crack pattern and full structural system delineation, shown in red in Table 1, was mostly incomplete, along with the information on basement, which was exclusively limited to whether the building has a basement or not. This is because the crews were forced to limit their assessment to the exterior of the buildings. Because of this limitation, the depth of assessment in this study can be said to be limited mainly to Tier 2.

TABLE 1. Key information deemed important for this study and the corresponding form within the LfE tool to record them.
In using the data to draw conclusions, EEFIT team decided that the key building characteristics and damage descriptions described in Table 1 should be available for each building in order to perform some basic statistical analysis. However, due to the way the data were collected not all information were readily available for analysis. For example, if an entry was added while the field crew was driving, only a picture and a quick assessment of the damage state was recorded. To ensure that the information in Table 1 were recorded in a form that could be used to draw overall conclusion on the behaviour and key characteristics of the building inventory, some remote team members assessed the field data remotely for a second time to reduce missing errors, identify and fix misclassification errors, and to ensure the consistency of the answers regarding the identification of the construction typologies, damage mechanisms and the damage levels. When this was available for the location in question, Google Street View was consulted to identify the levels of pre-earthquake damage, which were not accounted for while assigning the damage grades. In case of Turkey, the pre-30 October images from various neighbourhoods, donated to our mission by the Izmir Metropolitan Municipality were also used for the same purpose. The outcomes of this exercise are shown in Figure 4—as seen, apart from some changeover between DG0 and DG1, the outcomes from the field assessment and secondary, remote assessment of the field data are pretty much aligned for moderate to heavy damage cases (i.e., ≥DG2).
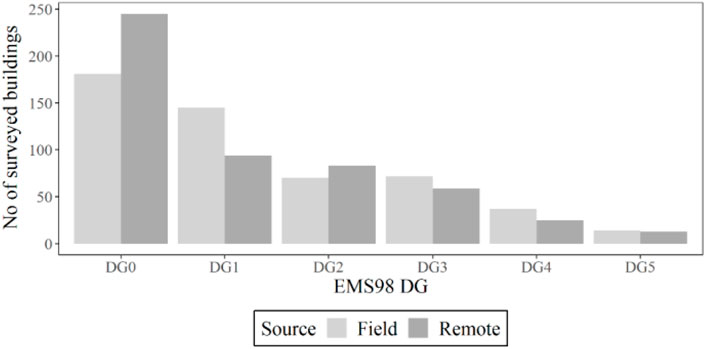
FIGURE 4. Comparative damage levels from field-assessment and remote- (or secondary-) assessment of field data.
3 Alternative Data Sources
The hybrid nature of EEFIT’s reconnaissance mission meant that several team members worked in parallel to fieldwork on identifying alternative sources of information which can be used for remote damage assessment or other purposes to complement the field data and enrich our understanding of the impact of the 2020 Aegean even in the affect area. The teams explored and extracted information from a wide variety of online sources including news outlets, social media and official channels and CCTV imagery. A summary of the key sources explored is depicted Table 2. The team also processed photographs sent by the field crew or other local contacts, without using the app. The data focused mainly on the impact of the earthquake and tsunami on buildings but data of seismotectonic and geotechnical interest were also collected.
As part of this approach, a total of 263 buildings were surveyed remotely using the same forms as used in EEFIT’s mobile app and with a focus on the building characteristic and damage description, from sources depicted in Table 2 and mapped in Figure 5. Most of the remotely surveyed buildings (i.e., 89%) were located in Samos. The main challenge with the remote assessment was that the buildings on which alternative data sources were available were more likely to be severely damaged, or their exact location could not be established. Despite these shortcomings, they added to the understanding of how buildings behaved in the villages in Samos.
4 Brief Evaluation of Strong Ground Motion Recordings
The evaluation of regional seismotectonic context, local geology and local site conditions together with the recorded strong ground motions may give an idea for the spatial variability of earthquake-induced structural damages. The complex tectonism of the region, in which strike-slip and mostly extensional motion exist, makes the Aegean one of the most seismically active areas in the world. The east-west-trending extensional system in the region is formed by the northeastward subduction of the African plate beneath the Aegean plate (Taymaz et al., 2007), within which the MW 6.9 shallow normal faulting (East-West striking and north-dipping) Aegean Sea earthquake occurred in the 30 October 2020 (Akinci et al., 2021; Kiratzi et al., 2021).
Mountainous areas and Neogene sedimentary basins separating them topographically dominate on the island of Samos, which is the closest terrestrial location to the epicentre. In addition to Samos Island, one of the other most affected areas from the main shock are İzmir, situated on Miocene sedimentary units and Plio-Quaternary alluvial deposits. Also, we would like to raise particular attention to the districts of Bayraklı and Bornova, which are settled in the sedimentary basin at the mouth of the İzmir Bay, due to heavy earthquake-induced destructiveness in these areas.
The well-distributed seismic ground motion stations -except for the west of the epicentre-of both the Greek and Turkish networks [the Ministry of Interior Disaster and Emergency Management Presidency (AFAD), Boğaziçi University’s Kandilli Observatory and Earthquake Research Institute (KOERI), National Observatory of Athens Seismic Network (NOA) and ITSAK Strong Ground Motion Network] have yielded a great number of recordings of the main shock. Ground motions have been recorded by 100 stations in a 200-km radius around the epicenter (Figure 6A). The numerous stations to the east and north of the epicentre are mostly (76%) operated by the AFAD while the closest station (GMLD) to the epicentre in the Turkish side belongs to the KOERI network. SMG1 and SAMA of the ITSAK and NOA, respectively, present excellent opportunities to assess strong ground motion characteristics to the near-south of the epicentre, however, the western section of the epicentre is devoid of stations. This circumstance makes it difficult to evaluate the inspected damaged buildings in terms of ground motions, particularly in the west of Samos Island. The stations around Bayraklı-Bornova districts of İzmir, where the mainshock causes the heavy structural damage, on the other hand, make a substantial contribution to the assessments.
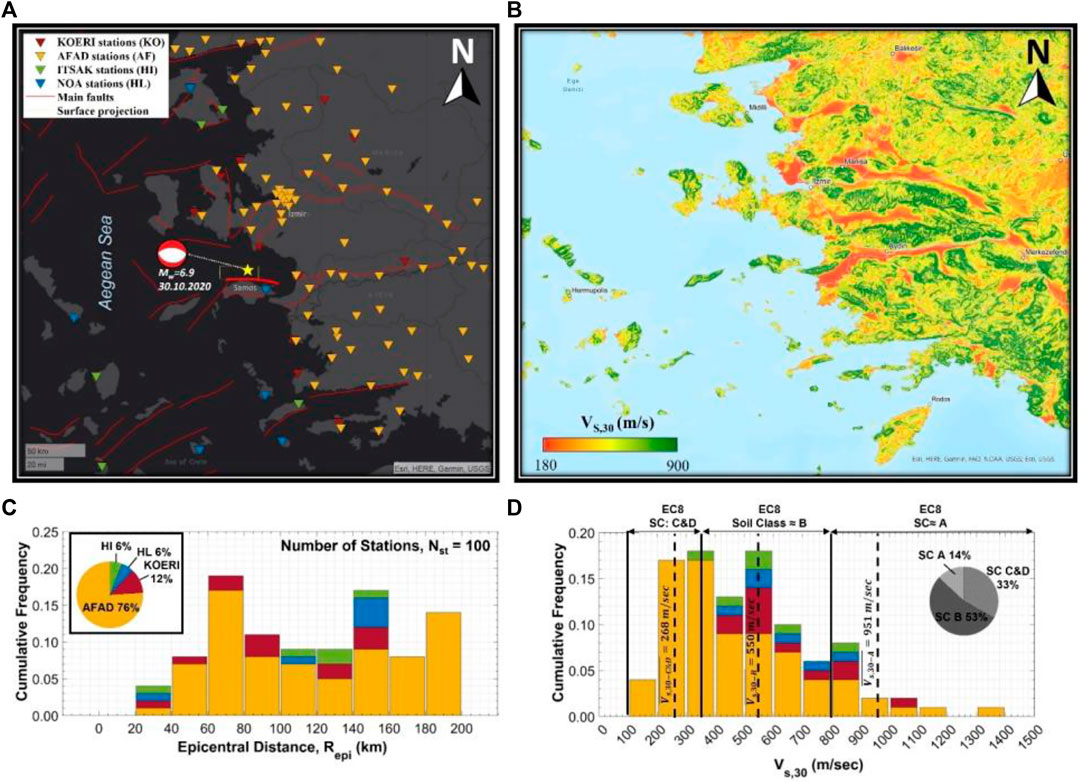
FIGURE 6. (A) Spatial distribution of network-based strong ground motion stations recording the 30.10.2020 MW 6.9 Aegean Sea earthquake within 200 km of epicentral distances, (B) USGS topography-based average shear wave velocity (VS30) distribution of the region, (C) The statistical distribution of epicentral distances of stations (D) The statistical distribution of VS30 values of stations (herein, it is considered the VS30 values provided by the networks instead of USGS topography-based values).
Figure 6 also incorporates the USGS topography–based VS30 map of the region to provide an insight into prevailing soil conditions. Since the networks provide VS30 values for the majority of stations, these network-based VS30 values have been considered in the histogram plot (Figure 6D) except for several stations. Based on the soil classification of EC8, there is a preponderance of the stations (53% of all stations) within soil class B, whose VS30 values range from 360 to 800 m/s. Then, soil classes C and D, which comprise 33% of all stations, come after ground class B. The rest of the stations are deployed on the stiff soil class (VS30 > 800 m/s).
The top panel of Figure 7 illustrates the spatial distribution of peak ground accelerations (PGA) and peak ground velocities (PGV). As expected, the highest peak ground acceleration (PGA) has been measured in NS component of one (SMG1) of two stations in the east of Samos Island, which are the closest territory to the earthquake epicentre, at about 0.24 g (232 cm/s2). This value is slightly smaller than the expected PGA value of the Greece zonation map (2003) for a 475- year return period. In Turkish territories, while a station in Gümüldür produced a maximum PGA (≈0.21 g), the softest soil station in İzmir Basin-the most damaged area—recorded 0.15 g, which is below the expected PGAs of the recent Turkish earthquake hazard map (TSHM, 2018) for the design basis earthquake. However, PGVs of soft soil stations (3513 and 3519) in the İzmir Basin is about as high as those of the nearest stations.
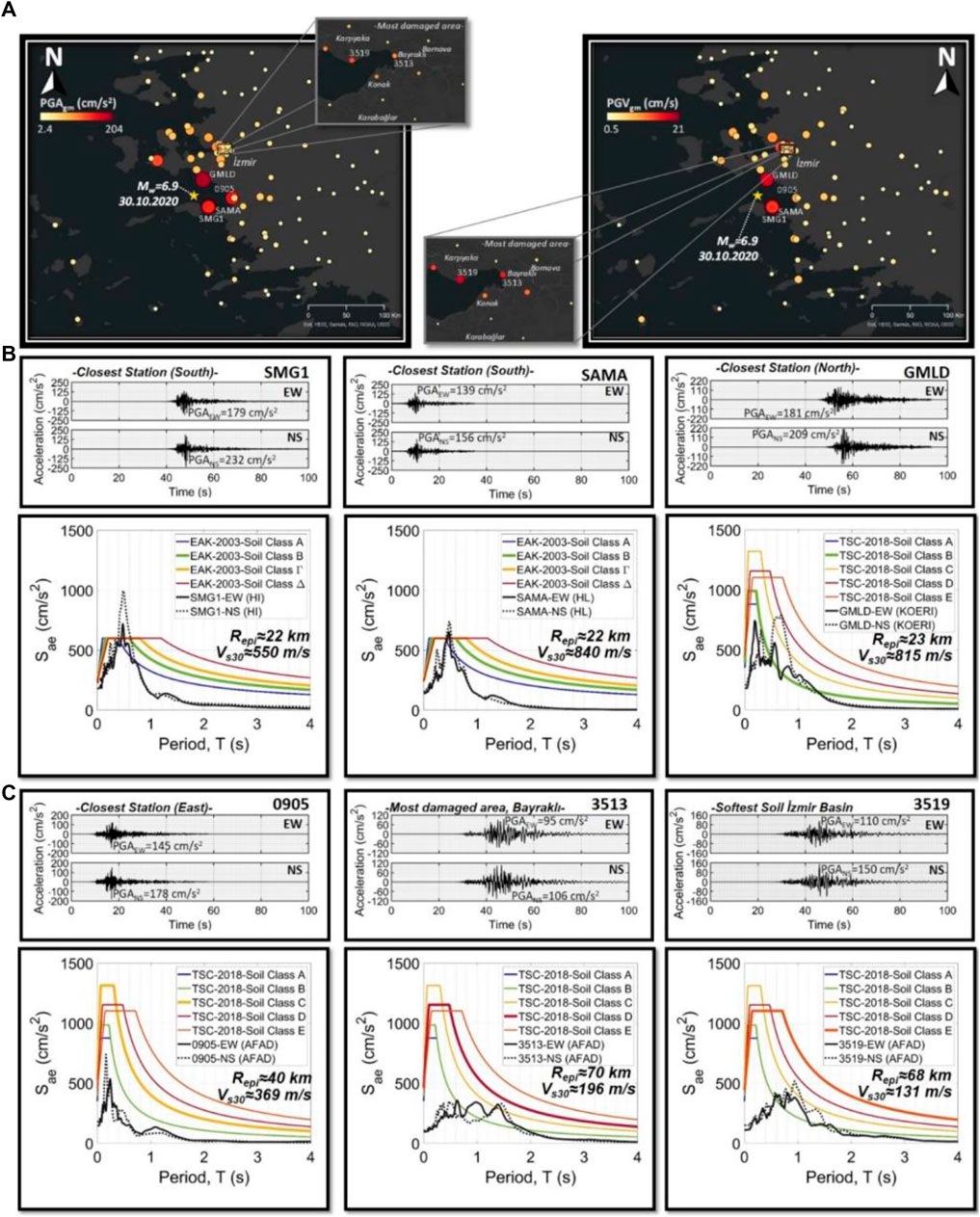
FIGURE 7. (A) Spatial distribution of the observed PGAs and PGVs with a focus view on the significantly damaged region: Bayraklı, (B) Acceleration-time histories and response spectra of two horizontal components belonging to closest stations on South (SMG1 and SAMA) and North (GMLD) of the epicentre, (C) those belonging to the closest station on East (0905) and two stations in Bayrakli (3513 and 3519).
Some prominent recordings are evaluated by comparing them with corresponding design spectra that are generated based on the current national seismic building codes of Turkey and Greece. The seismic demand proposed in the Greek code (EAK, 2003) is exceeded in both horizontal directions of the closest stations (SAMA and SMG1), which are approximately 22 km away from the mainshock epicentre, but more significantly in fault-normal direction, i.e., the North-South (Figure 7). Fault-normal counterparts of each of the two stations result in the highest spectral accelerations, especially in SMG1; the value is almost twice the maximum demand of the design spectrum. If the epicentral distance is neglected since both stations are roughly at the same distance, local soil conditions may govern the response in terms of the capability of amplification; station SAMA operates on a relatively stiffer soil (SC-A in EC8 soil classification) while the other is classified as SC-B based on EC8 (Figure 7). Conversely, the station GMLD, another closest station at 23rd km of the north of the source, is mostly in the design limitations set by the Turkish Seismic Code (TBSC, 2018) in relatively short period range. However, design accelerations levels are exceeded in the period portion of 0.5–1.5 s for SC-B of TBSC-2018 soil classification.
In the aftermath of the earthquake, the most damaged regions are Samos, which is as expected considering the building stock very near to the source, and Bayraklı/Izmir where a significant number of multi-storey RC buildings are either significantly damaged or collapsed. The latter of which necessitates a closer look to enlighten the reasons behind since the theoretical aspect is fulfilled in that the response spectra of two stations (3513 and 3519) in Bayraklı do not drastically exceed the spectral acceleration levels by design spectra of TBSC (2018). However, it should be emphasized herein the flat portion of the response spectrum of 3513 covers longer period range varying from 0.4 to 1.4 s when compared the design spectrum. Despite the lower spectral accelerations than the design demand, the highest damage distribution in the region implies that technical requirements during or after the construction were not fulfilled or were violated (for further discussion see Section 5.1).
5 Building Stocks and Earthquake Damage Observations
The building stocks in the affected areas by the 30 October 2020 Aegean Earthquake are primarily reinforced concrete (RC), masonry, and timber-masonry. The breakdown of these main construction typologies and others (primarily confined masonry and other masonry-concrete hybrids, as well as steel-based construction systems) from our damage assessment activities (only remote assessment of field data) based on location can be found in Figure 8A. As you can see reinforced concrete (RC) is the predominant structural typology in Turkey with 75% (226/302), as opposed to 14% in Samos (31/217). In lack of a systematic building census including construction type information, we are unable to understand the actual portion of RC structures within the whole building stock for the area under consideration in Turkey, however the field observations support that the majority of the buildings are indeed RC. Further our data shows that the RC buildings in Turkey are overwhelmingly mid-/high-rise with 2-storey buildings comprising 16% of all surveyed RC buildings, 3-storey 19%, 4-storey 15%, 5-storey 25% and + 6-storey another 20%, while in Samos low-/mid-rise are more common - 58% of all surveyed RC buildings are 2-storey, and another 29% are 3-/4-storey (Figure 8B). The predominant typology for Greece is masonry with 69% (150/217), which is only 16% of the sample set from Turkey (47/302). The 2011 building census results available for Greece reports the percentage for stone masonry structures for Voreio Aigaio (North Aegean Region inclusive of Samos) as 47.7%, which is very comparable to our estimations (Hellenic Statistical Authority, 2015). Assuming that the values are reflective of Samos specifically, the census however, suggests that we undersampled concrete structures and oversampled timber structures, as it reports 32.1% for the former and 0.2% for the latter, as opposed to our 10 and 8%, respectively (ibid). In both countries, more than 70% of all masonry buildings are low rise traditional typologies, built with local stone or brick and unreinforced (Aktas et al., 2022). The rest has a substantial portion of historic monumental structures, such as public buildings, libraries, churches, mosques, as well as more recent period masonry structures built with hollow clay and concrete blocks, some of which is very low quality. All the timber-masonry buildings are traditionally built hybrid structures with a masonry ground floor and timber framed upper stories, which are common over a wide geographic area, including Turkey and Greece.
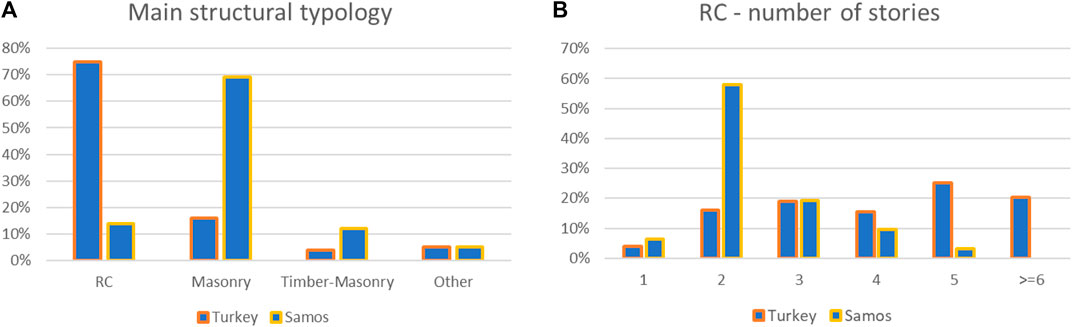
FIGURE 8. (A) Breakdown of main structural typologies based on country (B) Distribution of number of stories in RC structures Turkey and Samos.
The damage classification was made based on EMS-98 (European Macroseismic Scale), where DG0 delineates no damage whatsoever, DG1 no structural damage and negligible to slight damage to non-structural components (such as hairline cracks in all primary structural typologies, limited to mortar joints or plaster in masonry), DG2 moderate damage (including cracks, plaster fall and partial collapse of non-structural components such as chimneys), DG3 substantial to heavy damage (including large and extensive cracking, failure of individual non-structural components), DG4 very heavy damage (including serious failure of walls and partial structural failure of roofs and floors) and DG5 total collapse or near total collapse (ESC, 1998). While the no-/low-damage cases are generally the highest for any given primary structural system, gradually decreasing in number with increasing damage levels, the masonry and timber-masonry buildings in Samos demonstrate a spike at moderate damage levels (Figure 9). As mentioned above, these are predominantly traditional buildings, often old and in poor condition, which is considered to be the main reason behind elevated portion of moderate-to-high damage cases. Further, while the epicentral distance does not appear to be a relevant parameter to explain diverse damage levels (Figure 10A), the PGAmax values are slightly higher for the soft soil station on the east of Samos than for Turkey must be another reason for this discrepancy. Also, although there is no recorded PGA in the west of Samos, the proximity of the surface projection of the fault plane to Samos than Turkey can be associated with these moderate-to-high damage cases (Figures 6A, 10B, see Malcioglu et al., 2022 for further details). Despite the few dramatic collapse/heavy damage cases which have been the most memorable outcome of the 30 October event, the RC seems to be the primary structural system proportionally with the highest number of no-/low-damage cases.
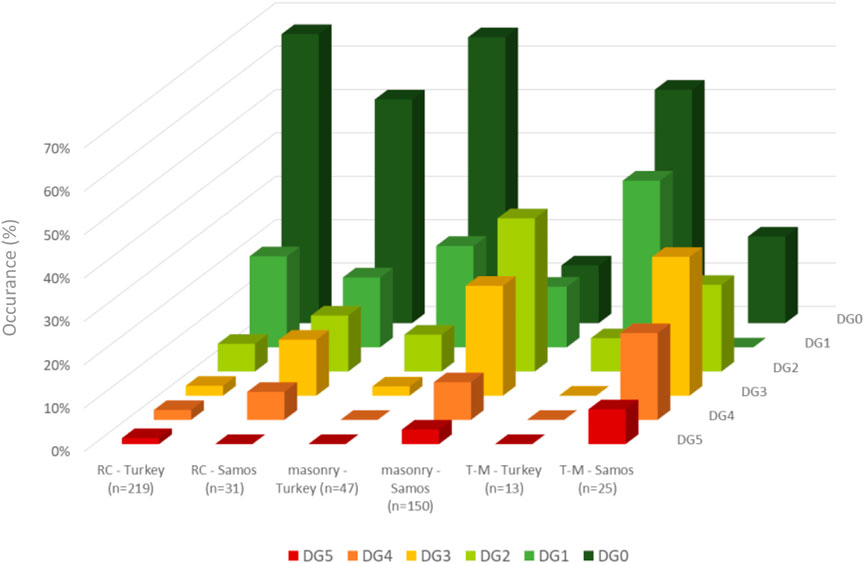
FIGURE 9. Damage level distribution based on location and primary structural system (T-M stands for timber-masonry).
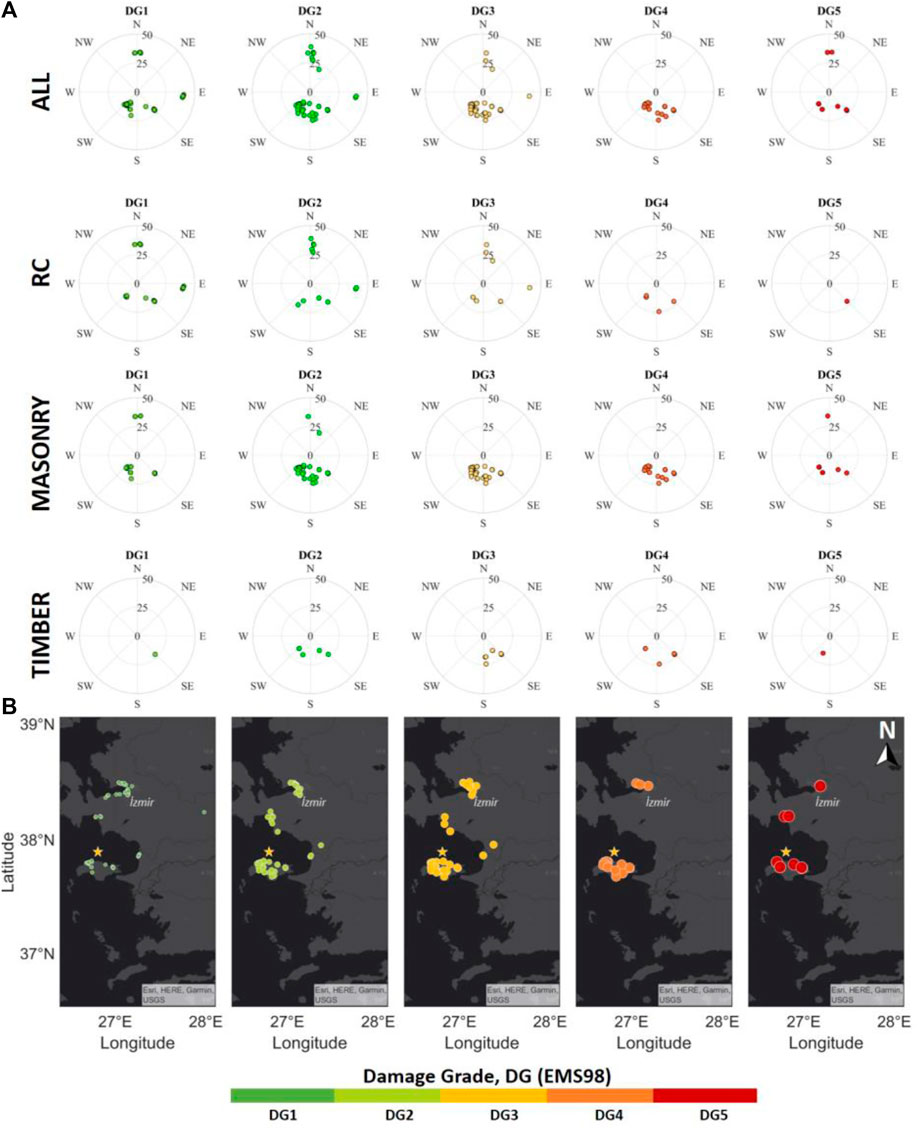
FIGURE 10. (A) Epicentral distance for different primary structural systems and damage levels (>DG1) and (B) The distribution of the damage levels (>DG1) of our surveyed buildings.
The performance of each of these three main primary structural systems is discussed in more detail in the subsequent sections. Please note that due to the failure bias intrinsic to the alternative data sources we used in this mission, the reported statistics are based on the field-data alone.
5.1 Reinforced Concrete Structures
This first Greek seismic code was developed in 1959 with no earthquake resistance considerations, and then updated in 1984 and 1995, to introduce ductility requirements and probabilistic seismic design concept, respectively. Through the integration of European pre-Standard in 2000 (EAK-2000), dynamic spectral method along with the behaviour factor depending on the ductility as well as the capacity design approach were started to be used. The EAK-2000 was reviewed in 2003 to include a new seismic hazard map, dividing the country into three seismic zones with a peak ground acceleration (PGA) ranging from 0.16 to 0.36 g (TR = 475 years) depending on soil conditions. The most recent National Codes are used in parallel with the EC8 8 (Eurocode, 2004) since 2011.
The age distribution of Samos’s RC building stock according to the EL STAT (2011) is given in Figure 11. This shows that a majority of the buildings was designed and constructed within the 1959–1985 bracket, where the Royal Decree on the Seismic Code for Building Structures (Gazette, 1959), i.e., the first Greek seismic code was in effect.
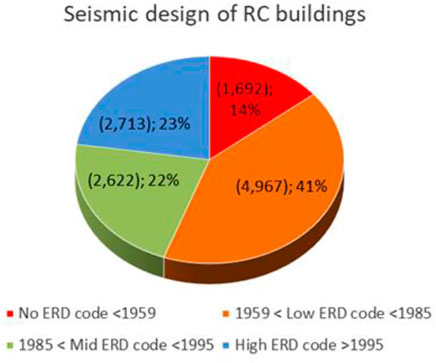
FIGURE 11. Age distribution of RC buildings in Samos in relation to the design code (ERD stands for Earthquake Resistant Design).
For Turkey, on the other hand, following the unofficial zonation map developed in 1932 based on Sieberg’s studies (Özmen, 2012), building codes started formally to consider the seismic design element in 1940—this includes a seismic zonation map developed in 1942 following the 1939 Erzincan Earthquake (Sezen et al., 2001; Ilki and Celep, 2012), which was updated in 1945 and again in 1947 (Soyluk and Harmankaya, 2012). Further updates have been introduced since then to arrive at the current Turkish Earthquake Code. The design spectrum concept was introduced in 1968. The 1975 version included ductile design concept and inelastic design spectrum, enforcing special reinforcement solutions and detailing. While in 1996 the probabilistic seismic hazard analysis became integral to the code. The code was then reviewed and amended following the Marmara Earthquake in 1999, and then again in 2007 and 2019, where a georeferenced contour map was introduced to calculate ground motion levels in conjunction with the soil conditions of the site, and earthquake design loads (Sucuoğlu, 2018).
However, we understand from Elyamac and Erdogan (2005), Ilki and Celep (2012) that these improvements in the code had little repercussion in the construction practice, with insufficient lateral load capacity, stiffness, ductility and strength endemic within the majority of the building stock, as also voiced by many others following significant earthquakes including the devastating Marmara Earthquake in 1999 (Bruneau, 2002; Erdil, 2017). Unfortunately, we do not have information on the design and construction dates of the structures we damage-assessed in this mission to put observed performance in perspective, however, it was widely discussed that the buildings that dramatically collapsed in Bayraklı and Bornova districts were from the 1975–1998 bracket, which were not designed or built up to the 1975 earthquake code: concrete quality was poor, lateral reinforcement was missing and beam-column connections were not detailed properly. Further large openings at the ground levels and alterations in the load-bearing structures to open space for commercial use was a critical irregularity common to buildings with extensive damage (Demirci et al., 2021). In conjunction with the soft soil conditions in Bayraklı area, which led to a ground motion amplification, all these design and construction issues played an important role in the damage. The Doğanlar (DG5 picture in Table 3), Karagül and Rıza Bey apartment buildings which collapsed during the event, killing around 50 people and receiving a lot of repercussions in the media, are good examples to demonstrate the extent of lack of code compliance, inefficient communication between institutions, and poor operable quality assurance regulations: Bayraklı Municipality Earthquake Study Center reported in 2008 that all these buildings were found to suffer from door jams, ground floor level irregularities and distortions in the balcony structures due to liquefaction and seismic activity. Another report in 2012 on Rıza Bey apartment building indicated inadequate rebar detailing, moisture induced material degradation, heavy overhangs, flat reinforcement bars and poor concrete quality, requesting a thorough survey. However, in both cases, it was claimed that the ministry was not informed about this (Gurcaner and Torlak, 2020). Other interesting examples are the Yılmaz Erbek Apartments (two buildings of the same design) and Barış building complex (four buildings of the same design), where respectively only one and two buildings collapsed, killing 33 people between them. The difference between the performance of individual buildings here was attributed to the commercial use of the ground floor (which allegedly removed some of the columns), but more interestingly to the use of different contractors during the construction stage as well as for the later modifications.
In addition to the widened openings at the ground floor or non-engineered intervention on the load bearing system, which was common to almost all collapsed buildings in Bayraklı, Turkey, some of the other common irregularities we observe in the reinforced concrete structures in both Turkey and Samos include changes in stiffness due to structures attached to the main buildings and plan and elevation irregularities (Figure 12). Examples of the damage cases at each class can be seen in Table 3. As can be seen, the damage mechanisms widely range from pancake collapse to shear failure of piers and spandrels, infill wall failure and column-beam failure. A few cases with minor pounding damage were also observed. Despite that, overall, RC structures performed well in this event with 88 and 67% of our surveyed buildings categorised as DG0 or DG1 in Turkey and Samos, respectively.
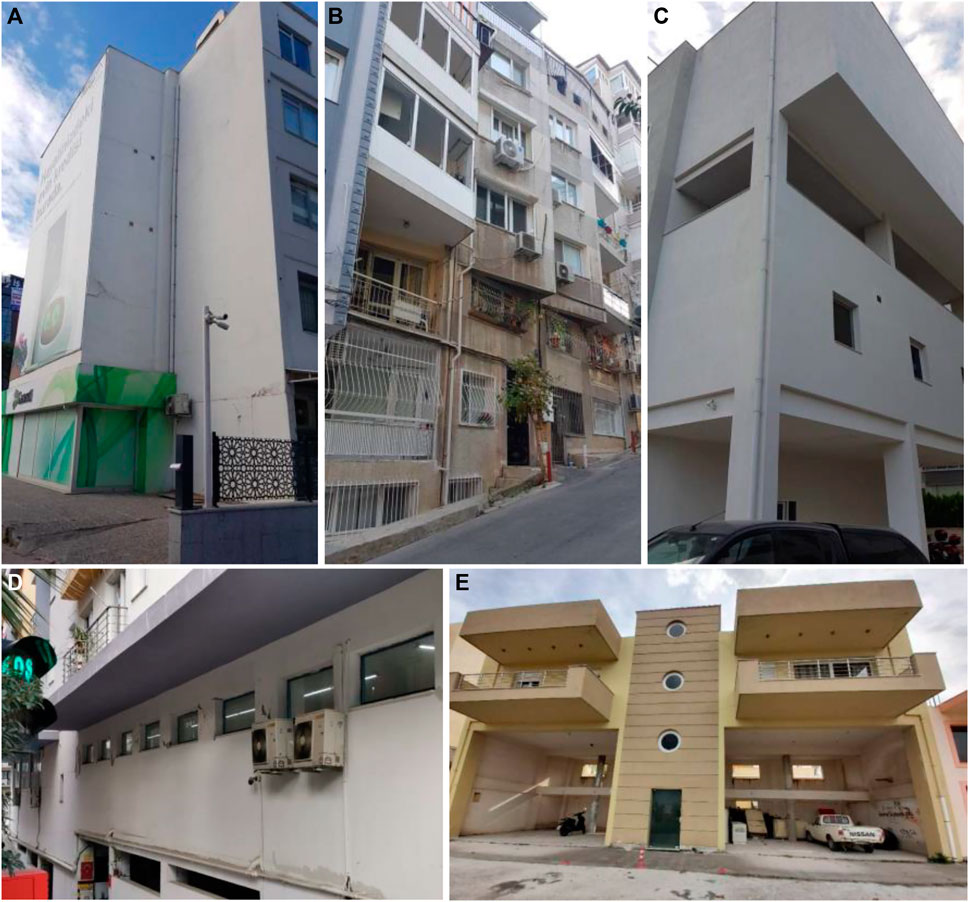
FIGURE 12. Examples of common irregularities in RC buildings [(A): Changes in stiffness due to structures attached to the main building, (B,C): Elevation and plan irregularities, (D): short columns, (E): plan and elevation irregularity, and large openings at the ground floor].
5.2 Masonry Structures
As mentioned in Section 4, masonry structures encountered during this mission include traditional unreinforced stone masonry buildings (see Aktas et al., 2022), public buildings of monumental or humbler scales and more recent masonry buildings with concrete or hollow terracotta blocks (Figure 13). The common irregularities observed in these structures include elevation or plan irregularities, significant variations in the storey heights and changes in stiffness due to structures attached to the main building, which also pose damage risk due to pounding. The damage mechanisms vary from various levels of in-plane and out-of-plane failure, which sometimes propagate partial or total collapse (Table 4). Most of these buildings are old and demonstrate a certain level of pre-earthquake damage/corrosion, which the team did account for while assigning the damage classes. As seen in Figure 9, masonry structures in Samos performed proportionally poorer compared to the ones in Turkey, with around 12% categorised as DG4 and DG5, and another 25% DG3. This can be attributed to both lower PGA values in the latter (Figure 10) and to less maintenance and more degradation in the Samos building stock.
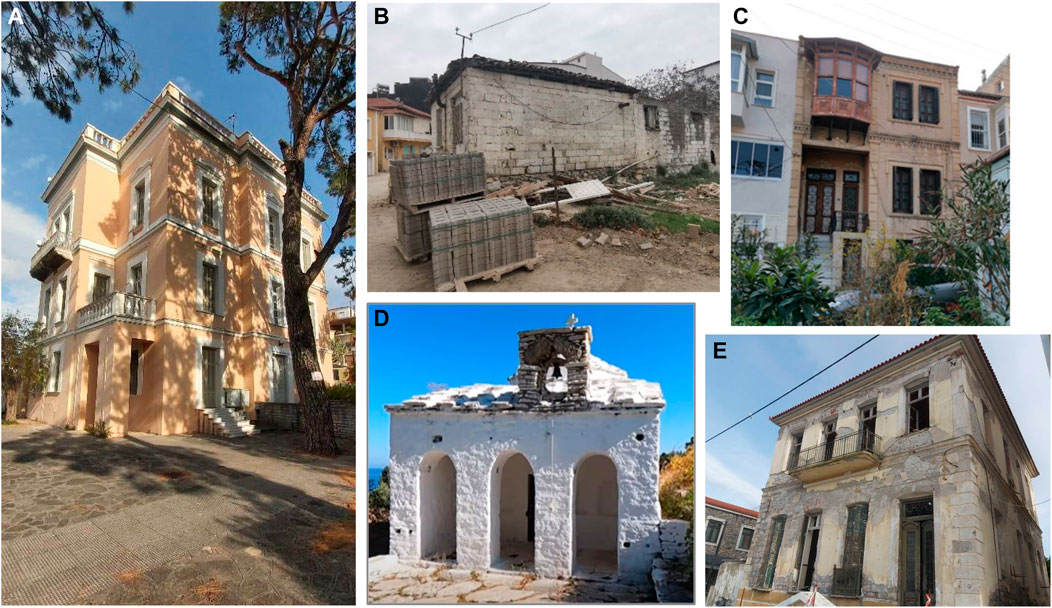
FIGURE 13. Examples of masonry building stock in the affected areas [(A,D,E) in Samos, (B,C) in Izmir].
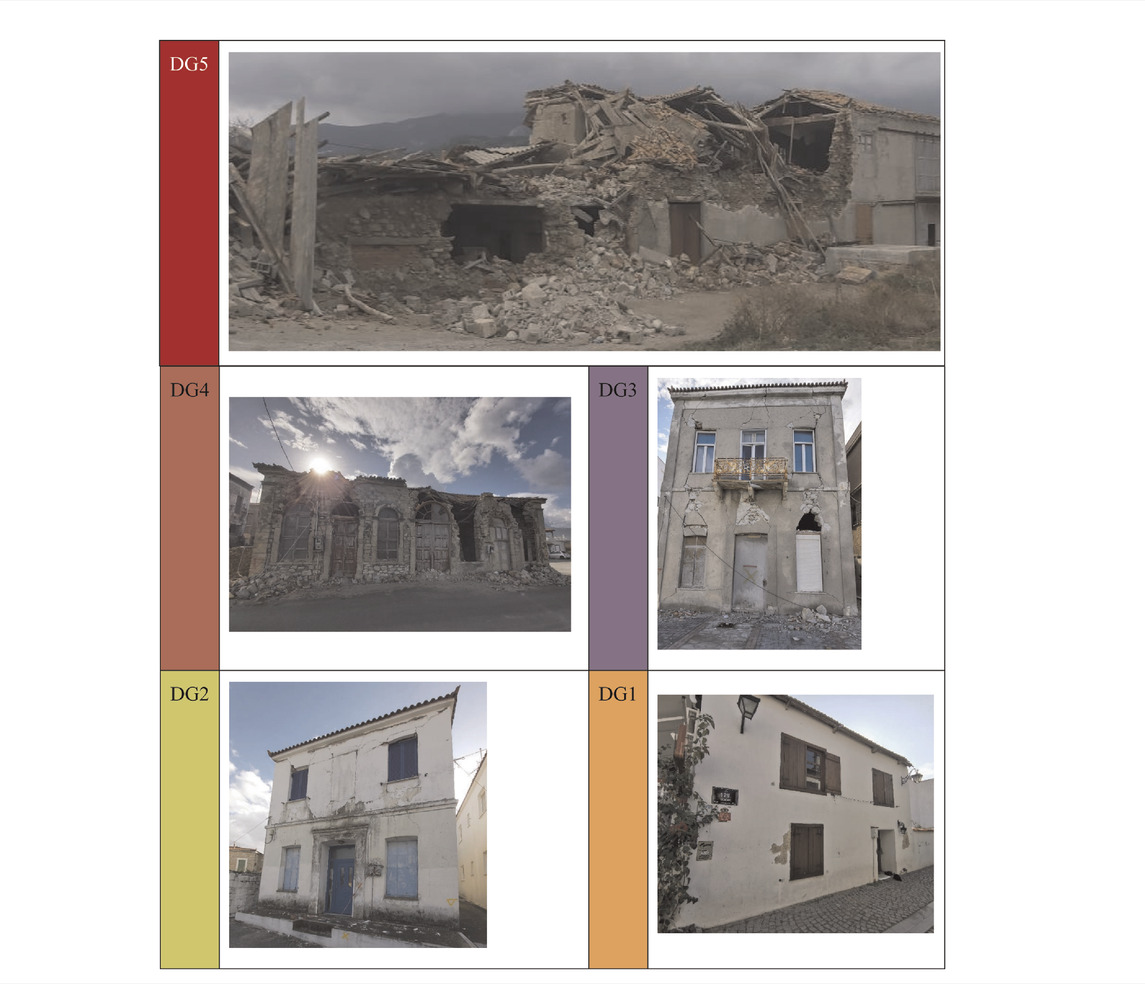
TABLE 4. Examples of masonry structures at different damage classes (DG1 in Sığacık, DG2-5 in Samos).
Masonry structures included a significant amount of larger scale or monumental historic buildings, which in general performed well in the 30 October 2020 Aegean Earthquake. Among our surveyed buildings were historic churches (e.g., Figures 14A,E,F) and monasteries, public buildings (Figure 14B), aqueducts (Figure 14C), industrial heritage buildings (Figure 14D), mosques (e.g., Figure 14G), large public buildings (schools, city halls, libraries, train stations), and hamams (public bathhouses) (Figure 14). The only few damage cases we recorded were in Samos, including the Koimisi Theotokou in Karlovasi and Panaghia Vrontiani in Konteika, which received damage to their domed superstructure and Agios Nikolaos in Kokkari and some small chapels, which suffered from serios in-plane failure. Many others in Samos and all surveyed historic structures in Turkey were categorised as DG0 and DG1. Even tall and slender structures, including the chimney of the historic gasworks (Figure 14D), minarets, the Izmir’s landmark clock tower (Saat Kulesi) (Figure 14H) and did not suffer any damage. The famous “Asansör” in Konak district, Izmir (Figure 14I), which is a 58 m tall elevator built in 1907 to provide access through a cliff, was also untouched by the event.
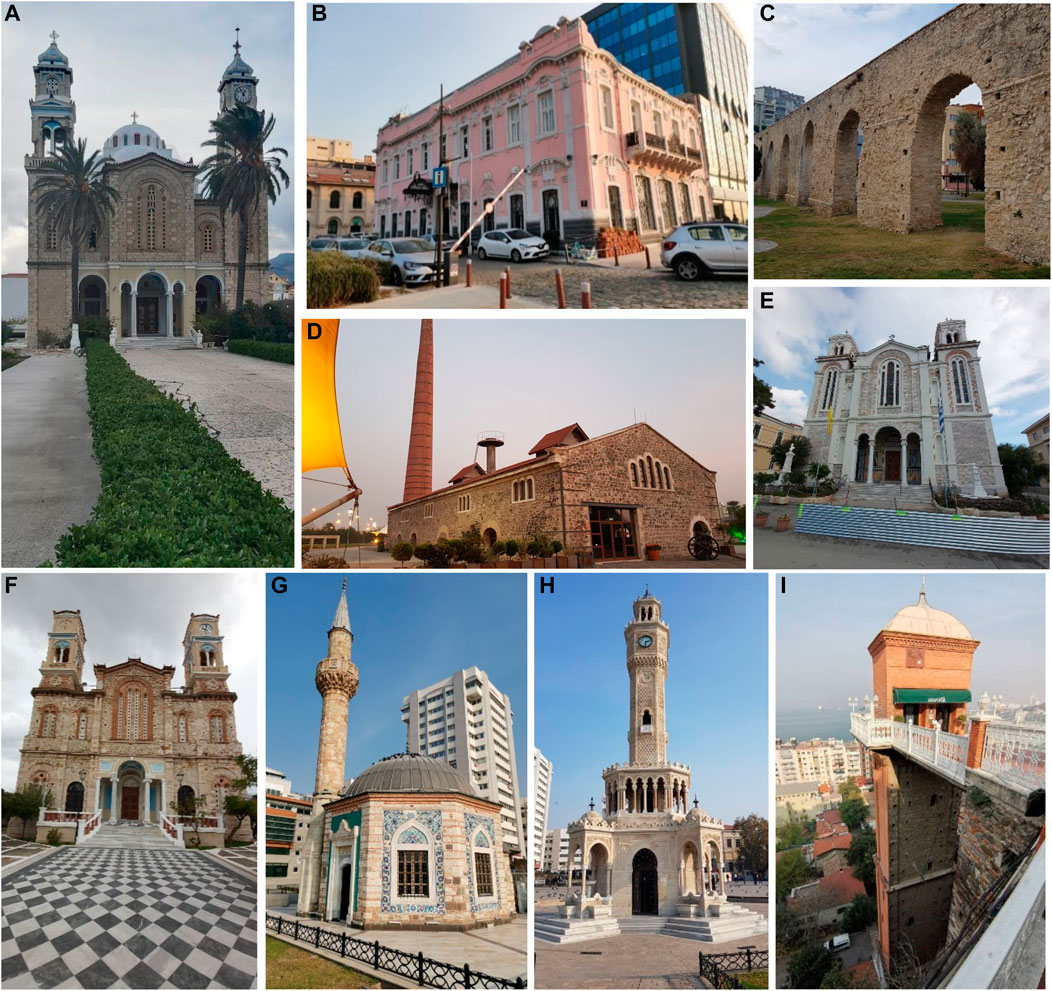
FIGURE 14. Historic masonry buildings in the affected areas: (A) Agios Nikolaos in Karlovasi, Samos (B) Izmir Culture and Tourism Building, Izmir (C)Aqueducts, Izmir (D) Gasworks, Izmir, Turkey (E) Saint Spyridon Church, Samos (F) Church of Presentation of Mary, Samos (G) Konak (Yalı) Mosque, Izmir (H) Izmir clock tower, Izmir (I) Asansör, Izmir.
5.3 Timber-Masonry Structures
Timber-masonry structures are a traditional typology common to Turkey, Greece and most of the Balkans, and are characterised by a masonry ground floor and timber framed upper floors. The timber frames can be infilled with stone or brickwork, or cladded by laths of various widths. Similarly, masonry ground floors show a rich variation in terms of the construction techniques adopted (Aktas, 2017). The affected areas on both sides of the Aegean Sea have good stocks of this hybrid typology (for a more extensive discussion of their performance at the 30 October event, see Aktas et al., 2022). The local examples are primarily of a stone masonry base, and either stonework infilled or bağdadi cladded (3–5 cm thick laths nailed onto the timber frames) upper floors (Figure 15). Damage in these buildings are most commonly due to masonry failure, either at the ground floor level or of the masonry infill, if used. Failure at the ground floor or of non-load-bearing masonry elements might propagate damage in timber framing. While a wide range of reconnaissance activities following significant events in those areas where this building typology is observed suggest that these buildings are intrinsically capable of demonstrating good seismic performance, mainly owed to high the energy dissipation capacity of nailed connections and light weight (Aktas et al., 2014; Aktas and Turer, 2016), we also understand that quality of connections between individual timber frames and those between masonry base and timber skeleton is critical for an overall desirable performance of these structures under earthquake loading. We observe a wide range of damage levels and mechanisms for these buildings following the 30 October Aegean earthquake (Table 5). Similarly for this typology of buildings, the sample set from Samos demonstrated more damage than that from Turkey (with 28% categorised as DG4 and DG5, and an additional 32% DG3), due to a clearly higher level of accumulated material and structural damage and higher levels of pga in the former (Figures 9, 10). For more information on the behaviour of these structures during the 30 October event, and their fragility assessment in light of observations made in our mission, see Aktas et al. (2022).
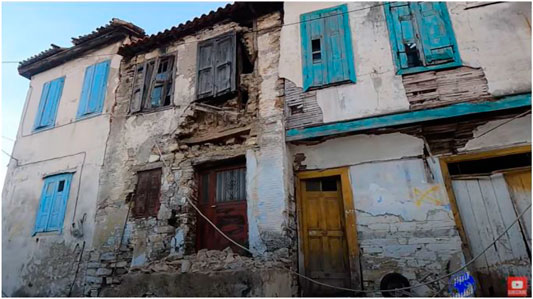
FIGURE 15. Examples of stone infilled (building in the middle) and bağdadi cladded (building on the right) timber-masonry homes (in Samos, picture from Mikres Diadromes Samou, 2020).
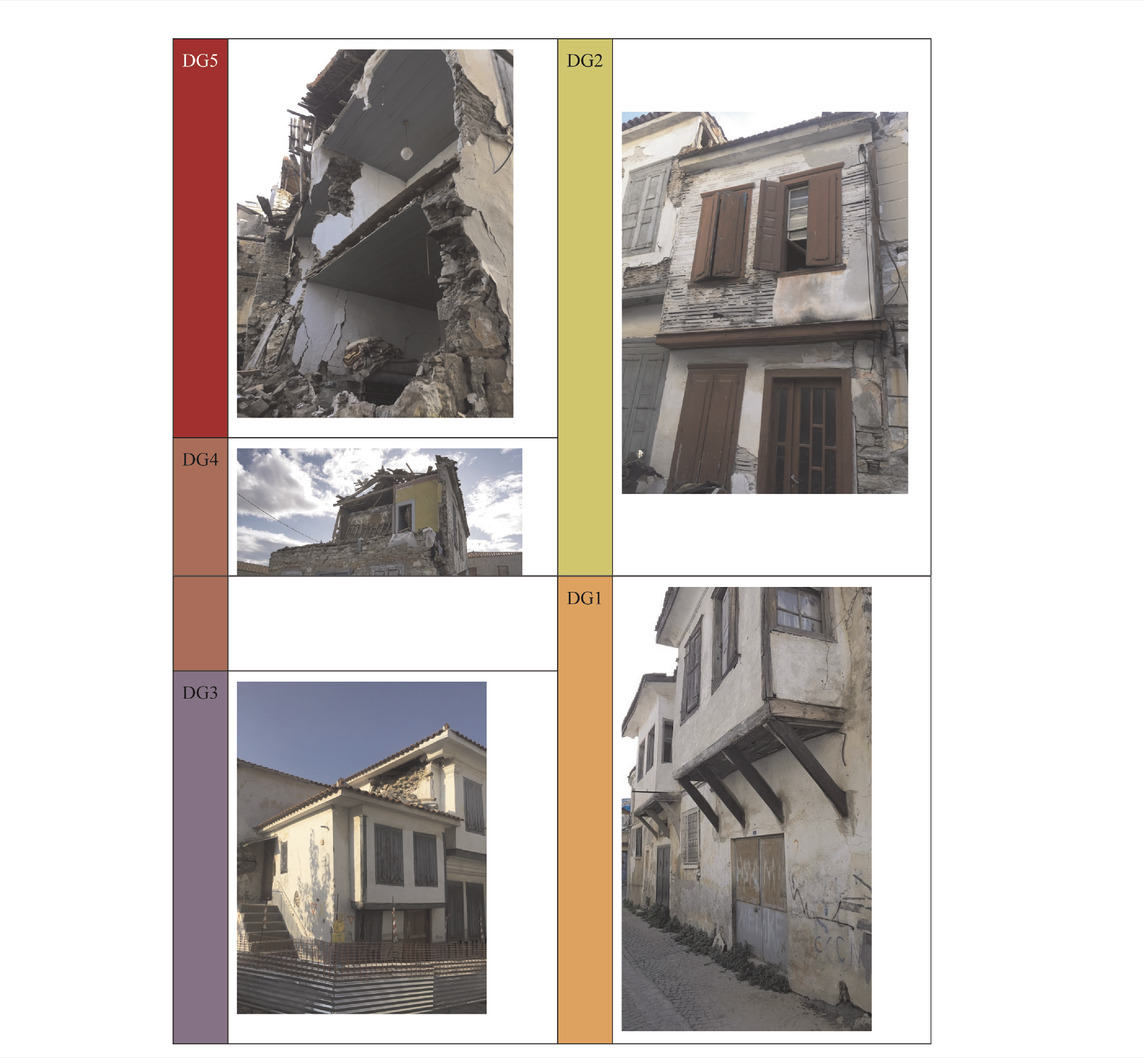
TABLE 5. Examples of timber-masonry structures at different damage classes (DG1 in Kuşadası, DG2-5 in Samos).
5.4 Other Structures
In addition to these well-defined structural typologies, the field investigation identified different RC-masonry-steel hybrid structures in the affected areas (including the very few well-established confined masonry structures), which however did not demonstrate much damage, or a common pattern in damage when they did.
6 Impact of Tsunami on Buildings and Infrastructure
The 30 October 2020 Aegean earthquake was accompanied by a tsunami, which is not an uncommon phenomenon in the region (Figure 16A). The tsunami waves reached up to 2.0 m in this particular event (Figure 16B). Therefore, the EEFIT mission also systematically investigated the tsunamic impact on buildings and infrastructure, through a set of questions embedded in the data acquisition system specifically to reflect typical damage mechanisms due to tsunami hydrodynamic effects on buildings. These being: the number and types of openings, particularly at ground floor level; the presence of a basement; assessment of surroundings, including perimeter walls and presence of debris; details of foundation type and damage (if that is visible); and evidence of water marks on the building. Some of these attributes were included in the damage assessment form developed in the 2018 EEFIT mission in Palu (Rossetto et al., 2019), which has been ad opted for the damage assessment carried out in this mission. By the time our field crews visited site however, it was already 1 month after the event, and all debris was cleared and no substantial structural and non-structural damage was found despite significant inundation in some localities. In certain cases, water marks were visible on buildings (Figure 17).
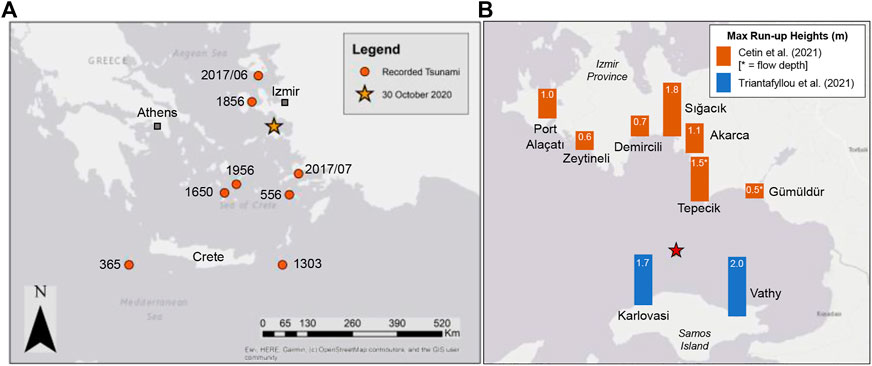
FIGURE 16. (A) Recorded major tsunami events in the Aegean Sea (B) Measurements of maximum tsunami run-up heights in m for the most-affected locations by the tsunami along the coast of Izmir Province and Samos Island, according to Cetin et al. (2021) and Triantafyllou et al. (2021).
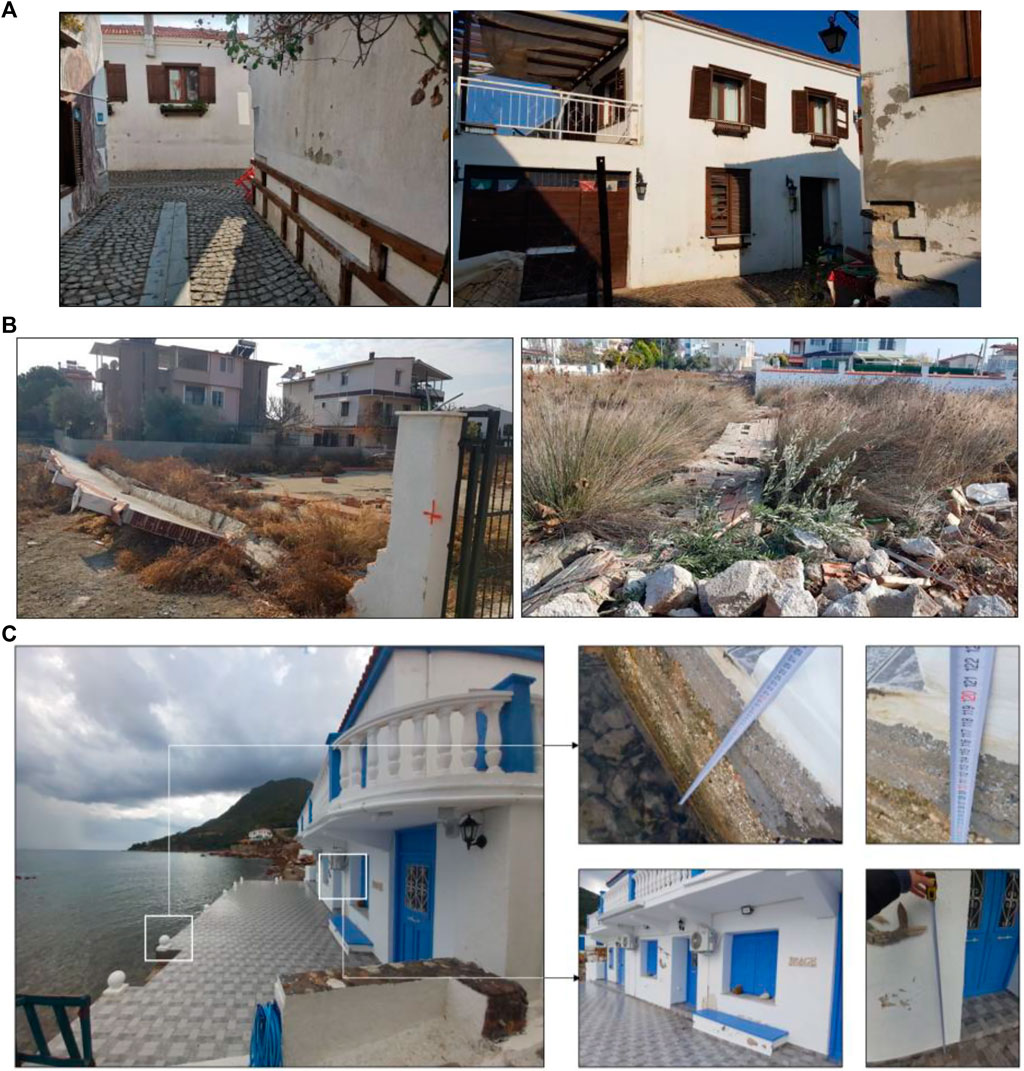
FIGURE 17. (A) Water marks on buildings in Sığacık, Seferihisar, (B) perimeter wall overturning due to tsunami hydrodynamic effects in Sığacık (C) water marks on building at Agios Nikolaos beach in Samos.
7 Discussion and Conclusion
The EEFIT Mission to the 30 October 2020 Aegean Sea Earthquake and Tsunami showed that the building stocks in both countries generally performed well, with the exception of a few RC structures exclusively in Turkey, which suffered from a high degree of damage despite the lower spectral accelerations than the design demand–a situation which can be attributed to a very unique combination of non-compliant design, poor construction practices, and in certain cases, post-occupancy amendments, flagging a lack of more operational quality assurance protocols. Additionally, the traditional building stock in Samos received mostly moderate- and high-damage, as a result of both the comparatively poor upkeep of these buildings and slightly higher recorded pga values here than in Turkey. It is noteworthy to state that the recorded pga values in Greece were also lower expected demand values for a 475-years return period, however the scarcity in the deployed stations in the Island of Samos hampers the reliable evaluation through studying accelerograms. If there were enough strong ground motion stations on Samos, it would probably record higher pgas in the central and north-western parts due to the proximity of the northern edge of Samos to the surface projection of the fault.
Importantly, one of the limitations of this study is that all buildings were assessed from outside due to COVID-19 related lockdown conditions, and the damage levels might have been underestimated.
This was a hybrid reconnaissance activity bringing together the field and remote survey strategies. The team partnered up with local organisations to recruit, train and deploy field crews for an in-situ examination of damage in diverse building stocks, which proved an efficient and speedy way of accessing the site under the COVID-19 pandemic conditions where travelling was not an option for the team. This strategy also brought ease in the overall damage assessment process due to the knowledge of the local field crews of the native languages, relevant national authorities and building stocks, and can be adopted in the future with diligent coordination by the remote team even when there are no extraordinary circumstances hampering international travels.
The mission also explored alternative data sources to gain an understanding of the extent and nature of the damage in buildings and infrastructures to assess their usability. Based on the experience from 30 October event it can be concluded that data availability and content for a remote assessment of the impact of the event on buildings and other structures is strongly country dependent. This may be down to different cultures of engaging with social media in a given context, or how informed citizens are regarding the disaster risk. In the case of the Aegean Mission we were able to find a higher amount of data from a wider set of sources for Greece than for Turkey thanks to a few comprehensive data sources on Youtube and Facebook. Another important factor governing the availability of data, representative of the overall damage levels, seems to be how dispersed damage is. In the case of localised dramatic damage (Turkey), the damage bias in remote data sources is much higher than when the damage levels are more even and more scattered across the affected area (Samos), making it harder to gain a reliable understanding of the damage levels overall within the affected areas.
The EEFIT mission to the 30 October 2020 Aegean Sea Earthquake and Tsunami showed that while relying only on remote investigation methods does not (yet) emerge as an ideal way forward, the hybrid model offers many critical advantages, which should be exploited in the future events.
Data Availability Statement
The datasets presented in this article are not readily available because of ongoing work, however can be made available at an aggregated level upon reasonable request. Requests to access the datasets should be directed to YA, eS5ha3Rhc0B1Y2wuYWMudWs=.
Author Contributions
Conceptualization, YA; writing–original draft preparation, YA and II; writing-review and editing, YA, II, FM, JB, and DD; data collection, AK, PD, ME, and EÇ; data analysis, all; methodology, YA; funding acquisition: YA and SW. All authors have read and agreed to the published version of the manuscript.
Funding
The EPSRC-funded Learning from Earthquake (LfE) Building Resilient Communities Through Earthquake Reconnaissance, Response and Recovery (EP/P025641/1) for supporting this activity.
Conflict of Interest
Author AK was co-funded by EPSRC and ARUP. Author MA was employed by the Mott Macdonald. Author DC was employed by the Atkins. Author MF was employed by the Arup.
The remaining authors declare that the research was conducted in the absence of any commercial or financial relationships that could be construed as a potential conflict of interest.
Publisher’s Note
All claims expressed in this article are solely those of the authors and do not necessarily represent those of their affiliated organizations, or those of the publisher, the editors and the reviewers. Any product that may be evaluated in this article, or claim that may be made by its manufacturer, is not guaranteed or endorsed by the publisher.
Acknowledgments
This study was carried out as an extension to the EEFIT Mission to 30 October 2020 Aegean Earthquake and Tsunami, and the authors are grateful to EEFIT and the EPSRC-funded Learning from Earthquake (LfE) Building Resilient Communities Through Earthquake Reconnaissance, Response and Recovery (EP/P025641/1) for supporting this activity. All pictures are EEFIT Aegean Mission Team’s, unless otherwise stated. We extend thanks to the Mikres Diadromes Youtube channel for their permission to use their videos for our study. We extend our gratitude to Prof Ahmet Yakut from the Earthquake Engineering Research Centre (EERC) at Middle East Technical University Civil Engineering Department for their help in the recruitment of Turkey field crew. We cordially thank our field crews in Turkey and Greece. Finally, we are indebted to the communities in the affected areas in both countries for their help and support during the data collection.
References
Akinci, A., Cheloni, D., and Dindar, A. A. (2021). The 30 October 2020, M7. 0 Samos Island (Eastern Aegean Sea) Earthquake: Effects of Source Rupture, Path and Local-Site Conditions on the Observed and Simulated Ground Motions. Bull. Earthq. Eng. 19, 1–27. doi:10.1007/s10518-021-01146-5
Aktas, Y. D., Akyuz, U., Turer, A., Erdil, B., and Sahin Guchan, N. (2014). Seismic Resistance Evaluation of Traditional Ottoman Timber-Frame Hımış Houses: Frame Loadings and Material Tests. Earthq. Spectra 30 (4), 1711–1732. doi:10.1193/011412EQS011M
Aktas, Y. D., Ioannou, I., Malcioglu, F. S., Vatteri, A. P., Kontoe, M., Donmez, K., et al. (2022). Traditional Structures in Turkey and Greece in 30 October 2020 Aegean Sea Earthquake: Field Observations and Empirical Fragility Assessments. Front. Built Environ.
Aktas, Y. D., O'Kane, A., Ozden, A. T., Vatteri, A. P., Durmaz, B., Kazantzidou-Firtinidou, D., et al. (2021). The Aegean Earthquake and Tsunami of 30 October 2020. London: EEFIT IStructE.
Aktas, Y. D. (2017). Seismic Resistance of Traditional Timber-Frame Hımış Structures in Turkey: a Brief Overview. Int. Wood Prod. J. 8 (Suppl. 1), 21–28. doi:10.1080/20426445.2016.1273683
Aktas, Y. D., and Turer, A. (2016). Seismic Performance Evaluation of Traditional Timber Hımış Frames: Capacity Spectrum Method Based Assessment. Bull. Earthq. Eng. 14 (11), 3175–3194. doi:10.1007/s10518-016-9943-2
Andonov, A., Andreev, S., Freddi, F., F GrecoGentile, R., Novelli, V., and Veliu, E. (2020). The Mw6.4 Albania Earthquake on the 26th November 2019 - A Field Report by EEFIT. London: EEFIT.
Bruneau, M. (2002). Building Damage from the Marmara, Turkey Earthquake of August 17, 1999. J. Seismol. 6, 357–377. doi:10.1023/a:1020035425531
Cetin, K. O., Mylonakis, G., Sextos, A., and Stewart, J. P. (2020). Seismological and Engineering Effects of the M 7.0 Samos Island (Aegean Sea) Earthquake. HAEE 2020/02, Earthquake Engineering Assoc of Turkey, Earthquake Foundstion of Turkey, EERI, GEER-069. doi:10.18118/G6H088
Contreras, D., Wilkinson, S., Aktas, Y. D., Fallou, L., Bossu, R., and Landes, M. (2022). Intensity-based Sentiment and Topic Analysis. The Case of the 2020 Aegean Earthquake. Front. Built Environ. 8, 1. doi:10.3389/fbuil.2022.839770
DASK (2021). DASK (Dogal Afet Sigortalari Kurumu). Available at: https://www.dask.gov.tr/. (Accessed December 11, 2021).
Demirci, H. E., Karaman, M., and Bhattacharya, S. (2021). A Survey of Damage Observed in Izmir Due to 2020 Samos-Izmir Earthquake. Nat. Hazards 111, 1047–1064. doi:10.1007/s11069-021-05085-x
Dogan, G. G., Yalciner, A. C., Yuksel, Y., Ulutaş, E., Polat, O., Güler, I., et al. (2021). The 30 October 2020 Aegean Sea Tsunami: Post-Event Field Survey along Turkish Coast. Pure Appl. Geophys. 178, 785–812. doi:10.1007/s00024-021-02693-3
Doğan, N. (2020). İzmir’de hasar ödemeleri başladı. Available at: https://www.hurriyet.com.tr/yazarlar/noyan-dogan/izmirde-hasar-odemeleri-basladi-41657926.
Elyamac, K. E., and Erdogan, A. S. (2005). Gecmisten Gunumuze Afet Yonetmelikleri Ve Uygulamada Karsilasilan Tasarim Hatalari. Kocaeli: Deprem Sempozyumu Kocaeli.
Erdil, B. (2017). Why RC Buildings Failed in the 2011 Van, Turkey, Earthquakes: Construction versus Design Practices. J. Perform. Constr. Facil. 31 (3), 1–13. doi:10.1061/(asce)cf.1943-5509.0000980
ESC (1998). European Macroseismic Scale 1998 (EMS-98). Luxembourg: European Seismological Commission.
Eurocode (2004). EC8: Design of Structures for Earthquake Resistance - Part 1: General Rules, Seismic Actions and Rules for Buildings, European Standard EN 1998-1:2004. Brussels: European Committee for Standardization.
Gurcaner, E., and Torlak, C. (2020). İzmir’de Rızabey ve Doğanlar Apartmanı’nda rapor skandalı! Belediye bildiği halde Bakanlığa bildirmemiş. Available at: https://www.sabah.com.tr/gundem/2020/11/02/son-dakika-izmirde-rizabey-ve-doganlar-apartmaninda-rapor-skandali-belediye-bildigi-halde-bakanliga-bildirmemis.
Ilki, A., and Celep, Z. (2012). Earthquakes, Existing Buildings and Seismic Design Codes in Turkey. Arab. J. Sci. Eng. 37, 365–380. doi:10.1007/s13369-012-0183-8
Izmir Metropolitan Municipality (2016). Izmir Kent Rehberi. Available at: https://kentrehberi.izmir.bel.tr/izmirkentrehberi. (Accessed December 11, 2021).
Kiratzi, A., Papazachos, C., Özacar, A., Pinar, A., Kkallas, C., and Sopaci, E. (2021). Characteristics of the 2020 Samos Earthquake (Aegean Sea) Using Seismic Data. Bull. Earthq. Eng. doi:10.1007/s10518-021-01239-1
KOERI (2020). Kandilli Observatory and Earthquake Research Institute (KOERI)-RETMC. (Boğaziçi University). Available at: http://www.koeri.boun.edu.tr/sismo/2/tr/2020/10/ (Accessed December 2, 2020).
Malcioglu, F. S., O'Kane, A., Donmez, K., and Aktas, Y. D. (2022). Characteristics of Strong Ground Motions in the 30 October 2020, MW6.9 Aegean Sea Earthquake. Front. Built Environ.
Mikres Diadromes Samou (2020). Mikres Diadromes Samou. Available at: https://www.youtube.com/channel/UC2eraxpvAND7VFC6F7GEqGw.
NOA (2020). National Observatory of Athens (NOA)-Institute of Geodynamics, Hellenic Strong Motion Network (HUSN). (Boğaziçi University). Available at: https://accelnet.gein.noa.gr/2020/11/09/the-earthquake-of-october-30th-2020-at-samos-eastern-aegean-sea-greece-preliminary-report/ (Accessed December 6, 2020).
Özmen, B. (2012). Türkiye Deprem Bölgeleri Haritalarının Tarihsel Gelişimi. Türkiye Jeol. Bülteni 55, 43.
Rossetto, T., Raby, A., Brennan, A., Lagesse, R., Robinson, D., Adhikari, R. K., et al. (2019). The Central Sulawesi, Indonesia Earthquake and Tsunami of 28th September 2018 – A Field Report by EEFIT-TDMRC. EEFIT IStructE.
Samos24 (2021). Additional Funding of 10 Million to Samos for Infrastructure and Ports. Available at: https://bit.ly/39POp1y (Accessed March 29, 2022).
Sezen, H., Elwood, K. J., and Whittaker, A. S. (2001). Evolution of Building Design and Construction Practice in Turkey. USGS Open-File Report 01-163 http://geopubs.wr.usgs.gov/open-file/of01-163/web/publication.htm.
So, E., Babic, A., Majetic, H., Putrino, V., Verrucci, E., Contreras Mojica, D., et al. (2020). The Zagreb Earthquake of 22 March 2020 - A Remote Study by the LfE UK Team for EEFIT. EEFIT.
Soyluk, A., and Harmankaya, Z. Y. (2012). Examination of Earthquake Resistant Design in the Education of Architecture. Procedia - Soc. Behav. Sci. 51, 1080–1086. doi:10.1016/j.sbspro.2012.08.291
Sucuoğlu, H. (2018). “New Improvements in the 2018 Turkish Seismic Code,” in International Workshop on Advanced Materials and Innovative Systems in Structural Engineering: Seismic Practices (IWAMISSE) (Istanbul).
Taymaz, T., Yilmaz, Y., and Dilek, Y. (2007). The Geodynamics of the Aegean and Anatolia: Introduction. Geol. Soc. Lond. Spec. Publ. 291 (1), 1–16. doi:10.1144/sp291.1
TBSC, (2018). Turkish Building Seismic Code. Turkish Disaster and Emergency Management Authority (AFAD), Prime Ministry of Turkey, Ankara
TMoEUCC (2020). Hasar Tespit Sorgulama. Available at: https://csb.gov.tr/en. (Accessed December 11, 2021).
Triantafyllou, I., Gogou, M., Mavroulis, S., Lekkas, E., Papadopoulos, G. A., and Thravalos, M. (2021). “The Tsunami Caused by the 30 October 2020 Samos (Aegean Sea) Mw7. 0 Earthquake: Hydrodynamic Features, Source Properties and Impact Assessment from Post-Event Field Survey and Video Records,” in Journal of Marine Science and Engineering, 9 (1).
TSHM, (2018). Turkish Seismic Hazard Map. Turkish Disaster and Emergency Management Authority (AFAD), Prime Ministry of Turkey, Ankara
USGS (2020). M 7.0 - 13 Km NNE of Néon Karlovásion, Greece. Available at: https://earthquake.usgs.gov/earthquakes/eventpage/us7000c7y0/dyfi/intensity. (Accessed December 11, 2021).
Keywords: remote reconnaissance, hybrid reconnaissance, COVID-19, Turkey, Greece, data collection
Citation: Aktas YD, Ioannou I, Malcioglu FS, Kontoe M, Parammal Vatteri A, Baiguera M, Black J, Kosker A, Dermanis P, Esabalioglou M, Cabuk E, Donmez K, Ercolino M, Asinari M, Verrucci E, Putrino V, Durmaz B, Kazantzidou-Firtinidou D, Cotton D, O’Kane A, Ozden AT, Contreras D, Cels J, Free M, Burton P, Wilkinson S, Rostami R and D’Ayala D (2022) Hybrid Reconnaissance Mission to the 30 October 2020 Aegean Sea Earthquake and Tsunami (Izmir, Turkey & Samos, Greece): Description of Data Collection Methods and Damage. Front. Built Environ. 8:840192. doi: 10.3389/fbuil.2022.840192
Received: 20 December 2021; Accepted: 13 April 2022;
Published: 01 July 2022.
Edited by:
Daniel V. Oliveira, University of Minho, PortugalReviewed by:
Hamed Azizi-Bondarabadi, Yazd University, IranAnna Karatzetzou, Aristotle University of Thessaloniki, Greece
Copyright © 2022 Aktas, Ioannou, Malcioglu, Kontoe, Parammal Vatteri, Baiguera, Black, Kosker, Dermanis, Esabalioglou, Cabuk, Donmez, Ercolino, Asinari, Verrucci, Putrino, Durmaz, Kazantzidou-Firtinidou, Cotton, O’Kane, Ozden, Contreras, Cels, Free, Burton, Wilkinson, Rostami and D’Ayala. This is an open-access article distributed under the terms of the Creative Commons Attribution License (CC BY). The use, distribution or reproduction in other forums is permitted, provided the original author(s) and the copyright owner(s) are credited and that the original publication in this journal is cited, in accordance with accepted academic practice. No use, distribution or reproduction is permitted which does not comply with these terms.
*Correspondence: Yasemin D. Aktas, WWFzZW1pbkQuQWt0YXNAdWNsLmFjLnVr