- 1Facultad de Ingeniería en Ciencias de la Tierra FICT, Escuela Superior Politécnica del Litoral, ESPOL, Guayaquil, Ecuador
- 2Facultad de Ingeniería Mecánica y Ciencias de la Producción, FIMCP, Escuela Superior Politécnica del Litoral ESPOL, Guayaquil, Ecuador
- 3Department of Civil and Environmental Engineering, Imperial College, London, United Kingdom
- 4School of Mechanical Engineering, Purdue University, West Lafayette, Indiana, United States
The need for social housing creates challenges for engineering. One of the most economical and ecological structural systems for certain areas is the cemented bahareque, which uses Guadua cane, a type of Bamboo with favorable properties for construction. Despite being an ancient technique for the construction of houses, there is not an extensive bibliography that allows making justified decisions regarding their design in most cases. One of the objectives of this article is to present a prototypical design of a housing case with appropriate characteristics to allow a decent occupant’s life with this construction system. For the selected house, the structural behavior is evaluated under gravitational and seismic loads. The constructive criteria that will provide good performance under seismic events are recommended. The most important criteria to follow for the design of wall systems are regularity, continuity, symmetry, bolted connections, rigid diaphragms for mezzanines and continuous maintenance of the Guadua cane elements that make up the framework of the walls. Finally, it is concluded that following the basic criteria of earthquake-resistant design for this type of housing, adequate structural performance can be obtained.
Introduction
According to Ministry of Urban Development and Housing, in Ecuador exists an increasing housing deficit, with an estimate of 2.7 million households living in housing deficiencies or poor housing conditions. The leading cause for this lack of access to decent housing conditions is poverty. Another important aspect underpinning the poor quality of housing can be attributed to the lack of penetration of construction standards in the country where, in many cases, people end up manufacturing their own homes without professional advice and not following structural codes. All these mean that a good structural performance cannot be guaranteed in the event of natural disasters (Ministry of Urban Development and Housing, 2020).
In this context, the need for social housing remains an important and unresolved issue. Social housing plans are based on low-cost projects that meet the demand for housing at a faster rate, granting financing advantages to people in need of covering the total cost of the property. These housing solutions are mostly constructed from reinforced concrete, which is the most common material in construction (Pérez-Pérez, 2016). Currently, reinforced concrete has been established as the standard alternative of material for high structural quality constructions. Unfortunately, this has been accompanied by an increasing rejection of traditional and ancestral constructive systems. One of these traditional materials is bahareque, a material traditionally used in some Latin American countries to cover their housing needs (Archila, et al., 2018). Bahareque is a system with several advantages, since its raw materials are affordable, readily available, structurally adequate, and is abundant in the territories of the Ecuadorian coastal region.
Bahareque (Quincha in other countries) is a composite construction system made from materials found in the dry forests of South America and Asia. It is composed of a bamboo structure (usually Guadua cane), forming a closed frame, also known as panel (Kaminski, et al., 2016). These panels are the main feature of bahareque housing structures. Normally, this is the material of interest for the structural analysis of buildings of this type (Málaga-Chuquitaype, et al., 2014). In addition to the Guadua cane, bahareque panels can be filled with clay (traditional bahareque) or mortar (cemented bahareque). During construction, wood boards, cement blocks, reinforcing steel, mesh, nails, among other elements, are also used (Elizabeth and Datta, 2013). In Latin America, most construction systems of bahareque walls have an aesthetically simple, sustainable, and low-cost architecture, thus constituting a set of characteristics suitable for projects of social interest. With proper care, a Bahareque home can be durable over time, with a life expectancy of at least 30 years before the Caña Guadua elements need to be replaced. However, many communities have lost their predisposition to continue using it due to false notions of being outdated and not structurally safe, due to the fact that the use of reinforced concrete systems became popular and standardized over other construction systems that include wood (Piedra-Landívar et al., 2019).
In addition to the above, bahareque structures do not have an extensive supporting scientific bibliography, in terms of standards, books, techniques and structural requirements, as opposed to reinforced concrete. Research in this field has been carried out primarily in Andean countries (Colombia, Ecuador, and Peru), which have explored empirical methods and investigations of the structural soundness of this material. As a result, there are guidelines and codes that can be used for the design, which are shown below:
⁃ Design Guide for Engineered Bahareque Housing-INBAR (Kaminski, et al., 2016)
⁃ Andean Standard for Design and Construction of One- and Two-Story Houses in Cemented Bahareque -INBAR (López-Muñoz, 2015)
⁃ NEC-SE-GUADÚA (Ministry of Urban Development and Housing, 2015a)
⁃ NSR-10: E-7 and Tittle G (Ministry of Environment, 2010)
⁃ Earthquake-resistant Construction, Evaluation and Rehabilitation Manual for Traditional Bahareque Housing (Colombian Association of Seismic Engineering - AIS, 2001)
The objective of this article is to provide a perspective on the structural design of a cemented bahareque housing unit. To this end, the concept of social housing will be introduced, and a model of housing design will be proposed. This is consistent with the characteristics and demands described above: economic, aesthetically pleasing, durable and structurally sound. Most considerations will be taken from the above-mentioned guides. To this end, this research aims to provide a systematic procedure to address bahareque housing with a satisfactory structural approach. Consequently, it is essential to understand the material to be used, such as bamboo and mortar. To assess the structural design of the house, this study will evaluate two load states: 1) Gravitational, which includes a factored load of the weight of the structural elements, that is, dead loads and live loads, and 2) dynamic, a response-history dynamic analysis of the proposed house will be carried to verify displacements and base shear. The results of this research work provide significant information on the adverse effects of the mentioned loads on the structure, and thus support future designs for this type of housing. Furthermore, the solution entertained herein has a good potential for reducing the environmental impact caused by other materials such as reinforced concrete.
Areas of implementation of the project
Structures must always be tested to meet the needs of a specific population. The Guadua cane is the most important genus of bamboo in America, endemic to this continent and consisting of about 30 species. Guadua angustifolia, native to Colombia, is the most important of these, thanks to its extraordinary physical-mechanical properties and the progress in its structural characterization that has been carried out in recent years. Although Guadua can be found in its natural state from Ecuador to Venezuela and between 0 and 2000 m above sea level, the optimal development of plants is reached between 500 and 1500 m, with temperatures ranging from 17°C to 26°C, rainfall of 700–1200 mm/year, and soils with moderate fertility and good drainage, characteristic of the central Andes region. For these reasons, many case studies have been reported by researchers in the aforementioned countries. In Asian countries such as India or China, there is also an important variety of bamboo for construction, reported extensively in the literature (Huang, 2021).
Figure 1 shows the natural growth of Guadua cane in the world and how it extends to both sides of Ecuador (National Geographic, 1980). This map is of importance because it gives an idea of where their constructions with this material are located and places where it is feasible to build them.
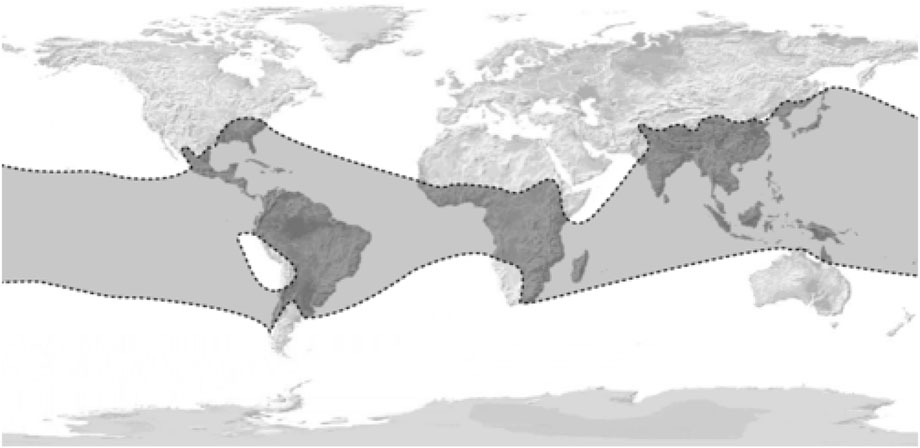
FIGURE 1. Global Natural Bamboo Habitat (National Geographic, 1980).
Figure 2 shows an example of a typical Bahareque house that can be found in the coastal region of Ecuador. This construction belongs to the city of Bahía de Caráquez located in the province of Manabí. As can be seen, the appearance of this type of building can even be very similar to that of traditional reinforced concrete houses due to social conventions.
This type of buildings can be found in countries such as Chile, Peru, Colombia and others in Latin America they often represent in historic constructions. This is the case of the study conducted by D’Ayala and Benzoni (2012), where they analyzed the response of historical structures to the earthquake that occurred in Chile in 2010. Although there were buildings that did not suffer major damage, they showed that it is essential to restrict the out-of-plane failure of such walls by adding buttresses or interior reinforcements in the walls (D’Ayala and Benzoni 2012). This failure is very common in this type of structure as opposed to traditional reinforced concrete structures. Another similar study carried out in Cusco, Peru (Brando, et al., 2019), also showed this behavior in the houses where 69% of the analyzed cases that did not take into consideration seismic aspects had presented it. The study also evidenced that the houses that did implement seismic design considerations including out-of-plane reinforcement were totally effective, so one of the recommendations given to rehabilitate the structures was to consider the out-of-plane reinforcement.
State of the art of cemented bahareque houses
For thousands of years construction systems have taken advantage of similar concepts to bahareque structures. Apart of its popularity in Andean countries, other latitudes have also implemented some form of this system. Consider for example adobe in Spain, wattle-and-daub in the United Kingdom, cuje in Cuba and Caribbean countries, Quincha in Peru, pao-a-pique in Brazil, and Tabiquería in other parts of Latin America, just to name a few. Each country has its own traditions and methods for the construction of these type of houses (Kaminski, et al., 2016). The bahareque is a structure in the form of a skeleton, composed of wood and/or bamboo, with an open or split layer of cane of smaller diameter. In Spanish, the term used for the smaller diameter cut cane is “Esterilla.” A galvanized hexagonal wire mesh is used as a crack deterrent. The design is completed with an outer layer of mud, or mixture of manure, straw, and soil or even cement mortar. This latter layer must fill all the spaces of the structure and is placed after the assembly of the former elements (Gatóo, et al., 2014). The bahareque construction system uses the position of the walls (symmetrical, regular, and continuous) and their bolted joints, to form a single rigid system, as shown in Figure 3 (Taochuan, et al., 2020). According to several studies reported in literature, a properly designed bahareque house shall include:
• Floors: No more than two story are recommended. As the height increases, the overturning action of lateral loads increases. Since Bahareque structures are very flexible and lack a well-defined yield point, excessive deformations can occur at higher demands, which can lead to the collapse of the structure. Indeed, it is possible to increase the number of floors of a Bahareque structure, but this would require an additional structural analysis where specific material testing campaigns are conducted where the deformation capacity of the material is corroborated. This increases the use of materials, therefore elevating the final cost (Dixon, et al., 2015).
• Foundation System: A lower layer must be used, which is typically a cyclopean concrete foundation, although ordinary concrete can also be used as an isolated foundation system. As an alternative, it is possible to use a foundation beam around the entire perimeter to overcome the differential settlement and finally, an external masonry support is introduced to prevent moisture from rising to the bamboo elements. The Guadua frames will begin to level at least 40 cm of the finished floor (Silva, et al., 2006). Cyclopean concrete is a technique where concrete is casted in situ with large stones. This is traditionally used for foundations or large walls.
• System of primary walls: This is the first portion of the panels of Guadua, which consists of vertical and horizontal studs of Guadua canes. A grid is then formed with bolted connections and cut cane sections for better support. Diagonal elements are also used. This system is installed throughout the perimeter of the structure (Elizabeth and Datta, 2013).
• One of the main failures that can occur in Guadua cane is the propagation of longitudinal cracks. Research on the types of bolted joints commonly used in this type of structures showed that joints such as the one shown in Figure 4, have a great influence on the energy dissipation capacity, since these frames can provide more than 10% damping to the structure (Hong et al., 2020).
• Mezzanine diaphragm: Wooden or bamboo joists, with wooden planks on the joists. The mezzanine should be unified with bolted connections to the lower and upper walls. In some cases, 5 cm thick mortar is used as the final floor (Kaminski, et al., 2016).
• Roof: Inclined frames are formed with wooden joists or cane, secured by bolted connections. Metal roofing materials such as Zinc or steel sheets are typically used for roofing.
• Wall system: The walls are finished with a lattice or matrix of “mat” or strips of Guadua. Then a galvanized steel mesh is nailed to the die. Finally, everything is gathered with cement mortar (cemented bahareque) or mud (traditional bahareque). It can be finished with waterproof paint on the outside (Armandei, et al., 2015).
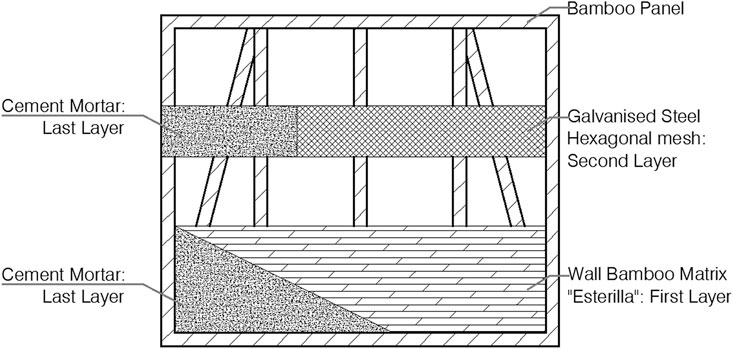
FIGURE 3. Cemented bahareque panel materials. Design inspired by the publication by López-Muñoz (2015).
It has been shown that the shape of the bamboo is adequate to dissipate energy and therefore reduce the concentrations of damage. In addition to being a ductile material, the frame with diagonal elements allows the reduction of horizontal displacements during a seismic event. For example, in the study “Experimental and numerical studies on hysteretic behavior of all-steel bamboo-shaped energy dissipaters” used a bamboo-shaped cored steel with external metallic restraints, able to dissipate energy at high stresses with low adverse effects (Wang, et al., 2018). The failure modes of their model were generated by high lateral loads that resulted in bending deformations. On the other hand, (Xu, et al., 2019) showed that for masonry walls, the addition of Guadua elements such as chopped bamboo grids (which are used in Bahareque) improve their seismic performance. This fact is observed if the grid matrix is added externally, showing improvements in shear strength, deformability, and ability to dissipate energy. In addition, the study “Dynamic modeling and optimal design of Tube–Diaphragm coupling beam inspired by bamboo” showed that bamboo is the natural structure with the best absorption of specific energy and specified stiffness, thus the impact tests presented, confirm that bamboo is capable of modifying its natural frequencies by adjusting the number of diaphragms and the thickness of the section to accommodate disturbances in the environment by (Taochuan, et al., 2020).
A considerable limitation of bahareque system are pests. Like most wood materials, the canes used in bahareque are, prone to host insects. Nevertheless, this can be easily overcome by an adequate preventive treatment and continuous maintenance. The insects that commonly attack Guadua cane include bedbugs and termites (Parrella and Molari, 2021).
Characteristics of social housing
Homes of social interest can be purchased by families with limited resources, with full or partial government aid. These must have a minimum area of 50 m2, and must comply with certain requirements, such as two bedrooms, one full bathroom, a living room, a kitchen, washing and drying areas, thus making it a decent and adequate home, focused on the low-income population (Pérez-Pérez, 2016). There are several price ranges for this type of housing, with costs ranging from US$ 13,000 to US$ 25,000. Low-income housing can be categorized according to its potential owners, if they are co-financed with government aid, or if they are completely financed by the government (Puri, et al., 2014).
Homes of social interest can be purchased by families with limited resources, with full or partial government aid (Puri, et al., 2014). These must have a minimum area of 50 m2, and must comply with certain requirements, such as two bedrooms, one full bathroom, a living room, a kitchen, washing and drying areas, thus making it a decent and adequate home, focused on the low-income population (Pérez-Pérez, 2016). For populations that have the construction material available in their regions, the Bahareque houses are a more economical, ecological, and cultural identity alternative for the populations. By 2021, social interest housing in Ecuador follows a total cost range between $13,000 and $25,000. These houses are usually built with reinforced concrete, which is susceptible to increased costs due to transportation or price increases from mining operations. The cemented Bahareque homes for populations that have the material available in their region, costs can decrease between 35 and 37% (Ministry of Urban Development and Housing, 2020).
By using mortar for the walls, the resistance to fire increases considerably compared to the construction system that uses mud for the walls. However, details that compromise the wood framework (Guadua cane or others) must be taken care of, before electrical connections, stoves, and any stimulus for fire.
Materials and methods
The design principles that govern bahareque housing are addressed in this section. Four design criteria are considered: 1) Earthquake resistance and general design guidelines; 2) architectural considerations; 3) Roof and high floor, and 4) structural considerations.
Earthquake resistance criteria
Bahareque, is a construction method that provides significant resistance to seismic events. Since Ecuador is located in an area of high seismic activity it is essential to ensure that these structures are safe from earthquakes. It should be noted that bamboo by itself is not efficient against lateral and compressive loads, and that its strength may be compromised by many other external factors such as: poor curation processes, variability in physical characteristics (diameter, length), variability in mechanical characteristics (aging, cracking, moisture), and unaware presence of insects (He, et al., 2014). In contrast, when bamboo is used as a component material of bahareque, embedded within cement or mud mortar, the earthquake-resisting capacity of the resulting composite panel increases dramatically. This was evident in seismic events such as the 2016 earthquake in Ecuador (M 7.8), where the seismic energy in some cases was mostly absorbed by the outer layers of the mortar, causing an exterior cracking also known as chipping. This damage to external portions of the wall could be easily repaired with a new coat of mortar and paint. Some damaged connections were corrected after inspection, (Moroz, et al., 2014).
An important characteristic of bahareque structures leading to a good seismic response is regularity in the walls, which shall rise in two directions perpendicular to the floor. Furthermore, it is not advisable to build walls on the strongest direction only and use another construction system on the weakest direction. The beam-column analysis of the Guadua elements does not provide an adequate view of the seismic resistance of this type of structure. Guadua elements must follow a regular pattern throughout the structure with height, thickness, design of the framework system and layers of homogeneous materials (Seixas, et al., 2017; 2021). On the other hand, the condition of symmetry in the walls must be followed: The proportion of the structural walls must be symmetrical in a bidirectional plane, both vertically and horizontally. In other words, a plan distribution as close to a square as possible is ideal, but rectangular models are allowed as long as their longer dimension does not exceed 1.5 times the dimension of the shorter side. If it is necessary to extend further the dimensions of one side with respect to the other, a separation of spaces must be made with simple joints, so that each space works independently and the desired proportion between walls is achieved (Xu, et al., 2019).
It is appropriate to add bracing elements or diagonals in the frames and floor diaphragms, this consideration provides stability against lateral loads, since it favors a more uniform dissipation of the seismic energy through the entire individual wall or through the floor diaphragm. In the case of the wall systems, the same Guadua cane is used for both the walls and the corner panels. For the individual walls, Guadua not only distributes the energy throughout the bamboo system, but also dissipates it on the outer walls (Wang, et al., 2018). Next, the floor diaphragms must be connected by bolted joints and ties, at their upper and lower walls to provide structural continuity in such a way that lateral loads are adequately transmitted. The wall system must be designed to support loads, both gravitational and lateral, and to achieve adequate load transmission, the connections must be bolted. To this end, a fish mouth cut, is used to connect perpendicular elements of Guadua. For diagonal connections 45° cuts can also be used. These connections are used to generate supports between elements and employ pre-drilled holes according to the bolt size, and then they are inserted between the Guadua connections and other wooden elements. For the connection of the foundation with vertical Guadua elements, anchor rods of sufficient length to reach the bottom of the foundation should be used.
It is important to consider water and humidity insulation. Water is one of the principal factors affecting the degradation of bahareque structures, so care must be taken such that it does not enter parts of the house. For the care of the roof, gutters distributed longitudinally in the fall of the roof slope are used, and then led to a collector through a PVC pipe. Waterproof paints are used along with overhangs to control moisture on the walls of the structure. Such overhangs must be designed at such a height that capillarity does not carry water from the floor to the walls. Although humidity and water are not direct causes of structural failure, they do represent serious damage to the wall system and reduce its mechanical properties (Archila, et al., 2018). Proper maintenance against insect pests must be established: this type of damage is extremely dangerous since it is not normally visible. In other cases, insects such as bed bugs or termites can eat a large part of the bamboo elements, causing the wall to collapse. It is important to check cracks and Guadua elements to certify that there is no insect damage and treat them if necessary (López-Muñoz, 2015). The quality of the Guadua cane should be according to the country’s standard with specifications that allow for the correct material to be identified by its condition, age, and color. However, these specifications are beyond the extent of the present study, and we suggest the interested reader to refer to NEC SE GUADUA (MIDUVI, 2016) for more information on this topic. Furthermore, the use of other types of wood in the construction of the wall system should follows similar guidelines.
Architectural considerations of the proposed house
To achieve a realistic and practical design, a two-story structure is presented and modeled herein. The distribution of spaces in the house was selected to take into account actual community demands, and to demonstrate that the proposed structural measures are feasible. Panels with vertical studs spaced 0.75 m from center to center are used. The extension of the longest side was defined to be 9 m, and the shortest side to be 6.30 m. These dimensions are in accordance with the principles of social housing, mentioned earlier. Perimeter walls and a central wall are used to generate greater stability. The walls are continuous since they are repeated both on the ground and high floor. The ground floor plan view is presented in Figure 5, whereas the upper floor plan view is shown in Figure 6. The wall systems, rigid diaphragm of both floors, can be seen as small circles between the wall lines in both figures.
Roof and mezzanine
The roof must have eaves large enough to cover the wall structure from rainwater. The eaves must be between 1 m and 5 m, measured horizontally from the end of the walls to the end of the roof. In this case, a surface of 9 m × 12 m is chosen. The roof must also function as a rigid element, so all joints must restrict further lateral motion. A steep slope between 20 and 25% is recommended, which allows for a rapid release of rainwater. Guadua sections with diameter of 0.1 m, every 0.50 m are used. The roof is shown in Figure 7.
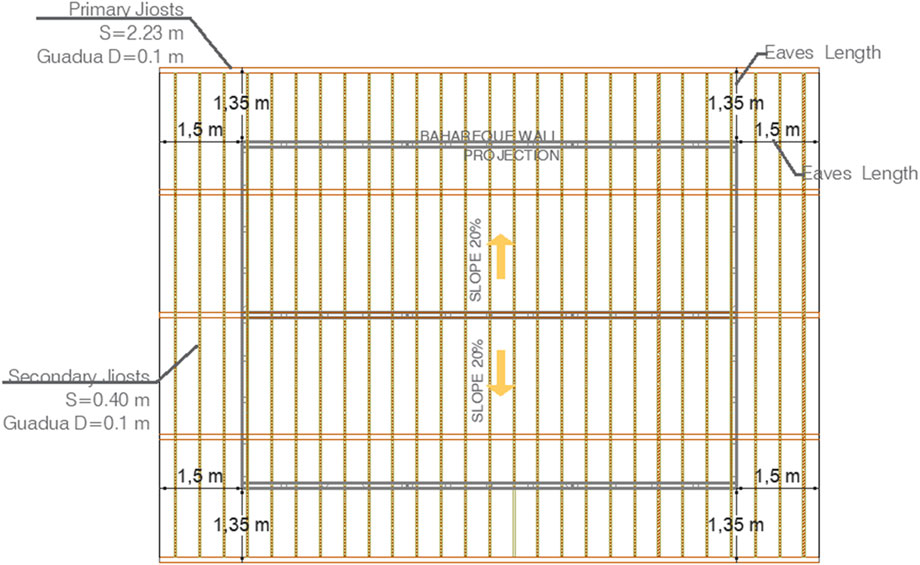
FIGURE 7. Roof Model. Design inspired by the publication by López-Muñoz (2015).
The configuration adopted for the mezzanine is shown in Figure 8. The mezzanine is completely flat, with an area of 6 m × 9 m, functioning as a rigid element that closes the area surrounded by the walls (54 m2). The joints of this element must be fixed to the upper walls of the first floor, and to the lower walls of the upper floor in such a way as to ensure continuity between the elements of the floor levels. Here rectangular sections with beams of 0.080 m × 0.18 m every 0.50 m are used. The diagonal elements must be distributed symmetrically. There is no restriction on how to distribute them, but it is important to add diagonal elements in the corners of the floor to ensure integrity (López-Muñoz, 2015).
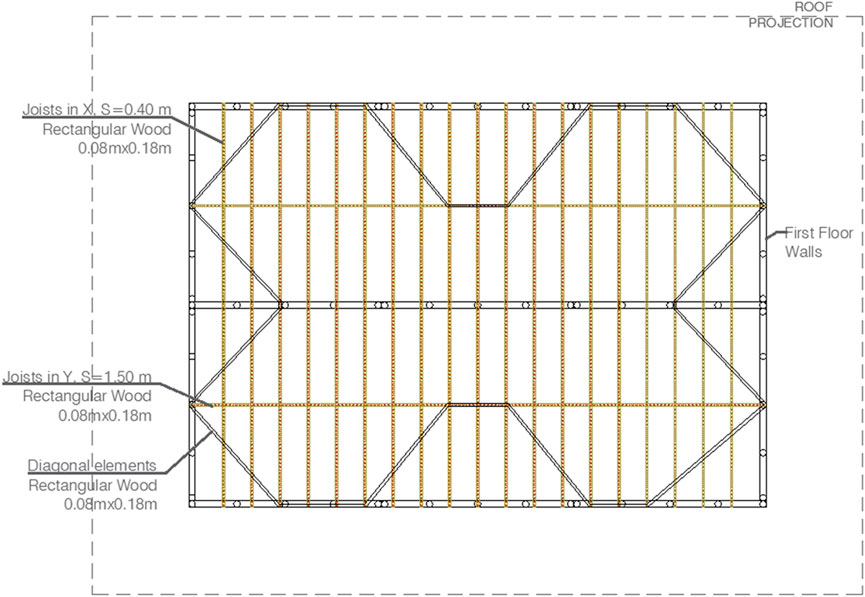
FIGURE 8. Mezzanine Diaphragm Model. Design inspired by the publication by López-Muñoz (2015).
Structural design
The structure must contain framework panels with diagonals located at the ends of the wall. The walls must contain framework panels just as the concrete must contain a steel reinforcement, so that the Guadua cane (tensile strength), combines with the mortar (compressive strength), and forms a single material (cemented Bahareque) capable of resist loads of both types. In this way, the bahareque shear walls are capable of providing greater resistance to the structure, fulfilling the roof with the minimum length and weight requirements that satisfy the design parameters.
The structural design process of a bahareque structure follows a standard procedure. A detailed structural analysis procedure is presented in this section. As mentioned before, the panels of the structure should be as uniform as possible and the same for both floors and all walls. In this case, Guadua with average diameter of 10 mm is used in vertical stud elements such as columns, and horizontal studs elements such as beams and diagonals. The center-to-center separation is 0.75 m. The total horizontal length is 3 m, and the height of the panel is 2.5 m. The designed Guadua panel is shown in Figure 9.
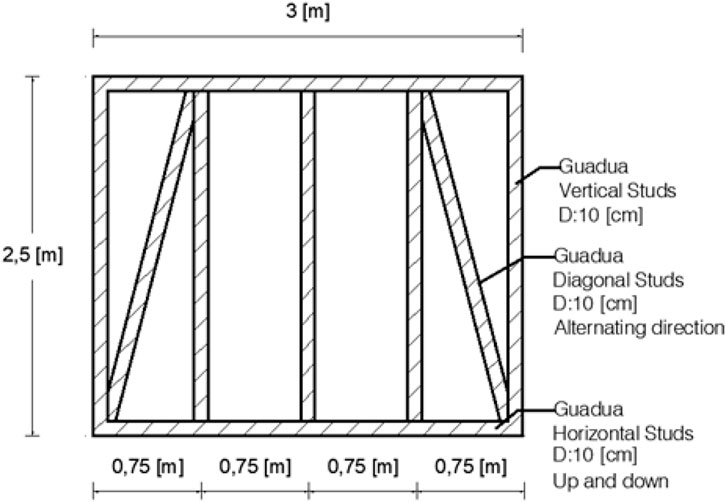
FIGURE 9. Design Framework panel. Design inspired by the publication by López-Muñoz (2015).
Loads and mechanical properties
The mechanical properties of the bamboo elements, such as inertia and cross-sectional area were calculated assuming tubular sections. Two values of modulus of elasticity are considered: 1) For cemented bahareque walls which includes the mortar and the bamboo matrix, and 2) for Guadua structural frames. The volumetric weight of the cemented bahareque is 1,700 kg/m3, while for the Guadua cane elements it is 700 kg/m3. Other additional properties are shown in Table 1.
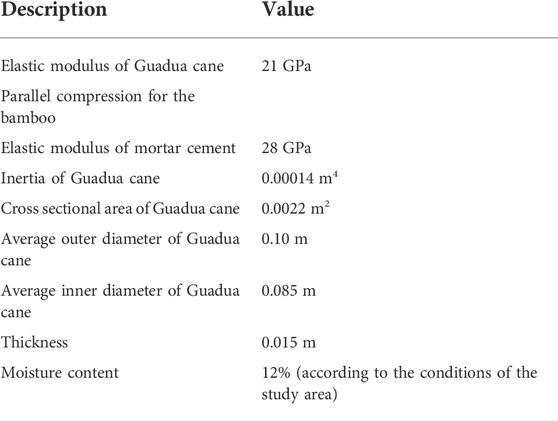
TABLE 1. Mechanical properties of materials (Ministry of Urban Development and Housing, 2015b).
The recommended values of the Ecuadorian Construction Standard in its chapter on Guadua Structures were adopted for the modulus of elasticity of Guadua cane. These guidelines to use the modulus of elasticity obtained for the parallel compression of a cane with a humidity of 12% for structural analysis and design (Ministry of Urban Development and Housing, 2015a).
The loads suggested by NEC-Loads (Ministry of Urban Development and Housing, 2014) for single-family homes are taken into account. The tributary area method is used to obtain the linear loads on the walls of the lower floor. The loads presented below are only useful for suggested homes or similar, which do not exceed a total weight of 60 tons and an area of about 60 m2. For the design, two types of analysis will be carried out: Gravitational and seismic, for which a factored dead load will be applied to the loads on the floors of the structure and distributed to the walls, to be resisted. The design dead load towards the floors is shown in Table 2. The calculation of these can be seen in the Appendix located in section 5.
Strength
The permissible strengths are obtained from the Andean Standard for Cemented Bahareque (López-Muñoz, 2015) in which values for different types of walls are found. The most specific case is about the stresses produced by gravitational loads. The stress limit states for Guadua members are detailed in Table 3. These values were obtained from the NEC-SE-Guadua 2015 standard.
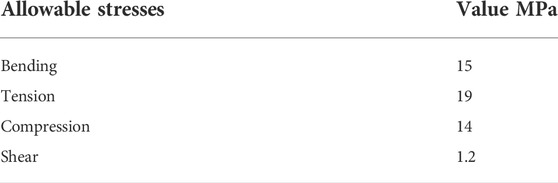
TABLE 3. Allowable Strength Design (ASD) for Guadua Members (Ministry of Urban Development and Housing, 2015b).
The analysis begins by calculating the bending stress, according to the NEC-SE-Guadua Standard (Ministry of Urban Development and Housing, 2015a), given by:
where
where
where,
where
Results and discussion
A finite element model (FEM) is carried out where the guadua elements of the house are considered as frame type elements in SAP 2000 (Elizabeth and Datta, 2013). They are organized as individual elastic frame elements and cracking of bamboo is disregarded. The elements are organized as individual frames. Two analyses are performed: 1) The structure subjected to the action of gravitational loads (static analysis); and 2) response history analysis (dynamic study). The framework panel is discretized in ten nodes, where the five upper nodes are always rigid diaphragms and the five lower nodes are distributed as follows: For the ground floor, the nodes are conditioned with a fixed support, while for the walls they are considered as rigid diaphragms. The panel framework has 15 Guadua cane elements, including horizontal, vertical, and diagonal studs. The house has a total of 26 frameworks panels, distributed in frames in the two main directions, that is, eight panels in the
The bahareque walls or panels are configured by a Guadua framework as mentioned throughout this document. The framework panels were configured symmetrically and continuously on the upper and lower floors. As shown in Figure 11, half of the frameworks are located on the ground floor and the other half above, the eight that correspond to the
The modelling process started with the definition of the horizontal, vertical, and diagonal elements, connected by means of rigid rather than hinged joints. This is a modelling decision that assumes that the concrete present in the joints would improve the crushing strength of bamboo while also increasing its resistance to local deformations, which in conjunction with the steel bracketry (if present), will stiffen the rotational response of the joint. It is acknowledged, however, that the actual response though closer to our rigid assumption will be semi-rigid. Nonetheless, the assumption of the simpler rigid model is made to balance between the computational costs and the uncertainties associated with the calibration of more advanced joint models. Moreover, an orthotropic material model with a modulus of elasticity in parallel compression and a modulus of elasticity in bending as provided by NEC-SE-GUADUA (Ministry of Urban Development and Housing, 2015a) was considered.
The material properties of the Guadua elements are assigned considering the properties already defined in Table 1. The floor slabs were considered in a way that loads are transmitted at all levels, whereas the wall surfaces are assumed made from concrete due to its similarity to the materials used in bahareque structures, and in such a way that the canes are embedded within the concrete material, minimizing the occurrence of a possible displacement. A snapshot of the 3D model is shown in Figure 12.
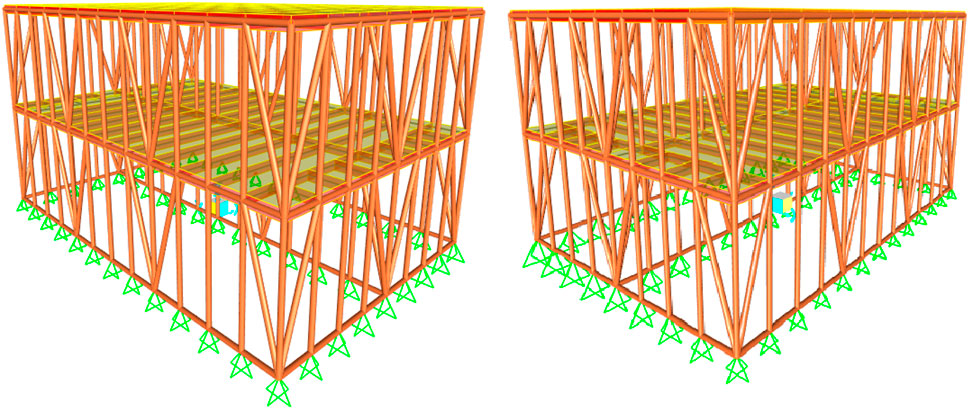
FIGURE 12. Bahareque structure modeled in SAP 2000. (Brown frame elements represent Guadua canes, shell elements represent floor slabs, and light thin lines represent boundary conditions).
The diagonals were added not only at the corners but in each panel, every other three vertical Guadua elements, that is, one single frame per vertical space used. Thus, in case of needing to increase the number of sections, this can be solved manually, as shown in Figure 13.
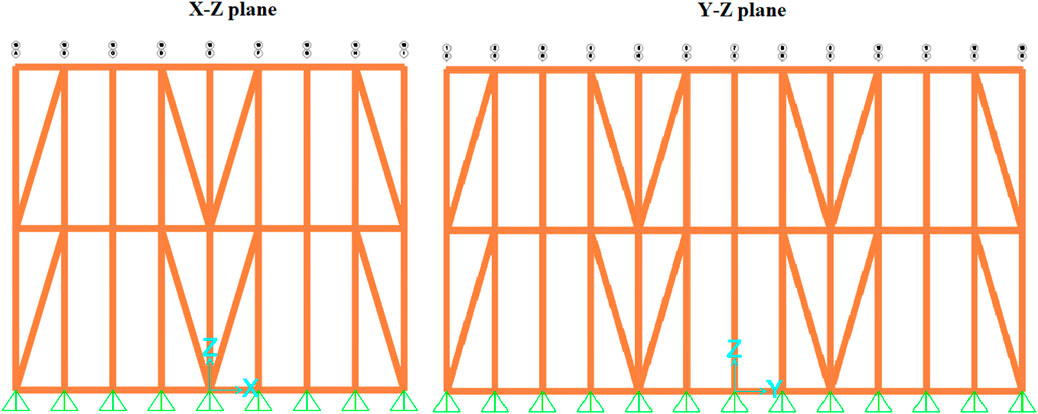
FIGURE 13. Generalized frames of the bahareque structure in both directions of the X-Z and Y-Z plane.
This structural model does not include some construction details, but it is essential to guarantee compliance with the allowable stresses. Analyses are further conducted out for seismic and gravitational loads.
Gravity load action analysis
The action of gravitational loads is caused principally by the weight of the walls with respect to other elements of the house. Therefore, the seismic design regulations must be followed. The results obtained with a test run in SAP2000 using gravitational loads are presented in Table 4.
The maximum values of internal forces produced by the gravitational load for the Guadua elements were thus obtained. With these values, compression, shear and bending stresses were also computed. For this model, the loads produced by the weight of the structure are of lesser importance and produce stresses within the range of allowable values. The admissible stress values can be reviewed in section 2.3.2. For the panels in both directions, the deformations produced by gravitational loads are as follows in Figure 14.
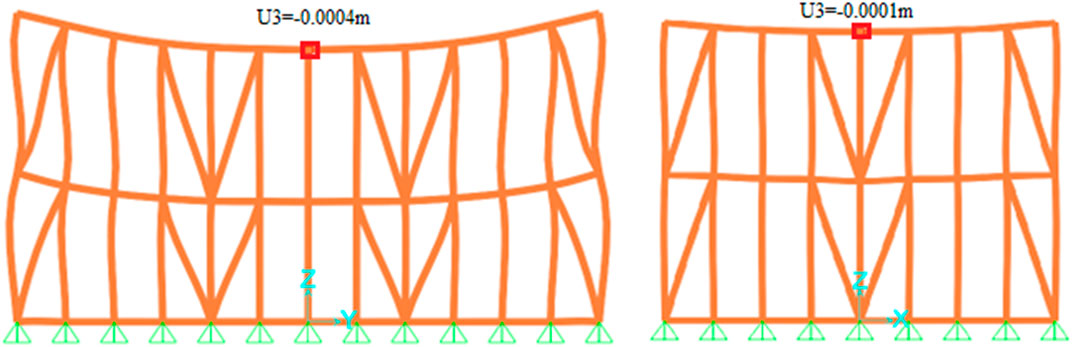
FIGURE 14. Deformations due to gravitational load. U3 represent in the model the maximum displacement in the z axis, that is the gravitational direction. U3 = 4.00 × 10−4 m.
The action of gravity loads causes very small deformations in the slabs. A scaled-up visualization of the deformed shape shows that the deformations are greater in the center of the slabs with a deflection of 2.3 × 10–4 m. This does not exceed the allowable deformation due to service loads for slabs stipulated by the IBC 2018 code in its table 1604.3 on Deflection Limits (ICC, INC, 2017), which for this building is 0.0125 m. Regarding the load supported by vertical studs, it should be noted that the admissible value of 16 kN was exceeded at the corners, where an action of almost 29 kN was observed. However, since, out of practical modelling considerations, the model employs one single frame per corner, and not the two-frame connection, this axial load is divided by two, producing a load of 14.5 kN, which falls within the ranges of the regulations.
Seismic load action analysis
Modal analysis
The modal analysis (and all seismic analyses) was performed considering 100% of the mass corresponding to the dead load and 30% of the live load. This load was the weight of the material, which is calculated by the program based on its specific weight properties and the imposed dead loads like interior walls and their linings.
The three fundamental modes of vibration are shown below in Figure 15.
The first mode represents the translational vibration that the structure will have in the x-axis and is associated with a fundamental period of 0.156 s (6.38 Hz). If the same building were compared with a reinforced concrete building with moment resisting frames, the maximum period allowed according to the standard seismic risk would be 0.304 s (3.28 Hz) (Ministry of Urban Development and Housing, 2015b). This suggests to the good seismic performance that can be achieved by well-designed bahareque buildings that limit lateral deformations. The short vibration period of the building is due to its low weight. The second mode represents translation in the y-axis, and finally the third mode represents torsional vibration.
Response history analysis
The proposed structural model was also subjected to a seismic analysis test. A nonlinear dynamic time history analysis was applied. For this purpose, ten historic seismic records with similar magnitudes were used, assuming soil type D and zone III. The characteristics and output time step number and sizes are listed in Table 5.
The time history of base shear in
The base shear is the maximum expected force due to the seismic action for this case as can be seen in Figure 16. The mean value is 222.95 kN, and the maximum is 373 kN.
In the case of the
A similar behavior can be observed in Figures 18, 19. The maximum value in both the
As can be seen in Table 6, the resulting forces at the base are small in comparison to other types of buildings, which means no need of stronger foundations and lower soil discharges. This makes this type of building more feasible in any type of soil without incurring in costly foundations.
Regarding the maximum displacements in the six degrees of freedom of a node, translational, rotational and in the two directions of plane movement. The node marked in red in Figure 20, indicates the maximum displacement of the structure produced by the seismic force.
The summary of the maximum deformations is shown in Table 7.
The maximum displacement is 0.01 m. This value was obtained from the average of the maximum displacements produced by each earthquake. In addition, the reduction in PGA allowed by the local code (Ministry of Urban Development and Housing, 2015a) has not been considered in the interest of the generalization of the analysis. This reduction in PGA is associated with specific site-dependent factors that aim to reduce the earthquake design intensity. But in this case, the structure has been subjected to all the recorded seismic force. With this value, the maximum drift of the structure is obtained. According to the Ecuadorian construction regulations (Ministry of Urban Development and Housing, 2015b), the admissible drift for buildings is 2%, which should be compared with an “inelastic” drift estimation obtained by multiplying the elastic value by the corresponding response factor. In the case of the building under consideration, the corresponding “inelastic” drift is 0.28%, which is well below the permissible limit and therefore it can be concluded that the structure shows a satisfactory seismic behavior. For the drift, it was mainly considered that the type of directional symmetry is orthotropic, due to the fact that the Guadua sections are hollow. Since the earthquakes were not reduced considering zone factors, but the structure faced all the seismic action, this drift is considered as the maximum drift that is intended to be obtained in the structure.
Foundation design
The standard recommends a continuous reinforced concrete foundation, prior to a layer of cyclopean concrete. Then, for the upstand foundation, a confined masonry is recommended. A schematic detail of the foundation system is shown in Figure 21. Since it is a two-story structure, a raised concrete beam is placed under the upstand foundation, with a section of 0.40 m × 0.25 m and with bars and stirrups designed as if it were a reinforced concrete foundation beam. In this case, the loads are lower than those of a reinforced concrete house and do not require reinforcements with diameters greater than
The foundation has to support the load of the Guadua cane elements with the mortar walls and its other components. This load including the dead load and live load on the foundation is approximately 12.54 kPa, which represents a much lower value compared to the contribution of resistance of the foundation elements of 21 MPa. It is important to clarify that the dimensions and steel design results were specifically calculated for a two-story house. The importance of the foundation relies in providing a bahareque housing the stability that it cannot achieve by itself due to its lightweight. The base must be anchored in each right foot so that it can contribute to the transfer of loads and interaction between the bahareque wall and the reinforced concrete foundation.
Conclusion
In this paper, a seismic design of a two-story bahareque structure of a house destined for social interest is presented and discussed. The design has been conducted following the recommendations and standards available for Ecuador such as Andean Standard for Cemented Bahareque and the Ecuadorian Standard for the Construction of Guadua Structures. The results of the gravitational analysis, show that for small houses such as the one proposed herein, the limit resistance parameters are met. A stress demand of 7.57 MPa is identified, which is far from the maximum permissible strength value of 14 MPa, in this case, crushing or compression is identified as the controlling stress. One of the main challenges of this project was to find the most appropriate way to structurally represent the typical low-income housing, considering the action of the loads generated by the elements that make up the system, in addition to its connections with bolts and the matrix of chopped cane.
Structure was the subjected to extremely intense seismic events in a simulation scenario, as smaller earthquakes, represent little risk for this type of structure. Although in the analysis the lateral loads generated on the structure exceeded the resistance limits, the deformations are in reality rather small, (less than 0.015 m). This fact is consistent with other records of earthquake resistance of bahareque structures, where the damage was only superficial. Thus, bahareque houses favorably withstand large lateral loads with minimal deformations. Moreover, the behavior against seismic loads is sufficiently acceptable to suggest building projects of this type. Regarding resilience, since repair costs are accessible to the affected population in the event of an earthquake, this type of construction constitute a highly resilient alternative for housing at a very affordable cost, with an added advantage of being very lightweight structures.
The structural design put forward in this paper uses a superelevated foundation beam instead of a squared type, as recommended by the standard. In addition, it is recommended that the height of the foundation be increased to 40 cm, exceeding by 10 cm what is established in the standard.
The continuous learning of the use of bahareque structures will bring about multiple benefits to the areas of the world where abundance of the material is apparent. Therefore, it is important that studies like this be added and become available to government institutions responsible of social housing construction projects, thus making visible the immense opportunity and potential that bahareque structures provide.
Data availability statement
The raw data supporting the conclusions of this article will be made available by the authors, without undue reservation.
Author contributions
All authors listed have made a substantial, direct, and intellectual contribution to the work, and approved it for publication.
Conflict of interest
The authors declare that the research was conducted in the absence of any commercial or financial relationships that could be construed as a potential conflict of interest.
Publisher’s note
All claims expressed in this article are solely those of the authors and do not necessarily represent those of their affiliated organizations, or those of the publisher, the editors and the reviewers. Any product that may be evaluated in this article, or claim that may be made by its manufacturer, is not guaranteed or endorsed by the publisher.
References
Archila, H., Kaminski, S., Trujillo, D., Zea-Escamilla, E., and Harries, K. A. (2018). Bamboo reinforced concrete: A critical review. Mat. Struct. 51 (4), 102. doi:10.1617/s11527-018-1228-6
Armandei, M., Darwish, I., and Ghavami, K. (2015). Experimental study on variation of mechanical properties of a cantilever beam of bamboo. Constr. Build. Mater. 101, 784–790. doi:10.1016/j.conbuildmat.2015.10.078
Brando, G., Cocco, G., Mazzanti, C., Peruch, M., Spacone, E., Crayla, A., et al. (2019). Structural Survey and Empirical Seismic Vulnerability Assessment of Dwellings in the Historical Centre of Cusco, Peru. Int. J. Archit. Heritage 15 (10), 1395–1423. doi:10.1080/15583058.2019.1685022
Colombian Association of Seismic Engineering - AIS (2001). “Manual de construcción evaluación y rehabilitación sismo resistente en viviendas de Bahareque Tradicional,” in Bogota: Fund for the reconstruction and social development of the coffee region - FOREC.
D’Ayala, D., and Benzoni, G. (2012). Historic and Traditional Structures during the 2010 Chile Earthquake: Observations, Codes, and Conservation Strategies. Earthquake Spectra 28 (S1), S425–S451. doi:10.1193/1.4000030
Dixon, P., Ahvenainen, P., Aijazi, A., Chen, S., Lin, S., Augusciak, P., et al. (2015). Comparison of the structure and flexural properties of moso, guadua and tre gai bamboo. Constr. Build. Mater. 90, 11–17. doi:10.1016/j.conbuildmat.2015.04.042
Elizabeth, S., and Datta, A. K. (2013). Retracted article: On the seismic performance of bamboo structure. Bull. Earthq. Eng. 18, 5413–5418. doi:10.1007/s10518-013-9561-1
Gatóo, A., Sharma, B., Bock, M., Mulligan, H., and Ramage, M. (2014). Sustainable structures: Bamboo standards and building codes. Proc. Institution Civ. Eng. - Eng. Sustain. 167 (5), 189–196. doi:10.1680/ensu.14.00009
He, M., Zheng, L., Sun, Y., and Ma, R. (2014). Experimental investigations on mechanical properties and column buckling behavior of structural bamboo. Struct. Des. Tall Spec. Build. 24 (7), 491–503. doi:10.1002/tal.1176
Hong, C., Li, H., Xiong, Z., Lorenzo, R., Corbi, I., Corbi, O., et al. (2020). Review of connections for engineered bamboo structures. J. Build. Eng. 30, 101324. doi:10.1016/j.jobe.2020.101324
Huang, Z. (2021). Resource-driven sustainable bamboo construction in asia-pacific bamboo areas. Guangzhou: Green Energy and Technology. ISBN: 978-3-030-73535-7.
Kaminski, S., Lawrence, A., and Trujillo, D. (2016). Design Guide for engineered bahareque housing. Quito: INBAR - International Bamboo and Rattan.
López-Muñoz, F. (2015). Norma Andina para diseño y construcción de casas de uno y dos pisos en Bahareque encementado. Quito: INBAR - International Bamboo and Rattan.
Málaga-Chuquitaype, C., Kaminski, S., Elghazouli, A. Y., and Lawrence, A. (2014). Seismic response of timber frames with cane and mortar walls. Proc. Institution Civ. Eng. - Struct. Build. 167 (12), 693–703. doi:10.1680/stbu.13.00090
Ministry of Environment, H. a. (2010). Colombian earthquake resistant construction regulations - Title G - wood structures and Guadua structures. Bogota: Government of Colombia.
Ministry of Urban Development and Housing (2020). Housing deficit in Ecuador. Retrieved from Government of Ecuador: https://www.habitatyvivienda.gob.ec/deficit-habitacional-nacional/.
Ministry of Urban Development and Housing (2014). Norma ecuatoriana de La construccion - cargas (No sísmicas). Quito: Government of Ecuador.
Ministry of Urban Development and Housing (2015a). Norma ecuatoriana de la Construcción - estructuras de Guadua. Quito: Government of Ecuador.
Ministry of Urban Development and Housing (2015b). Normativa ecuatoriana de la Construcción - diseño sismo resistente. Quito: Government of Ecuador.
Moroz, J. G., Lissel, S. L., and Hagel, M. D. (2014). Performance of bamboo reinforced concrete masonry shear walls. Constr. Build. Mater. 61, 125–137. doi:10.1016/j.conbuildmat.2014.02.006
Parrella, V., and Molari, L. (2021). Building retrofitting system based on bamboo-steel hybrid exoskeleton structures: A case study. Sustainability 13 (11), 5984. doi:10.3390/su13115984
Pérez-Pérez, A. (2016). El diseño de la vivienda de interés social y la satisfacción de las necesidades y expectativas del usuario. Rev. Arquit. 18 (1), 67–75. doi:10.14718/RevArq.2016.18.1.7
Piedra-Landívar, C., Achig-Balarezo, M., Caldas-Freire, V., Salazar-Silva, I., and Cardoso-Martínez, F. (2019). Analysis and proposal to retrofit the traditional construction systems (earth) of the former san josé school, cuenca, Ecuador. Struct. Analysis Hist. Constr. 18, 144–152. doi:10.1007/978-3-319-99441-3
Puri, V., Chakrabortty, P., and Majumdar, S. (2014). A review of low cost housing technologies in India. Adv. Struct. Eng. (3), 1943–1955. doi:10.1007/978-81-322-2187-6_150
Seixas, M., Eustáquio-Moreira, L., Stoffel, P., Bina, J., Mendes-Ripper, J., Ferreira, J., et al. (2021). Analysis of a self-supporting bamboo structure with flexible joints. Int. J. Space Struct. 36 (2), 137–151. doi:10.1177/09560599211001660
Seixas, M., Ripper, J., and Khosrow, G. (2017). Prefabricated bamboo structure and textile canvas pavilions. J. IASS. 57, 179–188. doi:10.20898/j.iass.2016.189.782
Silva, E. C., Walters, M., and Paulino, G. (2006). Modeling bamboo as a functionally graded material: Lessons for the analysis of affordable materials. J. Mat. Sci. 41 (21), 6991–7004. doi:10.1007/s10853-006-0232-3
Taochuan, Z., Duan, C., Wang, A., and Wang, Q. (2020). Dynamic modeling and optimal design of Tube–Diaphragm coupling beam inspired by bamboo. Thin-Walled Struct. 155, 106836. doi:10.1016/j.tws.2020.106836
Wang, C.-L., Liu, Y., and Zhou, L. (2018). Experimental and numerical studies on hysteretic behavior of all-steel bamboo-shaped energy dissipaters. Eng. Struct. 165, 38–49. doi:10.1016/j.engstruct.2018.02.078
Xu, Q., Chen, X., Chen, J.-F., Harries, K., Chen, L., and Wang, Z. (2019). Seismic strengthening of masonry walls using bamboo components. Adv. Struct. Eng. 22, 2982–2997. doi:10.1177/1369433219855902
Appendix
Calculation of accumulated dead loads in the structure
Since the walls were designed so that they all have the same dimensions, we proceed to calculate the mortar filling they will have, for this we will consider the dimensions of the wall and initially assume that the reeds are not present, and then proceed to subtract the volume of the reed to obtain the real volume.
similarly, we proceed to calculate the volume of the Guadua belonging to the wall.
then to obtain the mass of mortar we proceed to multiply its volume by its density
With:
for the mass of the Guadua we have:
With:
The total mass of the Framework panel is obtained:
Later, we obtain the weight of the Framework panel:
To obtain the total weight, we multiply by the number of walls that exist on the analyzed floor.
This weight is divided by the floor area to obtain the dead load per square meter generated by the walls.
Finally, this exercise should be done for each floor and the roof, and the load that each level will support should be obtained by acomenccumulating the loads above the analyzed level.
Keywords: bahareque, guadua, social housing, structural behavior, framework panel
Citation: Mite-Anastacio F, Tello-Ayala K, García-Troncoso N, Silva CE, Malaga-Chuquitaype C, Arévalo K and Villao D (2022) Structural behavior of cemented bahareque for social housing: A case study in Guayaquil City, Ecuador. Front. Built Environ. 8:922397. doi: 10.3389/fbuil.2022.922397
Received: 17 April 2022; Accepted: 03 October 2022;
Published: 20 October 2022.
Edited by:
Ibrahim Yitmen, Jönköping University, SwedenReviewed by:
Giuseppe Brando, University of Studies G. d’Annunzio Chieti and Pescara, ItalyElias G. Dimitrakopoulos, Hong Kong University of Science and Technology, Hong Kong SAR, China
Copyright © 2022 Mite-Anastacio, Tello-Ayala, García-Troncoso, Silva, Malaga-Chuquitaype, Arévalo and Villao. This is an open-access article distributed under the terms of the Creative Commons Attribution License (CC BY). The use, distribution or reproduction in other forums is permitted, provided the original author(s) and the copyright owner(s) are credited and that the original publication in this journal is cited, in accordance with accepted academic practice. No use, distribution or reproduction is permitted which does not comply with these terms.
*Correspondence: Natividad García-Troncoso, nlgarcia@espol.edu.ec