- Department of Interior Design College of Architecture and Design—CAD, Prince Sultan University, Riyadh, Saudi Arabia
Introduction: Sudan is taking proactive steps towards environmental sustainability, including initiatives to improve energy efficiency and reduce costs in a hot, dry climate. This article assesses the contribution of natural ventilation to heat transfer and temperature conditioning in urban areas in Khartoum, Sudan.
Methods: The research methodology used a combination of building modeling with computational fluid dynamics (CFD) integrated with IESV Autodesk software to model natural ventilation and simulate energy consumption by incorporating natural ventilation into home design.
Results: The best-case scenario for natural ventilation resulted in a 71.1% yearly energy savings. The design point of internal air speed was approximately 0.7 m/s, the point at which 95% of the indoor areas had an average air speed between 0.43 m/s and 0.9 m/s. The worst-case scenario occurred in the east/west direction, when 80% of the interior had an airflow between 0.05 m/s and 0.01 m/s; near open windows, the airflow was approximately 0.1 m/s.
Discussion: This study was the first research in Khartoum on the design of clean and energy-saving sustainable architecture. The architectural design process for energy-efficient living in the urban region of Al-Azhari City was implemented and defined in a city quarter in Khartoum. The results showed that natural ventilation can maintain a comfortable indoor temperature in summer and significantly reduce energy costs. The findings may have implications for the design of sustainable buildings in other hot, dry climates.
1 Introduction
Building design utilizing the energy consumption perspective of natural ventilation involves designing, choosing, and calculating the ratings of the system’s equipment (Shirzadi et al., 2018). This procedure is contingent on several variables, including geographic location, sun irradiation, and load requirements. As a developing nation, Sudan is experiencing rapid urbanization; the proportion of urban regions is anticipated to increase dramatically in a short time. Sudan is taking a proactive approach to environmental sustainability and is making progress in a number of key areas. These initiatives are a positive step toward a more sustainable future for the country. Consequently, it is essential to identify the obstacles to urban housing in Sudan (Elhassan et al., 2011a). Energy consumption is an important part of the optimal design of a dwelling, and natural ventilation, which is suitable for cooling a house via green architecture, reduces the electrical load. In this design, natural ventilation was used to cool the interior temperature to a comfortable level in summer while, at the same time, saving energy in the hot region of Sudan. IESV average energy consumption was calculated using Autodesk IES (VE) v5.9.0.3 software for computational fluid dynamics (CFD) design (Ray et al., 2014; Aldawoud, 2017). The study was conducted in Khartoum, with the goal of designing a clean and energy-saving house with sustainable architecture based on all the spatial planning requirements of the site, and of Khartoum state in general. For design purposes, researchers considered one type of residential area of Block 10 of Alazhari City (an urban area in Khartoum); The residential area type was an isolated load-bearing type, as shown in Elhassan et al. (2011b). The floor plans of houses in different residential area types differ slightly (Elhassan et al., 2011b; Zeinab et al., 2012a).
The principle of natural ventilation has been widely adopted by individuals and architects around the world. The use of natural ventilation in buildings is gaining popularity due to energy concerns, indoor air quality concerns, and environmental issues associated with mechanically ventilated structures (Ohba and Lun, 2010). A large number of national and international natural ventilation initiatives have been reported, and Hong et al. (2017) explained that the general analysis strategy regarding natural ventilation has evolved from traditional to computational methodologies. This implies that computer modeling is the dominant method for creating problem concepts and solutions for structures in the 21st century. Mochida and Lun (2008) and Ai and Mak (2017) highlighted that as wind modeling and computer technology has advanced, the use of natural ventilation has become increasingly popular. This is due to its adaptability, informative results, and cost-effectiveness in terms of labor and equipment. Additionally, CFD can be used to model both stationary objects, such as buildings, and non-stationary barriers, including moving cars and trees, in the surrounding environment (Cuce et al., 2019). According to Ai and Mak (2017), energy simulation and CFD have become integral in building design as they provide valuable complementary information on building performance. However, it is important to note that, because of the inherent assumptions of each approach, using them separately may not produce an accurate prediction of the thermal and flow behavior of a building (Hong et al., 2017). For example, many simulation programs assume that the air within indoor spaces is evenly mixed, that the temperature and pollutants are spread evenly throughout a zone, and that any impulse effects are not taken into account (Li and Li, 2015).
According to Wesseling (2009) and Li and Li (2015), predicting thermal comfort in naturally ventilated buildings using CFD modeling can be challenging as it involves simulating the effects of climate on both large and small-scale environments, from neighborhoods to individual rooms. It also includes indoor thermal environment boundary conditions and heat transfer/fluid dynamics calculations. The accuracy of CFD studies for external flows is limited and needs to be carefully evaluated. However, CFD can provide detailed results and information on specific conditions of a building when used to study the airflow around it. Therefore, the use of CFD-based urban climate assessment tools is crucial for its development and implementation (Guo et al., 2015; Zhong et al., 2016). Both the CFD method and the building simulation method have their unique drawbacks in terms of the amount of computational performance they require. However, recent advancements in computer performance IES (VE) v5.9.0.3 software integrated with building simulation have made it possible to improve the accuracy of evaluating the effectiveness of natural ventilation. Recent advancements in data analysis procedures have also made it possible to enhance the precision of IES (VE) v5.9.0.3 software when integrated with building simulation. This has been a key area of research for natural ventilation in buildings in recent years, and the integration of building modeling with CFD has become a popular trend in providing accurate solutions (Zhai, 2006).
The physical features of Alazhari City (an urban area in Khartoum) have been described by Hafazalla (2008) and Elkheir (2012). Greater Khartoum is the name given to the capital of Sudan, and the urban areas of Khartoum, Khartoum North, and Omdurman are at the junction of the Blue and White Niles at 15°36′N 32°32′E and 96.520 m above sea level (Hamid and Bahreldin, 2014; Yakoub et al., 2019). The Sudanese capital covers an area of more than 22,122 km2 (Mohammed, 2020; Hassan et al., 2017). The general topography of the land in Alazhari City, a part of Khartoum state, is flat with a gradual slope toward the north and the Nile (Mohammed, 2020). To the west is land-bearing Nubian stones and water-bearing sedimentary formations. To the south, it is covered with 30 m of dark, heavy silt called Gezira clay (Mohammed, 2020). The central ports of the three towns fall within the area of recent deposits of alluviums, wadi fill, terraces, and delta and swamp deposits in Greater Khartoum (Hafazalla, 2008; Elkheir, 2012).
2 Research area and data
2.1 Climatic conditions
The weather in Greater Khartoum, located at the southernmost tip of the Sahara Desert, has been described as a hot desert. From November to May, the climate is hot and dry, with a three-month cold spell between December and February. A three-month rainy season dominates from June to October (Hassan et al., 2017). Therefore, at least sixty percent of the average rainfall is relatively low, and the sloped land is susceptible to significant erosion. With yearly evaporation rates of 3,000 mm, the annual precipitation is just 150 mm. The wind direction follows a predictable pattern (Mohammed, 2020). During the dry season, the winds blow from the north, whereas during the brief wet season, the winds blow from the south. Soil corrugations discovered west of Omdurman are likely caused by the ferocity of the prevailing north winds. May and June are known for their volatility and, with that, comes the “Haboob” wind. Between May and September, the sun shines for an average of 95% of each day (Hafazalla, 2008). The area is connected with the center of Khartoum through the Medany highway, and also connected with the cities of Al-Ingaz, Mayo, and Idd-Hussain (Elnazir and Feng, 2004; Hafazalla, 2008).
2.2 Urban planning of Al-Azhari City (an urban area of Khartoum state)
The most central modern cities, characterized by the delimitation of the main street breaking into other main branches, as well as all blocks in central cities, consist of 50,000–100,000 inhabitants (Davies and Sin, 1991; Hamid and Bahreldin, 2013). Although the shape of the city includes different grades and blocks, both vertical and horizontal streets are planned. There is a main center where the different blocks are apparent. This type of planning presents some difficulties and needs to be improved to increase efficiency (Doxiadis, 1959a). A motive for this research toward solving the crisis in the design of the unplanned area in Block 10, which is not far from the block planning of the intact city of Al-Azhari, is maintenance of the aesthetic harmony and beauty of the area. The city planned comprehensive integration of all activities and various services, as well as social and human services (Abdallah and Fan, 2012). There are 18 mosques and a few corners, and only five primary schools located in blocks 11, 10, 15, 23, and 5. Another elementary school is under construction, and is already beginning to accept students. The completed rooms are without doors or windows. The average student’s walk to school is roughly 1 km. There is only one hospital in the region, and it is in building No. 15 (Elhassan et al., 2011a). The hospital receives medium cases, but it has no ambulance (Elhassan et al., 2011a; Abdallah and Fan, 2012). There is no market in the city because the city is located near Khartoum’s central market and local market. All organizational aspects of the city are funded publicly and through aid and donations from charitable societies and the citizens themselves (Elhassan et al., 2011a; Zeinab et al., 2012a). The city’s current situation does not meet the needs of a central modern city and does not meet the needs of citizens who require immediate assistance from public and state authorities. Blocks are similar in design and general form in network planning to the overall planning of Khartoum state (Doxiadis, 1959b). The services are extended from the first unit through to the center of the city. The city is divided into three classes, consisting of 29 blocks.
The first class represents 28.7% of the area, which consists of six blocks that comprise 5247 sections, of which 2098 sections are inhabited. The second class represents 13% of the city (Yakoub et al., 2019), consisting of seven blocks, including 8, 9, 25, 26, 27, 28, and 29, and has a total of 2469 sections and several populations that total 988 persons. The third class represents 58.7% of the city, as shown in Figure 1A, B; it consists of 16 blocks, 2469 sections, and 4398 inhabited sections (Zeinab et al., 2012a; Hamid and Bahreldin, 2013). The number of sections in the city is 18,710, and the inhabited neighborhoods comprise 38%–41% of all blocks (Elhassan et al., 2011a; Zeinab et al., 2012a). The average family size is six people (Zeinab et al., 2012b). The population of the entire city is approximately 52,388. Population statistics for the various classes and blocks have been reported by Zeinab et al. (2012a) and Saeed and Ali (2013).
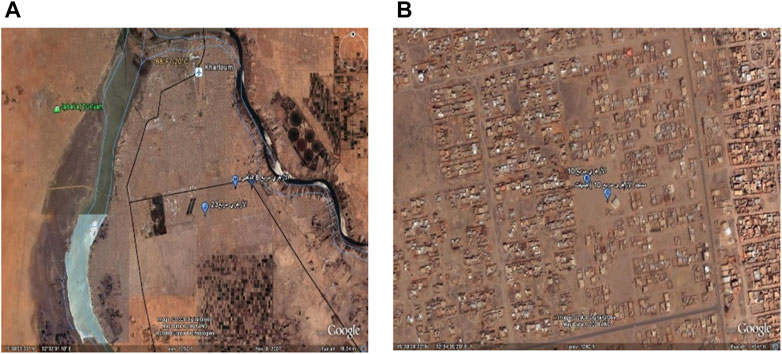
FIGURE 1. Satellite view of Al-Azhari City (suburban area of Khartoum). (A) Location of Al-Azhari City, Khartoum State. (B) Area of Azhari City Block 10.
3 Materials and methods
3.1 Design approach
This article considered climate and geographic location as major factors influencing home design (Givoni, 1992a). In the hot, dry climate, the house is kept cool in the summer and warm in the winter to conserve electricity (Ahmad et al., 2002).
3.1.1 Planning strategies and methods
The existing conditions in Khartoum result from socio-economic, political, and other forces (Ahmad, 1992; Mohammed, 2020). Since strategy deals with planning and implementation, the strategies of development are the focus of this article. Utilities, land, water, transportation, local resources for employment, and suitable living and working climates were all taken into consideration throughout the site selection process (Lindgren and Mukherjee, 1987; Givoni, 1992a). The main criteria of urban forms were vertical rather than horizontal by grouping clusters for shading, capturing cool breezes, and minimizing glare and solar heat reflection. Other design strategies included using water for cooling, using the courts for shading and ventilation of pedestrian networks confined to buildings or trees, and avoidance of large unshaded areas (Lindgren and Mukherjee, 1987; Lo et al., 2003). Optimally designed building materials were selected for house construction, including concrete floors with red brick walls (Elhassan et al., 2011a) as they provided even temperatures in the room and included a window for south-north thermal collection to absorb heat in the winter (Beck and Fowler, 2001; Koohsari et al., 2015).
3.1.2 Position and size of windows
Windows were strategically placed and sized depending on their orientation and the construction materials utilized in the house. Openable windows at strategic locations allowed for cross ventilation from the summer’s cooling breezes. Windows were either double-hung, casement, or sliding (Beck and Fowler, 2001), as shown in Figure 2.
The roof was well-developed enough to provide access to the sun in the winter while also sheltering the window from the sun in the summer when the angle is altered. The house architecture made excellent use of the shadows cast by external devices to reduce the interior temperature during the warm summer months (Beck and Fowler, 2001). North and west facing windows were in the shadow of the vertical sun protection devices all year (Pollock et al., 2009; Elhassan et al., 2011a), as shown in Figure 3. In the winter, high yield and good-quality windows did not allow heat loss (Elhassan et al., 2011a; Zeinab et al., 2012a), as detailed in the following sections.
3.1.3 Air movement and draught control
High-quality ventilation prevents common sources of heating air leaks. Ventilation was reduced during the day to exclude hot (Mohammed, 2020) and dust-laden outdoor air from the interior and was accomplished by the location of the windows (Ahmed et al., 2021). Well-mixed modeling around the building airflow was considered in CFD and simulations.
3.1.4 Computational simulation using IES (VE) v5.9.0.3 software
To conduct this case study, we chose to use Virtual Environment IES (VE) v5.9.0.3 as it provided the most flexibility in terms of model reuse across all study components. For the overheating analysis, the software had to estimate bulk airflow throughout the building, so the efficacy of the proposed ventilation method could be determined (Pollock et al., 2009). This part of the research utilized the MacroFlo module of the IES (VE) v5.9.0.3 in tandem with SunCast and the Apache simulation engine (Oleiwi et al., 2019). Advanced IE virtual modeling software was used in wind and ventilation simulations where the airflow around a building was modeled as well-mixed.
3.1.5 Modeling and simulation
The concept design layout for the Enterprise building was the first step in the process for this case study. A central courtyard was used in the original design to create natural ventilation (Elhassan et al., 2011a). As the warm air in the courtyard flowed to the roof, it generated cross ventilation, which allowed the building to be ventilated naturally. The aims of this case study were, first, to verify that the concept design was producing the desired results, and, second, to optimize the design for maximum efficiency (Roetzel, 2015). In the first phase, we examined the effectiveness of the building’s basic design by developing a model.
3.1.5.1 Ventilation
The simulations used the formulas of the national calculation method, which meant that a degree of control was allowed. Illumination energy decreased with increasing glazing area, while heating energy increased due to higher conduction losses and lower usable internal gains (Oleiwi et al., 2019). Following the energy analysis, a study of the building’s overheating and its effect on thermal comfort was conducted. The passive methods included an open chimney in the ceiling to draft the air and reveal a thermal mass, installation of external shade, and the opening of windows at night. In this research, the flow velocity and pattern of the outside air were predicted by creating a computational flow dynamics model (CFD) of the complete development. Therefore, one-story buildings were selected for in-house simulation (Oleiwi et al., 2019). Each of the CFD simulations produced detailed results that described air temperature and relative air flow velocity and pattern (Ramponi and Blocken, 2012). The macro flow was used to simulate the indoor environment. The displays presented in this report consist of three-dimensional views of the airflow pattern taken from either horizontal or vertical sections through the space (Tan and Wong, 2012). Most simulations were performed at a constant state temperature of 28°C in an isothermal environment. The apartment’s computational elements were all set to 0.2 m, whereas those nearer the buildings and the ground were all set to 1 m. The window diagram analysis used a northerly wind direction with a mean speed of 3 m/s as the external boundary condition (Jomehzadeh et al., 2017).
3.1.6 Detailed site description
The Ministry of Planning and Engineering in Khartoum, Sudan, was able to provide all available and essential data, including the plans of Al-Azhari City. The information was used to develop a blueprint for the development of Block 10 in Al-Azhari City, including suburban neighborhood homes (Elhassan et al., 2011a). The study developed a house design for a third-class urban area in Khartoum state by adopting the architectural requirements that are climatically appropriate (Zeinab et al., 2012a). The area of the family house was 200 m2 with an additional 100 m2 for the courtyard, which is a design that was also supported by Zeinab et al. (2012a), as shown in Figure 4A, B. The house plan was designed to accommodate 6–7 people. A comfortable year-round temperature in a home is inherently possible if the home is designed with the cooling effect of the site (hot, dry climate) in mind, as simulated in Table 1. Therefore, the design of the house allowed a significant amount of sunlight into the house throughout the winter to provide adequate internal heating, daylight, and natural ventilation for human needs (Elhassan et al., 2011a; Elhassan et al., 2011b; Zeinab et al., 2012a). In addition, the sunlight entering the house in summer was limited, as much as possible, to keep the interior cool. To this end, the architects and planners of the proposed site, Block 10, were sure to position the building so that it faced south, where it would receive the most sunlight and greatest benefit from the cooling effects of natural air circulation (Zhai et al., 2015). There was relatively unobstructed southern exposure, so the atmospheric air being heated by the sun could enter the house easily and make the interior cool during the summer season, similar to results reported by Elhassan et al. (2011a) and Yakoub et al. (2019). These effects of environment site planning allowed appropriate levels of natural ventilation and energy consumption for each dwelling, similar to results reported by Frampton (2015).
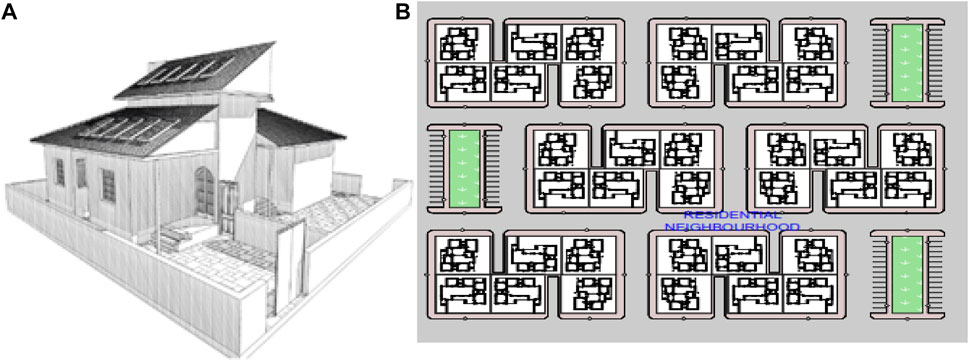
FIGURE 4. View of a designed residential house type designed in the Azhari City Block 10 area. (A) Perspective of a house. (B) Urban planning layout of Block 10.
4 Results and discussion
4.1 Low-energy house technology options at Al-Azhari City (an urban area of Khartoum state)
The design included wide windows with dimensions of 1.5 m × 1.5 m to cover the maximum area of the most indirect natural light of south and north exposure. By considering the cover on the roof and the refraction of the sunlight, according to the overall plan of the building, windows can be designed to selectively admit sunlight and distribute daylight throughout the day and year (Elhassan et al., 2011a; Elhassan et al., 2011b). Therefore, window orientations were integrated into the design to produce the optimal combination of light for the building, based on the northern climate and latitude of Khartoum state, for more effective natural ventilation (Zahnd and Kimber, 2009; Fuller and Taylor, 2010). The effect of the amount of light transmission through the windows was determined to be a main element affecting sustainable design and architecture, as well as the optimal design of buildings for low energy consumption (Nilsson and Roos, 2009; Doukas et al., 2012).
4.2 IES virtual environment (IES VE)
The IES Virtual Environment (IES VE) is a collection of applications that collaborate and have the same user interface and data model (Oleiwi et al., 2019). The software provides a framework for in-depth design evaluation, allowing structures and systems to be optimized in light of comfort and energy efficiency requirements (Chung and Ossen, 2012; Oleiwi et al., 2019). The virtual environment (VE) was used for the computer simulation chosen for this research as it allowed us to use a single component for all study requirements.
4.3 Weather data analysis
Khartoum Climate Design test data and the IESV software reference year were used to collect and analyze the weather data. The data used to create the IESV weather file were collected for a year to ensure that it represented the weather of a typical year (Amir et al., 2019). To collect and analyze weather data for Khartoum, the research utilized the Khartoum Climate Design test data and the IESV software reference year. The IESV weather file was created based on a year’s worth of data to represent typical weather conditions. This weather file was used as input for analyzing energy consumption and simulating the effects of local climate factors, such as temperature and wind, on building design.
4.3.1 Radiance energy plus integration
First, the simulation list used radiance to calculate daylight availability and lighting control response (Matsui et al., 2014); this information was then used as input to the energy plus model, as depicted in Figure 5. This is, in general, easily achieved by defining a light plan for the energy-plus model in the concentration, switching off the residents effect on daylighting simulation, employing energy-plus daylight calculation, and using the lighting schedule dictated by radiance-based simulation.
4.3.2 Air movement and ventilation
In hot and dry areas like Khartoum and Al-Azhari City, the outside air during the day is so hot that the movement of air into a building is not encouraged (Zeinab et al., 2012a). In this design, in the hot season of summer, cool air is found at night and nighttime ventilation is desirable to cool the structure of the building; this was detailed by using IESV software. As demonstrated in Figure 6, the sun at a high angle, high temperatures, and a moderate south airflow characterizes the summer months of March through June. The months of July to October, referred to as the rainy season, are usually colder than the rest of the year. In addition, air flowed down into the cabin while the heated interior air was exhausted. To prevent warming by day, a vent was opened for ventilation as there was no need for air movement during the day. The winter months (November–February) have the lowest solar angle and the mildest average temperatures. Furthermore, large windows were created to accommodate the climate conditions (Cheng et al., 2015) and contribute to optimal building design in Khartoum.
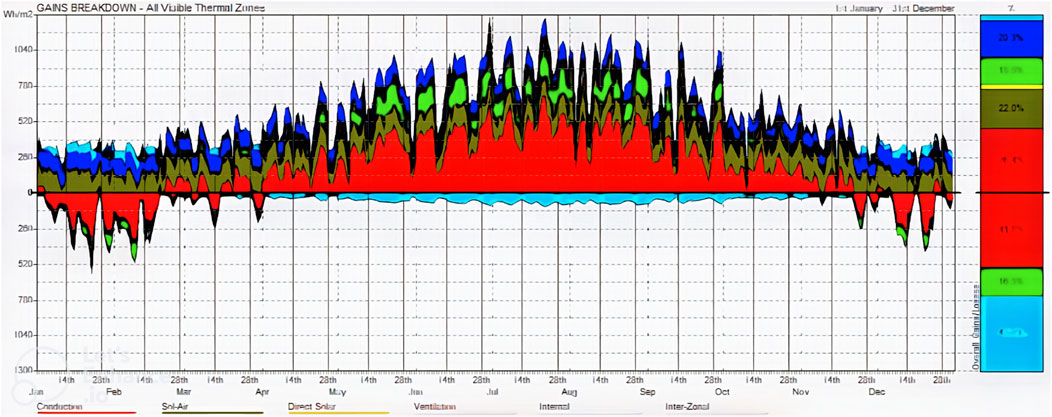
FIGURE 6. Gains breakdown in different thermal zones [w/m2]. Red: conduction; yellow: direct solar; green: ventilation; blue: internal gains; sky-blue: inter-zonal gains.
The designs considered for obtaining natural ventilation in buildings depended on the airflow in the area to reduce the temperature inside the building. Using IESV software, the annual air temperature was found to range from 21°C to 35°C, while the relative humidity ranged from 45 to 95%, except in July and August when it falls below 20%. This study utilized metropolitan Khartoum settings, which may be a crucial factor for natural ventilation design in Khartoum; it was comparable to results of studies by Givoni (1992b) and Karatasou et al. (2013), wherein similar natural ventilation maintained temperatures in the range of 30 °C–36 °C. At the site of the case study, buildings designed for hot and dry conditions had heavy structures. First study in urban areas for passive design using IVES and advanced simulations (Raja et al., 2001).
4.4 Inputs and assumptions for the simulation
To ensure consistency, all analyses were conducted using the same exterior constructions planned for the baseline building. In certain scenarios, bare concrete was used to finish interior ceilings instead of ceiling tiles to prevent overheating. The construction U-values employed in the study are presented in Figure 8. To accurately represent real-world usage, the study employed profiles and features that were representative of how the building would be utilized in practice. The study utilized occupancy profiles derived from the results of the investigation for analysis, and the activity database was used as the basis for internal heat gain assumptions. For the energy analysis, natural ventilation rates were determined by volume airflow analysis, replacing the minimum amount of fresh air required for 1 to 10 individuals, as in a previous study on overheating (Tantasavasdi et al., 2001). In indoor spaces, it is often assumed that the air is evenly mixed, the temperature and pollutants are distributed uniformly throughout the zone, and any impulse effects are not considered (Li and Li, 2015). Around-building airflow is considered in CFD, and simulations are used to achieve the most accurate results.
4.4.1 Cooling by natural ventilation in Al-Azhari City
In this design, the process of bringing natural air into occupied spaces, and offsetting heat and contaminants created by humans or brought into the space by outside air, is called ventilation. Indoor air quality is controlled by removing the outdoor element temperature. In these low-energy houses, the reduction in energy demand associated with cooling can be maximized by implementing natural ventilation. Natural ventilation was introduced into urban planning and architectural design to keep air movement at a comfortable level that would be suitable for the human body in summer (Heiskanen and Lovio, 2010).
4.4.2 Design and control of ventilation in a house
The design of the house was evaluated using IESV software while considering the architectural layout of the study location. The design of natural ventilation was optimized to ensure efficient airflow. Factors such as airflow path and window position were considered. The control of room temperature through natural ventilation relied on the prevailing wind patterns in Khartoum, which were north and south winds in winter and west and south winds throughout the year. The thermal wind was found to be the strongest, with a result of 41% in July and August, as shown in Figure 9. The results of the study varied depending on the prevailing wind patterns in different locations, leading to natural ventilation systems that constantly provided fresh air.
4.5 Boundary conditions
4.5.1 Atmospheric boundary conditions
All the simulations were performed under isothermal conditions, at an air temperature of 28°C, using conditions of the Khartoum state. The surrounding building and landscape coarseness were modeled using the method of Aynur et al. (2008), which is a tool for simulating airflow around buildings. The IESV model was utilized to investigate airflow within buildings. The accuracy and efficiency of the IESV model in studying airflow in and around buildings relied on two factors: the incoming wind to the building site and the size of the computational area. To achieve accurate results in a reasonable time frame, the following sections will cover how the headwind was simulated and the computational domain range was determined, which are critical for maximizing the computational efficiency of the IESV model.
4.5.2 Simulated wind
The on-site measurements and wind provided differing sets of data on the incoming wind, each using a 1/4 power law represent-wind profile and the same turbulent intensity. However, wind pressure variations across buildings and indoor airflow distributions are rarely consistent (Martilli, 2007). Differences in wind data could be attributed to the unpredictable ebb and flow of the wind. The natural wind’s speed and direction are highly unpredictable and challenging to reproduce in a wind tunnel (Negrao, 1995; Beausoleil-Morrison et al., 2001; Zhai et al., 2001). Natural wind changes direction and velocity over time, whereas traditional wind tunnels have fixed wind direction. The histogram for the simulated natural wind direction indicated a normal distribution at high wind speeds (4.46 m/s), consistent with the simulation results, but tended to be uniform at low wind speeds (1.17 m/s). Wind speed and direction at the Khartoum site are displayed. Xie and Castro (2009), and Jiang and Yoshie (2018) reported similar conclusions.
Using IESV, there were a variety of ways to simulate incoming flow, as natural wind and the macro flow from a wind tunnel have distinct properties. In the chase through the wind tunnel, IESV manipulated the wind’s direction. Figure 7 depicts the simple situation of a single location. Depending on the strength of the simulated wind, IESV generated a normal or uniform distribution (Pollock et al., 2009; Abdallah and Fan, 2012). Wang et al. (2017) found that the average change in wind direction over 15 min is roughly 80°. Time in the simulations was approximately 10–20 min, and the wind direction varied by 80°. On-site measurements showed that the wind speed ranged from 0.5 to 4 m/s just over the roof of the building. Below the roof, the wind speed was significantly less. This analysis, therefore, assumed that there was no discernible pattern to the varying incoming wind directions. According to the weather reports from Khartoum (Osman and Sevinc, 2019), which is closest to the development site, wind rose diagrams were drawn to show the amount or percentage of time out of the year that the wind was blowing from the north at an average speed of 3 m/s, as shown in Figure 8.
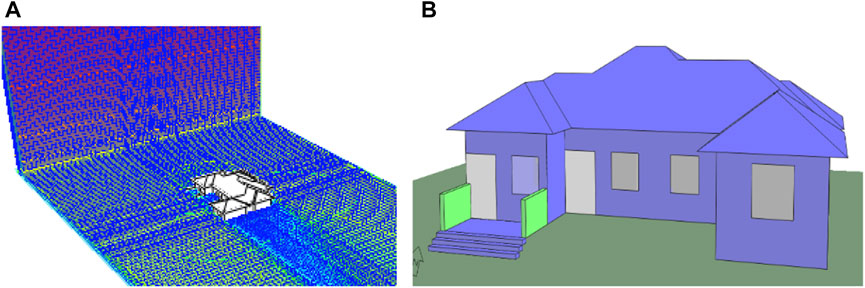
FIGURE 7. Wind analysis and related window size. (A) Wind analysis. (B) Size of windows matched to wind flow.
Based on the external CFD simulation of the entire development, the best, worst, and average units were identified and chosen for internal wind velocity ranging from 0.02 to 3 m/s. The spaces between the apartment blocks play an important role in providing negative pressure, which is required for the improvement of cross ventilation. The effect will enhance natural cross ventilation if there is sufficient window opening in both the front and back of the apartment. Refer to the following Figure 8.
The CFD simulations account for factors such as the location and size of the windows, the position of the building in relation to the wind, and the surrounding terrain. The simulations allow researchers to observe how the building design impacts the internal airflow and to adjust the design to optimize the air velocity for comfort and sustainability. CFD simulation methodology is widely accepted as a reliable and effective way to model the indoor environment and predict air velocity. This approach allows for a comprehensive and systematic evaluation of building design and its impact on the indoor environment, enabling researchers to make informed recommendations for the development of energy-efficient single-family houses that are comfortable and sustainable.
4.6 Analysis of the best- worst-, and average- case situations
4.6.1 Best-case situation
Based on CFD simulation, a one-story building has the best internal air velocity of approximately 0.7 m/s. The wind velocity starts to decrease toward the rear of the apartment spaces. Approximately 95% of the internal areas have an average air velocity between 0.43 m/s and 0.9 m/s, as shown in Figure 8. The internal airflow pattern for a single-floor house plan in Al-Azhari City is illustrated in Figure 8, which shows an internal air velocity of 0.7 m/s near open windows, as detailed in Figure 9, along with the wind directions and air pressure of the worst-case scenario, as shown in Figure 4.58. The air velocity and temperature (average of
4.6.2 Worst-case situation
According to the CFD simulation results, the eastern/western direction of a one-story building in Al-Azhari has the lowest air velocity. Approximately 80% of the internal space in this direction has air flow ranging from 0.05 m/s to 0.01 m/s, with a slightly higher flow of approximately 0.1 m/s near open windows. The absence of window openings on the east and west sides of the building means that the window openings located on the north and south sides are insufficient for improving cross ventilation in the eastern/western direction (Murga et al., 2020). This is illustrated in more detail in Figure 10.
This worst-case scenario highlights the importance of window size in determining the effectiveness of natural ventilation of a building. The lack of window openings on the eastern and western sides of the building resulted in low air flow and limited cross ventilation, highlighting the need for an adequate number and size of windows for effective ventilation. The results of this simulation stress the need for careful consideration of window placement and size during the design process to ensure optimal natural ventilation and improve indoor air quality.
4.6.3 CFD simulation situation
The surviving one-story building type has more windows opening to the north of the house. Approximately 60% of indoor spaces have an average indoor air velocity of 0.49 m/s. Results from a study by Fang et al. (2016) demonstrated the typical case situation in Malaysia, where the opposite unit with three stories or a semi-detached apartment type were able to obtain an average internal airflow velocity of 0.46 m/s near window openings.
4.7 Energy savings
This design, which includes modifications to improve the indoor environment, may increase the energy consumption of a building. Figure 11 illustrates the proportional breakdown of the energy consumption of a basic building. The simulation application IES Virtual Environment (VE) was utilized to run a series of sensitivity analyses on a collection of design parameters that had the potential to affect the building’s performance. The results revealed that the yearly energy savings as the primary objective were 71.1%. It is relatively simple to determine the optimal solution for a single analysis criterion based on the previous results. A design must meet an acceptable quality across all analyzed criteria. This selection can be aided by an integrated performance view that compares the characteristics of each design choice. The scenario that provides the finest overall performance can be determined from the fact that receiving the site indicates its performance. The saved energy is less than thermal energy that can be recovered in a naturally ventilated environment (Murga et al., 2020).
4.8 Validation
This research focused on analyzing the effects of physical diffusion on cross ventilation flow and validating CFD models of coupled outdoor wind flow and internal airflow for four different configurations of simple, isolated, single houses. However, it should be noted that the scope of the research has limitations, such as
• The effects of physical diffusion are subject to the same restrictions. Buildings located in suburban and urban areas, which are dominated by high-velocity wind jets through channels between buildings, are expected to experience at least the same, if not more, severe effects (Blocken and Carmeliet, 2007; Defraeye et al., 2012).
• The analysis only considered natural ventilation. Further research is needed to better understand how physical diffusion influences one-sided ventilation flow.
• The study was the first to examine the effects of physical diffusion on cross ventilation flow in the Khartoum area in such depth. There is no agreement in the scientific literature on how to obtain turbulent kinetic energy profiles from the recorded mean wind speed and turbulence intensity profiles; thus, understanding the effects of physical diffusion is crucial (Gousseau et al., 2011; Ray et al., 2014). We propose a parameter value of a = 1, which was found to be optimal in the current investigation.
• The computational grids for suburban and urban configurations are generally unstructured, and convergence can only be attained by introducing adequate diffusion through schemes that are specific for these types of configurations (Blocken and Carmeliet, 2007; Defraeye et al., 2012).
• The velocity-vector fields showed that the size of the upstream standing current was decreased, leading to increased spread of the jet inside the buildings. The impact of this change was most apparent along the central axis, between the apertures within the structure, while the effects were less pronounced outside the building.
It should be noted that, despite these limitations, this research provides valuable insights into the impacts of physical diffusion on cross ventilation flow in the Khartoum area.
4.9 Policies
Sudan is actively pursuing a more environmentally sustainable future. The country has implemented several initiatives to achieve this goal. One of the key initiatives is the development of renewable energy sources such as hydro, solar, and wind power. Sudan has made significant progress in this area and is now looking to expand its renewable energy sector (Agnolucci and Ekins, 2015). The government of Sudan is also working to conserve its forest resources. This includes the creation of national parks and wildlife reserves, as well as the implementation of policies aimed at preserving the country’s forests. Another important area of focus for Sudan is sustainable agriculture. The country is promoting practices such as the use of organic fertilizers, water conservation techniques, and agroforestry systems. This helps ensure that the country’s agriculture sector is both environmentally friendly and economically viable (Agnolucci and Ekins, 2015).
5 Conclusion
In conclusion, this research focused on designing an urban plan and appropriate solutions for implementing low-energy design and natural ventilation in residential buildings in Al-Azhari City, Khartoum, Sudan. The main finding was that the orientation and inclination of roof tilt greatly impacted results. The final design included energy-saving measures, such as natural ventilation, CFD simulation in a hot and dry climate, sustainable design, sustainable architecture, and low energy consumption for cooling. The high installation effort at the site was a fundamental aspect of this research. Based on the findings of this study on the design of low-energy residential buildings and natural ventilation in Al-Azhari City in Khartoum, Sudan, the following policies are suggested for use by the government to promote sustainable and green architecture:
• Promote the adoption of low-energy designs: The government should encourage and incentivize the use of low-energy designs in residential buildings, such as those successfully implemented in Al-Azhari City.
• Raise public awareness: The government could run public education campaigns to raise awareness about the benefits of natural ventilation and energy-efficient building design.
• Develop building codes and standards: The government could develop building codes and standards that require buildings to meet certain energy-efficiency and natural ventilation specifications. This would help ensure that all new buildings are designed and constructed in a sustainable and efficient manner.
The results of this research were dependent on orientation of the building and its windows to the north and south. Natural ventilation was used to cool the house and reduce energy consumption, particularly in Sudan’s hot climate. This study was the first research in Khartoum on designing clean, energy-efficient, and sustainable architecture. The results of this design indicated that a grid plan for Block 29 in Alazhari City was appropriate based on all spatial planning requirements at the site and in Khartoum state in general.
The results are consistent with previous studies that have focused on natural ventilation in similar climatic regions outside Sudan, with average air velocities between 0.43 and 0.9 m/s in 95% of the indoor areas. This study provides valuable insight into the potential for improving the indoor environment through proper design in Khartoum and similar hot and dry regions.
The findings of this study highlight the critical role of roof orientation and tilt angle in the performance of natural ventilation in residential buildings. The study focused on the design of a sustainable and energy-efficient house in Al-Azhari City in Khartoum, using solid brick as the base material and CFD simulation in hot and dry climates. The design incorporated natural ventilation, resulting in comfortable indoor temperatures and reduced energy consumption, especially during the summer months. The design process incorporated principles of energy-saving and green architecture, resulting in a low-energy house. The results of this study suggest the potential to implement similar designs in other regions and cities in Sudan. This study adds valuable insights to the field of sustainable architecture and emphasizes the importance of considering natural ventilation in residential building design to reduce energy consumption and promote sustainability.
Data availability statement
The datasets presented in this study can be found in online repositories. The names of the repository/repositories and accession number(s) can be found at: Not.
Author contributions
The author confirms being the sole contributor to this work and has approved it for publication.
Acknowledgments
The author would like to express special appreciation and thanks to the department of Interior Design—College of Architecture and Design—Prince Sultan University-KSA.
Conflict of interest
The authors declare that the research was conducted in the absence of any commercial or financial relationships that could be construed as a potential conflict of interest.
Publisher’s note
All claims expressed in this article are solely those of the authors and do not necessarily represent those of their affiliated organizations, or those of the publisher, the editors, and the reviewers. Any product that may be evaluated in this article, or claim that may be made by its manufacturer, is not guaranteed or endorsed by the publisher.
References
Abdallah, S., and Fan, I.-S. (2012). Framework for e-government assessment in developing countries: Case study from Sudan. Electron. Gov. Int. J. 9 (2), 158–177. doi:10.1504/eg.2012.046267
Agnolucci, J., and Ekins, P. (2015). Green policies and their impacts on the environment and the economy.
Ahmad, A. M., Sultan, A. E. I. M., Abdalla, M. M., Malik, A. O., Said, M. A., Mukhtar, E. S. M., et al. (2002). Low-cost housing projects in Khartoum with special focus on housing patterns. Habitat Int. 26 (2), 139–157. doi:10.1016/s0197-3975(01)00037-6
Ahmad, A. M. (1992). The neighbourhoods of Khartoum: Reflections on their functions, forms and future. Habitat Int. 16 (4), 27–45. doi:10.1016/0197-3975(92)90052-z
Ahmed, T., Kumar, P., and Mottet, L. (2021). Natural ventilation in warm climates: The challenges of thermal comfort, heatwave resilience and indoor air quality. Renew. Sustain. energy Rev. 138, 110669. doi:10.1016/j.rser.2020.110669
Ai, Z., and Mak, C. M. (2017). CFD simulation of flow in a long street canyon under a perpendicular wind direction: Evaluation of three computational settings. Build. Environ. 114, 293–306. doi:10.1016/j.buildenv.2016.12.032
Aldawoud, A. (2017). Windows design for maximum cross-ventilation in buildings. Adv. Build. energy Res. 11 (1), 67–86. doi:10.1080/17512549.2016.1138140
Amir, A., Mohamed, M. F., Sulaiman, M., and Yusoff, W. F. M. (2019). Assessment of indoor thermal condition of a low-cost single story detached house: A case study in Malaysia. Alam Cipta 12, 80–88.
Aynur, T. N., Hwang, Y., and Radermacher, R. (2008). Simulation evaluation of the ventilation effect on the performance of a VRV system in cooling mode—Part II, simulation evaluation. HVAC&R Res. 14 (5), 783–795. doi:10.1080/10789669.2008.10391039
Barbason, M., and Reiter, S. (2014). Coupling building energy simulation and computational fluid dynamics: Application to a two-storey house in a temperate climate. Build. Environ. 75, 30–39. doi:10.1016/j.buildenv.2014.01.012
Beausoleil-Morrison, I., Clarke, J. A., and Denev, J. (2001). Further developments in the conflation of CFD and building simulation.
Blocken, B., and Carmeliet, J. (2007). Validation of CFD simulations of wind-driven rain on a low-rise building facade. Build. Environ. 42 (7), 2530–2548. doi:10.1016/j.buildenv.2006.07.032
Cheng, Y., Lin, Z., and Fong, A. M. (2015). Effects of temperature and supply airflow rate on thermal comfort in a stratum-ventilated room. Build. Environ. 92, 269–277. doi:10.1016/j.buildenv.2015.04.036
Chung, L. P., and Ossen, D. R. (2012). Comparison of integrated environmental solutions< virtual environment> and autodesk ecotect simulation software accuracy with field measurement for temperature. Sustain. Built Environ. I, 85.
Cuce, E., Sher, F., Sadiq, H., Cuce, P. M., Guclu, T., and Besir, A. B. (2019). Sustainable ventilation strategies in buildings: CFD research. Sustain. Energy Technol. Assessments 36, 100540. doi:10.1016/j.seta.2019.100540
Davies, H., and Sin, M. A. (1991). “The heart of the Sudan,” in The future of Sudan’s capital region: A study in development and change, 1–10.
Defraeye, T., Blocken, B., Koninckx, E., Hespel, P., and Carmeliet, J. (2012). Aerodynamic study of different cyclist positions: CFD analysis and full-scale wind-tunnel tests. J. biomechanics 43 (7), 1262–1268. doi:10.1016/j.jbiomech.2010.01.025
Doukas, H., Papadopoulou, A., Savvakis, N., Tsoutsos, T., and Psarras, J. (2012). Assessing energy sustainability of rural communities using Principal Component Analysis. Renew. Sustain. Energy Rev. 16 (4), 1949–1957. doi:10.1016/j.rser.2012.01.018
Doxiadis, C. (1959). Khartoum: A long term programme and a master plan for the. Athens: Development of the Town.
Elhassan, Z. A. M., Zain, M. F. M., and Sopian, K. (2011). Optimum arrangement of photovoltaic systems in housing at Khartoum: Application of renewable energy in urban design. Urban Stud. Res. 2011. doi:10.1155/2011/983742
Elhassan, Z. A. M., Zain, M. F. M., Sopian, K., and Awadalla, A. (2011). Output energy of photovoltaic module directed at optimum slope angle in kuala lumpur, Malaysia. Res. J. Appl. Sci. 6 (2), 104–109. doi:10.3923/rjasci.2011.104.109
Elkheir, O. (2012). “The urban environment of Khartoum,” in Proceedings of national civic forum (NCF), Rio+ 20 civil society preparatory process.
Elnazir, R., and Feng, X.-z. (2004). Urban planning: A tool for urban poverty alleviation in Sudan. Chin. Geogr. Sci. 14 (2), 110–116. doi:10.1007/s11769-004-0017-z
Fang, Y., Fan, J., Kenneally, B., and Mooney, M. (2016). Air flow behavior and gas dispersion in the recirculation ventilation system of a twin-tunnel construction. Tunn. Undergr. space Technol. 58, 30–39. doi:10.1016/j.tust.2016.04.006
Frampton, K. (2015). A genealogy of modern architecture. Thames & Hudson, London, United Kingdom: Comparative Critical Analysis of Built Form.
Fuller, R., and Taylor, P. (2010). “Better definitions, better buildings?,” in Aubea 2010: Construction management (s): Proceedings of the 35th australasian universities building education association annual conference (Princeton, New Jersey: Princeton University Press).
Givoni, B. (1992). Climatic aspects of urban design in tropical regions. Atmos. Environ. Part B. Urban Atmos. 26 (3), 397–406. doi:10.1016/0957-1272(92)90015-k
Givoni, B. (1992). Comfort, climate analysis and building design guidelines. Energy Build. 18 (1), 11–23. doi:10.1016/0378-7788(92)90047-k
Gousseau, P., Blocken, B., Stathopoulos, T., and van Heijst, G. (2011). CFD simulation of near-field pollutant dispersion on a high-resolution grid: A case study by LES and RANS for a building group in downtown montreal. Atmos. Environ. 45 (2), 428–438. doi:10.1016/j.atmosenv.2010.09.065
Guo, W., Liu, X., and Yuan, X. (2015). Study on natural ventilation design optimization based on CFD simulation for green buildings. Procedia Eng. 121, 573–581. doi:10.1016/j.proeng.2015.08.1036
Hafazalla, A. A. (2008). “The urban development planning of greater Khartoum: Coping with urban dynamics,” in Urban housing in Sudan, proceedings of the architects’ third scientific conference (Khartoum, Sudan.
Hamid, G. M., and Bahreldin, I. Z. (2013). Environmental sustainability in Greater Khartoum between natural assets and human interventions. Int. J. Sustain. Build. Technol. Urban Dev. 4 (2), 100–110. doi:10.1080/2093761x.2013.801804
Hamid, G. M., and Bahreldin, I. Z. (2014). Khartoum 2030 towards an environmentally-sensitive vision for the development of greater Khartoum, Sudan. L'architettura delle città-the. J. Sci. Soc. Ludovico Quaroni 2 (3-4-5).
Hassan, S. S., Elkheir, O., and Kobylarczyk, J. (2017). Urban planning of Khartoum. History and modernity. Part I. History. Cham, Switzerland: Springer.
Heiskanen, E., and Lovio, R. (2010). User- producer interaction in housing energy innovations. J. Industrial Ecol. 14 (1), 91–102. doi:10.1111/j.1530-9290.2009.00196.x
Hong, S.-W., Exadaktylos, V., Lee, I. B., Amon, T., Youssef, A., Norton, T., et al. (2017). Validation of an open source CFD code to simulate natural ventilation for agricultural buildings. Comput. Electron. Agric. 138, 80–91. doi:10.1016/j.compag.2017.03.022
Jiang, G., and Yoshie, R. (2018). Large-eddy simulation of flow and pollutant dispersion in a 3D urban street model located in an unstable boundary layer. Build. Environ. 142, 47–57. doi:10.1016/j.buildenv.2018.06.015
Jomehzadeh, F., Nejat, P., Calautit, J. K., Yusof, M. B. M., Zaki, S. A., Hughes, B. R., et al. (2017). A review on windcatcher for passive cooling and natural ventilation in buildings, Part 1: Indoor air quality and thermal comfort assessment. Renew. Sustain. Energy Rev. 70, 736–756. doi:10.1016/j.rser.2016.11.254
Karatasou, S., Santamouris, M., and Geros, V. (2013). “Urban building climatology,” in Environmental design of urban buildings (Berlin, Germany: Springer), 119–143.
Koohsari, M. J., Mavoa, S., Villanueva, K., Sugiyama, T., Badland, H., Kaczynski, A. T., et al. (2015). Public open space, physical activity, urban design and public health: Concepts, methods and research agenda. Health & place 33, 75–82. doi:10.1016/j.healthplace.2015.02.009
Li, Y., and Li, X. (2015). “Natural ventilation potential of high-rise residential buildings in northern China using coupling thermal and airflow simulations,” in Building simulation (Springer).
Lindgren, I., and Mukherjee, D. (1987). On the connectivity criteria in the open-shell coupled-cluster theory for general model spaces. Phys. Rep. 151 (2), 93–127. doi:10.1016/0370-1573(87)90073-1
Lo, S., Yiu, C., and Lo, A. (2003). An analysis of attributes affecting urban open space design and their environmental implications. Manag. Environ. Qual. Int. J. 14, 604–614. doi:10.1108/14777830310495759
Martilli, A. (2007). Current research and future challenges in urban mesoscale modelling. Int. J. Climatol. A J. R. Meteorological Soc. 27 (14), 1909–1918. doi:10.1002/joc.1620
Matsui, T., Santanello, J., Shi, J. J., Tao, W. K., Wu, D., Peters-Lidard, C., et al. (2014). Introducing multisensor satellite radiance-based evaluation for regional Earth system modeling. J. Geophys. Res. Atmos. 119 (13), 8450–8475. doi:10.1002/2013jd021424
Mochida, A., and Lun, I. Y. (2008). Prediction of wind environment and thermal comfort at pedestrian level in urban area. J. wind Eng. industrial aerodynamics 96 (10-11), 1498–1527. doi:10.1016/j.jweia.2008.02.033
Mohammed, H. E. (2020). Evaluation of Sudan's experience with foreign urban planning consultancy: The case of greater Khartoum. Sudan Geogr. J. 2 (1).
Murga, A., Long, Z., Yoo, S. J., Sumiyoshi, E., and Ito, K. (2020). Decreasing inhaled contaminant dose of a factory worker through a hybrid Emergency Ventilation System: Performance evaluation in worst-case scenario. Energy Built Environ. 1 (3), 319–326. doi:10.1016/j.enbenv.2020.04.007
Negrao, C. O. R. (1995). Conflation of computational fluid dynamics and building thermal simulation. Berlin, Germany: Springer-Verlag.
Nilsson, A. M., and Roos, A. (2009). Evaluation of optical and thermal properties of coatings for energy efficient windows. Thin Solid Films 517 (10), 3173–3177. doi:10.1016/j.tsf.2008.11.083
Ohba, M., and Lun, I. (2010). “Overview of natural cross-ventilation studies and the latest simulation design tools used in building ventilation-related research,” in Advances in building energy research (Tokyo, Japan: Springer), 137–176.
Oleiwi, M. Q., Farid Mohamed, M., Khairul Azhar Mat Sulaiman, M., Irfan Che-Ani, A., and Raman, S. N. (2019). Thermal environment accuracy investigation of integrated environmental solutions-virtual environment (IES-VE) software for double-story house simulation in Malaysia. ARPN J. Eng. Appl. Sci. 14 (11), 3659–3665. doi:10.36478/jeasci.2019.3659.3665
Osman, M. M., and Sevinc, H. (2019). Adaptation of climate-responsive building design strategies and resilience to climate change in the hot/arid region of Khartoum, Sudan. Sustain. Cities Soc. 47, 101429. doi:10.1016/j.scs.2019.101429
Pollock, M., Roderick, Y., and McEwan, D. (2009). Building simulation as an assisting tool in designing an energy efficient building: A case study. Glasgow: Building Simulation.
Raja, I. A., Nicol, J., McCartney, K. J., and Humphreys, M. A. (2001). Thermal comfort: Use of controls in naturally ventilated buildings. Energy Build. 33 (3), 235–244. doi:10.1016/s0378-7788(00)00087-6
Ramponi, R., and Blocken, B. (2012). CFD simulation of cross-ventilation flow for different isolated building configurations: Validation with wind tunnel measurements and analysis of physical and numerical diffusion effects. J. Wind Eng. Industrial Aerodynamics 104, 408–418. doi:10.1016/j.jweia.2012.02.005
Ray, S. D., Gong, N. W., Glicksman, L. R., and Paradiso, J. A. (2014). Experimental characterization of full-scale naturally ventilated atrium and validation of CFD simulations. Energy Build. 69, 285–291. doi:10.1016/j.enbuild.2013.11.018
Roetzel, A. (2015). “Occupant behaviour simulation for cellular offices in early design stages—architectural and modelling considerations,” in Building simulation (Springer).
Saeed, A. M., and Ali, D. E. (2013). Sustainability of the livelihood strategies of the internally displaced residents of Soba El Aradi settlement in Khartoum State, Sudan.
Shirzadi, M., Mirzaei, P. A., and Naghashzadegan, M. (2018). Development of an adaptive discharge coefficient to improve the accuracy of cross-ventilation airflow calculation in building energy simulation tools. Build. Environ. 127, 277–290. doi:10.1016/j.buildenv.2017.10.019
Tan, A. Y. K., and Wong, N. H. (2012). Natural ventilation performance of classroom with solar chimney system. Energy Build. 53, 19–27. doi:10.1016/j.enbuild.2012.06.010
Tantasavasdi, C., Srebric, J., and Chen, Q. (2001). Natural ventilation design for houses in Thailand. Energy Build. 33 (8), 815–824. doi:10.1016/s0378-7788(01)00073-1
Wang, H., Liu, Y., Cheng, W. Y. Y., Zhao, T., Xu, M., Liu, Y., et al. (2017). Improving lightning and precipitation prediction of severe convection using lightning data assimilation with NCAR WRF-RTFDDA. J. Geophys. Res. Atmos. 122 (2212), 12,296–12,316. doi:10.1002/2017jd027340
Wang, L., Robertson, A., Kim, J., Jang, H., Shen, Z. R., Koop, A., et al. (2022). Validation of CFD simulations of the moored DeepCwind offshore wind semisubmersible in irregular waves. Ocean. Eng. 260, 112028. doi:10.1016/j.oceaneng.2022.112028
Wesseling, P. (2009). Principles of computational fluid dynamics, 29. Springer Science & Business Media.
Xie, Z.-T., and Castro, I. P. (2009). Large-eddy simulation for flow and dispersion in urban streets. Atmos. Environ. 43 (13), 2174–2185. doi:10.1016/j.atmosenv.2009.01.016
Yakoub, W. A., Mahmoud, M. F., and Eleinen, O. M. A. (2019). “Urbanism as it should be: An overview of environmental urban movements and initiatives towards sustainability,” in Proceedings of the second international conference (tenth conference of sustainable environmental development) (Egypt: Sharm El Sheikh).
Zahnd, A., and Kimber, H. M. (2009). Benefits from a renewable energy village electrification system. Renew. Energy 34 (2), 362–368. doi:10.1016/j.renene.2008.05.011
Zeinab, A. M. E., Muhammad, F. M. Z., and Kamaruzzaman, S. (2012). Building integrated photovoltaics (BIPV) module in urban housing in Khartoum: Concept and design considerations. Int. J. Phys. Sci. 7 (3), 487–494.
Zeinab, A. M. E., Muhammad, F. M. Z., and Kamaruzzaman, S. (2012). Design and performance of photovoltaic power system as a renewable energy source for residential in Khartoum. Int. J. Phys. Sci. 7 (25), 4036–4042. doi:10.5897/IJPS11.346
Zhai, Z., Chen, Q., Klems, J. H., and Haves, P. (2001). Strategies for coupling energy simulation and computational fluid dynamics programs.
Zhai, Z. (2006). Application of computational fluid dynamics in building design: Aspects and trends. Indoor built Environ. 15 (4), 305–313. doi:10.1177/1420326x06067336
Zhai, Z. J., El Mankibi, M., and Zoubir, A. (2015). Review of natural ventilation models. Energy Procedia 78, 2700–2705. doi:10.1016/j.egypro.2015.11.355
Keywords: CFD—computational fluid dynamics modeling, passive house, air conditioning, energy consumption, urban, rural
Citation: Elhassan ZA (2023) Energy consumption performance using natural ventilation in dwelling design and CFD simulation in a hot dry climate: A case study in Sudan. Front. Built Environ. 9:1145747. doi: 10.3389/fbuil.2023.1145747
Received: 16 January 2023; Accepted: 07 February 2023;
Published: 08 March 2023.
Edited by:
Alfrendo Satyanaga, Nazarbayev University, KazakhstanReviewed by:
Roberto Alonso González-Lezcano, CEU San Pablo University, SpainRobby Yussac Tallar, Universitas Kristen Maranatha, Indonesia
Copyright © 2023 Elhassan. This is an open-access article distributed under the terms of the Creative Commons Attribution License (CC BY). The use, distribution or reproduction in other forums is permitted, provided the original author(s) and the copyright owner(s) are credited and that the original publication in this journal is cited, in accordance with accepted academic practice. No use, distribution or reproduction is permitted which does not comply with these terms.
*Correspondence: Zeinab A. Elhassan, emFiZGFsbGFoQHBzdS5lZHUuc2E=
†ORCID: Zeinab A. Elhassan, orcid.org/0009-0004-2652-4109