- 1Department of Civil Engineering, Faculty of Engineering, Sakarya University, Sakarya, Türkiye
- 2Department of Civil Engineering, Graduate Education Institute, Sakarya University of Applied Science, Sakarya, Türkiye
- 3Department of Civil Engineering, Faculty of Engineering, Istanbul University-Cerrahpasa, İstanbul, Türkiye
Transportation structures that provide rescue, first aid, and emergency services after an earthquake are critical for ensuring mobility within affected regions. The Tarsus–Adana–Gaziantep (TAG) motorway, passing through the Gaziantep Nurdağı district, is one of the most important transportation arteries in the earthquake-affected region. This study aims to assess the earthquake performance of transportation infrastructures along the TAG motorway, which is under the authority of the 5th Regional Directorate of the General Directorate of Highways (KGM). In this study, transportation structures such as viaducts, underpasses, overpasses, road retainers, drainage systems, and traffic safety elements along the TAG motorway were inspected and documented through on-site photography. The coordinates of these structures were determined using Global Navigation Satellite Systems (GNSS). The collected data was integrated with earthquake data from the Ministry of Internal Affairs Disaster and Emergency Management Presidency (AFAD) and analyzed using Geographic Information System (GIS) software. The inspection of the TAG motorway revealed that it is a vital transportation route connecting airports, state roads, and urban networks. Significant deformations were observed in the transportation structures, especially in the province of Hatay, where the road leading to the city’s airport was notably affected by the earthquake. Furthermore, the study also evaluated the impact on the transportation networks and traffic characteristics of Gaziantep and Hatay provinces. The findings highlight the vulnerability of transportation infrastructures in earthquake-prone areas and the importance of assessing and reinforcing these structures to ensure functionality during future seismic events. The study provides valuable data that can be used to improve the design and reinforcement strategies for transportation structures to withstand earthquakes.
1 Introduction
On 6 February, 2023, two earthquakes with magnitudes of MW: 7.7 and MW: 7.6 occurred in Türkiye at 04:17 and 13:27 local time with epicenters at Pazarcık-Kahramanmaras (N37.288°, E37.043°) and Elbistan-Kahramanmaras (N38.089°, E37.239°), respectively. The 7.7 magnitude earthquake occurred at a focal depth of 8.6 km and was located approximately 40 km northwest of Gaziantep and approximately 33 km southeast of Kahramanmaras. The 7.6 magnitude earthquake occurred at a depth of 7 km under the ground and was located approximately 98 km northwest of Adıyaman and 62 km northeast of Kahramanmaras (AFAD Disaster and Emergency Management Presidency, 2023).
As of 18/02/2023, a total of 7,451 aftershocks occurred in the region approximately 200 km from the epicenter—433 with magnitudes exceeding Mw 5.0. As the aftershocks of these earthquakes continue, a triggered earthquake occurred on 20 February, 2023 at Yayladagı (Hatay) of MW: 6.4. All these earthquakes caused a very severe loss of life and damage to the regional cities of Kahramanmaras, Hatay, Adıyaman, Gaziantep, Malatya, Kilis, Diyarbakır, Adana, Osmaniye, Sanlıurfa, and Elazıg. As would be expected, earthquakes of these magnitudes may result in a huge number of people being dead or injured. Official figures state that 50,783 people died and 115,353 people were injured, most of them with lifelong physical inabilities. Along with all these direct human related effects, the Turkish Presidential Strategy and Budget Directorate reported that 35,355 buildings had collapsed, 17,491 buildings needed urgent demolition, and 179,786, 1,712,182, and 431,421 buildings were severely, medium, and slightly damaged, respectively, causing over 100 billion dollars of economic loss (METU Orta Doğu Teknik Üniversitesi, 2023). Among the collapsed or severely damaged residential buildings were historical, administrative cultural buildings, schools, hospitals, and hotels affected by the earthquake, from collapse to minor damage (TMMOB Union of Chambers of Turkish Engineers and Architects, 2025).
The seismotectonic structure of the region is shaped by the complex interactions between the Anatolian, Arabian, and Eurasian Plates. Two major fault systems dominate this region: the North Anatolian Fault Zone (NAFZ) and the East Anatolian Fault Zone (EAFZ). The North Anatolian Fault is a right-lateral strike–slip fault that accommodates the westward movement of the Anatolian Plate. The East Anatolian Fault is a left-lateral strike–slip fault that forms the boundary between the Anatolian and Arabian Plates. The movement of these plates generates significant seismic activity in the region.
The earthquake that occurred in 2023 was associated with the East Anatolian Fault. In this region, the northward movement of the Arabian Plate led to the accumulation of stress along plate boundaries, which eventually resulted in a sudden rupture along the fault line. Due to its shallow depth, the earthquake caused intense ground shaking over a vast area, leading to widespread destruction. The presence of active fault lines and the history of past major earthquakes indicate that such seismic events are likely to recur in the future.
Historically, significant earthquakes occurred along the East Anatolian Fault in 1822, 1905, and 2020 (Chen et al., 2020). These seismic events suggest that stress accumulates along the fault over time and is released periodically. The 2023 earthquake can be considered a result of this process.
Figure 1 below illustrates the active fault lines, the earthquakes that occurred, a bird-eye view distance of the region to the epicenter of the 7.6 MW earthquake.
This paper mainly focuses on the earthquake impact evaluation of Gaziantep, Kahramanmaras, and Hatay provinces by analysing their transportation infrastructure and traffic characteristics. Thus, viaducts, underpasses, overpasses, road bodies, road retainers, and drainage structures along with traffic safety elements in the sections of the TAG (Tarsus–Adana–Gaziantep) motorway, located within the borders of Gaziantep province and passing through the Nurdagi district were examined. The deformations arose on the highway connecting city of Hatay to the airport were also examined. The field research was carried out between 20 and 26 February, 2023 to collect the data related to the Tarsus–Adana–Gaziantep (TAG) Highway which passes through the Nurdagı District of Gaziantep and is under the authority of the 5th Regional Directorate of the General Directorate of Highways (KGM).
2 Literature review
Infrastructure resilience after earthquakes is of critical importance, especially for the rapid reconstruction of transportation systems. Transportation infrastructure plays a vital role in the conduct of emergency response activities, ensuring smooth evacuation processes, and maintaining economic activities following natural disasters like earthquakes.
Kumar et al. (2017) analyzed the resilience of transportation infrastructure after earthquakes and examined the effects of bridge and road collapses on transportation systems in detail. They also emphasized the necessity of emergency action plans for the reconstruction of damaged bridges and roads. The findings bear similarities to the post-earthquake situation of the TAG Motorway in Türkiye regarding its structural problems raised in this research.
Fukushima et al. (2018) examined the improvements performed after the 2011 Tōhoku earthquake in Japan, highlighting the role of engineering solutions in increasing infrastructure resilience. The study particularly emphasized the effectiveness of seismic isolators used in bridges to minimize earthquake-induced damage. Japan’s engineering approaches may serve as an example for earthquake-prone countries like Türkiye.
Miyamoto (2000) discussed infrastructure improvement following the 1995 Kobe earthquake. After the severe damage to transportation infrastructure during that earthquake, new engineering methods and planning strategies that could be applied in Türkiye were developed.
Tuzun et al. (2019) evaluated post-earthquake transportation infrastructures in Türkiye by examining the damage caused in various cities. They particularly focused on the severity of bridge damage and proposed solutions for their repair.
Yılmaz et al. (2021) proposed strategies to enhance the seismic resilience of transportation projects in Türkiye. They study emphasized the need to review the seismic design standards for infrastructure projects in high-risk areas. Additionally, they recommended the integration of new technologies and engineering solutions for the rapid repair of earthquake-damaged roads and bridges.
Seismic design and engineering solutions play a fundamental role in developing earthquake-resistant infrastructure systems. Bertero (1992) evaluated the effectiveness of seismic design practices in California. Çelik et al. (2022) detailed engineering solutions that could be applied to improve the seismic resilience of transportation infrastructure.
Aydın and Güler (2018) assessed the seismic performance of highway bridges in Türkiye, presenting key findings on bridge resilience. Their study highlighted the effectiveness of seismic isolators in bridges and emphasized the need for their widespread use. Karaca and Duman (2021) conducted a comprehensive evaluation of the seismic performance of urban bridges, emphasising the necessity to revise seismic design standards to prevent structural damage.
The rapid reconstruction of transportation systems after an earthquake is critical for the recovery process in general. Sönmez and Yıldırım (2020) emphasized the importance of quickly rebuilding transportation systems following a disaster. Liu and Wang (2019) analyzed infrastructure damage after the 2008 Sichuan earthquake in China and proposed various strategies to enhance resilience.
Smith et al. (2016) examined post-earthquake infrastructure repair processes by evaluating their effectiveness. They highlighted the efficiency of the technologies used in repair processes and stressed the need for their widespread adoption. Johnson et al. (2018) focused on infrastructure planning in high-risk seismic areas and developed planning strategies. Their research suggested the reassessment of seismic design standards for infrastructure projects in earthquake-prone regions.
GNSS-based deformation analysis is an effective method for monitoring and evaluating infrastructure deformations after earthquakes. Huang and Chen (2019) assessed the seismic risks of urban transportation systems in Beijing using GNSS-based methods, and it was also used as the methodological framework for analysing deformations on the TAG Motorway and Hatay Airport.
Salgado and Silva (2021) presented a framework for evaluating the resilience of urban transportation systems, emphasizing the importance of GNSS-based analyses. Mehta and Raghavan (2020) examined infrastructure vulnerability and resilience in the face of disasters, discussing the role of GNSS-based analyses. Wilson et al. (2021) evaluated the role of technology in post-earthquake infrastructure resilience, emphasizing the effectiveness of GNSS-based methods. They study highlighted the importance of these analyses in improving infrastructure resilience, and they recommended their widespread implementation.
International experiences and applications serve as a valuable guide for post-earthquake infrastructure improvement efforts. Gonzalez et al. (2020) evaluated infrastructure rehabilitation efforts following earthquakes in Mexico and discussed sustainable infrastructure development methods. Gibbons and McCarthy (2020) assessed global earthquake engineering applications by examining successful cases in different countries.
Khalil et al. (2021) addressed earthquake risks in the Middle East and proposed recommendations for strengthening the region’s infrastructure. Their study suggested the reassessment of seismic design standards for infrastructure projects in high-risk seismic areas. Elnashai and Di Sarno (2008) examined the fundamental principles of earthquake engineering and compared strategies applied worldwide. They emphasized the effectiveness of earthquake engineering applications and recommended their use.
The economic and social impacts of post-earthquake infrastructure rehabilitation should also be considered. Brown et al. (2019) analyzed financing models for post-earthquake infrastructure projects and evaluated their effectiveness. They discussed various financing models that could be used for infrastructure rehabilitation after an earthquake.
Taylor et al. (2020) examined the social impacts of post-earthquake infrastructure projects, emphasizing the importance of public participation. Their study suggested that public involvement in post-earthquake infrastructure rehabilitation would enhance effectiveness. Anderson et al. (2022) explored public–private partnership models for post-earthquake infrastructure rehabilitation and argued that infrastructure rehabilitation could be more effectively managed through public–private partnerships.
Lee et al. (2024) analyzed the environmental impacts of post-earthquake infrastructure rehabilitation projects and proposed sustainable solutions. Their study recommended various strategies to minimize the environmental impact of post-earthquake infrastructure rehabilitation efforts.
The TAG Motorway is a critical transportation axis in south-eastern Türkiye; enhancing its seismic resilience is of utmost importance. This study investigates the structural and operational performance of the regional transportation network in the area profoundly affected by the earthquakes. The TAG motorway is at the core focus of the regional network. As this study involves only immediate analysis of the network, data collected through a time gap regarding the physical conditions of the structures was not taken into consideration for the analysis. Those related data will be analyzed in the future studies by obtaining them from the official state organizations. Such analysis will be significant in sustaining the resilience of the entire regional transportation network.
The following section explains the process employed for the detail analysis of the infrastructure elements and the road network performances right after the earthquake regarding the provision of the acceptable level of service which was vital for an effective access to the region.
3 Methodology
The level of deformation on the roads and engineering structures in the study area was examined using the precise location data obtained through a GNSS (global navigation satellite systems) device and the correction broadcast of the TUSAGA-AKTİF (CORS-TR) system.
TUSAGA-AKTİF (CORS-TR), consisting of 158 fixed GNSS stations, operated jointly by the Turkish General Directorate of Land Registry and Cadastre (TKGM) and the Turkish General Directorate of Mapping (HGM), is used to obtain locational information within couple of seconds without requiring reference and polygon points in places where a sufficient number of GNSS satellites can be seen and communication facilities are possible (Cankurt et al., 2019). Following the earthquakes, new coordinates were defined by TKGM to the system by calculating the coordinate changes of some fixed stations belonging to the TUSAGA-AKTİF (CORS-TR) system in the region. Those coordinates were available free of charge with the effect of 10 February, 2023 in order to ensure coordinate unity in the region (TKGM, 2023).
In this study, field measurements were carried out along the TAG Motorway, the access road for Hatay Airport, and viaducts using Trimble R10 brand high precision GNSS receivers. The real time kinematic (RTK) method was used to increase the accuracy of GNSS data and minimize signal errors. RTK corrections were made by employing TUSAGA-AKTIF base stations with the obtained average accuracy at the level of ±2 cm. Thanks to this process, error sources in raw GNSS signals (ionospheric and tropospheric delays, clock errors, etc.) are corrected and precise position information is obtained for fixed or mobile receivers. During the measurements, attention was paid to tall buildings, trees, and other obstacles to prevent errors due to signal reflection (multipath effect). GNSS measurements performed by using the WGS84 (EPSG: 4326) coordinate reference system were converted to the ITRF96/TUREF (EPSG: 5266) coordinate reference system to be used in GIS (geographic information system) analyses. These data were then transferred to ArcGIS Pro software to perform spatial analyses in the GIS environment.
ArcGIS Pro GIS software was used to analyze the post-earthquake deformations and damage distributions in the transportation infrastructure by employing GNSS measurements. At this stage, vector maps were created for the roads in the field where the measurements were taken to establish the horizontal geometries of the viaducts and bridges on the motorway along with the airport road. The horizontal distances of the measured areas to the epicenter points of the earthquake were determined and illustrated on the map. Regions where the highest damage occurred were thus determined and the spatial distribution maps for the earthquakes were obtained by transferring the coordinate data provided by AFAD to the ArcGIS Pro environment.
GNSS data obtained especially along the Hatay Airport access road were transferred to the NetCAD program to analyze the deformations in the road geometry for both directions.
4 Analysis
Proximity analysis is a basic analysis method used to evaluate the spatial relationships and distances between elements in a geographic data set. In this study, elevation changes along the Hatay airport access road were analyzed using GNSS measurements in order to determine the spatial distribution of post-earthquake road deformations. The elevation values obtained with GNSS devices were measured at certain intervals and evaluated to determine the vertical movements occurring along the road. Various spatial statistics methods were applied within the context of this research to determine the spatial patterns of road deformations along with settlement or uplift trends to understand whether these changes are systematic.
Data obtained from the measurements along the Hatay Airport Road were transferred to NetCAD software to create separate profiles for the inbound and outbound directions in the vertical and horizontal axes to analyze the elevation changes. ArcGIS Pro’s Optimized Hot Spot Analysis tool was used to determine spatial clustering among deformation values. This analysis allowed the determination of spatial distribution by classifying the vertical changes (settlement or rise) as hot and cold spots that occurred along the road after the earthquake.
In addition, the infrastructure of the transportation network in the region, especially the viaducts on TAG motorway, were investigated regarding their post-earthquake performance, as explained below.
4.1 TAG motorway
The TAG motorway is part of the 230-km-long O-52 motorway connecting Adana–Osmaniye–Kahramanmaras–Gaziantep and Sanlıurfa; it was constructed with public resources between April 1993 and June 1998 as the largest infrastructure investment of its period.
This motorway provides two-way directions of movement and has six lanes with three at each direction. This motorway has 17 interchanges, 14 viaducts, and 5 tunnels with a total length of 5232 m and 5352 m, respectively. Figure 2 presents the motorways available in Türkiye.
As this sketch-like image was obtained from KGM website, it was not possible to place the coordinate grid on it due to the fact that a coordinate reference system needs to be defined.
The Atatürk Viaduct, the longest of the 14 viaducts on the TAG motorway, is the second longest in Europe and the longest in Türkiye at the time it was built, with a length of 801.5 m and height of 149.5 m.
One of the most important features of the TAG motorway is its long-span continuous steel viaducts. In order to provide protection against corrosion throughout the life of the project without the need for painting, corten-type structural steel was used in these viaducts’ construction (AFAD Disaster and Emergency Management Presidency, 2023; Anadolu Agency, 2023).
Five viaducts were investigated on TAG motorway in the vicinity of Nurdagi province:
• Sehitler Viaduct (310 m)
•Nurdagi Viaduct (266 m)
•Ataturk Viaduct (801 m)
•Turgut Ozal Viaduct (424 m)
•Baspinar Viaduct (210 m)
Considering the fault lines taken from the AFAD official website and represented in different colors according to their sizes through GIS software, the distance of these examined viaducts to the epicenter of the earthquake at 04:17, 6 February, 2023 in Kahramanmaras Pazarcık with a magnitude of 7.7 MW was approximately 28 km. The approximate distance from the epicenter of the earthquake which occurred in Gaziantep Nurdagi with a magnitude of 6.6 MW was 20 km. These were 108 km from the Elbistan earthquake with a magnitude of 7.6 MW.
Figure 3 below displays the distribution of these five viaducts on the TAG motorway around Nurdagi and the general view of the D.400 State Highway, starting from Datca district of Mugla on the Aegean coast and ending at the Esendere border gate in Hakkari’s Yuksekova district. The D.825 State Highway passing through Nurdagi is also illustrated in Figure 3. These viaducts from west to east are the Sehitler, Nurdagi, Ataturk, Turgut Ozal, and Baspınar Viaducts.
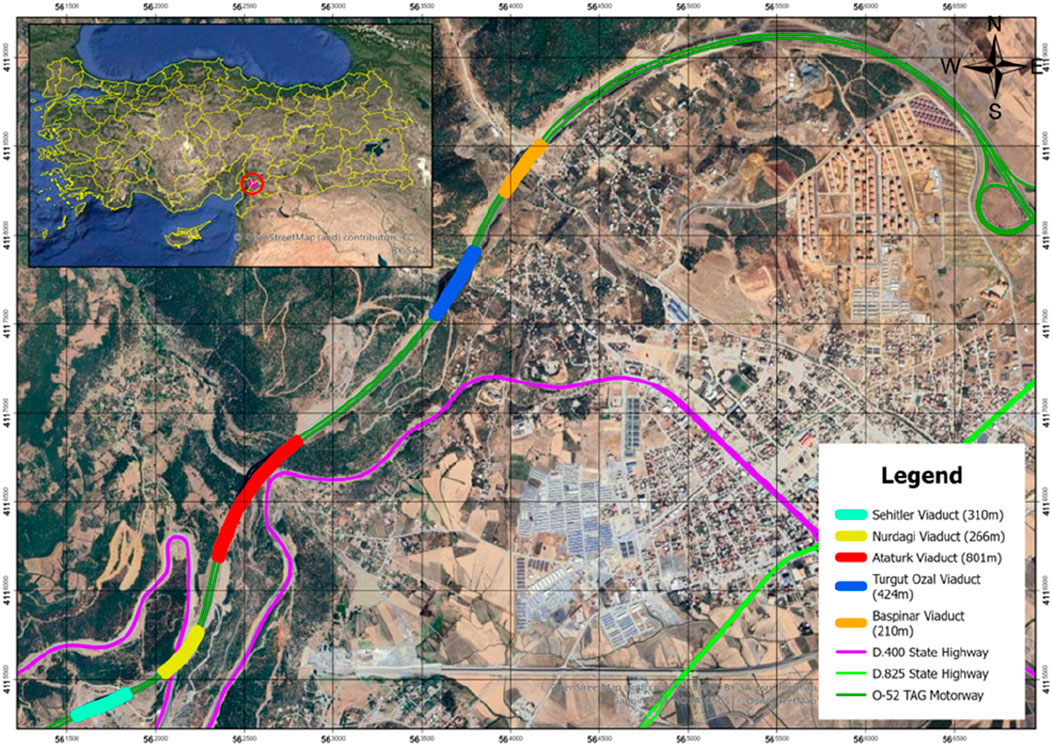
Figure 3. General view of the study area, TAG Motorway Nurdagi Viaducts along with D.400 and D.825 state highways.
Figure 4 below illustrates the view of the Nurdagi viaducts from the direction of Gaziantep–Osmaniye on the TAG motorway.
Each transportation structure within the study area in Figure 4 was examined and photographed on site, and its coordinates were collected using a GNSS device. These data were combined with earthquake data obtained from the official website of the Ministry of Internal Affairs, Disaster and Emergency Management Presidency (AFAD) and spatially analyzed using geographical information system software. The structural deformations detected in the field were examined in detail in the data evaluation table. In the light of all these findings obtained as a result of the field study, evaluations were carried out regarding the structural damages that occurred to propose the precautions that should be taken before any future earthquakes.
Earthquake-related highway deterioration manifests as cracks in the flexible superstructure (asphalt) of the road, deformations on the surface (settlement and collapse, cave-ins, and splitting of the road body), damage to engineering structures and deformation of traffic safety elements. These can cause costly damage to vehicles and be life-threatening for drivers and passengers. Furthermore, damage to the transportation system may prevent the timely delivery of first aid, rescue, and emergency services after an earthquake. The repair process, usually organized by government agencies, begins with emergency repairs and is followed by permanent ones. Alternative route suggestions can also be taken into consideration in this process to ensure the usability of a road as quickly as possible.
The following sections explain the findings of different deficiencies and negative impacts of the earthquake on the transportation network available in the region studied.
4.1.1 Stretch of road collapses and subsidence: deformation in road protective structures
In the main body of the TAG motorway, no serious subsidence problems occurred due to the rigid structure of the viaducts. Although good performance was thus observed, some collapses occurred through some sections of the motorway with the earthquake effect. When viewed from this perspective, it was observed that 50–60 cm collapses occurred at a scale that caused structural problems. These collapses were around the rainwater down-pipe and were effective along approximately 200 m of the road section. In addition, damage occurred in the intercepting ditches, side ditches, and support structures related to subsidence problems. Furthermore, gaps of up to 30 cm were measured between the road pavement and the side ditch (Figure 5). This damage necessitated the closure of the related sections of the motorway to the traffic on the first day of the earthquake, except for emergency vehicles.
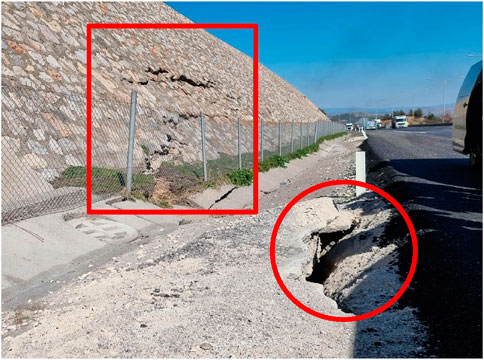
Figure 5. Collapses and applied emergency repairs on the motorway [Latitude: 37.183063–Longitude: 36.710260].
For the repair of the main road structure, a surface course was laid to re-open the highway and viaduct sections to serve to normal traffic on the second day.
4.1.2 Cracks and collapses in the road body
Collapses occurred in the road body as a result of the deterioration of the road infrastructure due to the earthquake movement and fault effect. Slight collapses such as shown in Figure 6 were repaired, and the roadway was opened to traffic as a result of temporary measures taken by the General Directorate of Highways within the first 24 h after the earthquake.
4.1.3 Deformation on road protective structures
Due to earthquake movements, cracks and displacements occurred in the stone retaining wall on the mixed section of the road consisting of cut and fill (Figure 7). Due to deformation, the stone retaining wall was damaged and lost its functionality. It was observed that no repair work was carried on cracks and deformations as they were not considered within the scope of emergency repair. In order to ensure road safety, it is necessary to repair the stone wall within the scope of permanent repairs after emergency repairs.
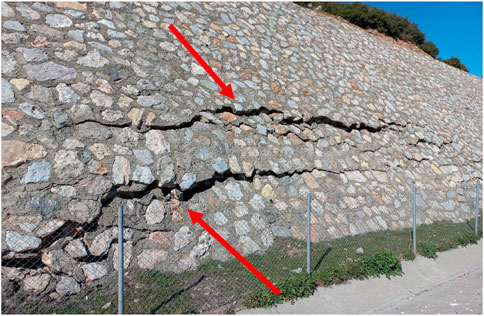
Figure 7. Deformation on road protective structures (retaining wall) between Ataturk and Turgut Ozal Viaducts [ Latitude: 37.183063–Longitude: 36.710260].
4.1.4 Clogging in the drainage ditch
As a result of soil and rock erosion caused by earthquake movements, a blockage occurred in the drainage ditch (Figure 8). The intercepting ditch on the edge of the Nurdagi Viaduct was blocked due to soil movement during the earthquake. A road intercepting ditch is a gutter constructed to drain water coming off the road to protect the cutting slope. As seen in Figure 8, the head ditch was filled with large stones and rock rubble after the earthquake. If this blockage is not removed, it will be difficult to drain the water, and serious problems may arise, including significant negative effects for viaduct footing foundations.
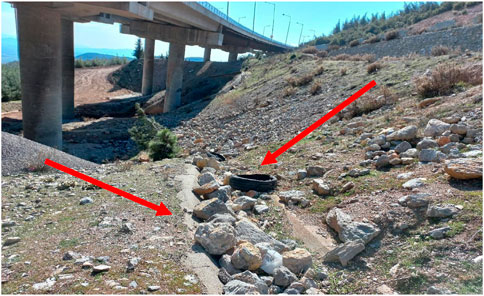
Figure 8. Drainage blockage between Nurdagi Viaduct (East) and D.400 Highway [Latitude: 37.171412–Longitude: 36.700336].
4.1.5 Expansion joint damage in reinforced concrete viaduct
Expansion joints are used in bridges to prevent the superstructure from being damaged by effects such as temperature changes and earthquake displacements. As seen in Figure 9, the joints available absorbed the damage caused by earthquake shifts and prevented structural damage to the viaduct beams. The main function of the expansion joints is to sacrifice themselves in an earthquake and ensure that the main structure remains standing. When considered from this perspective, the expansion joints did fully accomplished their function. The joint profiles used in the Nurdagi viaduct are of 240 mm cross-sectional profiles; as a result of displacement exceeding this value, the relevant viaduct joint profiles were seriously damaged. Traffic flow was ensured using steel plates within the scope of temporary repair.
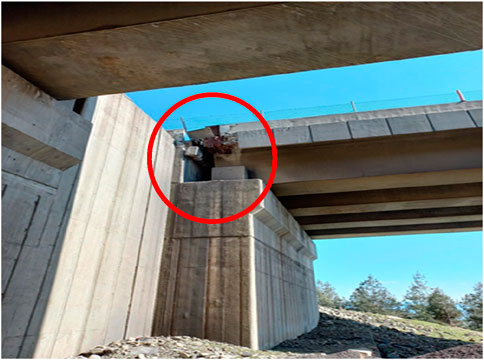
Figure 9. Expansion joint damage in reinforced concrete, Nurdagi Viaduct [Latitude: 37.171443–Longitude: 36.700860].
4.1.6 Damage in the middle of the circular viaduct column
Due to the great earthquake effect, the forces acting in the longitudinal direction of the bridge created deformation in the middle parts of the circular cross-section column. It is recommended that this damage be repaired by strengthening the column. Figure 10 demonstrates the damage that occurred to one of the columns of Nurdagi viaduct.
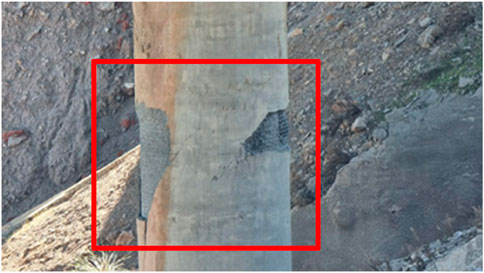
Figure 10. Plasticated concrete parts and damaged reinforcement of the abutments at Nurdagi Viaduct [Latitude: 37.170928–Longitude: 36.700281].
4.1.7 Offsets at reinforced concrete viaduct beams
The earthquake caused an average longitudinal displacement of 10 cm in the bearings on which the beams sit (Figure 11). It is recommended that all the damaged structural bearings be replaced with the new ones.
4.1.8 Box office canopy damage
During the earthquake, due to the effect of the fault, the foot on which the toll booth canopy was based moved and the canopy fell to the ground at the Komurler tool booths (Figure 12). The collapsed toll booth canopy caused one lane to be closed, resulting in traffic being diverted through other lanes.
4.1.9 Performance of steel versus concrete viaducts
When the reinforced concrete viaducts were examined, it was determined that they were damaged as a result of the longitudinal effect of the earthquake rather than its lateral effect. The elastomeric bearings at the beams fulfilled their duties during the earthquake and had permanent deformation. Accordingly, measurements showed that the viaduct beams shifted longitudinally in the range of 5–10 cm. These elastomer bearings that have completed their duties must be immediately replaced with the new ones.
Plastification and cracks caused by longitudinal movements were especially observed at the middle points of the mid-high bridge piers. It is important to reinforce these piers where the stated damage occurred on a limited scale.
The use of composite materials such as fiber-reinforced polymer (FRP) is recommended for the strengthening process of the existing concrete viaducts. Additionally, adopting modular steel designs can facilitate both reinforcement and maintenance processes, thereby extending the lifespan of the infrastructure. Base isolation techniques or energy dissipation devices (e.g., dampers) should be employed in both new and existing viaducts to mitigate the effects of seismic forces.
The examination of steel bridges revealed that they performed better than the reinforced concrete bridges as related expansion joint and bearing damage was not observed.
4.2 Evaluation of the access road to Hatay Airport
Hatay Airport, located in the city of Hatay, was put into service on 9 December, 2007; it is approximately 27 and 30 km away from Hatay and Iskenderun city centers, respectively. It serves Hatay, İskenderun, Reyhanlı, and other nearby regions. The total size of the terminal building is 46,826 m2, and its geographical coordinates are 36°22′20N–36°17′55E (DHMI, 2023; Fidan and Mete, 2020).
The airport was built after drying of Lake Amik on the Amik Plain formed by the accumulation of alluvia brought by the Asi, Karasu, and Afrin Rivers (Özşahin and Kaymaz, 2013). Its average altitude is approximately 100 m above sea level. A general view of the airport can be seen in Figure 13.
As can be seen in Figure 14, transportation to the airport is provided via the 5-km-long road with highway ID number 31-13, connected to the 825-08 section of the D.825 State Highway (KGM General Directorate of Highways, 2019). Amik Lake, which came into existence with the rise of water in the plain due to the heavy rains in Hatay and its surroundings in January 2019, reached the border of the Antakya–Iskenderun Road and destroyed the road leading thence to the airport (Ünal and Canlı, 2019). Subsequently, the road was raised approximately 1 m by the General Directorate of Turkish Highways and re-opened after the withdrawal of the water (Anadolu Agency, 2023).
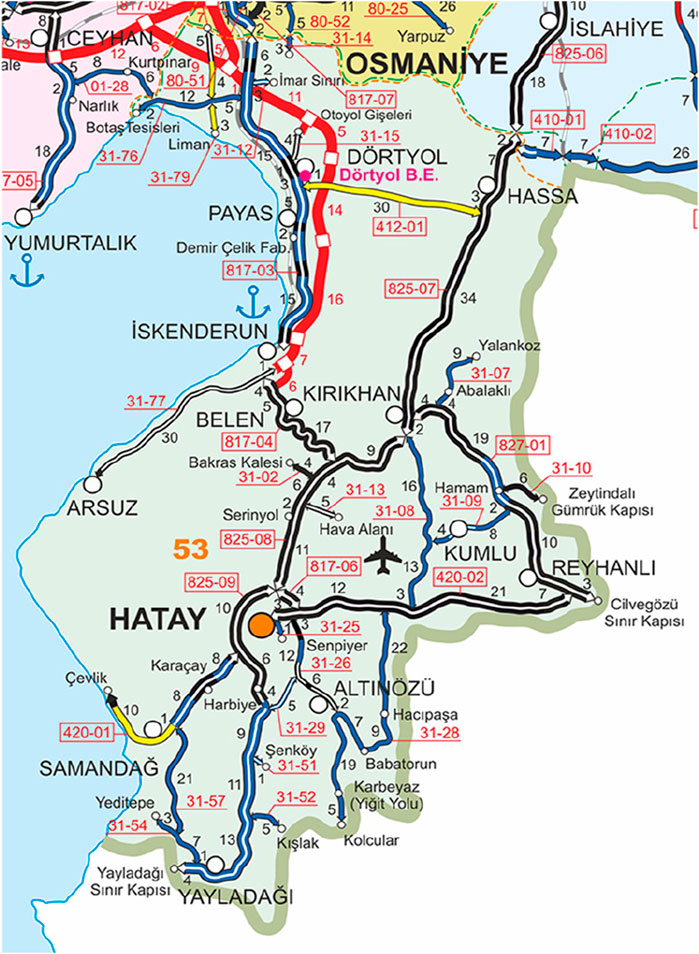
Figure 14. 5th Regional Road map of General Directorate of Highways around Hatay airport (KGM, 2023).
The earthquakes that occurred in Pazarcık and Elbistan districts of Kahramanmaras on 6 February, 2023 caused damage to the Hatay Airport runway and the access road leading to it, resulting in the airport being temporarily out of use. Figure 15 shows some images of collapses, cracks, and slides that occurred on the access road to the airport recorded by our team on 23 February, 2023 after the repair of the road.
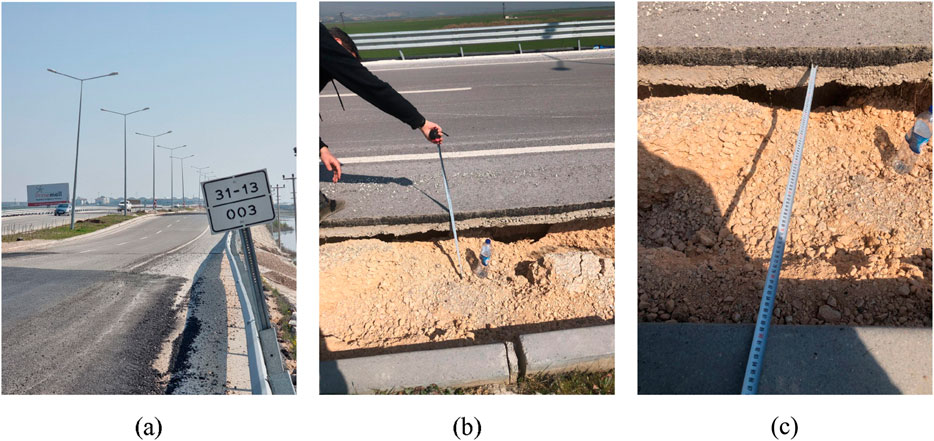
Figure 15. (a–c) Field observations and measurements carried out on Hatay airport road on 23.02.2023. (a) General view of the damaged road section; (b) Deformation detail with measurement; (c) Close-up view showing the depth and crack width measurements.
The damaged parts that occurred due to deformation on the airport access road were quickly repaired to ensure that access to the airport after the earthquake was provided. However, as can be seen in Figure 15 obtained from the field observations on 23 February, 2023, 17 days after the earthquakes, there were still visible defects on the road axis.
Following the field observations, the GNSS device was connected to the TUSAGA-AKTİF (CORS-TR) network on the approximately damaged 600 m section of the road, and measurements were made at almost equal intervals (Figure 16). The data obtained from these measurements were evaluated by computer analysis. Subsequently, the cross sections and profile of the current state of the road were obtained to analyze its geometrical characteristics.
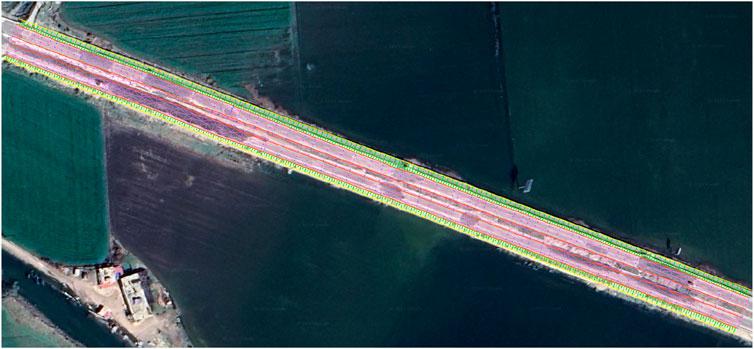
Figure 16. General condition of the damaged 600-m section of the airport road with measurements taken.
The road geometry was studied and evaluated in two separate ways in the east–west direction as a round trip. Figure 17 illustrates the airport inbound road profile with a 100/1000 (vertical/horizontal) scale created at 1-m intervals in the east–west direction. While the current ground elevation at the starting point of the profile (0 + 000) is 108.075 m, it is 106.598 m at the end point (0 + 607.84).
Figure 18 below displays the airport outbound road profile with 100/1000 (vertical/horizontal) scale created at 10-m intervals in the east–west direction. On the surface model developed using the data obtained after the measurements, cross sections were created every 1 m after the starting point, taking the direction of the road as a reference. However, in order to eliminate complexity in the images, these cross-sections are shown only in units of 10 m and its multiples. As can be seen from the figure below, while the current ground elevation at the starting point (0 + 000) of the profile is 108.093 m, its value at the end point (0 + 607.56) is 106.896 m.
In Figure 19, the cross-sections of the ingoing and outgoing roads were compared, and it was seen that the ground level difference between the two roads at the chainage point of 0 + 436 is over 1 m. The projection of this situation at 0 + 436 on both profiles is compared in Figure 20.
The elevation changes measured along in and out-bound routes were analyzed using the Optimized Hot Spot Analysis tool of ArcGIS Pro. Accordingly, a hot spot analysis map of the change was created (Figure 21).
5 Results and discussion
The functioning of transportation networks at the service level expected from them both after disasters, like earthquakes, and the change in daily traffic demand has been a field of intensive academic studies after the Kobe earthquake in Japan in 1995. It may be inevitable that there will be reductions in the service capacities of some elements of transportation networks such as intersections, viaducts, bridges, tunnels, alignments and curve sections, and cross-section elements, depending on the magnitude and severity of the earthquake or disaster. However, ensuring a strong control mechanism in both the topological design of the network and the manufacturing quality in the construction processes, as well as precise and accurate calculations in determining the project elements, will ensure that these negativities are kept to a minimum level. Rapid access by professional search and rescue teams along with relatively heavy tonnage vehicles carrying relief and rescue materials to the earthquake zone is expected in order to minimize loss of life and sustain the quality of the daily life of earthquake victims. It has thus been determined that the primary elements of the transportation network in the research and study region of the earthquake area maintained their basic functionality. The damage that occurred on the Hatay–Reyhanlı road can be cited as an example of the greatest damage caused by the earthquake to the regional transportation network. In addition, the closure of some village and state roads through which the fault line passes due to rockfalls and the damage to the Hatay airport access road can be mentioned as adverse situations on the regional network system. However, it should be noted that emergency vehicles did not have any access problem in the region. The tunnels in the region, which are of vital logistical importance, remained functional for emergency vehicles to provide swift initial access to the area affected by the earthquake. The transportation network elements that were out of service were repaired very quickly and put into service. Furthermore, the mobility of emergency vehicles was not hindered during this repair process.
In addition to the structural damage observed, the role of soil–structure interaction and resonance effects should not be overlooked. The differential settlements and landslides observed in the road sections highlight the importance of considering geotechnical factors in the design and evaluation of transportation infrastructure. After the earthquake, without affecting emergency transportation, noteworthy landslips of up to 50 cm were formed on the highway section of slip road 360-01 connecting state roads of D.835 and D825 [Latitude: 37.45429 Longitude: 36.989258]. These deformations can be attributed to soil-structure interaction (SSI) effects, particularly soil liquefaction and loss of bearing capacity during the seismic event. The differential settlement in the road body suggests that the underlying soil may have experienced uneven stiffness or liquefaction, leading to localized failures. Detailed geotechnical analysis with this respect should be carried out to better understand the soil properties and their interaction with the structural foundations.
Furthermore, resonance effects may have amplified the seismic forces on viaducts, leading to the failure of expansion joints and bearings. Future studies should incorporate detailed geotechnical analysis by considering this phenomenon to achieve improved seismic resilience of transportation networks.
As this paper investigates the immediate performance and failure analysis of the road networks after the earthquake, this aspect could not be examined in detail. However, the failures of the networks observed clearly indicate that soil related analysis must be put into practice to obtain more resilient transportation networks.
Although it is an important part of the reliability analysis of transportation networks, this study does not involve the performance of urban transportation networks. It mainly focused on the performance of the rural highway networks and presented the resilience of structural elements with a specific involvement of the TAG motorway.
Due to the rapid repair of the damage on some parts of the highways to re-open them to traffic, detailed preliminary determinations for these parts of the road network could not be performed regarding the scale of the damage that occurred. The quick repairs suggest that these kinds of damage were not significant, reflecting their relatively unimportant effect on the general transportation system. The use of continuous structural monitoring systems for important bridges and viaducts to determine the effects of even small size earthquakes will better support an understanding of the damage that may occur in these structures.
When the scope and magnitude of the earthquake were evaluated, it was determined that the bridges, viaducts, and tunnels of the transportation network in the region performed well and did not cause any profound adverse effects that would reduce the service level. However, it should be noted that there were some medium or minor scale damage on the roads and road structures fixed by regional directorates of Turkish Highways as a very swift way providing service continuity. Within the scope of the reliability analysis of urban transportation systems in this sense, it was concluded that the networks present in the cities of Kahramanmaras, Hatay, Kilis, and Gaziantep and their affiliated districts affected by the earthquake stayed robust and served the traffic at an acceptable level.
The location of the Hatay airport is the main reason why the access road has been damaged significantly. Not just earthquakes but heavy rainfall may also have caused serious problems as far as the functionality of this road is concerned. If there is not any opportunity to change the present location of the airport, the main body of the roadway must be elevated to a safe level of alarming points where a harmful water level should not reach by always keeping in mind that the roadway is durable and resistant to possible earthquakes likely in the future.
The General Directorate of Turkish Highways made temporary repairs to the damaged expansion joints and road body subsidence within 24 h after the earthquake and re-opened the structurally damaged roads to traffic, providing an effective response to the needs of emergency vehicles. On the other hand, during the field studies, it was observed that repair works were carried out by KGM on some parts of the roads through the provision of transportation safety in a controlled manner. Hence, it is quite important to use the structural monitoring systems for important bridges, tunnels, and viaducts to determine the effects of future earthquakes on transportation structures in the region so that the scale of the damage may be determined quite rapidly and accurately for proper action.
The investigations conducted on the region’s viaducts reveal that steel viaducts performed better than concrete. Field observations and inspections showed that concrete viaducts exhibit more deformation than their steel counterparts. This demonstrates the brittleness and limited flexibility of concrete structures, especially under dynamic loads such as earthquakes.
The finding of the better performance of steel bridges compared to reinforced concrete bridges revealed that future motorways and highways in the region should be designed and constructed by taking this outcome into consideration.
In high-seismic risk areas, comprehensive maintenance protocols should be established to ensure regular inspections of viaducts and the identification of weak points. Furthermore, strengthening critical infrastructure should be prioritized based on risk assessments and historical performance. Additionally, infrastructure design codes should be updated to reflect findings on the seismic performance of steel viaducts, and the incorporation of earthquake-resistant features in new projects should be encouraged.
In this study, the spatial distribution of deformations along the Hatay Airport access road was analyzed using height difference data obtained by GNSS measurements. For this purpose, hot spot maps were created with optimized hot spot analysis using ArcGIS Pro software. The following results were thus obtained.
• High deformation zones were identified providing the intervention priorities to be obtained effectively.
• It was proposed where preventive maintenance and improvement works should be concentrated.
• The findings were obtained based on spatial statistics regarding the types of deformation on the road surface.
Thus, it was statistically tested whether the deformations were systematically concentrated in certain areas or randomly distributed. The findings indicated that there was a significant vertical movement on the airport road surface forming notable clusters at certain points. This outcome provides a scientific basis to decision makers for the spatial management of post-earthquake road infrastructure deteriorations.
In real-time damage estimation and automated structural assessment processes, the integration of machine learning (ML) and artificial intelligence (AI)-based models with GNSS and GIS data can enable faster and more precise detection of deformations in transportation infrastructure after earthquakes. While deep learning algorithms can detect abnormal deformations by analyzing the movements of roads and viaducts continuously monitored through GNSS data over time, GIS-based spatial models can produce risk maps based on different scenarios and predict possible future damage areas. In the long term, slow deformations in transportation infrastructure can be predicted in advance using time series analysis and statistical regression models to enable maintenance and repair processes to be managed more effectively. Combining IoT-supported systems that provide instant data flow from GNSS sensors with GIS-based spatial analyses can significantly improve crisis management by enabling proactive measures to be taken in terms of transportation safety before earthquake. Such automated data processing and assessment systems can thus be integrated into disaster management strategies to reduce future earthquake risks.
The swift and effective rehabilitation of transportation infrastructure following an earthquake is critical not only for disaster management but also for economic and social sustainability. Lessons learned from past earthquakes and international engineering solutions serve as a guide to making Turkish transportation infrastructure more resilient. Specifically, the use of seismic isolators, retrofitting projects, and effective disaster management strategies can enable transportation systems to recover quickly to enhance societal resilience.
The outcomes indicated in this study provide an extremely valuable basis for the measures to be taken against the possible regional earthquakes in the future in terms of providing effective and uninterrupted transportation service, which play a vital role in minimizing loss of life and delays to the emergency requirements.
6 Conclusion
The findings of this research can be summarised as follows.
• It is important to be aware that the role of soil–structure interaction and resonance affecting structural damage must be observed to achieve more resilient transportation networks.
• The bridges, viaducts, and tunnels of the transportation network in the region performed well and did not cause any profound adverse effects that would reduce the level of service. Although there were some minor and medium size disruptions, the authorities fixed those problems quite rapidly and maintained traffic movement effectively.
• One of the most significant findings of this research is the conclusion that steel viaducts performed better than the concrete viaducts. This clearly suggests that the application of steel viaducts in the region would result in more a robust and resilient transportation network in future earthquakes.
• Regular inspection of the structures in the transportation network and collecting continuous data may result in earlier identification of weak points to allow an assessment of prioritized plans and related actions to be taken.
• Optimized hot spot analysis revealed high deformation zones, allowing the intervention priorities to be obtained effectively.
The implementation of comparative structural and statistical analysis between steel and concrete elements will be the next step for the evaluation of failure rates, repair costs, and longevity. The interaction between climate change-induced factors and socio-economic factors with earthquake-induced damage level in the region will also be investigated as the further stages of this research.
Data availability statement
The raw data supporting the conclusions of this article will be made available by the authors, without undue reservation.
Author contributions
HA: Conceptualization, Investigation, Methodology, Project administration, and Writing – review and editing. HK: conceptualization, investigation, software, and writing – review and editing. RS: conceptualization, investigation, and writing – review and editing. IT: conceptualization, investigation, and writing – review and editing.
Funding
The author(s) declare that financial support was received for the research and/or publication of this article. This study was supported by The Scientific and Technological Research Council of Türkiye (TÜBİTAK) under the 1002 - C Fieldwork Emergency Support Program Focused on Natural Disasters.
Conflict of interest
The authors declare that the research was conducted in the absence of any commercial or financial relationships that could be construed as a potential conflict of interest.
Generative AI statement
The authors declare that no generative AI was used in the creation of this manuscript.
Publisher’s note
All claims expressed in this article are solely those of the authors and do not necessarily represent those of their affiliated organizations, or those of the publisher, the editors and the reviewers. Any product that may be evaluated in this article, or claim that may be made by its manufacturer, is not guaranteed or endorsed by the publisher.
References
AFAD Disaster and Emergency Management Presidency. (2023). 06 Subat 2023 Pazarcik-Elbistan Kahramanmaraş (Mw: 7.7 – Mw: 7.6) depremleri raporu. Report, Ankara, 02 Haziran.
Anadolu Agency (2023). Hatay Havalimanı yolu trafiğe açıldı. Available online at: https://www.aa.com.tr/tr/turkiye/hatay-havalimani-yolu-trafige-acildi/13776 (Accessed March 30, 2023).
Anderson, J., Brown, K., and Taylor, L. (2022). Public-private partnership models in post-earthquake infrastructure recovery. J. Infrastructure Dev. 14 (2), 123–145. doi:10.1177/09749306221098765
Aydın, M., and Güler, K. (2018). Seismic performance evaluation of highway bridges in Turkey. J. Struct. Eng. 144 (5), 04018030. doi:10.1061/(ASCE)ST.1943-541X.0002011
Bertero, V. V. (1992). Seismic design of structures: lessons from California. Earthq. Spectra. 8 (1), 1–23. doi:10.1193/1.1585668
Brown, J., Smith, R., and Taylor, L. (2019). Financing models for post-earthquake infrastructure recovery. J. Infrastructure Syst. 25 (3), 04019015. doi:10.1061/(ASCE)IS.1943-555X.0000489
Cankurt, I., Salgın, O., Karan, Z. S., and Ilbey, A. (2019). Tusaga-aktif (Cors-Tr) sistemi Isletilmesi Ve Guncellestirilmesi. Ankara: Cografi Bilgi Sistemleri Kongresi.
Çelik, O., Yılmaz, T., and Karaca, E. (2022). Engineering solutions for enhancing seismic resilience of transportation infrastructure in Turkey. J. Earthq. Eng. 26 (4), 567–589. doi:10.1080/13632469.2021.1984567
Chen, K., Zhang, Z., Liang, C., Xue, C., and Liu, P. (2020). Kinematics and dynamics of the 24 january 2020 Mw 6.7 elazig, Turkey earthquake. Earth Space Sci. 7 (11), e2020EA001452. doi:10.1029/2020ea001452
DHMI (2023). General directorate of state airports authority Hatay airport. Available online at: https://www.aa.com.tr/tr/turkiye/hatay-havalimani-yolu-trafige-acildi/1377656 (Accessed March 30, 2023).
Elnashai, A. S., and Di Sarno, L. (2008). Fundamentals of earthquake engineering. NJ United States: Wiley.
Fukushima, Y., Tanaka, T., and Nakamura, H. (2018). Post-earthquake infrastructure improvements in Japan: lessons from the 2011 Tōhoku earthquake. J. Disaster Res. 13 (2), 234–245. doi:10.20965/jdr.2018.p0234
Gibbons, S., and McCarthy, T. (2020). Global practices in earthquake engineering: a comparative analysis. Int. J. Disaster Resil. Built Environ. 11 (3), 345–360. doi:10.1108/IJDRBE-12-2019-0087
Gonzalez, M., Lopez, R., and Martinez, P. (2020). Sustainable infrastructure development in post-earthquake Mexico. Int. J. Disaster Risk Reduct. 45, 101476. doi:10.1016/j.ijdrr.2019.101476
Huang, Y., and Chen, X. (2019). GNSS-based seismic risk assessment of urban transportation systems in Beijing. J. Geodesy 93 (7), 987–1001. doi:10.1007/s00190-018-1218-1
Johnson, L., Smith, J., and Wilson, R. (2018). Infrastructure planning strategies in high seismic risk areas. J. Urban Plan. Dev. 144 (2), 04018007. doi:10.1061/(ASCE)UP.1943-5444.0000432
Karaca, E., and Duman, T. (2021). Seismic performance analysis of urban bridges: a comprehensive review. J. Bridge Eng. 26 (7), 04021045. doi:10.1061/(ASCE)BE.1943-5592.0001745
KGM (2023). General directorate of highways. Available online at: https://www.kgm.gov.tr/SiteCollectionImages/KGMimages/Haritalar/b5.jpg (Accessed March 30, 2023).
KGM General Directorate of Highways. (2019). 2019 İl yolları trafik ve ulaşım bilgileri. (Accessed March 30, 2023). Available online at: https://www.kgm.gov.tr/SiteCollectionDocuments/KGMdocuments/Istatistikler/TrafikveUlasimBilgileri/19IlYollariTrafikUlasimBilgileri.pdf
Khalil, A., Rahman, M., and Elnashai, A. (2021). Earthquake risks in the Middle East: strengthening infrastructure resilience. Nat. Hazards Rev. 22 (3), 04021012. doi:10.1061/(ASCE)NH.1527-6996.0000483
Kumar, S., Sharma, R., and Gupta, A. (2017). Resilience of transportation infrastructure after earthquakes. Transp. Res. Part D Transp. Environ. 52, 1–12. doi:10.1016/j.trd.2017.02.012
Lee, S., Park, J., and Kim, H. (2024). Environmental impacts of post-earthquake infrastructure recovery: strategies for sustainability. J. Clean. Prod. 320, 128765. doi:10.1016/j.jclepro.2021.128765
Liu, X., and Wang, Y. (2019). Infrastructure damage and recovery strategies after the 2008 Sichuan earthquake. Disaster Prev. Manag. 28 (4), 456–470. doi:10.1108/DPM-12-2018-0375
Mehta, R., and Raghavan, S. (2020). Infrastructure vulnerability and resilience in the face of disasters: the role of GNSS-based analysis. J. Infrastructure Syst. 26 (3), 04020020. doi:10.1061/(ASCE)IS.1943-555X.0000567
METU Orta Doğu Teknik Üniversitesi. (2023). 6 Şubat 2023 Kahramanmaras-Pazarcık Mw=7.7 ve Elbistan Mw=7.6 depremleri Ön değerlendirme raporu. Report No: METU/EERC 2023-01, 20 Şubat.
Miyamoto, H. K. (2000). Lessons from the 1995 Kobe earthquake: infrastructure recovery and reconstruction. Earthq. Eng. and Struct. Dyn. 29 (5), 609–627. doi:10.1002/(SICI)1096-9845(200005)29:5<609::AID-EQE868=3.0.CO;2-M
Özşahin, E., and Kaymaz, Ç. K. (2013). Taşkın riskinin değerlendirmesine bir örnek: amik Ovası taşkınları. Turk. Stud. Elektron. 8 (8), 2021–2039. doi:10.7827/TurkishStudies.2013.11.020
Salgado, R., and Silva, M. (2021). A framework for assessing the resilience of urban transportation systems using GNSS data. Transp. Res. Part A Policy Pract. 145, 1–15. doi:10.1016/j.tra.2020.12.001
Smith, J., Johnson, L., and Wilson, R. (2016). Effectiveness of post-earthquake infrastructure repair processes. J. Infrastructure Syst. 22 (3), 04016007. doi:10.1061/(ASCE)IS.1943-555X.0000289
Sönmez, A., and Yıldırım, K. (2020). Rapid reconstruction of transportation systems after earthquakes. Nat. Hazards 103 (2), 1457–1475. doi:10.1007/s11069-020-04101-4
Taylor, L., Brown, J., and Smith, R. (2020). Social impacts of post-earthquake infrastructure recovery projects. J. Urban Plan. Dev. 146 (3), 04020025. doi:10.1061/(ASCE)UP.1943-5444.0000598
TKGM (2023). General directorate of Land Registry and Cadastre. Available online at: https://www.tkgm.gov.tr/tusaga-aktif-sistemi-hizmetine-devam-etmektedir (Accessed March 31, 2023).
TMMOB Union of Chambers of Turkish Engineers and Architects (2025). 6 Şubat depremleri 8. Ay değerlendirme raporu. Available online at: www.tmmob.org.tr.
Tuzun, G., Yilmaz, H., and Karaca, E. (2019). Assessment of post-earthquake transportation infrastructure in Turkey. J. Perform. Constr. Facil. 33 (4), 04019035. doi:10.1061/(ASCE)CF.1943-5509.0001298
Ünal, M., and Canlı, M. (2019). Sulak alanlarin yok edilmesinin etkileri ve amik gölü örneği. Doğanın Sesi 4, 49–66.
Wilson, R., Smith, J., and Johnson, L. (2021). The role of technology in enhancing post-earthquake infrastructure resilience. J. Infrastructure Syst. 27 (2), 04021010. doi:10.1061/(ASCE)IS.1943-555X.0000612
Keywords: risk analysis, reliability of transportation network infrastructure, earthquake, GNSS, failure analysis
Citation: Aslan H, Kocaman H, Sıkar RB and Turan İC (2025) Impact and GNSS-based deformation analysis of the earthquake in Türkiye on 6 February, 2023: a case study of the TAG (Tarsus–Adana–Gaziantep) motorway and Hatay airport. Front. Built Environ. 11:1572885. doi: 10.3389/fbuil.2025.1572885
Received: 07 February 2025; Accepted: 26 March 2025;
Published: 30 April 2025.
Edited by:
Abdullah Ansari, Sultan Qaboos University, OmanReviewed by:
Md. Alquamar Azad, Banaras Hindu University, IndiaFalak Zahoor, National Institute of Technology, Srinagar, India
Copyright © 2025 Aslan, Kocaman, Sıkar and Turan. This is an open-access article distributed under the terms of the Creative Commons Attribution License (CC BY). The use, distribution or reproduction in other forums is permitted, provided the original author(s) and the copyright owner(s) are credited and that the original publication in this journal is cited, in accordance with accepted academic practice. No use, distribution or reproduction is permitted which does not comply with these terms.
*Correspondence: Hakan Aslan, aGFzbGFuQHNha2FyeWEuZWR1LnRy