A World for Reactive Phenotypes
- 1MARBEC, Univ. Montpellier, Ifremer, IRD, CNRS, Palavas-Les-Flots, France
- 2COISPA Tecnologia & Ricerca, Stazione Sperimentale per lo Studio delle Risorse del Mare, Bari, Italy
- 3ESE, Ecology and Ecosystem Health, Institut Agro, INRAE, Rennes, France
- 4Department of Ecology and Evolutionary Biology, University of California, Los Angeles, Los Angeles, CA, United States
Humans currently occupy all continents and by doing so, modify the environment and create novel threats to many species; a phenomenon known as human-induced rapid environmental changes (HIREC). These growing anthropogenic disturbances represent major and relatively new environmental challenges for many animals, and invariably alter selection on traits adapted to previous environments. Those species that survive often have moved from their original habitat or modified their phenotype through plasticity or genetic evolution. Based on the most recent advances in this research area, we predict that wild individuals with highly plastic capacities, relatively high basal stress level, and that are generally shy—in other words, individuals displaying a reactive phenotype—should better cope with sudden and widespread HIREC than their counterparts' proactive phenotypes. If true, this selective response would have profound ecological and evolutionary consequences and can therefore impact conservation strategies, specifically with respect to managing the distribution and abundance of individuals and maintaining evolutionary potential. These insights may help design adaptive management strategies to maintain genetic variation in the context of HIREC.
Introduction
Conservation scientists have long recognized the importance of genetic variation for the management of sustainable populations (Loeschcke et al., 2013), including in the context of global changes (Rice and Emery, 2003). However, much less is known regarding the significance of evolutionarily important phenotypic traits, including physiological and personality traits, on the likelihood of successful management. Emphasizing the role of physiology is relatively recent, as exemplified by the recent launch (2013) of the journal “Conservation Physiology” (see also Cooke et al., 2014). Animal personality has also been recently included in the myriad of factors that could have profound consequences on population management (Réale et al., 2010a).
When within-individual variation of a behavioral trait is less than among-individual variation, this is defined as “personality” (Roche et al., 2016). Sometimes different personality traits co-vary (e.g., boldness is positively correlated with aggressiveness), and this defines a behavioral syndrome (Sih et al., 2004; Bell, 2007). Behavioral syndromes are related to the older notion of “coping styles” (Koolhaas et al., 1999), in that individuals are described on a reactive-proactive continuum based on their physiological and behavioral responses to a challenge. At one end of the continuum, reactive animals are those that are shy, less aggressive and less active compared to their proactive counterparts. But they are also characterized by high hypothalamic-pituitary-interrenal/adrenal (HPI/A) responses to stressors and low sympathetic activity compared to proactive ones (Koolhaas et al., 2010). In this sense, Koolhaas et al. (2010) specified that “proactive coping is characterized by low flexibility expressed as rather rigid, routine-like behavioral tendencies and reduced impulse control (behavioral inhibition) in operant conditioning paradigms.” Many studies have identified a range of behavioral and physiological traits that are linked to being reactive or proactive. Figure 1 illustrates to what extent proactive and reactive animals differ based on two authoritative reviews on the subject (Koolhaas et al., 1999, 2010).
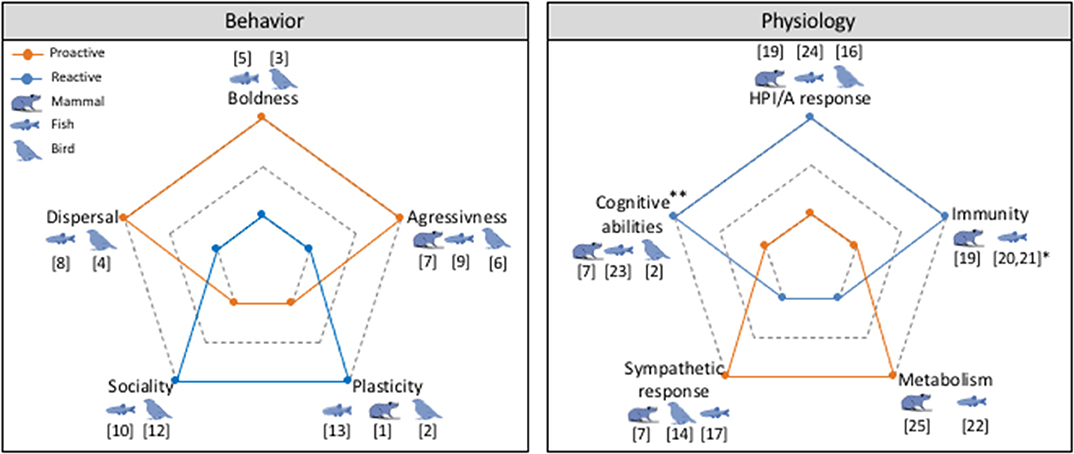
Figure 1. Radar charts describing the behavioral and physiological features of proactive (orange) and reactive (blue) coping style. *Note that concerning immune capacities, fishes exhibit opposite tendency to that described in Koolhaas et al. (2010) and Réale et al. (2010b). **Here, cognitive capacities are referring to cognitive capacities in changing environment context, where reactive animals displayed better performances. A non-exhaustive list of examples is presented in this figure from mammals, fishes and birds. Numbers refer to the studies as follows: Behavior: [1] (Benus et al., 1990); [2] (Verbeek et al., 1994); [3] (Carere and van Oers, 2004); [4] (Mettke-Hofmann et al., 2005); [5] (Øverli et al., 2006); [6] (Kralj-Fišer et al., 2007); [7] (Koolhaas et al., 2010); [8] (Chapman et al., 2011); [9] (Castanheira et al., 2013); [10] (Geffroy et al., 2014); [11] [12] (Kralj-Fišer et al., 2007); [13] (Ruiz-Gomez et al., 2008). Physiology: [2] (Verbeek et al., 1994); [14] (Korte et al., 1998); [15] (Bolhuis et al., 2003); [16] (Carere et al., 2003); [17] (Brelin et al., 2005); [18] (Huntingford et al., 2010); [7] (Koolhaas et al., 2010); [19] (De Miguel et al., 2011); [20] (Kittilsen et al., 2012); [21] (Vargas et al., 2018); [22] (Yuan et al., 2018); [23] (Baker and Wong, 2019); [24] (Wong et al., 2019); [25] (Careau et al., 2011).
Coping styles can evolve. Significant heritability in coping styles has been reported multiple times in wild and captive contexts and for various taxa including mammals, birds, reptiles, and fishes (Carere et al., 2003; Drent et al., 2003; Øverli et al., 2007; Ferrari et al., 2016; Øverli and Sørensen, 2016; Navas González et al., 2018; Wong et al., 2019). These studies all provide evidences that coping styles are genetically driven and can be shaped by selective pressures that lead to evolved changes. Additionally, coping styles may have fitness consequences where proactive or reactive individuals are differently favored depending on the environment (Smith and Blumstein, 2008; Réale et al., 2010b; Monestier et al., 2015; Moiron et al., 2020).
Early research on coping styles was carried out on animals selected for aggressiveness, or for HPI response, thus giving the well-known proactive/reactive dichotomy (Koolhaas et al., 1999; Øverli et al., 2007). However, later work in non-selected populations observed a continuum between these two extremes responses rather than a bimodal distribution (Ferrari et al., 2013; Monestier et al., 2015; Bensky et al., 2017; Daniel and Bhat, 2020). It is worth noting that some studies failed to discover consistent coping styles in the wild (Qu et al., 2018), possibly because predation pressure (a major selective force) might constrain the variability of behavioral and physiological traits (Geffroy et al., 2020), by selecting proactive or reactive individuals as a function of predation intensity.
From an ecological perspective, this structure has major implications for ecosystem dynamics since it drives intra-population competition as well as inter-species interactions (Bolnick et al., 2011; Sih et al., 2012). From an evolutionary point of view, variability in coping styles is also of primary importance since it constrains a population's capacity to adapt to environmental changes and determines the response to natural selection (Dingemanse and Réale, 2005). Yet, most of the studies focusing on coping styles in different contexts have been performed in fishes (Figure 2) and we suggest that a more comprehensive understanding of coping styles in all taxa must be developed because they may play a vital demographic role and hence influence population persistence and ecosystem dynamics.
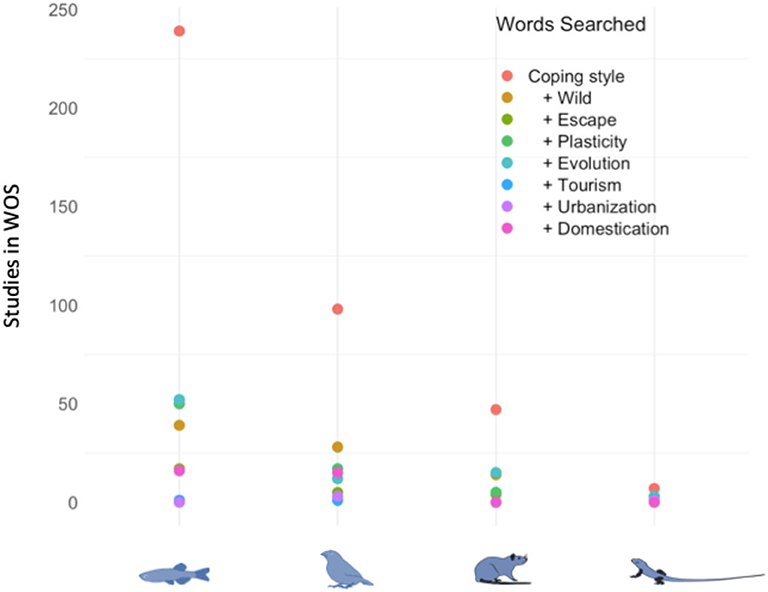
Figure 2. Number of studies in Web of Science investigating coping styles and various HIRECs in 4 taxa: Fish, Birds, Mammals, and Lizards (searched on the 14 September 2020 with Ifremer access).
Through extensive harvesting, environmental pollution, habitat loss and fragmentation, the introduction of exotic species, urbanization and climate change, humans modify the strength and direction of natural selection. This has profound consequences on the behavior and physiology of many species. These human-induced rapid environmental changes, or HIREC (Sih et al., 2011), have rapidly proliferated across the world, and better understanding the response to these changes is of primary importance for conservation and management Another emerging threat for wild species concerns the increased pressure caused by tourism and eco-tourism on wildlife (Geffroy et al., 2015; Blumstein et al., 2017), so that we suggest here to include mass tourism as a new HIREC. While behavioral responses to HIREC have previously been reviewed (Sih et al., 2011; Tuomainen and Candolin, 2011; Sih, 2013; Wong and Candolin, 2015), the associated underlying physiological mechanisms of the differences in coping style have been relatively ignored (Figure 2). We argue that they are essential traits to consider when animals will have to cope with HIREC, since behavioral responses alone do not necessarily map on perfectly with physiological responses and long-term associated costs.
Here, we focus on the multiple physiological and behavioral processes by which HIREC modifies the coping style structure of populations. Based on recent literature, we hypothesize that evolutionary responses to HIREC that resulted in increased selection at the intraspecific level may generally favor reactive individuals. Under this hypothesis, the reactive-proactive continuum of a population influenced by HIREC is assumed to shift toward reactive individuals. We propose that this change is the consequence of three different time-related steps starting with habitat changes (migration or dispersal), where proactive animals would be more likely leave unfavorable situations. Then, we suggest that reactive individuals have enhanced survival due to higher physiological and behavioral plasticity when facing unexpected events. Finally, we propose that long-term exposure to HIREC can result in genetic evolution favoring more reactive phenotypes (Figure 3). We provide accumulated evidence for all three described processes, but also note the limits of this perspective by providing relevant counter-examples. We discuss the ecological consequences of this speculative loss of proactive phenotypes and highlight its conservation relevance.
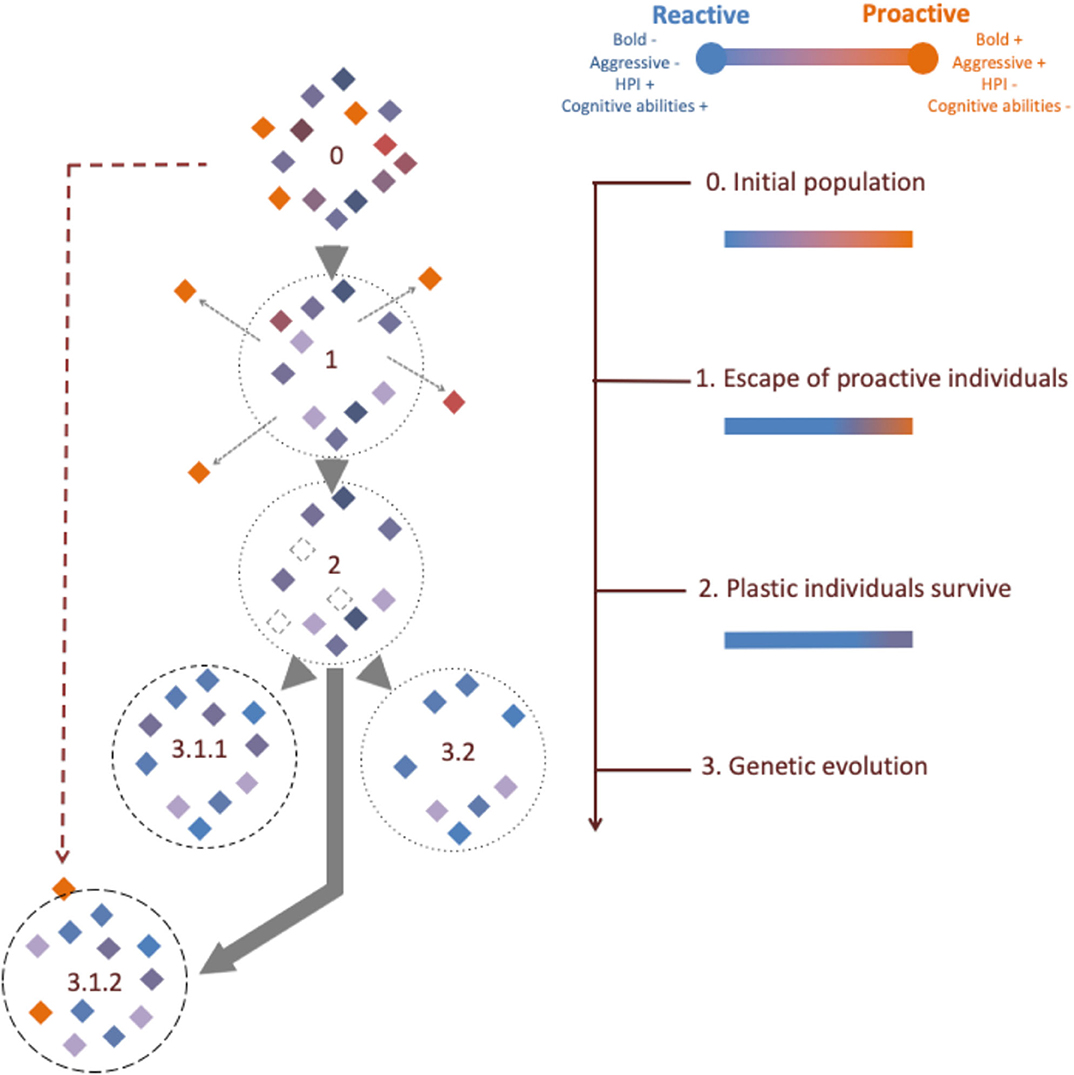
Figure 3. Temporal consequences of Human Induced Rapid Environmental Changes (HIREC) on the reactive-proactive continuum. Animals are from 0. An initial pristine population exposed to HIREC with effects on 1. dispersal, 2. phenotypic plasticity, 3. genetic evolution related to HIREC that produce 3.1. a human predator shield such as 3.1.1. Tourism and 3.1.2. Urbanization or 3.2. that do not produce a human shield (e.g., Climate Change, Pollution, or Harvesting). The dashed purple arrow highlights the fact that some proactive individuals could invade urban areas. The shape of the border of each circle directly correlates to the intensity of human-shield, from no contact (light dashed circle), to high contact (full circle). The diamond color corresponds to individual phenotypic coping style.
Challenging Situations Make Proactive Individuals Change Habitats
The quickest response for individuals in a wild population to avoid human presence and associated environmental changes is to flee and change habitats. This first step in response to HIREC (Figure 3.1), is nevertheless highly dependent upon an individual's personality. Prior work has indeed shown that inter-individual differences in the propensity to disperse are linked to personality traits (Cote and Clobert, 2007; Clobert et al., 2009; Cote et al., 2010a). Bold individuals are generally more active, explore novel areas faster and are more likely to disperse farther (reviewed in Réale and Montiglio, 2020). Harrison et al. (2015) also differentiated “resident” from “mobile” individuals. Mobile individuals tend to explore more, be more active and be less site-specific, and thus, they resemble proactive individuals. In this sense, less socially embedded yearling female marmots (Marmota flaviventer) are also more likely to disperse (Blumstein et al., 2009), and in the month preceding dispersal these marmots upregulate a suite of specific genes, many of which are associated with migration (Armenta et al., 2018).
Yet, the fact that bolder individuals are more likely to disperse might not be always true. For example, resident wild elk (Cervus canadensis) habituated to human presence in two Canadian national parks (Banff and Jasper) were shown to be bolder and more dominant than migrants elk (Found and St. Clair, 2019). One reason could be that this was because dominant individuals were residents. But this observation could also be the result of high contact with humans in the parks, where some individuals are, or become bold to exploit new food sources or due to habituation (Found and St. Clair, 2019), a case we specifically discuss below (see HIREC relaxing selection pressure section). Interestingly, these individuals also exhibited characteristics of reactive phenotypes (i.e., high cognitive flexibility), which could support the second hypothesis where initially shy individuals become bold following human contact.
Overall, when the outcome of the encounter is positive (e.g., increased access to food or protection from predators), then we might expect that bold individuals remain. However, when HIREC reduces habitat quality or when the situation becomes more challenging, then we expect bold individuals to be better at escaping (Figure 3.1). For instance, bold crabs were the first to disperse when habitat quality was reduced (Belgrad and Griffen, 2018). Bolder fish make more attempts to escape laboratory-induced hypoxia (Brelin et al., 2005; Ferrari et al., 2016), salinity challenges (Zeng et al., 2019) or high ammonia concentrations (Gesto et al., 2020). Bold individuals are also better at avoiding or escaping fishing gear (Diaz Pauli and Sih, 2017). And, individual great tits (Parus major) that are relatively more exploratory are located further away from polluted sites (Grunst et al., 2019a,b), although we cannot exclude that pollutants directly affects their exploration abilities.
Most studies conducted to date on the subject have investigated behavioral traits, while ignoring physiological ones. With respect to coping styles that associate behavior and physiology, most of our knowledge comes from fishes (Figure 2). A recent study conducted on juvenile eels pinpointed that individuals that were more likely to climb dams to accomplish their upstream migration, also had lower levels of transcription of synapse-related genes (which are associated with cognitive abilities) compared to others, and were thus coined as proactive (Podgorniak et al., 2016). In European sea bass and Atlantic salmon, individuals who flee from hypoxic environments also have lower HPI stress response and greater serotonin turnover ratio than individuals who remain in hypoxic environments, which is indicative of proactive coping style (Damsgård et al., 2019; Ferrari et al., 2020). Thus, this suggests that proactive individuals are more prone to leave HIREC-impacted areas. Additional work on distinct taxa is, however, needed to clearly identify the physiological and cognitive features of bold/mobile animals in the context of HIREC. We suggest that in many cases, bold/mobile individuals with lower stress responses (i.e., those that are relatively proactive) are more likely to change habitats in stressful situations related to HIREC (Figure 3.1) and this may have evolutionary and ecological consequences (Hebblewhite et al., 2005).
HIREC-Driven Advantages of Plastic Individuals
Phenotypic variations for individuals exposed to HIREC are largely documented in their responses to exposure to contaminants, changes in temperature, acidification, environmental noise, etc. Individuals with the best capacities to respond rapidly via behavioral plasticity (van Baaren and Candolin, 2018) or physiological plasticity (Taff and Vitousek, 2016) are therefore the most likely to survive HIREC, as seen with how some species respond to climate change (Beever et al., 2017). In a wide range of taxa, proactive, and reactive phenotypes diverge in their capacities to respond to environmental changes (Figure 1). Overall, case studies highlighted the ability of reactive individuals to be more behaviorally plastic in a wide range of circumstances. In house mice (Mus musculus) selected for their aggressive behavior, proactive individuals developed routines; a putatively superior strategy in predictable environments (or situations) but not when the environment frequently changed (Benus et al., 1991).
Plasticity may nevertheless be energetically costly (Moran, 1992; Murren et al., 2015). Consequently, in a stable environment, proactive individuals, which are less plastic than reactive individuals, are expected to better perform because less energy is invested in coping abilities, memory and learning capacities (Figure 1). The cost of plasticity has been highlighted in great tits, where fast exploring/proactive individuals performed better in stable environments, while the slow exploring/reactive individuals performed better in a variable and fluctuating environment (Dingemanse and de Goede, 2004). In rainbow trout (Oncorhynchus mykiss) moved from the UK to Norway, reactive individuals had greater feeding motivation and started to win dyadic fights against proactive opponents; the opposite outcome that occurred prior to being moved (Ruiz-Gomez et al., 2008). Moreover, reactive rainbow trout are also more efficient at finding food when it is relocated (Ruiz-Gomez et al., 2011). Overall, these examples show that reactive individuals have better coping capacities in modified environments, and this highlights the benefits of plasticity. Similarly, in brook trout (Salvelinus fontinalis), reactive fish performed better in cognitively complex foraging tasks, even after environmental modifications (White et al., 2017). Comparable conclusions were drawn in birds (Verbeek et al., 1994) and pigs (Sus scrofa; Bolhuis et al., 2004), where proactive individuals were less successful in reversal learning than reactive pigs, suggesting that proactive individuals are less plastic. In monogamous red point cichlids (Amatitlania siquia), reactive individuals were better at varying their behavioral profile within the pair (Laubu et al., 2016), a capacity that enhances their reproductive success (Gabriel and Black, 2012; Harris and Siefferman, 2014). A recent study on gray seals (Halichoerus grypus) also highlighted that mothers displaying reactive coping style presented a more variable energy expenditure in reproduction and reproductive success compared to their proactive counterparts (Twiss et al., 2020); while mean fitness was equal across coping styles (Twiss et al., 2020). The authors proposed that this high variability was linked to reactive mothers attempting to match pup phenotypes to the local environmental conditions (Twiss et al., 2020). Hence, in case of a sudden extreme event (i.e., HIREC), one might expect higher fitness for reactive mothers.
Altogether, the various studies conducted on the subject showed that reactive individuals have increased behavioral capacities to cope with rapid environmental changes, and this might be essential in the context of HIRECs. This is also seen at the physiological level, with reactive individuals having higher capacities to mount physiological responses required to cope with environmental challenges (Figure 3.2), partly due to greater HPI/A axis activation when exposed to stressors.
Evolutionary Shifts Observed in Response to HIREC
In response to persistent HIREC, populations might evolve to adapt to new environmental constraints (Figure 3.3). It should however be noted that sometimes, environmental (biotic and abiotic) pressures are so intense and ubiquitous (climate, predation, competition and parasitism) that the population does not have sufficient time to adapt, and may instead collapse (White et al., 2014).
Due to its intrinsic variability, it appears essential to categorize HIREC according to its effect since some are acting as additional new direct selective forces on wild populations (e.g., climate change, harvesting, etc.), while others act by reducing selective forces by, for example, protecting prey from their predators (e.g., urbanization, ecotourism). Below, we will explain why this distinction is essential because the two categories have different evolutionary consequences.
i) HIREC increasing selection pressure
HIREC increasing selective pressures drive both behavioral and physiological changes. For example, fish with bold phenotypes can be preferentially, although unintentionally, harvested, resulting in the selective depletion of bold individuals (Biro and Post, 2008). While intensive fishing selects on life history traits (growth, maturation, reproduction), demonstrations of fishing's effects on behavior remained scarce until the last decade (Uusi-Heikkilä et al., 2008). Fishing methods are diverse, and lead to different selection pressures on behavior (Diaz Pauli et al., 2015; Arlinghaus et al., 2017). Passive fishing (e.g., using long-lines, angling, trapping or gill nets) preferentially catch proactive individuals (Biro and Post, 2008; Arlinghaus et al., 2017), while active gear (e.g., trawls or purse seines) unintentionally targets reactive individuals (Heino and Godø, 2002; Diaz Pauli et al., 2015).
Hunting may have weaker effects on wild populations than fishing, yet it too selects for specific behavioral traits. Shyer captive-reared and released pheasants (Phasianus colchicus) were more likely to survive to the hunting season than bolder ones (Madden and Whiteside, 2014). In wild reindeer (Rangifer tarandus), a long-term study showed that hunting pressure was correlated with increased flight distance over time, suggesting that populations become shyer under consistent hunting pressure (Reimers et al., 2009). Importantly, those elk (Cervus elaphus) that were successfully hunted were bolder (Ciuti et al., 2012). Thus, hunting leads to the same result as passive fishing because it selects against bolder individuals and therefore favors reactive phenotypes. Notably, fewer active animals die from active hunting techniques, such as when dogs are used to chase animals, as seen in bear-hunting (Leclerc et al., 2019). As a consequence, the HIREC linked to fishing and hunting seems to favor one coping style over another depending on whether the harvesting technique is active or passive.
We have long known that populations evolve in response to exposure to pollutants and, with the toxification of Earth, chemical pollution has become a common new direct selective force. Empirical studies in the lab monitoring the evolution of behavioral and physiological traits over many generations following exposure to pollutants are rare, despite abundant evidence of rapid evolutionary responses (Whitehead et al., 2017; Saaristo et al., 2018). If the exposure to only one pollutant selected one behavioral response (e.g., boldness or activity), it could be attenuated by a plastic behavioral response (Saaristo et al., 2018). However, there are many pollutants, each with different properties, and the possible interactions between them (Peterson et al., 2017; Saaristo et al., 2018). Additionally, the method of exposure varies and together these pollutants may act as multiple environmental stressors for organisms which must continuously respond to new threats and stressors. This makes it difficult to make concrete predictions about the precise selective forces that may act on a specific coping style.
Nevertheless, pollution is known to generally alter various behavioral traits–such as boldness, activity, dispersal and sociability–as well as physiological traits–such as cognitive abilities or metabolic rate (reviewed in Jacquin et al., 2020). Pollutants can disrupt the syndrome linking physiology and behavior (Jacquin et al., 2020) and could even increase plasticity of physiological and behavioral traits (Tan et al., 2020). Such a variety of responses to the different stressors are likely context-dependent and species-specific, so that it is difficult to anticipate the direction of changes over generations (toward reactive or proactive). In agreement with other authors (Jacquin et al., 2020), we suggest that plasticity, a characteristic more often seen in individuals that tend to be reactive, will be essential to cope with the diversity of stressors created by pollution, as recently highlighted in fishes (Tan et al., 2020).
In the context of climate change, both progressive changes (e.g., increased temperature) as well as an increase in the frequency of extreme events are expected, leading to new direct selective forces. This combinations of threats may also lead to evolutionary changes in the physiology and behavior of wild population by selecting highly plastic individuals who can respond to increased variability (Nussey et al., 2005). Following this reasoning we may thus infer that climate change is driving the evolution of reactive phenotypes (Figure 3.3.2). It is nevertheless worth noting that in some very specific cases, opposite results were observed with, for example, tropical cyclones that select for aggressive phenotypes (Little et al., 2019). This suggests that, sometimes, proactive individuals may be better equipped to survive environmental alterations related to climate change.
ii) HIREC relaxing selection pressure
Contrary to previous examples, urbanization and ecotourism are known to relax selective pressure related to predation for species inhabiting these areas, due to the well-described “human shield” they create (Berger, 2007). Studies of the consequences of urbanization and ecotourism have proposed that boldness increases in populations subjected to these HIRECs (Geffroy et al., 2015) in response to the “human shield.” A recent phylogenetic meta-analysis showed that antipredator traits of urbanized animals decreased to a similar manner to that of domesticated animals, though at a rate 3 times slower (Geffroy et al., 2020). Throughout the process of urbanization, individuals are becoming bolder and less stressed over generations (Geffroy et al., 2020), and in that sense, more proactive.
This increase in boldness has been seen many times. Birds from urban areas have reduced flight initiation distances (Samia et al., 2015), and engage in more risk-taking behaviors than rural individuals (Miranda et al., 2013). With respect to physiological responses, urban birds facing humans have reduced stress reactivity (Partecke et al., 2006; Atwell et al., 2012). However, increased stress reactivity was observed in urban lizards compared to rural ones in the context of inter-individual social interactions (Batabyal and Thaker, 2019). These results suggest that the response might be tightly associated with the intensity of human-animal interactions, since lizards might be less likely to interact with humans compared to birds, which may view humans as a source of food. Being able to live in urban environments requires substantial individual plasticity. For instance, in response to urban noise, great tits increase their pitch during mating calls to increase the likelihood that potential mates receive the signal (Slabbekoorn and Peet, 2003).
It's important to consider that we still do not really know whether some individuals in urbanized areas become bolder due to consistent interactions with humans or that bold individuals are the one who invade towns (Sol et al., 2013, 2018) (Figure 3.3.1.2; dashed arrow). For tourism and ecotourism, biological consequences directly depends on the intensity of human contact, with strong negative interactions triggering avoidance, not habituation (Geffroy et al., 2017) (Figure 3.3.1.1). For instance, increased stress reactivity was found in fish interacting closely with tourists (so that they were bold toward humans), but this also came with increased production of neurogenesis markers, suggesting higher plasticity (Geffroy et al., 2018).
Hence, to identify the better coping style under HIREC, two essential parameters must be considered: (1) Does HIREC trigger relaxed selection in the wild? (Lahti et al., 2009), and (2) Does HIREC trigger an ecological trap depending on the scale of the impact? (Hale and Swearer, 2016). For the first parameter, if a HIREC protects animals (i.e., through a human shield), then some proactive phenotypes would thrive in these impacted areas (e.g., an urbanized area). By contrast, if HIREC are increasing selection (by being, for example, consumptive through hunting or fishing), then we expect that reactive individuals will perform better. Note that in both cases, we expect that plastic individuals will do better coping with human presence. For the second parameter, if HIREC are relatively local, then we expect that proactive individuals would perform better due to their higher propensity to escape (reactive individuals will face ecological traps: e.g., localized pollution). However, if HIREC are widespread (which many are), then reactive individuals would likely benefit, due to their greater plasticity. To summarize, we predict that more reactive phenotypes would thrive in most HIREC contexts (note however that urbanized individuals are likely a mixture of proactive and reactive phenotypes).
Possible Ecological Consequences of the Vanishing of One Phenotype: Extrapolating the Potential loss of Proactive
Intra-species variation is a powerful driver of ecological success (Forsman and Wennersten, 2016). Removing proactive phenotypes might lead to a reduction in intra-species variability with consequences on the population to cope with environmental variation. We know that behavioral variation is an index of genetic variation (Smith and Blumstein, 2013). If we selectively reduce phenotypic variation, we likely reduce genotypic variation. Such a reduction may be ultimately costly if it reduces a population's adaptive potential to what may ultimately be a more variable environment. This loss of behavioral diversity may be particularly acute in conservation management when animals are brought into captivity for breeding with subsequently planned translocations and reintroductions (e.g., Smith and Blumstein, 2012; White et al., 2014; Merrick and Koprowski, 2017). We expect captivity to reduce genetic variation and also to eliminate the very variation that may be essential for maintaining sustainable populations.
Many studies have noted the essential role of keystone species that warrant specific conservation efforts due to their central position in their ecological network (Mills et al., 1993; Paine, 1995; Betts et al., 2015), and whose extinction or population decline could have drastic consequences on community structure (Cortés-Avizanda et al., 2015). Recent work has focused on the essential role played by some individuals in a group and coined the term keystone individuals (Pruitt and Keiser, 2014). Modlmeier et al. (2014, p. 55) defined these individuals as having “a disproportionally large, irreplaceable effect on other group members and/or the overall group dynamics relative to its abundance.”
The systematic loss of proactive phenotypes could initially influence group composition and population dynamics because these individuals are likely to be keystone individuals. As noted above, proactive individuals tend to disperse more, while reactive individuals are more likely to join newly colonized areas (Cote et al., 2010a). If new populations are mostly composed of reactive individuals, this may constrain dispersal and space use. For instance, average group personality scores (boldness, activity, and sociability) of some feral guppy (Poecilia reticulata) populations were not associated with exploratory propensity. Rather, group exploratory propensity was driven by the personality of key individuals, whereby slow individuals tended to slow down the shoal's exploration rates (Brown and Irving, 2014). In mosquitofish (Gambusia affinis) average group personality appeared to also drive group dispersion in the expected pattern; groups with many asocial individuals dispersed further. However, this study failed to identify keystone individuals (Cote et al., 2010b). Because exploration is likely associated with resource harvesting, we can envision that HIREC may modify these patterns and have consequences on other trophic levels. Thus, if a system evolved with a mix of shy and bold individuals, and HIREC systematically eliminated one type, we should expect changes in species composition.
The potential disappearance of one phenotype could have substantial consequences on prey-predator relationships. A recent review noted that whether a prey has a proactive or a reactive response depends on the predictability of an encounter with a predator (Creel, 2018). The former response will have an energetic cost (fleeing) while the latter would have a stress-mediated cost, by activating the HPI/A axis (Creel, 2018). However, this previous analysis largely ignored potential intra-individual differences in coping abilities by assuming that all individuals can mount a similar response according to the situation.
We suggest here that the type of response would also depend on the coping style of each individual, although the ecological consequences may be the same. If a population loses all proactive individuals, then responses to a predator consisting of displaying aggressive behaviors, modifying activity periods, or engaging in particular patterns of vigilance (Creel, 2018) may likely be quite different. Specifically, without proactive responses, we will only see reactive responses, driving a variety of associated stress-related costs. For instance, in the snowshoe hare-lynx system, predation risk increased glucocorticoid production with direct consequences in the decline of offspring production (Krebs et al., 1995). Hence, we can expect that if prey mount a reactive response to predatory encounters, prey will become chronically stressed and this will have a cascading effect on reproductive success (Krebs et al., 1995).
For predators too, the ecological consequences of losing proactive individuals could be dramatic. For instance, when comparing the behavior of predatory fishes living in relatively unprotected areas (recreational fishing permitted) to old no-take kelp forests (that have been protected for 40 years), some species are 6.5 times less bold toward prey (as quantified by the number of attacks) and consequentially consume nearly half the prey compared to those in fully protected areas (Rhoades et al., 2019). Spotted hyenas (Crocuta crocuta) inhabiting highly disturbed areas (close to humans) are less bold toward a mock intruder, compared to those living in undisturbed areas (Turner et al., 2020). As a consequence, shyer hyaenas had higher survival rates (Turner et al., 2020). Taken together, these examples illustrate how the vanishing of proactive phenotypes due to human presence or harvesting could have profound impacts on predatory-prey dynamics. In addition to predator-prey interactions, it is worth noting that physiological and behavioral changes induced by HIRECs on wild populations may also affect the host-parasite/pathogen dynamics and beneficial partnerships between species, causing cascading impacts on ecosystem functioning (Hammond et al., 2020).
Conclusions and Conservation Relevance
Taken together, human-induced rapid environmental changes (HIREC) have profound and potentially cascading effects on the behavior and physiology of wild animals that may impact the proportion of proactive and reactive individuals in a population. These alterations may lead to modifications in species' distribution and abundance. If this occurs, this would be the result of three distinct time-related steps: (1) the loss of proactive individuals because they move away, (2) the enhanced survival of reactive individuals, and (3) genetic evolution. Based on our current knowledge, we suggest that HIREC that do not systematically relax predation pressure—such as seen with climate change, pollution or harvesting—would lead to genetic evolution toward reactive individuals. This, of course, assumes that individuals are able to initially survive these widespread changes, which of course is not a given. Knowledge of this systematic selection is essential to inform conservation actions to maintain genetic diversity, and hence evolutionary potential, in natural populations. Importantly, these insights may help design adaptive management strategies to maintain genetic variation within populations in the context of HIREC.
Data Availability Statement
The original contributions presented in the study are included in the article/supplementary material, further inquiries can be directed to the corresponding authors.
Author Contributions
BG: conceptualization, writing—review, and editing. SA and BS: writing—review and editing. DTB: supervision, writing–review, and editing. All authors contributed to the article and approved the submitted version.
Conflict of Interest
DTB is the Chief Editor of Frontiers in Conservation Science.
The authors declare that the research was conducted in the absence of any commercial or financial relationships that could be construed as a potential conflict of interest.
References
Arlinghaus, R., Laskowski, K. L., Alós, J., Klefoth, T., Monk, C. T., Nakayama, S., et al. (2017). Passive gear-induced timidity syndrome in wild fish populations and its potential ecological and managerial implications. Fish Fish. 18, 360–373. doi: 10.1111/faf.12176
Armenta, T. C., Cole, S. W., Geschwind, D. H., Blumstein, D. T., and Wayne, R. K. (2018). Gene expression shifts in yellow-bellied marmots prior to natal dispersal. Behav. Ecol. 30, 267–277. doi: 10.1093/beheco/ary175
Atwell, J. W., Cardoso, G. C., Whittaker, D. J., Campbell-Nelson, S., Robertson, K. W., and Ketterson, E. D. (2012). Boldness behavior and stress physiology in a novel urban environment suggest rapid correlated evolutionary adaptation. Behav. Ecol. 23, 960–969. doi: 10.1093/beheco/ars059
Baker, M. R., and Wong, R. Y. (2019). Contextual fear learning and memory differ between stress coping styles in zebrafish. Sci. Rep. 9:9935. doi: 10.1038/s41598-019-46319-0
Batabyal, A., and Thaker, M. (2019). Social coping styles of lizards are reactive and not proactive in urban areas. Gen. Comp. Endocrinol. 270, 67–74. doi: 10.1016/j.ygcen.2018.10.007
Beever, E. A., Hall, L. E., Varner, J., Loosen, A. E., Dunham, J. B., Gahl, M. K., et al. (2017). Behavioral flexibility as a mechanism for coping with climate change. Front. Ecol. Environ. 15, 299–308. doi: 10.1002/fee.1502
Belgrad, B. A., and Griffen, B. D. (2018). Personality interacts with habitat quality to govern individual mortality and dispersal patterns. Ecol. Evol. 8, 7216–7227. doi: 10.1002/ece3.4257
Bell, A. M. (2007). Future directions in behavioural syndromes research. Proc. R. Soc. B Biol. Sci. 274, 755–761. doi: 10.1098/rspb.2006.0199
Bensky, M. K., Paitz, R., Pereira, L., and Bell, A. M. (2017). Testing the predictions of coping styles theory in threespined sticklebacks. Behav. Processes 136, 1–10. doi: 10.1016/j.beproc.2016.12.011
Benus, R. F., Bohus, B., Koolhaas, J. M., and van Oortmerssen, G. A. (1991). Heritable variation for aggression as a reflection of individual coping strategies. Experientia 47, 1008–1019. doi: 10.1007/BF01923336
Benus, R. F., Daas, S. D., Koolhaas, J. M., and Van Oortmerssen, G. A. (1990). Routine formation and flexibility in social and non-social behaviour of aggressive and non-aggressive male mice. Behaviour 112, 176–193. doi: 10.1163/156853990X00185
Berger, J. (2007). Fear, human shields and the redistribution of prey and predators in protected areas. Biol. Lett. 3, 620–623. doi: 10.1098/rsbl.2007.0415
Betts, M. G., Hadley, A. S., and Kress, W. J. (2015). Pollinator recognition by a keystone tropical plant. Proc. Natl. Acad. Sci. U.S.A. 112, 3433–3438. doi: 10.1073/pnas.1419522112
Biro, P. A., and Post, J. R. (2008). Rapid depletion of genotypes with fast growth and bold personality traits from harvested fish populations. Proc. Natl. Acad. Sci. U.S.A. 105, 2919–2922. doi: 10.1073/pnas.0708159105
Blumstein, D. T., Geffroy, B., Samia, D. G. M., and Bessa, E. (2017). Ecotourism's promise and peril: A biological evaluation.
Blumstein, D. T., Wey, T. W., and Tang, K. (2009). A test of the social cohesion hypothesis: interactive female marmots remain at home. Proc. R. Soc. B Biol. Sci. 276, 3007–3012. doi: 10.1098/rspb.2009.0703
Bolhuis, J. E., Parmentier, H. K., Schouten, W. G. P., Schrama, J. W., and Wiegant, V. M. (2003). Effects of housing and individual coping characteristics on immune responses of pigs. Physiol. Behav. 79, 289–296. doi: 10.1016/S0031-9384(03)00090-8
Bolhuis, J. E., Schouten, W. G., de Leeuw, J. A., Schrama, J. W., and Wiegant, V. M. (2004). Individual coping characteristics, rearing conditions and behavioural flexibility in pigs. Behav. Brain Res. 152, 351–360. doi: 10.1016/j.bbr.2003.10.024
Bolnick, D. I., Amarasekare, P., Araújo, M. S., Bürger, R., Levine, J. M., Novak, M., et al. (2011). Why intraspecific trait variation matters in community ecology. Trends Ecol. Evol. 26, 183–192. doi: 10.1016/j.tree.2011.01.009
Brelin, D., Petersson, E., and Winberg, S. (2005). Divergent stress coping styles in juvenile brown trout (Salmo trutta). Ann. N. Y. Acad. Sci. 1040, 239–245. doi: 10.1196/annals.1327.033
Brown, C., and Irving, E. (2014). Individual personality traits influence group exploration in a feral guppy population. Behav. Ecol. 25, 95–101. doi: 10.1093/beheco/art090
Careau, V., Thomas, D., Pelletier, F., Turki, L., Landry, F., Garant, D., et al. (2011). Genetic correlation between resting metabolic rate and exploratory behaviour in deer mice (Peromyscus maniculatus). J. Evol. Biol. 24, 2153–2163. doi: 10.1111/j.1420-9101.2011.02344.x
Carere, C., Groothuis, T. G. G., Möstl, E., Daan, S., and Koolhaas, J. M. (2003). Fecal corticosteroids in a territorial bird selected for different personalities: daily rhythm and the response to social stress. Horm. Behav. 43, 540–548. doi: 10.1016/S0018-506X(03)00065-5
Carere, C., and van Oers, K. (2004). Shy and bold great tits (Parus major): body temperature and breath rate in response to handling stress. Physiol. Behav. 82, 905–912. doi: 10.1016/S0031-9384(04)00312-9
Castanheira, M. F., Herrera, M., Costas, B., Conceição, L. E. C., and Martins, C. I. M. (2013). Linking cortisol responsiveness and aggressive behaviour in gilthead seabream Sparus aurata: indication of divergent coping styles. Appl. Anim. Behav. Sci. 143, 75–81. doi: 10.1016/j.applanim.2012.11.008
Chapman, B. B., Hulthén, K., Blomqvist, D. R., Hansson, L.-A., Nilsson, J.-Å., Brodersen, J., et al. (2011). To boldly go: individual differences in boldness influence migratory tendency. Ecol. Lett. 14, 871–876. doi: 10.1111/j.1461-0248.2011.01648.x
Ciuti, S., Muhly, T. B., Paton, D. G., McDevitt, A. D., Musiani, M., and Boyce, M. S. (2012). Human selection of elk behavioural traits in a landscape of fear. Proc. R. Soc. B Biol. Sci. 279, 4407–4416. doi: 10.1098/rspb.2012.1483
Clobert, J., Galliard, J.-F. L., Cote, J., Meylan, S., and Massot, M. (2009). Informed dispersal, heterogeneity in animal dispersal syndromes and the dynamics of spatially structured populations. Ecol. Lett. 12, 197–209. doi: 10.1111/j.1461-0248.2008.01267.x
Cooke, S. J., Blumstein, D. T., Buchholz, R., Caro, T., Fernández-Juricic, E., Franklin, C. E., et al. (2014). Physiology, behavior, and conservation. Physiol. Biochem. Zool. 87, 1–14. doi: 10.1086/671165
Cortés-Avizanda, A., Colomer, M. À., Margalida, A., Ceballos, O., and Donázar, J. A. (2015). Modeling the consequences of the demise and potential recovery of a keystone-species: wild rabbits and avian scavengers in Mediterranean landscapes. Sci. Rep. 5:17033. doi: 10.1038/srep17033
Cote, J., and Clobert, J. (2007). Social personalities influence natal dispersal in a lizard. Proc. R. Soc. B Biol. Sci. 274, 383–390. doi: 10.1098/rspb.2006.3734
Cote, J., Clobert, J., Brodin, T., Fogarty, S., and Sih, A. (2010a). Personality-dependent dispersal: characterization, ontogeny and consequences for spatially structured populations. Philos. Trans. R. Soc. B Biol. Sci. 365, 4065–4076. doi: 10.1098/rstb.2010.0176
Cote, J., Fogarty, S., Weinersmith, K., Brodin, T., and Sih, A. (2010b). Personality traits and dispersal tendency in the invasive mosquitofish (Gambusia affinis). Proc. R. Soc. B Biol. Sci. 277, 1571–1579. doi: 10.1098/rspb.2009.2128
Creel, S. (2018). The control of risk hypothesis: reactive vs. proactive antipredator responses and stress-mediated vs. food-mediated costs of response. Ecol. Lett. 21, 947–956. doi: 10.1111/ele.12975
Damsgård, B., Evensen, T. H., Øverli, Ø., Gorissen, M., Ebbesson, L. O. E., Rey, S., et al. (2019). Proactive avoidance behaviour and pace-of-life syndrome in Atlantic salmon. R. Soc. Open Sci. 6:181859. doi: 10.1098/rsos.181859
Daniel, D. K., and Bhat, A. (2020). Bolder and Brighter? Exploring correlations between personality and cognitive abilities among individuals within a population of wild zebrafish, Danio rerio. Front. Behav. Neurosci. 14:138. doi: 10.3389/fnbeh.2020.00138
De Miguel, Z., Vegas, O., Garmendia, L., Arregi, A., Beitia, G., and Azpiroz, A. (2011). Behavioral coping strategies in response to social stress are associated with distinct neuroendocrine, monoaminergic and immune response profiles in mice. Behav. Brain Res. 225, 554–561. doi: 10.1016/j.bbr.2011.08.011
Diaz Pauli, B., and Sih, A. (2017). Behavioural responses to human-induced change: why fishing should not be ignored. Evol. Appl. 10, 231–240. doi: 10.1111/eva.12456
Diaz Pauli, B., Wiech, M., Heino, M., and Utne-Palm, A. C. (2015). Opposite selection on behavioural types by active and passive fishing gears in a simulated guppy Poecilia reticulata fishery. J. Fish Biol. 86, 1030–1045. doi: 10.1111/jfb.12620
Dingemanse, N. J., and de Goede, P. (2004). The relation between dominance and exploratory behavior is context-dependent in wild great tits. Behav. Ecol. 15, 1023–1030. doi: 10.1093/beheco/arh115
Dingemanse, N. J., and Réale, D. (2005). Natural selection and animal personality. Behaviour 142, 1159–1184. doi: 10.1163/156853905774539445
Drent, P. J., van Oers, K., and van Noordwijk, A. J. (2003). Realized heritability of personalities in the great tit (Parus major). Proc. R. Soc. Lond. B Biol. Sci. 270, 45–51. doi: 10.1098/rspb.2002.2168
Ferrari, C., Pasquaretta, C., Carere, C., Cavallone, E., von Hardenberg, A., and Réale, D. (2013). Testing for the presence of coping styles in a wild mammal. Anim. Behav. 85, 1385–1396. doi: 10.1016/j.anbehav.2013.03.030
Ferrari, S., Horri, K., Allal, F., Vergnet, A., Benhaim, D., Vandeputte, M., et al. (2016). Heritability of boldness and hypoxia avoidance in European seabass, Dicentrarchus labrax. PLoS ONE 11:e0168506. doi: 10.1371/journal.pone.0168506
Ferrari, S., Rey, S., Høglund, E., Øverli, Ø., Chatain, B., MacKenzie, S., et al. (2020). Physiological responses during acute stress recovery depend on stress coping style in European sea bass, Dicentrarchus labrax. Physiol. Behav. 216:112801. doi: 10.1016/j.physbeh.2020.112801
Forsman, A., and Wennersten, L. (2016). Inter-individual variation promotes ecological success of populations and species: evidence from experimental and comparative studies. Ecography 39, 630–648. doi: 10.1111/ecog.01357
Found, R., and St. Clair, C. C. (2019). Influences of personality on ungulate migration and management. Front. Ecol. Evol. 7:438. doi: 10.3389/fevo.2019.00438
Gabriel, P. O., and Black, J. M. (2012). Behavioural syndromes, partner compatibility and reproductive performance in Steller's Jays. Ethology 118, 76–86. doi: 10.1111/j.1439-0310.2011.01990.x
Geffroy, B., Bru, N., Dossou-Gbété, S., Tentelier, C., and Bardonnet, A. (2014). The link between social network density and rank-order consistency of aggressiveness in juvenile eels. Behav. Ecol. Sociobiol. 68, 1073–1083. doi: 10.1007/s00265-014-1719-6
Geffroy, B., Sadoul, B., Bouchareb, A., Prigent, S., Bourdineaud, J.-P., Gonzalez-Rey, M., et al. (2018). Nature-based tourism elicits a phenotypic shift in the coping abilities of fish. Front. Physiol. 9:13. doi: 10.3389/fphys.2018.00013
Geffroy, B., Sadoul, B., and Ellenberg, U. (2017). “Physiological and behavioral consequences of human visitation,” in Ecotourism's Promise and Peril, eds D. T. Blumstein, B. Geffroy, D. G. M. Samia, and E. Bessa (Cham: Springer), 9–27.
Geffroy, B., Sadoul, B., Putman, B. J., Berger-Tal, O., Garamszegi, L. Z., Møller, A. P., et al. (2020). Evolutionary dynamics in the Anthropocene: life history and intensity of human contact shape antipredator responses. PLOS Biol. 18:e3000818. doi: 10.1371/journal.pbio.3000818
Geffroy, B., Samia, D. S. M., Bessa, E., and Blumstein, D. T. (2015). How nature-based tourism might increase prey vulnerability to predators. Trends Ecol. Evol. 30, 755–765. doi: 10.1016/j.tree.2015.09.010
Gesto, M., Zupa, W., Alfonso, S., Spedicato, M. T., Lembo, G., and Carbonara, P. (2020). Using acoustic telemetry to assess behavioral responses to acute hypoxia and ammonia exposure in farmed rainbow trout of different competitive ability. Appl. Anim. Behav. Sci. 230:105084. doi: 10.1016/j.applanim.2020.105084
Grunst, A. S., Grunst, M. L., Daem, N., Pinxten, R., Bervoets, L., and Eens, M. (2019a). An important personality trait varies with blood and plumage metal concentrations in a free-living songbird. Environ. Sci. Technol. 53, 10487–10496. doi: 10.1021/acs.est.9b03548
Grunst, A. S., Grunst, M. L., Pinxten, R., and Eens, M. (2019b). Personality and plasticity in neophobia levels vary with anthropogenic disturbance but not toxic metal exposure in urban great tits: urban disturbance, metal pollution and neophobia. Sci. Total Environ. 656, 997–1009. doi: 10.1016/j.scitotenv.2018.11.383
Hale, R., and Swearer, S. E. (2016). Ecological traps: current evidence and future directions. Proc. R. Soc. B Biol. Sci. 283:20152647. doi: 10.1098/rspb.2015.2647
Hammond, T. T., Ortiz-Jimenez, C. A., and Smith, J. E. (2020). Anthropogenic change alters ecological relationships via interactive changes in stress physiology and behavior within and among organisms. Integr. Comp. Biol. 60, 57–69. doi: 10.1093/icb/icaa001
Harris, M. R., and Siefferman, L. (2014). Interspecific competition influences fitness benefits of assortative mating for territorial aggression in eastern bluebirds (Sialia sialis). PLoS ONE 9:e88668. doi: 10.1371/journal.pone.0088668
Harrison, P. M., Gutowsky, L. F. G., Martins, E. G., Patterson, D. A., Cooke, S. J., and Power, M. (2015). Personality-dependent spatial ecology occurs independently from dispersal in wild burbot (Lota lota). Behav. Ecol. 26, 483–492. doi: 10.1093/beheco/aru216
Hebblewhite, M., White, C. A., Nietvelt, C. G., McKenzie, J. A., Hurd, T. E., Fryxell, J. M., et al. (2005). Human activity mediates a trophic cascade caused by wolves. Ecology 86, 2135–2144. doi: 10.1890/04-1269
Heino, M., and Godø, O. R. (2002). Fisheries-induced selection pressures in the context of sustainable fisheries. Bull. Mar. Sci. 70, 639–656.
Huntingford, F. A., Andrew, G., Mackenzie, S., Morera, D., Coyle, S. M., Pilarczyk, M., et al. (2010). Coping strategies in a strongly schooling fish, the common carp Cyprinus carpio. J. Fish Biol. 76, 1576–1591. doi: 10.1111/j.1095-8649.2010.02582.x
Jacquin, L., Petitjean, Q., Côte, J., Laffaille, P., and Jean, S. (2020). Effects of pollution on fish behavior, personality, and cognition: some research perspectives. Front. Ecol. Evol. 8:86. doi: 10.3389/fevo.2020.00086
Kittilsen, S., Johansen, I. B., Braastad, B. O., and Øverli, Ø. (2012). Pigments, parasites and personalitiy: towards a unifying role for steroid hormones? PLoS ONE 7:e34281. doi: 10.1371/journal.pone.0034281
Koolhaas, J. M., de Boer, S. F., Coppens, C. M., and Buwalda, B. (2010). Neuroendocrinology of coping styles: towards understanding the biology of individual variation. Front. Neuroendocrinol. 31, 307–321. doi: 10.1016/j.yfrne.2010.04.001
Koolhaas, J. M., Korte, S. M., De Boer, S. F., Van Der Vegt, B. J., Van Reenen, C. G., Hopster, H., et al. (1999). Coping styles in animals: current status in behavior and stress-physiology. Neurosci. Biobehav. Rev. 23, 925–935. doi: 10.1016/S0149-7634(99)00026-3
Korte, S. M., Ruesink, W., and Blokhuis, H. J. (1998). Heart rate variability during manual restraint in chicks from high- and low-feather pecking lines of laying hens. Physiol. Behav. 65, 649–652. doi: 10.1016/S0031-9384(98)00206-6
Kralj-Fišer, S., Scheiber, I. B. R., Blejec, A., Moestl, E., and Kotrschal, K. (2007). Individualities in a flock of free-roaming greylag geese: behavioral and physiological consistency over time and across situations. Horm. Behav. 51, 239–248. doi: 10.1016/j.yhbeh.2006.10.006
Krebs, C. J., Boutin, S., Boonstra, R., Sinclair, A. R. E., Smith, J. N. M., Dale, M. R. T., et al. (1995). Impact of food and predation on the dnowshoe hare cycle. Science 269, 1112–1115. doi: 10.1126/science.269.5227.1112
Lahti, D. C., Johnson, N. A., Ajie, B. C., Otto, S. P., Hendry, A. P., Blumstein, D. T., et al. (2009). Relaxed selection in the wild. Trends Ecol. Evol. 24, 487–496. doi: 10.1016/j.tree.2009.03.010
Laubu, C., Dechaume-Moncharmont, F.-X., Motreuil, S., and Schweitzer, C. (2016). Mismatched partners that achieve postpairing behavioral similarity improve their reproductive success. Sci. Adv. 2:e1501013. doi: 10.1126/sciadv.1501013
Leclerc, M., Zedrosser, A., Swenson, J. E., and Pelletier, F. (2019). Hunters select for behavioral traits in a large carnivore. Sci. Rep. 9:12371. doi: 10.1038/s41598-019-48853-3
Little, A. G., Fisher, D. N., Schoener, T. W., and Pruitt, J. N. (2019). Population differences in aggression are shaped by tropical cyclone-induced selection. Nat. Ecol. Evol. 3, 1294–1297. doi: 10.1038/s41559-019-0951-x
Madden, J. R., and Whiteside, M. A. (2014). Selection on behavioural traits during ‘unselective’harvesting means that shy pheasants better survive a hunting season. Anim. Behav. 87, 129–135. doi: 10.1016/j.anbehav.2013.10.021
Merrick, M. J., and Koprowski, J. L. (2017). Should we consider individual behavior differences in applied wildlife conservation studies? Biol. Conserv. 209, 34–44. doi: 10.1016/j.biocon.2017.01.021
Mettke-Hofmann, C., Wink, M., Winkler, H., and Leisler, B. (2005). Exploration of environmental changes relates to lifestyle. Behav. Ecol. 16, 247–254. doi: 10.1093/beheco/arh159
Mills, L. S., Soulé, M. E., and Doak, D. F. (1993). The keystone-species concept in ecology and conservation. BioScience 43, 219–224. doi: 10.2307/1312122
Miranda, A. C., Schielzeth, H., Sonntag, T., and Partecke, J. (2013). Urbanization and its effects on personality traits: a result of microevolution or phenotypic plasticity? Glob. Change Biol. 19, 2634–2644. doi: 10.1111/gcb.12258
Modlmeier, A. P., Keiser, C. N., Watters, J. V., Sih, A., and Pruitt, J. N. (2014). The keystone individual concept: an ecological and evolutionary overview. Anim. Behav. 89, 53–62. doi: 10.1016/j.anbehav.2013.12.020
Moiron, M., Laskowski, K. L., and Niemelä, P. T. (2020). Individual differences in behaviour explain variation in survival: a meta-analysis. Ecol. Lett. 23, 399–408. doi: 10.1111/ele.13438
Monestier, C., Morellet, N., Gaillard, J.-M., Cargnelutti, B., Vanpé, C., and Hewison, A. J. M. (2015). Is a proactive mum a good mum? A mother's coping style influences early fawn survival in roe deer. Behav. Ecol. 26, 1395–1403. doi: 10.1093/beheco/arv087
Moran, N. A. (1992). The evolutionary maintenance of alternative phenotypes. Am. Nat. 139, 971–989. doi: 10.1086/285369
Murren, C. J., Auld, J. R., Callahan, H., Ghalambor, C. K., Handelsman, C. A., Heskel, M. A., et al. (2015). Constraints on the evolution of phenotypic plasticity: limits and costs of phenotype and plasticity. Heredity 115, 293–301. doi: 10.1038/hdy.2015.8
Navas González, F. J., Jordana Vidal, J., León Jurado, J. M., Arando Arbulu, A., McLean, A. K., and Delgado Bermejo, J. V. (2018). Genetic parameter and breeding value estimation of donkeys' problem-focused coping styles. Behav. Processes 153, 66–76. doi: 10.1016/j.beproc.2018.05.008
Nussey, D. H., Postma, E., Gienapp, P., and Visser, M. E. (2005). Selection on heritable phenotypic plasticity in a wild bird population. Science 310, 304–306. doi: 10.1126/science.1117004
Øverli, Ø., and Sørensen, C. (2016). On the role of neurogenesis and neural plasticity in the evolution of animal personalities and stress coping styles. Brain Behav. Evol. 87, 167–174. doi: 10.1159/000447085
Øverli, Ø., Sørensen, C., and Nilsson, G. E. (2006). Behavioral indicators of stress-coping style in rainbow trout: do males and females react differently to novelty? Physiol. Behav. 87, 506–512. doi: 10.1016/j.physbeh.2005.11.012
Øverli, Ø., Sørensen, C., Pulman, K. G. T., Pottinger, T. G., Korzan, W., Summers, C. H., et al. (2007). Evolutionary background for stress-coping styles: relationships between physiological, behavioral, and cognitive traits in non-mammalian vertebrates. Neurosci. Biobehav. Rev. 31, 396–412. doi: 10.1016/j.neubiorev.2006.10.006
Paine, R. T. (1995). A conversation on refining the concept of keystone species. Conserv. Biol. 9, 962–964. doi: 10.1046/j.1523-1739.1995.09040962.x
Partecke, J., Schwabl, I., and Gwinner, E. (2006). Stress and the city: urbanization and its effects on the stress physiology in European blackbirds. Ecology 87, 1945–1952. doi: 10.1890/0012-9658(2006)87[1945:SATCUA]2.0.CO;2
Peterson, E. K., Buchwalter, D. B., Kerby, J. L., LeFauve, M. K., Varian-Ramos, C. W., and Swaddle, J. P. (2017). Integrative behavioral ecotoxicology: bringing together fields to establish new insight to behavioral ecology, toxicology, and conservation. Curr. Zool. 63, 185–194. doi: 10.1093/cz/zox010
Podgorniak, T., Blanchet, S., De Oliveira, E., Daverat, F., and Pierron, F. (2016). To boldly climb: behavioural and cognitive differences in migrating European glass eels. R. Soc. Open Sci. 3:150665. doi: 10.1098/rsos.150665
Pruitt, J. N., and Keiser, C. N. (2014). The personality types of key catalytic individuals shape colonies' collective behaviour and success. Anim. Behav. 93, 87–95. doi: 10.1016/j.anbehav.2014.04.017
Qu, J., Fletcher, Q. E., Réale, D., Li, W., and Zhang, Y. (2018). Independence between coping style and stress reactivity in plateau pika. Physiol. Behav. 197, 1–8. doi: 10.1016/j.physbeh.2018.09.007
Réale, D., Dingemanse, N. J., Kazem, A. J. N., and Wright, J. (2010a). Evolutionary and ecological approaches to the study of personality. Philos. Trans. R. Soc. B Biol. Sci. 365, 3937–3946. doi: 10.1098/rstb.2010.0222
Réale, D., Garant, D., Humphries, M. M., Bergeron, P., Careau, V., and Montiglio, P.-O. (2010b). Personality and the emergence of the pace-of-life syndrome concept at the population level. Philos. Trans. R. Soc. B Biol. Sci. 365, 4051–4063. doi: 10.1098/rstb.2010.0208
Réale, D., and Montiglio, P.-O. (2020). “Evolution of adaptive individual differences in non-human animals,” in Adaptive Shyness: Multiple Perspectives on Behavior and Development, eds L. A. Schmidt and K. L. Poole (Cham: Springer International Publishing), 279–299.
Reimers, E., Loe, L. E., Eftestøl, S., Colman, J. E., and Dahle, B. (2009). Effects of hunting on response behaviors of wild reindeer. J. Wildl. Manag. 73, 844–851. doi: 10.2193/2008-133
Rhoades, O. K., Lonhart, S. I., and Stachowicz, J. J. (2019). Human-induced reductions in fish predator boldness decrease their predation rates in kelp forests. Proc. R. Soc. B Biol. Sci. 286:20182745. doi: 10.1098/rspb.2018.2745
Rice, K. J., and Emery, N. C. (2003). Managing microevolution: restoration in the face of global change. Front. Ecol. Environ. 1, 469–478. doi: 10.1890/1540-9295(2003)001[0469:MMRITF]2.0.CO;2
Roche, D. G., Careau, V., and Binning, S. A. (2016). Demystifying animal ‘personality’ (or not): why individual variation matters to experimental biologists. J. Exp. Biol. 219, 3832–3843. doi: 10.1242/jeb.146712
Ruiz-Gomez, M. L., Huntingford, F. A., Øverli, Ø., Thörnqvist, P.-O., and Höglund, E. (2011). Response to environmental change in rainbow trout selected for divergent stress coping styles. Physiol. Behav. 102, 317–322. doi: 10.1016/j.physbeh.2010.11.023
Ruiz-Gomez, M. L., Kittilsen, S., Höglund, E., Huntingford, F. A., Sørensen, C., Pottinger, M. J., et al. (2008). Behavioral plasticity in rainbow trout (Oncorhynchus mykiss) with divergent coping styles: when doves become hawks. Horm. Behav. 54, 534–538. doi: 10.1016/j.yhbeh.2008.05.005
Saaristo, M., Brodin, T., Balshine, S., Bertram, M. G., Brooks, B. W., Ehlman, S. M., et al. (2018). Direct and indirect effects of chemical contaminants on the behaviour, ecology and evolution of wildlife. Proc. R. Soc. B Biol. Sci. 285:20181297. doi: 10.1098/rspb.2018.1297
Samia, D. S. M., Nakagawa, S., Nomura, F., Rangel, T. F., and Blumstein, D. T. (2015). Increased tolerance to humans among disturbed wildlife. Nat. Commun. 6:8877. doi: 10.1038/ncomms9877
Sih, A. (2013). Understanding variation in behavioural responses to human-induced rapid environmental change: a conceptual overview. Anim. Behav. 85, 1077–1088. doi: 10.1016/j.anbehav.2013.02.017
Sih, A., Bell, A., and Johnson, J. C. (2004). Behavioral syndromes: an ecological and evolutionary overview. Trends Ecol. Evol. 19, 372–378. doi: 10.1016/j.tree.2004.04.009
Sih, A., Cote, J., Evans, M., Fogarty, S., and Pruitt, J. (2012). Ecological implications of behavioural syndromes. Ecol. Lett. 15, 278–289. doi: 10.1111/j.1461-0248.2011.01731.x
Sih, A., Ferrari, M. C. O., and Harris, D. J. (2011). Evolution and behavioural responses to human-induced rapid environmental change. Evol. Appl. 4, 367–387. doi: 10.1111/j.1752-4571.2010.00166.x
Slabbekoorn, H., and Peet, M. (2003). Ecology: birds sing at a higher pitch in urban noise. Nature 424:267. doi: 10.1038/424267a
Smith, B. R., and Blumstein, D. T. (2008). Fitness consequences of personality: a meta-analysis. Behav. Ecol. 19, 448–455. doi: 10.1093/beheco/arm144
Smith, B. R., and Blumstein, D. T. (2012). Structural consistency of behavioural syndromes: does predator training lead to multi-contextual behavioural change? Behaviour 149, 187–213. doi: 10.1163/156853912X634133
Smith, B. R., and Blumstein, D. T. (2013). Animal personalities and conservation biology. Anim. Personal. Behav. Physiol. Evol. 379–411. doi: 10.7208/CHICAGO/9780226922065.003.0014
Sol, D., Lapiedra, O., and González-Lagos, C. (2013). Behavioural adjustments for a life in the city. Anim. Behav. 85, 1101–1112. doi: 10.1016/j.anbehav.2013.01.023
Sol, D., Maspons, J., Gonzalez-Voyer, A., Morales-Castilla, I., Garamszegi, L. Z., and Møller, A. P. (2018). Risk-taking behavior, urbanization and the pace of life in birds. Behav. Ecol. Sociobiol. 72:59. doi: 10.1007/s00265-018-2463-0
Taff, C. C., and Vitousek, M. N. (2016). Endocrine flexibility: optimizing phenotypes in a dynamic world? Trends Ecol. Evol. 31, 476–488. doi: 10.1016/j.tree.2016.03.005
Tan, H., Polverino, G., Martin, J. M., Bertram, M. G., Wiles, S. C., Palacios, M. M., et al. (2020). Chronic exposure to a pervasive pharmaceutical pollutant erodes among-individual phenotypic variation in a fish. Environ. Pollut. 263:114450. doi: 10.1016/j.envpol.2020.114450
Tuomainen, U., and Candolin, U. (2011). Behavioural responses to human-induced environmental change. Biol. Rev. 86, 640–657. doi: 10.1111/j.1469-185X.2010.00164.x
Turner, J. W., LaFleur, R. M., Richardson, A. T., and Holekamp, K. E. (2020). Risk-taking in free-living spotted hyenas is associated with anthropogenic disturbance, predicts survivorship, and is consistent across experimental contexts. Ethology 126, 97–110. doi: 10.1111/eth.12964
Twiss, S. D., Shuert, C. R., Brannan, N., Bishop, A. M., and Pomeroy, P. P. (2020). Reactive stress-coping styles show more variable reproductive expenditure and fitness outcomes. Sci. Rep. 10:9550. doi: 10.1038/s41598-020-66597-3
Uusi-Heikkilä, S., Wolter, C., Klefoth, T., and Arlinghaus, R. (2008). A behavioral perspective on fishing-induced evolution. Trends Ecol. Evol. 23, 419–421. doi: 10.1016/j.tree.2008.04.006
van Baaren, J., and Candolin, U. (2018). Plasticity in a changing world: behavioural responses to human perturbations. Curr. Opin. Insect Sci. 27, 21–25. doi: 10.1016/j.cois.2018.02.003
Vargas, R., Balasch, J. C., Brandts, I., Reyes-López, F., Tort, L., and Teles, M. (2018). Variations in the immune and metabolic response of proactive and reactive Sparus aurata under stimulation with Vibrio anguillarum vaccine. Sci. Rep. 8:17352. doi: 10.1038/s41598-018-35863-w
Verbeek, M. E., Drent, P. J., and Wiepkema, P. R. (1994). Consistent individual differences in early exploratory behaviour of male great tits. Anim. Behav. 48, 1113–1121. doi: 10.1006/anbe.1994.1344
White, T. H. Jr, Collazo, J. A., Dinsmore, S. J., and Llerandi-Roman, I. C. (2014). Niche Restriction and Conservatism in a Neotropical Psittacine: The Case of the Puerto Rican Parrot. Available online at: https://pubs.er.usgs.gov/publication/70191982 (accessed November 2, 2020).
White, S. L., Wagner, T., Gowan, C., and Braithwaite, V. A. (2017). Can personality predict individual differences in brook trout spatial learning ability? Behav. Processes 141, 220–228. doi: 10.1016/j.beproc.2016.08.009
Whitehead, A., Clark, B. W., Reid, N. M., Hahn, M. E., and Nacci, D. (2017). When evolution is the solution to pollution: key principles, and lessons from rapid repeated adaptation of killifish (Fundulus heteroclitus) populations. Evol. Appl. 10, 762–783. doi: 10.1111/eva.12470
Wong, B. B. M., and Candolin, U. (2015). Behavioral responses to changing environments. Behav. Ecol. 26, 665–673. doi: 10.1093/beheco/aru183
Wong, R. Y., French, J., and Russ, J. B. (2019). Differences in stress reactivity between zebrafish with alternative stress coping styles. R. Soc. Open Sci. 6:181797. doi: 10.1098/rsos.181797
Yuan, M., Chen, Y., Huang, Y., and Lu, W. (2018). Behavioral and metabolic phenotype indicate personality in zebrafish (Danio rerio). Front. Physiol. 9:653. doi: 10.3389/fphys.2018.00653
Keywords: coping style, antipredator behavior, evolution, stress physiology, ecology, predation, urbanization, pollution
Citation: Geffroy B, Alfonso S, Sadoul B and Blumstein DT (2020) A World for Reactive Phenotypes. Front. Conserv. Sci. 1:611919. doi: 10.3389/fcosc.2020.611919
Received: 29 September 2020; Accepted: 18 November 2020;
Published: 16 December 2020.
Edited by:
Carlos R. Ruiz-Miranda, State University of the North Fluminense Darcy Ribeiro, BrazilReviewed by:
Thomas H. White, United States Fish and Wildlife Service, Puerto RicoColleen Cassady St. Clair, University of Alberta, Canada
Copyright © 2020 Geffroy, Alfonso, Sadoul and Blumstein. This is an open-access article distributed under the terms of the Creative Commons Attribution License (CC BY). The use, distribution or reproduction in other forums is permitted, provided the original author(s) and the copyright owner(s) are credited and that the original publication in this journal is cited, in accordance with accepted academic practice. No use, distribution or reproduction is permitted which does not comply with these terms.
*Correspondence: Benjamin Geffroy, benjamin.geffroy@ifremer.fr; Sébastien Alfonso, salfonso@coispa.eu; Bastien Sadoul, bastien.sadoul@agrocampus-ouest.fr; Daniel T. Blumstein, marmots@ucla.edu