- The Swiss Institute for Desert Energy and Environmental Research, Blaustein Institutes for Desert Research, Ben-Gurion University of the Negev, Beer-Sheva, Israel
While road-side productivity attracts wildlife, roads are also a major cause of mortality. Thus, roads are potentially an attractive sink. We investigated whether roads in a desert environment in southern Israel act as an ecological trap for the territorial mourning wheatear (Oenanthe lugens). We applied an individual-based mechanistic approach to compare the apparent survival of individually-marked wheatears between roadside territories and territories in natural habitats farther away from the road, and determined directionality in territorial shifting to and from the road. Analysis was based on mark-resight techniques and multi-model inference in a multi-strata approach (program MARK). Wheatear survival in road-side territories was too low to be compensated by the maximum possible recruitment, but shifted territories from natural habitat toward the roadside habitat as these territories were vacated by mortality. Vacated territories along the road were re-occupied faster than vacated territories in natural habitat. Thus, the roadside habitat in our study area fulfilled all conditions for an ecological trap. Roads may act as widespread ecological traps and their impact, therefore, may extend well-beyond the existing perception of narrow dissecting elements causing local mortality and/or animal avoidance. In species where habitat selection is based on contest competition (e.g., territorial species) and contest success has a genetically heritable component, ecological traps will induce a paradoxical selection process.
Introduction
Roads cover only a small fraction of the planet's land surface (~0.5%), yet exert a considerable impact on biodiversity due to fragmentation, road-kills, contamination and habitat modification (Brady and Richardson, 2017). Many studies demonstrate that road-side productivity and plant diversity attracts wildlife while at the same time inflicting mortality (Coffin, 2007). Reduced survival rates along roads are not only caused by vehicle collisions, but are also induced by indirect factors stemming from the specific conditions created by the road (e.g., increased presence of predators—Gates and Gysel, 1978; Blouin-Demers and Weatherhead, 2001). If mortality rates in populations inhabiting roadside habitats are not compensated by improved recruitment stemming from the high roadside productivity, then roadside habitats become attractive sinks—i.e., ecological traps (Coffin, 2007). Considering the abundance of roads and the evidence regarding their attractiveness and impact on survival to wildlife only few empirical studies have focused specifically on roads as ecological traps (Norris et al., 2013; Egri et al., 2017), although many studies speculate they may act as such.
Theoretical work suggests that ecological traps can have a considerable negative impact on the viability of populations (Fletcher et al., 2012). Nevertheless, there are still few empirical examples of ecological traps that provide insight into the mechanisms underlying their formation (Robertson et al., 2013). Ecological traps are expected to evolve as a result of rapid habitat alteration decoupling the cues animals use to assess habitat quality from their actual quality. Consequently, habitats that are poor and cannot support a viable population appear to animals as good habitats and attract them (Schlaepfer et al., 2002). This can occur when a good habitat is degraded but the cues the animals use to assess it are maintained (e.g., Hawlena et al., 2010), and/or when cues are created in a poor habitat that are falsely interpreted by animals to be signals of quality (Schlaepfer et al., 2002).
Ecological traps occur primarily in anthropogenically-modified habitats. They are generally perceived as an insular issue resulting from a unique combination of conditions. However, it is possible that certain, relatively common anthropogenic habitat modifications such as roads (Forman, 1998) may be prone toward generating ecological traps. Therefore, traps may be far more pervasive throughout the landscape than currently realized (Battin, 2004).
Here we report a study demonstrating that typical road conditions generate “severe traps” (as opposed to equal-preference traps—Robertson et al., 2013). The study focused on the territorial mourning wheatear (Oenanthe lugens) population in the Negev Desert highlands, Israel. In deserts, the richer vegetation along roadside ditches supports a rich population of insects that is expected to be attractive for the wheatears. However, residing along the roadside is risky, not only because of vehicle-related mortality and increased risk of predation by reptiles attracted to this habitat (Klauber, 1939; Rosen and Lowe, 1994), but also because preliminary observations suggested that wheatears tend to get trapped inside the poles of roadside signposts.
To understand the mechanics of such a process in an ecological-trap scenario, an individual-based approach is required that focuses on preference measures such as settlement order or sequential patch occupancy (Arlt and Pärt, 2007). An individual-based mechanistic approach is important because it reveals potential mitigation solutions (Gilroy and Sutherland, 2007; Robertson, 2012) and provides better insight into the rapidity (and therefore the risk involved) of the process as well as what are the specific attributes that make the trap attractive. Following Robertson and Hutto (2006) we addressed the three basic questions necessary to determine the existence of an ecological trap: (1) Do the wheatears prefer the roadside habitat over natural habitats farther away from the road? (2) Does a reliable surrogate of fitness differ between the roadside habitats and the natural habitats? (3) Is the fitness outcome of settling along the road lower than in the natural habitats and does it indicate a negative population growth rate? We addressed these questions by tracking individual birds over time and in doing so elucidating the behavioral mechanisms underlying the process of entrapment.
Methods
Study Area
The mourning wheatear is common territorial insectivore occupying high rocky mountainsides, rocky slopes, and dry riverbeds. The species ranges over North Africa to Sinai, Saudi Arabia and the Persian Gulf. In Israel, it is found in the highlands and rocky areas of the Negev desert and the Arava valley. Mourning wheatears nest in cavities, mainly under rocks. Breeding season is roughly mid-March to end of June and most pairs are double brooders, producing 4–5 eggs with usually 2–3 chicks that fledge in each brood (Shirihai, 1996). Wheatears pair for the season and both parents contribute to raising the chicks, so both parents must survive through the nesting cycle for the chicks to fledge.
Our study was carried out from May 2009 to May 2011. The study area was located in the Negev highlands, Israel, north west of the town of Mitzpe Ramon, along road # 171 from the 6th km [E669052 N(3)390554 UTM] to the 12th km [E665610 N(3)387070 UTM] west of road # 40 at the northern edge of Mt. Negev nature reserve. In this biome the preferred wheatear habitat is dry desert riverbeds (Shirihai, 1996) with a diverse vegetative community, rich with insects (Krasnov and Shenbrot, 1996) and good perches. The study area included two 2-km road sections, 750 m away from each other. Like all desert roads, roadside ditches in our study area were visibly more productive (in terms of vegetation cover) than the surrounding landscape due to runoff from the road and blockage of surface flow (Holzaphel and Schmidt, 1990; Abd El-Ghani, 1998). The high vegetation cover supports an abundance of insects and emulates the preferred habitat of a dry riverbed (Brooks and Lair, 2005). In each section we identified and delineated wheatear territories (see below) and classified them as either: roadside habitat (RSH)—territories traversed by the road, or territories in “natural habitat” (NH)—territories located within a dry riverbed 300–1,200 m away from the road.
Defining Territories, Trapping, and Marking
Mourning wheatears do not cross the border with neighboring territories. We carried out weekly transects on foot in the study area at the early-mid breeding season (May–August 2009 and March–August 2010). Each sighted unmarked wheatear was followed on foot repeatedly flushing it. In this manner we could determine whether the bird was territorial and its territorial boundaries. Specifically, each time the bird made a turn to avoid a neighboring territory, we recorded the location with a GPS. We also recorded locations of observed aggressive interactions between neighboring birds. For each bird we obtained ≥12 locations. These locations were then placed on a map to reveal the territory borders and classified each territory as either RSH or NH. Territory size was calculated by connecting the outermost points of all locations into a convex polygon and calculating the area within this polygon. To determine if NH territories actually reflect the common natural territory of the species in terms of size, we surveyed natural areas 5–10 km south of the road and using the same technique estimated territory sizes of sighted wheatears.
After territorial boundaries of a specific individual became apparent, we placed a half-domed spring trap with a 40 cm diameter within the assumed territory. The traps were baited with beetle larva. Captured wheatears were individually marked with one numbered standard ornithologist's metal ring, and three plastic rings with a unique color combination allowing individual identification each time the bird was re-sighted. Trapping was carried out continuously throughout the study.
Habitat Preference
Marked individuals were relocated weekly from May 2009 to February 2010 and from March 2010 to February 2011, using NIKON 12 × 42 binoculars. We devoted 15 min to each known territory to relocate the marked individuals associated with the territory. We recorded the position of relocated individuals to the nearest 3 m using an ArcGIS GPS program on a palm top computer. This information helped validate the pre-tagging territory assessment. Individuals not re-sighted during this time were marked as absent. If an individual was sighted in a territory that was not its own for more than 2 consecutive weeks, we considered it as a territorial shift. The new territory was then delineated as described above and classified as RSH or NH and whether it was the first, second, or third territory of the season (we never recorded more than two shifts/individual/season). During the weekly relocations, attention was payed to the presence of marked birds in the immediate surroundings of each section to detect birds that have shifted their territory to outside of the study area.
We predicted that the high productivity of the RSH would attract individual wheatears and, therefore, territorial shifts would be mostly from NH to RSH. However, such directional shifting may also occur if the wheatears recognize the risks associated with RSH so competition for NH will be fiercer forcing a directional movement from NH to RSH due to despotic activity; i.e., the RSH is a regular sink (Gundersen et al., 2001) and as RSH territories are vacated due to high mortality, their reoccupation is caused by subordinate individuals from the NH territories being expelled by dominant competitors. To validate that a directional shift in territories is by choice, we monitored the sequence of the territorial establishment at the onset of the 2010 breeding, and the time it took during the breeding season for abandoned territories in both habitats to become re-occupied. The presence of vacant territories and slow re-occupation in one habitat while the other is fully occupied but has a faster turnover, combined with a directional shift in territories toward the more saturated habitat, provides the necessary evidence of a preferred habitat.
We complimented the dynamics of territory-holding with an analysis of territory size in the RSH vs. NH. Territory size is a proxy of habitat productivity and/or intraspecific competition (Hixon, 1980), where smaller territories indicate more resources and more competition. Although this is not a condition for determining ecological traps, it provides additional evidence regarding territorial quality in terms of available resources, which is indicative of the attractiveness of a given area.
Assessing Population Growth Rate
We used mark/resight techniques to assess apparent survival of individuals occupying RSH vs. NH territories. In addition, we searched for nests and monitored the number of breeding cycles and chicks per nest. We used these data as a proxy for recruitment in the RSH vs. NH. We estimated recruitment as the expected number of fledglings/pair/breeding cycle in the successful nests multiplied by the probability of both parents surviving a single cycle. This is, of course, an overestimate of recruitment, as not all fledglings survive to breed successfully. By combining survival and the proxy for recruitment we could compare population growth rates between the two territory types and determine if growth rate was negative in any of the habitats. If our overestimate of recruitment would still be too low to compensate for adult mortality, this would be conclusive evidence of a sink population.
Data Analysis
Apparent survival and territorial shifts were estimated using the multi-state module in Program MARK (White and Burnham, 1999; Doherty and Grubb, 2002), based on capture/resight of ringed individuals from the population in the study area. The estimation uses maximum likelihood for fitting potential predictor models to the data, and provides estimates for the models' parameters. Models were ranked using multi-model inference (Burnham and Anderson, 1998) based on an information-theoretic approach and Akaike's Information Criteria (AIC). In this approach, the probability of an individual being sighted is a combination of two parameters: (φ)—the probability the bird survived and remained in the sample area from one survey to the next, and (p)—the probability that the bird was encountered conditional on being alive and in the sample area (White and Burnham, 1999).
In the MARK multi-state module Ψ represents the probability of moving among strata. Strata are discrete locations, states, or conditions, in which the marked individual may potentially be encountered, conditional on being alive and in that stratum. In this study the strata are the territories and Ψ is the probability of abandoning a former territory and establishing a new one.
In this study four strata inscribed “A,” “B,” “C,” “D” were defined: The first territory established by an individual in a given habitat, either RSH or NH, was inscribed as “A” or “B,” respectively. If an individual shifted a territory within the habitat the second territory was assigned “C” or “D” for RSH or NH, respectively. If a bird shifted a territory from one habitat to another, then the new territory was considered a first territory in that specific habitat and inscribed, depending on the habitat, as “A” or “B.” In this study birds never shifted their territory more than once per season within a habitat. Thus, within this constellation, six types of territorial shifts were possible (Figure 1): A →C, B →D, A →B, B →A, D →A, and C →B. Territorial shift from D →A and C →B were defined as having equal probabilities as B →A and A →B, respectively (i.e., the probability of shifting between habitats is the same if done from the first or second territory the current habitat). Under this scheme, territory shifts from B →C, A →D, C →D, or D →C are not possible as they reflect shifts directly into a second territory in the other habitat, and their probability in the analyses, therefore, was fixed to zero. Similarly, because territorial shifts within a habitat never occurred more than once, territory shifts of C→A and D→B were not possible and were fixed to zero as well.
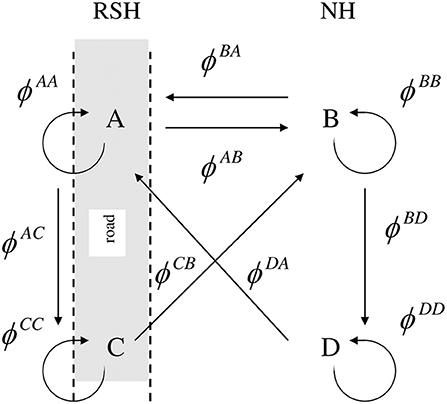
Figure 1. Transition options (territorial shifts) between the four strata (territory types) with arrows indicating the possible transitions: Roadside Habitat (RSH) first (A) and second (C) territory of the season, and Natural Habitat (NH) first (B) and second (D) territory of the season. ϕ values are the probabilities of both surviving and moving and can be decomposed into its components. Assuming that survival from time i to i+1 does not depend on stratum at time i+1, then where is the probability of survival from time i to i + 1, given that the individual is in state r at time i, and is the conditional probability that a bird in stratum r at time i is in stratum s at time i+1, given that it is alive at i+1.
We estimated survival and territorial-shifting probabilities using weighted model averaging (Anderson, 2008) across all models considered. Predictors used in the analysis included: road sections, years, territory location (RSH vs. NH) and season (dry season up to December and wet season from December).
Roadside Signposts as a Cause of Mortality
Sign posts in the study area are typically 10–15 cm diameter, 2 m high uncapped steel tubes. This design is commonly used in many countries for roadside posting. Wheatears (and other cavity nesters) get trapped within them presumably because they enter them in search of a nesting cavity and cannot exit because they are unable to spread their wings. While we were unable to assess most specific causes of mortality (vehicle collisions, predation, or disease), we were able to quantify mortality due to entrapment in signposts by withdrawing carcasses from within the posts along the studied road sections. To estimate mortality rate due to signposts alone, we extracted wheatear carcasses from all posts in the study area at the end of the study, and identified those individual marked during the study. We then used the “dead recoveries only” module in program MARK to assess the rates of signpost-related mortality setting the week after their last sighting as the time of mortality.
Results
We marked 96 wheatears: 48 were classified as holding RSH territories and 48 as NH. Observations made on five nesting pairs (two RSH and three NH) during the spring-summer 2010 breeding season (following a high-precipitation/high-productivity winter) indicated that there were three breeding cycles with an average of 3 fledglings per cycle per pair. In the 2011 breeding season (following an extremely dry winter), four nesting pairs were observed (one RSH and three NH) with an average of 1.5 fledglings per pair and only one cycle.
Territories
The two sections studied supported up to 32 known territories of individuals or pairs of mourning wheatears. Territories averaged 8.4 ha. (n = 31). RSH territories were considerably smaller than NH territories (p < 0.0001, 3.7 ha. ±1.8 vs. 11.4 ha. ±5.3, n = 12 and n = 19, respectively). One natural habitat territory flanked a vineyard and therefore, could not defensibly be considered as NH and was excluded from this analysis. The size of territories observed outside the research area averaged 16.8 ha. (n = 5).
Survival and Territorial Shifts
Fourteen different models of survival and movement were tested for likelihood using the multi-strata module in Program MARK. The three best models are essentially not distinguishable (ΔAIC <0.4), and together carry over 70% of the weight (Table 1).
In terms of survival, the type of habitat (RSH vs. NH) was included in all six leading models composing >99% of the weight. Models that assumed a difference in survival between RSH and NH occupants were more than 83,000 times more likely to represent the observed data than models assuming equal survival. After model averaging, weekly survival probability was 0.9665 in NH territories (SE 0.0074, 95% CI for Wgt. Ave. Est. is 0.9482–0.9785) vs. 0.8847 in RSH territories (SE 0.0177, 95% CI for Wgt. Ave. Est. 0.8449–0.9154).
The structure of the three leading models was very similar also in terms of weekly probabilities of shifting a territory. The only difference between these three models was in the probabilities of territorial shifts within a habitat, i.e., p(B→D) was either the same as p(A→C) (model 1) same as p(B→A) (model 2), or unique (model 3). Calculating the likelihood ratio between the leading model and the 4th (first one to impart movement between RSH and NH both ways) shows the 1st is 2.65 times more likely.
Detected territorials shifts were overwhelmingly to nearby territories which, presumably, could be evaluated by the shifting bird. We documented no shifts between the two studied sections. After model averaging, weekly probabilities of a territorial shift (Ψ) were lowest for movements from a first or second territory in RSH to a territory in the NH [(A→B) = (C→B) = 0.0062; SE = 0.0034] (Figure 2). By contrast, weekly probabilities of a territorial shift from first or second territory in the NH to a territory in RSH were nearly three-fold [(B→A) = 0.0174; SE = 0.0034 and (D→A) = 0.01740; SE = 0.0048, respectively]. The probabilities of shifting from a first to second territory within the habitats were intermediate [with (A→C) = 0.0073; SE = 0.0034 and (B→D) = 0.0106; SE = 0.0035]. The “road section” parameter was not an important factor in terms of territory shifting or survival.
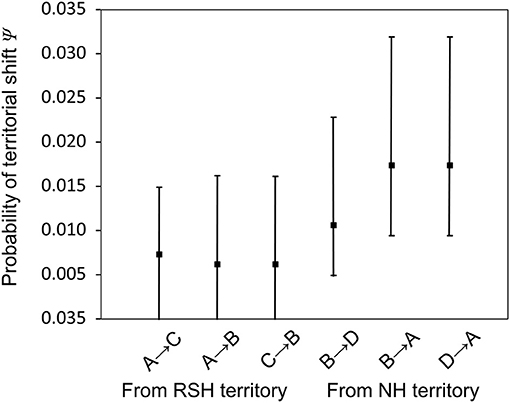
Figure 2. Weekly probability of territorial shifts (Ψ) and their 95% confidence interval based on model averaging. (A,C) Are first and second territory in the roadside habitat (RSH) and (B,D) are first and second territory in the natural habitat (NH), respectively. Arrows indicate the direction of shift, thus A→C and B→D are shift from a first to second territory within habitat and the others are between habitats.
Establishing Territories at the Beginning of the Season and the Reoccupation of Abandoned Territories
Territories were established during March of both study years. The establishment was rapid, occurring within 1–2 weeks. Because resighting attempts were on a weekly schedule we could not, at this resolution, discern differences in time of establishment between NH and RSH territories. However, abandoned NH territories were reoccupied after a minimum of 3 weeks. In 31% of the cases, the territory remained vacant until the end of the breeding season. By contrast, RSH territories were re-occupied in 92.8% of the cases within <2 weeks and only in 7.2% of cases RSH territories remained unoccupied.
The Role of Signposts
Carcass counts in the 31 signposts along the roadside in the study area produced a total of 478 bird skulls in 23 of the 31 posts [eight posts were corked by house sparrow (Passer domesticus) nests]. Of these, 298 were identified as mourning wheatear. One hundred and seventy four skulls were classified as house sparrow (Passer domesticus), five skulls were of kestrel (Falco tinnunculus), and one owl (Athene noctua).
Seventeen of the wheatears found in the signposts were birds marked during the study and accounted for 16.3% of the total marked birds (N = 96) in the research area. Twelve of the 17 were originally captured in RSH territories. The remaining five individuals were originally captured in NH territories and we have no record of them changing territory in our observations prior to their disappearance, suggesting that either they performed a foray to the road or were trapped immediately after shifting to a RSH territory.
Weekly mortality in signposts of RSH residents was at least 3% (i.e., estimated survival = 0.9704, calculated only for known RSH territory holders using MARK “Dead recoveries only” module with SE = 0.01336, and 95% CI 0.9295–0.9879). When considering overall weekly RSH survival from the multi strata analysis (Survival = 0.8847, 95% CI for Wgt. Ave. Est. 0.8449–0.9154), then the posts account for roughly 25% of the mortality. The high end CI survival rate in the RSH translates into 8.46% weekly mortality while low end survival rate translates into 15.51% mortality (95% CI boundaries). Given that mortality due to entrapment in the signpost tubes is at least 1.21% and at most 7% (95% CI boundaries) of the population per week, then the signposts account for 7.8% (1.21/15.51) – 82.35% (7/8.46) of all mortalities. Although the range is large, the extremes are unlikely (as they are the squared upper end percentile −0.0252) and the conclusion is that the signposts account for only part of the mortalities but likely present a substantial threat to wheatear survival along the road.
Discussion
Is the Road in the Study Site an Ecological Trap for Morning Wheatears?
For an ecological traps to form, individual fitness at the given site must be <1 and individuals must be attracted to the site because it is viewed as good-as or better than the surrounding habitat (Delibes et al., 2001; Donovan and Thompson, 2001; Schlaepfer et al., 2002; Battin, 2004).
Survival in this study is apparent and maybe underestimated if birds shifted territories to outside the study area. However, we believe this bias is minor as territorial shifts were: (a) overwhelmingly to nearby territories that were vacated, (b) we regularly monitored the areas adjacent to the outer most territories in the study site, (c) we detected no shifts between the two study sections, and (d) horizontal shifts (along the road) were rare even though territories were vacated. Furthermore, such a bias would be stronger in the NH territories, as they were abandoned more often, and a substantial part of the reduced survival in RSH was accounted for by detected mortality in the sign posts.
Approximately 40 days (5.7 weeks) are necessary before wheatear hatchlings become independent. Both parents must survive this time period for the young to fledge. The probability that a parent in the RSH survives the 5.7 weeks of nesting (pi) is pw5.7 = 0.5 (pwis weekly survival = 0.8847). Because both parents must survive to the fledging stage, the probability of a nest reaching this point successfully is pi2 = 0.25. Assuming n is the number of pairs in the population and X is the number of fledglings per successful pair, n(pi2)X will be total fledglings per one nesting cycle. The probability that an individual adult does not survive to the fledging stage of its young is 1– pi, so total adult mortality in 5.7 weeks of nesting is 2n(1– pi).
For the population to end a breeding cycle having the same amount of individuals when entering the cycle, the number of fledgling added (recruitment) must equal to the number of adults that died. Thus, for a wheatear population to exit the breeding season with the same number of individuals that entered it: n(pi2)x = 2n(1-pi) (recall that for RSH territories pi = 0.5). Solving this equation for x, produces one realistic result of x = 4, which is the number of fledglings/pair necessary for the RSH population not to decline during the breeding season. This exceeds the maximum fledging success of the species. Furthermore, even if wheatears could sustain such a high level of fledging, for the population to sustain itself between years would require an unrealistic assumption of 100% survival during the non-breeding period. Solving for x in the NH territories (pw weekly survival = 0.9665, and pi = 0.82) we find a value ~0.6 fledglings per breeding cycle. Thus, while the NH population has the potential to grow, the RSH population is undoubtedly a sink.
The smaller-sized RSH territories indicate higher habitat productivity or increased competition due to habitat attractiveness (Smith and Shugart, 1987), but could also be explained the presence weaker individuals displaced from the NH habitat and which cannot sustain a large territory. However, RSH territories were also reoccupied quicker than NH territories, and a much higher proportion of NH territories remained unoccupied once they were abandoned. Thus, the directional movement toward the RSH cannot be explained by despotic activity meaning that wheatears moved at will to what they perceived as superior habitat, and consequently fulfilling the necessary conditions for an ecological trap.
The type of anthropogenic change determines the short- and long-term impacts of the trap (Fletcher et al., 2012). In this study the roadside habitat acted as a severe ecological trap (as opposed to equal preference traps—Robertson et al., 2013) and is extremely effective because it offers, on the one hand, more attractive foraging grounds than the natural habitat, in combination with a novel yet deadly cue (sign posts for perching and nesting) and increased mortality rates due to other factors. The above results demonstrate the mechanics of the ecological trap we studied. Wheatears seek to improve their territory during the breeding season, and as RSH territories are vacated due to road-related mortality, individuals occupying NH territories will move to occupy these vacated lots.
Ecological Traps as a Selective Force
In addition to impacting the numerical and spatial dynamics of a population, ecological traps are potentially a selective force (Fletcher et al., 2012). To be a selective force, the response to the trap must vary between the individuals of the given population and this variance must have some genetic basis. Such intraspecific variation in the response to ecological traps has received little attention in empirical studies. The type of selection and its impact depends on the behavioral characteristics of the species. The establishment of territories in territorial species, as described herein, is a good example. If the genetic basis of territorial establishment is manifested only by individual habitat preferences, then the genotype preferring the trap will be selected against and the population will evolve to avoid the trap regardless of the type of competition (contest or scramble). By contrast, if the genetic basis of territorial establishment is manifested through contest competition, a paradoxical selection process will take place where the stronger competitors will have lower fitness countering that existing in the natural habitats.
Behavioral Rigidity, Nest Construction, and Roads
The ability of an organism to cope with human-induced rapid environmental change (HIREC) depends, in part, on the plasticity of the species behavior which, in turn, depends on the evolutionary history and the stability of the environment in which the species evolved (Sih et al., 2011). Because predation risk is inherently temporally variable, birds exhibit flexibility in nesting behavior in response to changes in the risk imposed by predators (Lima, 2009). Nest-building, on the other hand, is strongly linked to the physical environment which is not as dynamic as predation risk and nest construction strategies are characteristically evolutionarily conservative (Martin, 1993). Rigidity of behavioral responses can be a major threat to wild populations (Berger-Tal et al., 2011) when anthropogenic changes to the environment take place. Thus, HIREC can have a considerable impact on bird-fitness if mediated through nest construction behavior which is relatively inflexible. In the study reported here, a simple common, and apparently harmless, human structure—signposts—interact with the cavity nesting behavior of wheatears to produce an effective and potentially wide-spread ecological trap along roads.
Carcasses we collected from sign-posts show that other, less common, bird species also entrapped. Other road-related detrimental factors (e.g., car collisions and increased predation risk—Blouin-Demers and Weatherhead, 2001) are, also, not species specific. Thus, it is likely that roads are ecological traps for other insectivorous/predatory and/or cavity-nesting birds because of the abundance of resources and nesting sites along the roadside ditches. Furthermore, this impact may cascade and adversely affect the entire community structure and penetrate deep into the natural areas away from the road (see below).
Ecological traps are formed when novel elements that mimic attractive cues are added to an environment, or when natural cues normally used by individuals change in intensity, type, or number (Weldon and Haddad, 2005), or when habitat quality is reduced without significant change in the settlement cues (e.g., Lloyd and Martin, 2005). The underlying mechanism for all these scenarios is a mismatch between cues and habitat quality (Kokko and Sutherland, 2001; Schlaepfer et al., 2002; Kristan, 2003). In our study the trap was formed by a combination of several factors: (1) increased production along the road shoulders—an honest attractive cue, (2) sign posts—an attractive but deadly cue, (3) vehicle related mortality—a reduction in habitat quality not perceived by the birds.
Roads as Ecological Traps
Past studies have demonstrated that roadside abundance of wild populations may be higher, lower, or unchanged, relative to the abundance of the same species farther away from the road (Fahrig and Rytwinski, 2009). Typically, lower densities along roads are interpreted as avoidance (Benítez-López et al., 2010) while higher densities are interpreted as attraction. However, if based on snapshot estimates with no temporal replication at the correct scale and monitoring of individual movements, such data may be misleading if the road acts as an ecological trap. For example, high densities along the road may occur when the road acts as a severe ecological trap, so while the abundance reflects the attractiveness of the road it does not reveal the negative growth rate of the population alongside it. Similarly, if the road is an equal-preference trap and animals exhibit a quick spatial response using an ideal-free-distribution strategy, the roadside habitat and the habitat away from the road will have equal densities as individuals move quickly toward the road to fulfill vacant patches and equalize densities. If this is the case, densities in both habitats will gradually decline over time and, without temporal replication, will go un-noticed. If the road is an equal-preference trap but animals are slow in their spatial response, abundance along the road may appear lower and interpreted as avoidance while, in fact, the road is acting as a trap. Thus, snapshot data regarding population abundance along roads are insufficient to draw conclusions, highlighting the importance of gaining insight into the mechanisms underlying population-level responses for sound decision making and conservation protocols (Bro-Jørgensen et al., 2019).
Data Availability Statement
The raw data supporting the conclusions of this article will be made available by the authors, without undue reservation.
Ethics Statement
This animal study was carried out under a bird-handling permit held by Nimrod Ben-Aharon, issued by the Israel Nature and Parks Authority.
Author Contributions
NB-A carried out the field study. DK performed the statistical analysis. NB-A, DK, and DS wrote the manuscript. All authors contributed to the article and approved the submitted version.
Funding
This study was funded by a grant from the Israel Nature ad Parks Authority. Scholarship for NB-A was provided by the Albert Katz International School and the Mitrani Department of Desert Ecology, Ben Gurion University.
Conflict of Interest
The authors declare that the research was conducted in the absence of any commercial or financial relationships that could be construed as a potential conflict of interest.
Acknowledgments
We thank Dr. Dror Hawlena from the Hebrew University, Drs. S. Pilosof, O. Berger-Tal, and G. Rotem from Ben Gurion University, N. Weiss and Y. Perelman from the Society for the Protection of Nature, and O. Hatzofe from the Israel Nature and Parks Authority for advice, technical and field assistance along this research. Special thanks to the two reviewers for providing constructive criticism.
References
Abd El-Ghani, M. M. (1998). Environmental correlates of species distribution in arid desert ecosystems of Eastern Egypt. J. Arid Environ. 38, 297–313. doi: 10.1006/jare.1997.0323
Anderson, D. R. (2008). Model Based Inference in Life Sciences. New York, NY: Springer. 184. doi: 10.1007/978-0-387-74075-1
Arlt, D., and Pärt, T. (2007). Nondual breeding habitat selection: a mismatch between preference and fitness. Ecology 88, 792–801. doi: 10.1890/06-0574
Battin, J. (2004). When good animals love bad habitats: ecological traps and the conservation of animal populations. Conserv. Biol. 18, 1482–1491. doi: 10.1111/j.1523-1739.2004.00417.x
Benítez-López, A., Alkemade, R., and Verweij, P. A. (2010). The impacts of roads and other infrastructure on mammal and bird populations: a meta-analysis. Biol. Conserv. 143, 1307–1316. doi: 10.1016/j.biocon.2010.02.009
Berger-Tal, O., Peled, T., Oron, A., Kotler, B., Lubin, Y., and Saltz, D. (2011). Integrating animal behavior and conservation biology: a conceptual framework. Behav. Ecol. 22, 236–239. doi: 10.1093/beheco/arq224
Blouin-Demers, G., and Weatherhead, P. J. (2001). Habitat use by black rat snakes (elaphe obsolete obsoleta) in fragmented forests. Ecology 82, 2882–2896. doi: 10.1890/0012-9658(2001)082[2882:HUBBRS]2.0.CO;2
Brady, S. P., and Richardson, J. L. (2017). Road ecology: shifting gears toward evolutionary Perspectives 15, 91–98. doi: 10.1002/fee.1458
Bro-Jørgensen, J., Franks, D. W., and Meise, K. (2019). Linking behaviour to dynamics of populations and communities: application of novel approaches in behavioural ecology to conservation. Philos. Trans. R. Soc. Lond. B 374:20190008. doi: 10.1098/rstb.2019.0008
Brooks, M. L., and Lair, B. (2005). “Ecological effects of vehicular routes in a desert ecosystem,” in Report Prepared for the US Geological Survey, Recoverability and Vulnerability of Desert Ecosystems Program (Henderson, NV: Western Ecological Research Center).
Burnham, K. P., and Anderson, D. R. (1998). Model Selection and Inference: A Practical - Theoretical Approach. New York, NY: Springer-Verlag. doi: 10.1007/978-1-4757-2917-7
Coffin, A. W. (2007). From roadkill to road ecology: a review of the ecological effects of roads. J. Transport Geogr. 15, 396–406. doi: 10.1016/j.jtrangeo.2006.11.006
Delibes, M., Gaona, P, and Ferreras, P. (2001). Effects of an attractive sink leading into maladaptive habitat selection. Am. Nat. 158, 277–285. doi: 10.1086/321319
Doherty, P. F. J. R., and Grubb, T. C. J. R. (2002). Survivorship of permanent-resident birds in a fragmented forested landscape. Ecology 83, 844–857 doi: 10.1890/0012-9658(2002)083[0844:SOPRBI]2.0.CO;2
Donovan, T. M., and Thompson, F. R. (2001). Modeling the ecological trap hypothesis: a habitat and demographic analysis for migrant songbirds. Ecol. Appl. 11, 871–882. doi: 10.1890/1051-0761(2001)011[0871:MTETHA]2.0.CO;2
Egri, Á., Pereszlényi, Á., Farkas, A., Horváth, G., Penksza, K., and Kriska, G. (2017). How can asphalt roads extend the range of in situ polarized light pollution? A complex ecological trap of Ephemera danica and a possible remedy. J. Insect Behav. 30, 374–384. doi: 10.1007/s10905-017-9623-3
Fahrig, L., and Rytwinski, T. (2009). Effects of roads on animal abundance: an empirical review and synthesis. Ecol. Soc. 14:21. doi: 10.5751/ES-02815-140121
Fletcher, R. J. Jr., Orrock, J. L., and Robertson, B. A. (2012). How the type of anthropogenic change alters the consequences of ecological traps. Proc. R Soc. B 279, 2546–2552. doi: 10.1098/rspb.2012.0139
Forman, R. T. T. (1998). Road ecology: a solution for the giant embracing us. Landsc. Ecol. 13, 3–5. doi: 10.1023/A:1008036602639
Gates, J. E., and Gysel, L. W. (1978). Avian nest dispersion and fledging success in field-forest ecotones. Ecology 59, 871–888. doi: 10.2307/1938540
Gilroy, J. J., and Sutherland, W. J. (2007). Beyond ecological traps: perceptual errors and undervalued resources. Trends Ecol. Evol. 22, 351–356. doi: 10.1016/j.tree.2007.03.014
Gundersen, G. E, Johannesen, H. P., and Andreassen, Ims, R. A. (2001). Source-sink dynamics: how sinks affect demography of sources. Ecol. Lett. 4, 14–21. doi: 10.1046/j.1461-0248.2001.00182.x
Hawlena, D., Saltz, D., Abramsky, Z., and Bouskila, A. (2010). Ecological trap for desert lizards caused by anthropogenic changes in habitat structure that favor predator activity. Conserv. Biol. 24, 803–809. doi: 10.1111/j.1523-1739.2010.01477.x
Hixon, M. A. (1980). Food production and competitor density as the determinants of feeding territory size. Am. Natural. 115, 510–530. doi: 10.1086/283577
Holzaphel, C., and Schmidt, W. (1990). Roadside vegetation along transects in the Judean desert. Israel J. Bot. 39, 263–270.
Klauber, L. M. (1939). Studies of the reptile life in the arid Southwest. Part I. Night collecting on the desert with ecological statistics. Bull. Zool. Soc. San Diego, 14, 2–64. doi: 10.5962/bhl.title.130503
Kokko, H., and Sutherland, W. J. (2001). Ecological traps in changing environments: ecological and evolutionary consequences of a behaviourally mediated Allee effect. Evol. Ecol. Res. 3, 537–551.
Krasnov, B. R., and Shenbrot, G. I. (1996). Structure of darkling beetle communities and factors influencing their spatial distribution in the Negev highlands (Israel). Ecography 19, 139–152. doi: 10.1111/j.1600-0587.1996.tb00164.x
Kristan, W. B. (2003). The role of habitat selection behavior in population dynamics: source-sink systems and ecological traps. Oikos 103, 457–468. doi: 10.1034/j.1600-0706.2003.12192.x
Lima, S. L. (2009). Predators and the breeding bird: behavioral and reproductive flexibility under the risk of predation. Biol. Rev. 84, 485–513. doi: 10.1111/j.1469-185X.2009.00085.x
Lloyd, J. D., and Martin, T. E. (2005). Reproductive success of chestnut-collared longspurs in native and exotic grassland. Condor 107, 363–374. doi: 10.1093/condor/107.2.363
Martin, T. E. (1993). Nest predation and nest sites: new perspectives on old patterns. Bioscience 43, 523–532. doi: 10.2307/1311947
Norris, D. R., Tyler Flockhart, D. T., and Strickland, D. (2013). Contrasting patterns of survival and dispersal in multiple habitats reveal an ecological trap in a food-caching bird. Oecologia 173, 827–835. doi: 10.1007/s00442-013-2680-1
Robertson, B. A. (2012). Investigating targets of avian habitat management to eliminate an ecological trap. Avian Conserv. Ecol. 7:2. doi: 10.5751/ACE-00533-070202
Robertson, B. A., and Hutto, R. L. (2006). A framework for understanding ecological traps and an evaluation of existing evidence. Ecology 87, 1075–1085. doi: 10.1890/0012-9658(2006)87[1075:AFFUET]2.0.CO;2
Robertson, B. A., Rehage, J. S., and Sih, A. (2013). Ecological novelty and the emergence of evolutionary traps. Trends Ecol. Evol. 28, 552–560. doi: 10.1016/j.tree.2013.04.004
Rosen, P. C., and Lowe, C. H. (1994). Highway mortality of snakes in the Sonora desert of southern Arizona. Biol. Conserv. 68, 143–148. doi: 10.1016/0006-3207(94)90345-X
Schlaepfer, M. A., Runge, M. C., and Sherman, P. W. (2002). Ecological and evolutionary traps. Trends Ecol. Evol. 17, 474–480. doi: 10.1016/S0169-5347(02)02580-6
Shirihai, H. (1996). The Birds of Israel, eds E. Dovrat and D. A. Christie. San Diego, CA: Academic Press INC. 452–453.
Sih, A., Ferrari, M. C. O., and Harris, D. J. (2011). Evolution and behavioural responses to human-induced rapid environmental change. Evol. Appl. 4, 367–338. doi: 10.1111/j.1752-4571.2010.00166.x
Smith, T. M., and Shugart, H. H. (1987). Territory size variation in the ovenbird: the role of habitat structure. Ecology 68, 695–704. doi: 10.2307/1938475
Weldon, A. J., and Haddad, N. M. (2005). The effects of patch shape on indigo buntings: Evidence for an ecological trap. Ecology 86, 1422–1431. doi: 10.1890/04-0913
Keywords: Oenanthe lugens, mourning wheatear, territorial shift, survival, sink
Citation: Ben-Aharon N, Kapota D and Saltz D (2020) Roads and Road-Posts as an Ecological Trap for Cavity Nesting Desert Birds. Front. Conserv. Sci. 1:614899. doi: 10.3389/fcosc.2020.614899
Received: 07 October 2020; Accepted: 25 November 2020;
Published: 22 December 2020.
Edited by:
Shermin De Silva, Trunks & Leaves Inc., United StatesReviewed by:
Artur Andriolo, Juiz de Fora Federal University, BrazilMichael Sievers, Griffith University, Australia
Copyright © 2020 Ben-Aharon, Kapota and Saltz. This is an open-access article distributed under the terms of the Creative Commons Attribution License (CC BY). The use, distribution or reproduction in other forums is permitted, provided the original author(s) and the copyright owner(s) are credited and that the original publication in this journal is cited, in accordance with accepted academic practice. No use, distribution or reproduction is permitted which does not comply with these terms.
*Correspondence: David Saltz, ZHNhbHR6QGJndS5hYy5pbA==