- 1Central Department of Botany, Tribhuvan University, Kathmandu, Nepal
- 2Department of Plant Biotechnology, Annapurna Research Centre, Kathmandu, Nepal
Introduction: Dendrobium species have been widely used for many health disorders since ancient times. However, due to unrelenting collection to meet the increasing demand for their use in medication and other health products, the natural habitats of medicinal Dendrobium transparens have been devastated and are on the verge of extinction.
Methods: An efficient in-vitro propagation protocol for Dendrobium transparens using seed derived protocorms was established and genetic homogeneity of the in-vitro regenerants and the wild plant was studied.
Results: The maximum seed germination was observed in Full strength Murashige and Skoog medium (FMS). Induction of protocorms were achieved on basal as well as half-strength MS medium. The highest number of shoot (11.9 shoots/explant) was achieved in half MS medium fortified with 100 mL/L coconut water in addition with Benzyl amino purine (BAP) 1 mg/L and Kinetin 2 mg/L. Further, elongated shoots were transferred to full and half strength MS root initiating medium supplemented with different concentration of auxins. However, a maximum of (8.3 ± 0.6, 4.9 ± 0.1 cm) roots were achieved in full MS medium fortified with 100 mL/L coconut water and Napthalene acetic acid (NAA) 1.5 mg/L. Ten rapid Random Amplified Polymorphic DNA (RAPD) and Inter Simple Sequence Repeats (ISSR) primers were used to analyze genetic stability among in-vitro and mother plant. RAPD primers produced a total of 23 fragments while ISSR primers produced a total of 16 fragments.
Conclusion: The amplified bands of all the samples of in-vitro plants were similar to bands of mother plant. The present research reported here is indicating the applicability of tissue culture for true-to-type plant production and conservation of D. transperens.
Introduction
Dendrobium is one of the largest genera in Orchidaceae represented by more than 1,100 species in the world and having 30 species in Nepal (Rajbhandari, 2015). These are most prized orchid species for their beautiful long-lasting flowers exhibiting an incredible range of diversity in size, shape and color. Beside ornamental value, Dendrobium species are widely used for many health disorders from ancient period of time. The stems of Dendrobium species have been widely used in traditional Chinese medicine (TCM) for improving digestion, promoting and production of body fluids, nourishing, clearing heat, and as tonic (Xu et al., 2013). Dendrobium species are sources of large amount of polysaccharides, phenanthrenes, revesteral, polyphenol compounds acting as potent source of antioxidant and cytotoxic effects (Gutiérrez, 2010; Pant, 2013).
D. transparens (Figure 1) is mildly fragment epiphytic sympodial orchid distributing along geographical ranges from Nepal, Bangladesh, eastern Himalayas, India, Bhutan, Myanmar, and Vietnam. These are found in dense forest at elevations of 500 to 2100 meters above sea level. It ranks among the important ornamental orchids with graceful pendulous racemes of medium sized flowers, usually pristine white except purple blemish at tip of the sepals, petals and throat of labellum (efloras, 2020). D. transparens based herbal formulations used for geriatric diseases & disorders in Indian traditional healing system. As a result, it has been collected illegally from wild Due to unremitting collection to meet the increasing demand for their use in medication and other health products, the natural habitats of medicinal D. transparens has been devastated and are under danger of extinction. Rate of multiplication of Dendrobium species in nature is extremely very slow with 2-4 shoots per year as well as rate of seed germination of orchids is very complex as these require specific fungal partner due to lack of endosperm and nutritive materials (Otero et al., 2002; Pant et al., 2017). Thus, micro propagation by tissue culture is particularly useful to conserve their germplasm and for medicinal utilization (Pant et al., 2018). Past couple of decades have magnified the role of in-vitro techniques in orchid conservation efforts, it would be one of the most suitable alternative tools to minimize the pressure on natural population of medicinal orchids (Pfab & Scholes, 2004; Pant, 2014) and thus help in their sustainable utilization and conservation. Furthermore, in-vitro techniques are highly useful for providing sustainable sources of optimal plant-derived natural products (Bourgaud et al., 2001; Vanisree et al., 2004; Hussain et al., 2012; Pradhan et al., 2014; Ochoa-Villarreal et al., 2016; Espinosa-Leal et al., 2018; Regmi et al., 2017). Orchid species have been cultured with various techniques to synthesize bioactive molecules in-vitro (Bourgaud et al., 2001; Vanisree et al., 2004; Giri et al., 2012b; Hussain et al., 2012).
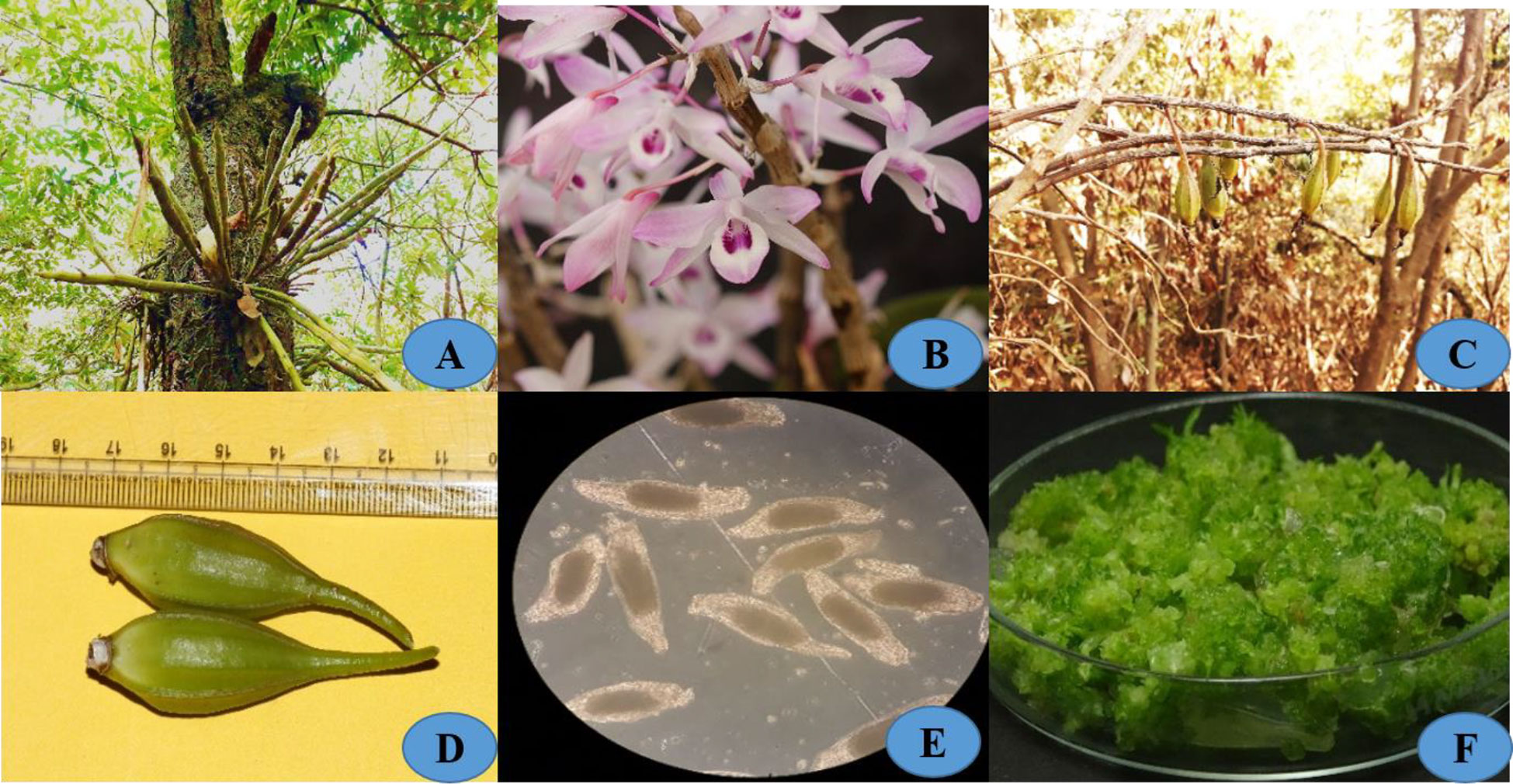
Figure 1 (A) Habitat; (B) Close up flower; (C) Seed pods in nature; (D) Seed pods of length 4-5 cm long; (E) seeds of (D) transparens; (F) Protocorms developed earlier most of 4 weeks in FMS medium.
Several medicinal Dendrobium species has been in-vitro propagated through asymbiotic seed germination or direct shoot regeneration or protocorm formation from different explants including D. candidum (Shiau et al., 2005), D. chrysotoxum (Xu et al., 2001), D. fimbriatum (Roy and Banerjee, 2003; Sharma et al., 2005), D. nobile (Nayak et al., 2002; Malabadi et al., 2005), D. tosaense (Lo et al., 2004), D. chrysanthum (Rao & Barman, 2014), D. longicornu (Dohling et al., 2012), (Teixeira da Silva et al., 2015b). So that these important species can be conserved and sustainably utilized for different medicinal use (Teixeira da Silva et al., 2014). Somaclonal variations are often observed within the in-vitro raised plants. To ascertain the genetic similarity between the in-vitro grown plantlets and the mother plant, molecular markers are often used to study the variation within the germplasm (Kumar et al., 2014). Traditionally, various conventional molecular markers like random amplified polymorphic DNA (RAPD), inter simple sequence repeats (ISSR), and simple sequence repeats (SSR) are used extensively in the assessment of clonal fidelity in a wide range of plants species including orchids (Devi et al., 2013; Bhattacharyya et al., 2014; Devi et al., 2014; Bhattacharyya et al., 2015; Pandey et al., 2020; Oliya et al., 2021). Moreover, use of more than one molecular marker to evaluate clonal fidelity of tissue culture-raised plants is significant (Palombi and Damiano, 2002).
Because of this, in the current study, RAPD and ISSR were used to assess the clonal fidelity of the regenerates. The former method uses a single primer to detect nucleotide sequence polymorphism while the latter allows the detection of polymorphism in inter-microsatellite loci using a primer made from dinucleotide or trinucleotide simple sequence repeats (Parab and Krishnan, 2008). The wild population of the medicinal and ornamentally significant orchid D. transparens is being exploited as a result of random collection. Because micropropagation of D. transparens can help scale up several fold through in-vitro propagation, the present investigation was carried out on D. transparens to examine the effects of various plant growth regulators on seed germination, protocorm induction, and shoot development.
Material and methods
Plant material and surface sterilization
Immature capsules of D. transparens were collected during June and July of 2018 from Bhaktapur district (27.6451°N, 85.4427°E) of central Nepal. They were used as explant to produce protocorms in vitro. A voucher specimen of this plant was deposited in the Tribhuvan University Central Herbarium (TUCH) (voucher number P08), Kathmandu, Nepal. The capsules were surface sterilized with 1-2 drops of TWEEN-20 and washed under running tap water for at least half an hour. The capsules were then dipped in 1% sodium hypochlorite solution for 15 minutes. After that they were submerged in 70% ethanol with the help of long forceps and were rapidly set a flame for a few seconds. The capsules were rinsed in sterile water intermittently. The explants were then blot dried using a Whatman filter paper placed onto sterile petri plates and inoculated in test tubes containing Murashige and Skoog (MS) (Murashige and Skoog, 1962) medium containing 3% sucrose (w/v), 0.1% myoinositol supplemented with coconut water and plant growth hormones The pH of the medium was adjusted to 5.8 with 1N NaOH or 1N HCL and solidified with 0.8% plant tissue culture tested agar-agar (w/v) (Himedia, India), prior to autoclaving (121°C, 20 min), The cultures were maintained at 25°C ± 2°C and 1000 flux lux under 16/8 h photoperiod with 75% relative humidity (RH). These surface sterilized capsules were then cut longitudinally with a sterile scalpel and the exposed seeds were transferred to an agar gel (8gm/L) nutrient medium for seed germination.
Inoculation in culture medium and shoot proliferation, elongation and root formation
Immature seeds were placed on MS solid medium supplemented with 6-benzylaminopurine (BAP) 0.5–2.0 mg/L, in combination with α-naphthalene acetic acid (NAA) 0.5–1.0 mg/L for the formation of protocorms. The Protocorms were transferred to the basal MS medium for proliferation. After 2 months of maturation, protocorms were cultured on growth regulator-free MS medium as control and the media containing NAA, BAP or Kin with a concentration range from 0.5 to 2.0 mg/L in various combinations for shoot development. Healthy shoots with 2–3 leaves were sub cultured on the basal MS medium for further development. For root initiation, the basal MS medium was supplemented with NAA, indole-3-acetic acid (IAA), indole-3-butyric acid (IBA) each at 1–1.5 mg/L and coconut water (100 mL/L) in different combination. The frequency and number of shoots formed were evaluated after 4 weeks of inoculation. Morphological changes were recorded on the basis of visual observations.
Genetic stability study of in-vitro developed plants compared with mother plants
Selection of in-vitro samples for DNA isolation
For the genetic profiling, leaves of in vivo mother plants were used. The rooted plants and protocorms of in-vitro materials were selected based on medium that provided the optimal growth responses. Leaves of four in-vitro plant samples grown on FMS medium containing 2 mg/L BAP+1 mg/LNAA, 10% CW and protocorm developed on HMS medium were used for the genetic fidelity studies. The genetic profiling experiment was performed in Annapurna Research Center, Maitighar, Kathmandu, Nepal.
Extraction of DNA by CTAB method
Genomic DNA from mother plants and in-vitro grown plant samples were extracted using the CTAB (hexadecyltrimethyl ammonium bromide) method of Doyle (1991) with some modifications. Briefly, 0.2 g of in vivo (leaf) and in-vitro (leaf and protocorm) samples were taken and grinded to a fine powder in liquid nitrogen using mortar and pestle. To this, 1 mL of CTAB buffer (2% CTAB, 0.5 M EDTA, 5 M NaCl, 1 M Tris HCl, Ph 8, 0.2% β-mercaptoethanol) was added and mixed to a fine paste. The paste was transferred into a clean sterilized micro-centrifuge tube (vol. 1.5 mL) and incubated in water bath at 65°C for 30 min. with a gentle inversion every 10 min. After incubation samples were allowed to cool for 3− 5 min. and then centrifuged at 12,000 rpm for 8 min. to spin down cell debris. The resultant supernatant was transferred to a clean sterilized microcentrifuge tube and an equal volume of chloroform: isoamyl alcohol in the ratio of 24: 1 was added and mixed gently by inversion for 5− 8 min. It was again centrifuged at 13,000 rpm for 5 min. and the upper aqueous phase was transferred to sterilized microcentrifuge tube. Then 1/10th volume of ammonium acetate (7.5 M) was added to each sample followed by the addition of 500 μL chilled ice-cold absolute ethanol. The tubes were slowly inverted several times (3− 5 min.) to precipitate the DNA. After that, the DNA was centrifuged at 13, 000 rpm for 2 min. to form the pellet. The supernatant was discarded and the pellet was washed with ice-cold 70% ethanol (Vol. 500 μL). Again, it was centrifuged at 13,000 rpm for 1 min. to get rid of salt. The remaining ethanol was then pipetted out and the pellet was allowed to dry inside a Laminar Flow Cabinet for 30 min. Finally, the extracted DNA was diluted in 1X TE buffer and the quality of the extracted DNA was evaluated using 1% agarose gel (w/v) electrophoresis. The DNA was stained by ethidium bromide for 45 min. with a continuous supply of 70 voltage power. After that, it was visualized by UV gel documentation system (UVITEC Cambridge). The quantity of the DNA was evaluated using a fluorimeter (Thermo Fisher scientific, USA). The concentration of extracted DNA was adjusted to 30 ng and stored at − 20 °C.
DNA amplification and RAPD, ISSR analysis
A total of ten RAPD and ISSR markers were screened and markers with clear and reproducible bands were considered for the analysis. The PCR amplification was performed on a final volume of 15 μL containing 30 ng of genomic DNA, 6.5 μL of Dream Taq PCR master mix (Thermo Fisher Scientific, USA) and 1 μM each primer. The cycling condition was carried out in a Pro Flex PCR (Thermo Fisher Scientific, USA) programmed at 94 °C for 5 min. followed by 40 cycles of denaturing at 94 °C for 45 s, annealing at 40-45°C for 1min for RAPD and 50-58°C for 1 min for ISSR, extension at 72 °C for 30 s, and then a final extension at 72 °C for 5 min. To test the utility of the primers, PCR products were detected on 1% agarose gels with a constant voltage of 70 V for 1.5 h. The size of the amplification product was determined by comparison with the 100 bp DNA ladder marker (Thermo Fisher Scientific, USA). The clear and reproducible bands were considered for counting alleles.
Experimental design and statistical analysis
Experiment was carried out in a randomized design and replicated with repetition at least three times. Visual observations were made every week. Explants forming protocorms and number of protocorms per explant were recorded after 4 weeks of protocorms induction. Protocorms number developing into shoots was counted after 4 weeks of shoot induction. The percentage of shoots producing roots and number of roots per plantlet were recorded after 25 days of root initiation. Data were statistically analyzed for significance using one way analysis of variance (ANOVA) and the differences were contrasted via Duncan’s test at P ≤ 0.05 using the SPSS version 20
Result
Seed germination and protocorms induction
The seeds of D. transparens were cultured in-vitro on FMS alone and full MS supplemented with BAP (0.5 to 2.0) mg/L and NAA (1 mg/L) (Table 1). The quick response on seed germination of D. transparens was observed on hormone free FMS medium that took 4 weeks of culture (Table 1; Figure 1). Protocorms were initiated earlier (4 weeks of seed culture) with longest span life on FMS medium. Thus, protocorms developed in full MS medium were used for phytochemical extraction. The full MS medium supplemented with BAP 2 mg/L and NAA 1 mg/L initiated protocorms after 6 weeks of seed culture however, the primary shoot and root were premature within 8 weeks and 11 weeks of culture respectively (Table 1; Figure 2).
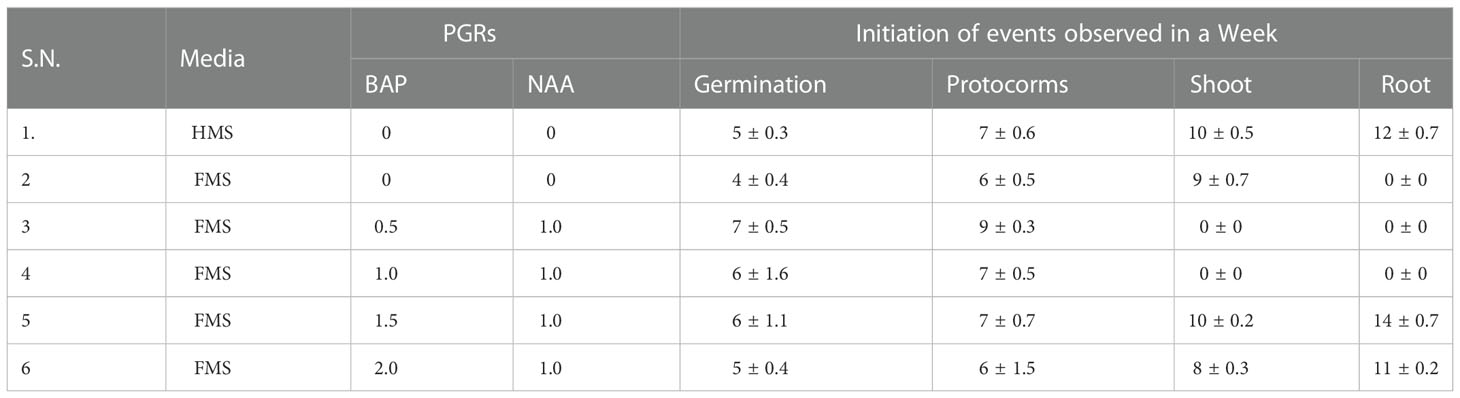
Table 1 Effect of different strength of MS medium with/out BAP and NAA for in-vitro culture of D. transparens.
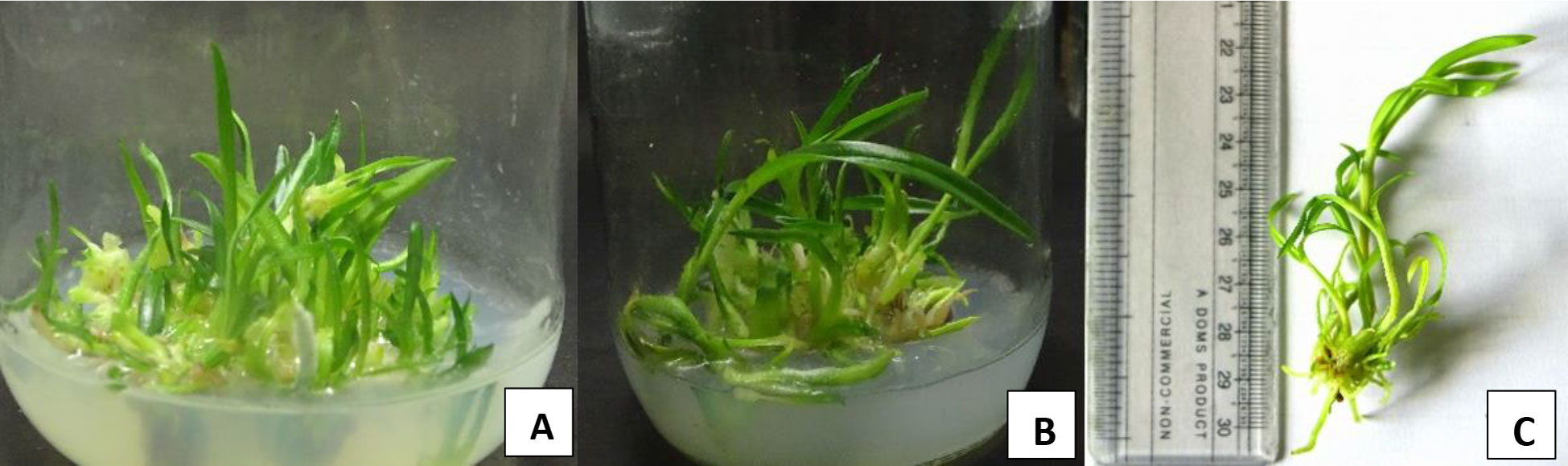
Figure 2 (A) From left: shoot development on HMS+10%CW +BAP (1mg/L) + KIN(2mg/L); (B) Root development on FMS+10% CW+NAA (1.5 mg/L); (C) Plantlet developed in HMS+ 7NAA.
This condition was followed by full MS supplemented with BAP 1.5 mg/L and NAA 1 mg/L which took 10 weeks of culture for shoot initiation and 14 weeks of culture for root initiation. It was found that the lower concentration of BAP in addition with NAA were not effective for the development of shoot and root until 16 weeks of culture.
Shoot multiplication using protocorms
For the shoot multiplication, MS medium with full (FMS) and half strength (HMS) MS medium supplemented with or without hormone and coconut water were used. During study, the maximum shoot multiplication of D. transparens was observed on half MS medium fortified with 100 mL/L coconut water in addition with BAP 1 mg/L and Kinetin 2 mg/L with highest number of shoot (9.0 shoots/plant) with shoot length (4.5 ± 0.1 cm) (Figure 2).
In-vitro root initiation
The maximum numbers and length of root was observed on full MS medium fortified with 100 mL/L coconut water and NAA 1.5 mg/L (8.3 ± 0.6 no, 4.9 ± 0.1 cm) (Table 2; Figure 2). In the present study, full strength of MS medium was found very effective for proper germination. For better growth, germinating seedlings were transferred to half strength medium supplemented with 100 mL/L coconut water after formation of protocorms after 6 weeks of germination. The efficacy of multiple shoot formation differed with concentrations and combinations of BAP, Kinetin, and NAA and coconut water. Multiple shoots were derived from the protocorms like bodies. Explants cultured on half-strength MS medium supplemented with BAP 1 mg/L, Kinetin 2 mg/L with 100 mL/L coconut water developed multiple shoots (Figure 2). Well-developed roots were induced in all the shoots derived from protocorms with different auxins within 15 d of culture. In control treatment, where full and half strength of MS medium was not supplemented with any auxin, the rooting of plants developed from shoots delayed and roots appeared after 25 to 30 days of culture. Rooting efficiency under such condition when analyzed among the auxin treatments, IBA with 100 mL/L coconut water deliberated the highest number of roots developed (10 roots/plant), differing significantly from other treatments. However, development of maximum mean root length (4 cm) was favored by full MS medium supplemented with NAA 1.5 mg/L.
However, best response of rooting in the present study was found on full strength MS medium supplemented with NAA (1.5 mg/L) and coconut water, resulting in higher number of early rooting than when cultured on an auxin free medium. The auxin, NAA exerted better response on longer root length. The well shooted and rooted plantlet were transferred in various concentration of NAA before acclimatization from 3mg/l to 10mg/l where we found that high concentration of NAA (7mg/l) was best for plantlet development before acclimatization. During the study it was observed that that FMS supplemented with 1.5 mg/L NAA was found best for the robust root and shoot elongation in case of D. transparens (Table 3).
Genetic fidelity analysis of in-vitro plantlets
Somaclonal variations causes genetic defects which can limit the use of the in-vitro propagation system (Salvi et al., 2001). However, for large-scale propagation, efficiency of propagation methods along with genetic stability of the regenerated plants is of paramount importance (Haisel et al., 2001). In the present study, out of the 10 RAPD primers tested only eight primers (OPA 10, OPA 03, OPA 06, OPA 07, OPC 15, OPC19, OPC 20, OPA 01) gave similar and reproducible banding patterns between the in-vitro raised plantlets and protocorm and the mother plant (Table 4; Figure 3). A total of 18 bands were scored from in-vitro plants of D. transparens. The number of scorable bands varied from 2 to 5 within the approximate size range of 200-1300 bp while compared to ladder marker (1000bp sized). Similarly, five ISSR markers (UBC 880, UBC 834, UBC 826, UBC 844, UBC 807) showed clear and reproducible band. A total of 17 band were scored from in-vitro and mother plants of D. transparens which varied from two to three within the approximate size range of 500-700 bp while compared to ladder marker (1000bp sized) (Table 5; Figure 4).
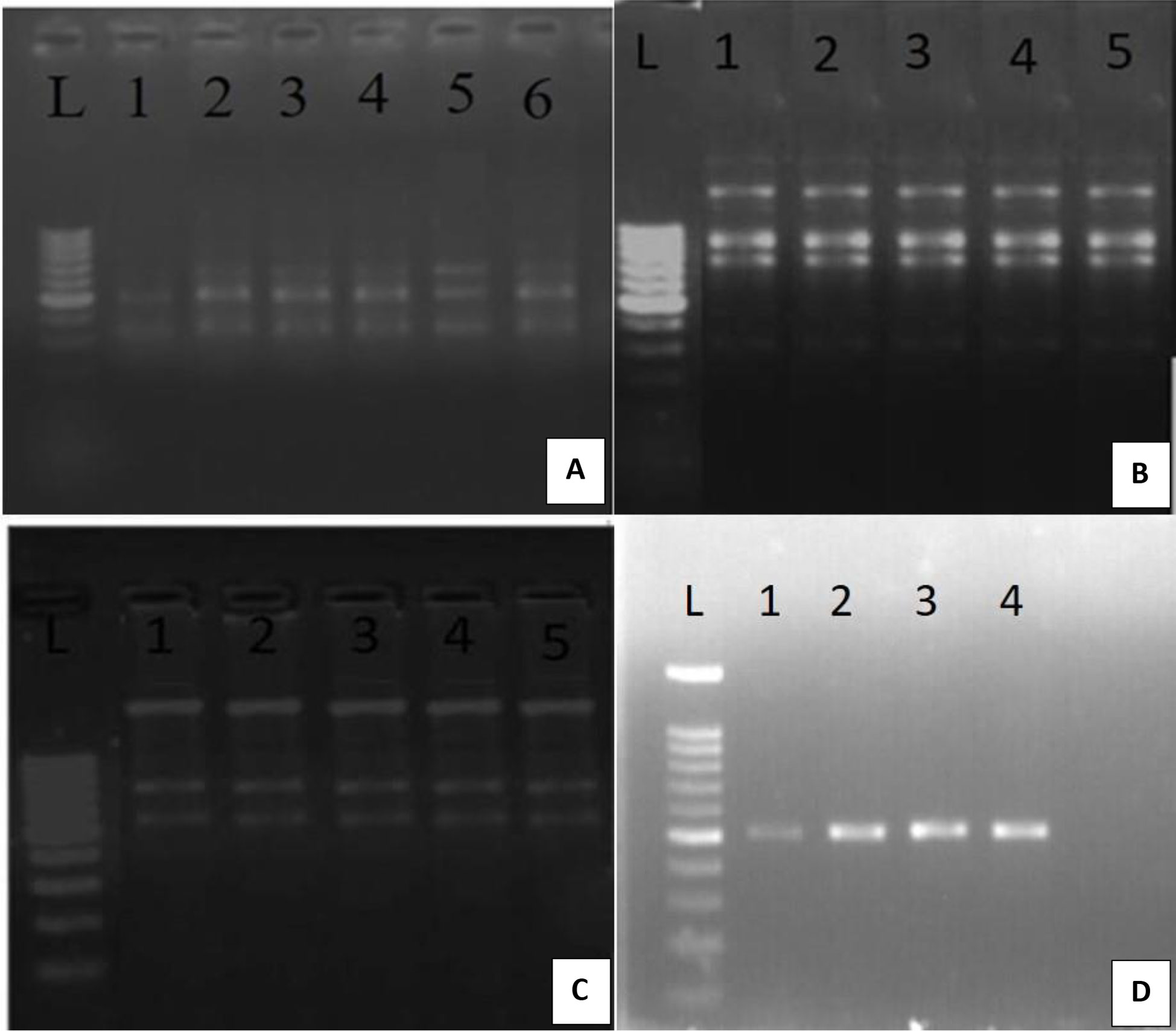
Figure 3 Genetic fidelity analysis of in-vitro and mother plants of D transparens using RAPD marker (A) OPA 10 Primer L- Lader, 1 to 5 in-vitro plants; 6 mother plant; (B) OPA03 Primer L- Lader, 1 to 4 in-vitro plants; 5 mother plant. (C) OPA06 Primer L- Lader, 1 to 4 in-vitro plants, 5 mother plant, (D) OPA07 Primer L- Lader, 1 to 3 in-vitro plants 4 mother plant; (Size of ladder- 1000 bp).
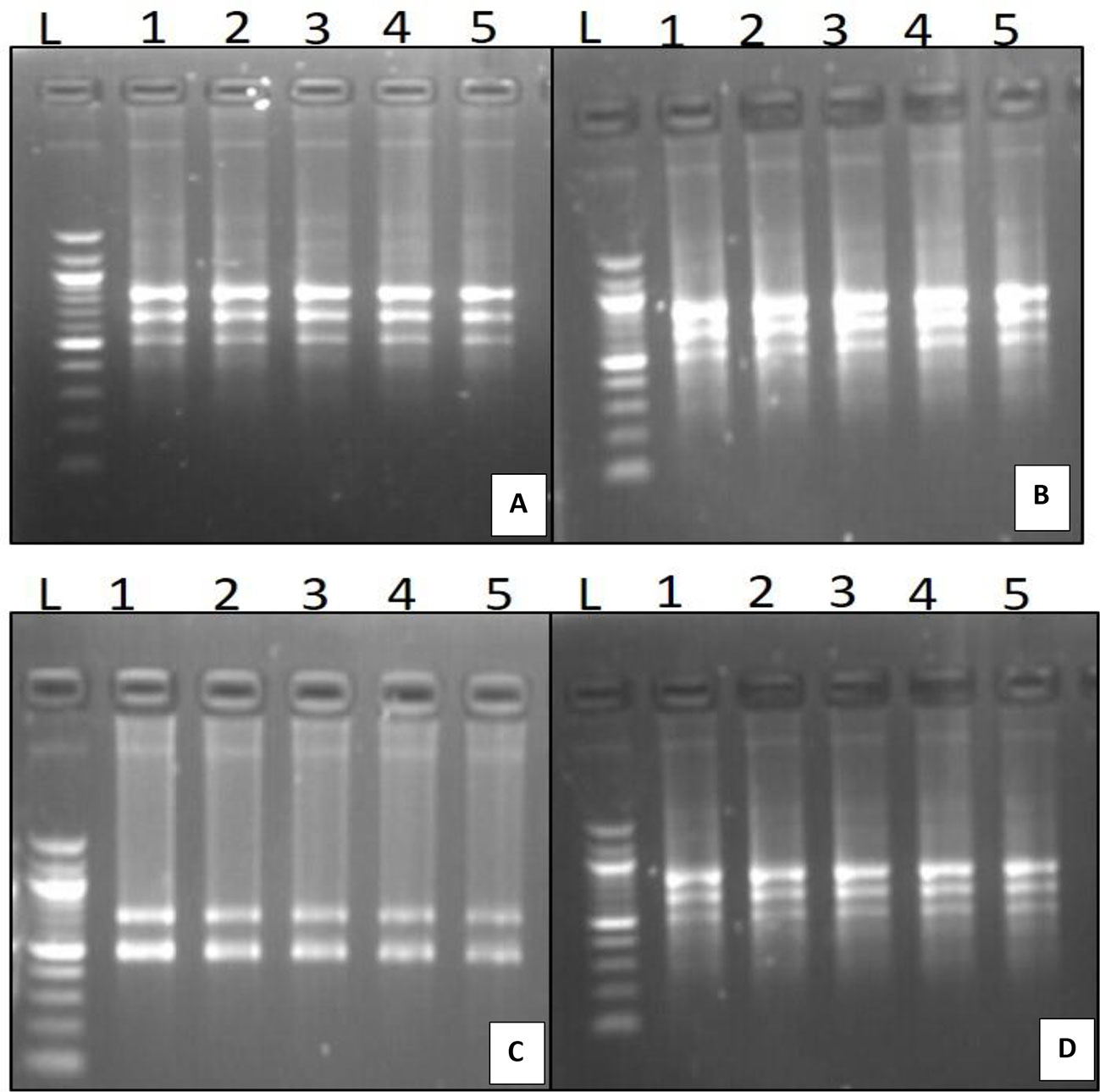
Figure 4 Genetic fidelity analysis of in-vitro and mother of D.transparens using ISSR marker (A) UBC 826 Primer L- Lader, 1 to 4 in-vitro plants; 5 mother plant; (B) UBC 834 Primer L- Lader, 1 to 4 in-vitro plants; 5 mother plant. (C) UBC 844 Primer L- Lader, 1 to 4 in-vitro plants, 5 mother plant, (D) UBC 880 Primer L- Lader, 1 to 4 in-vitro plants 4, 5mother plant; (Size of ladder- 1000 bp).
Discussion
Since mycorrhizal fungus association is necessary for orchid seed germination in nature, ex situ conservation of threatened medicinal orchid species is crucial. The most popular method for multiplying various orchid species is the in-vitro seed germination technique, which has been developed for a number of species. The most trustworthy method for preserving and using plant materials sustainably is the production of true-to-type plants using plant tissue culture (Teixeira da Silva et al., 2015a; Teixeira da Silva et al., 2015b). In the current study, the micropropagation procedure for D. transparens, a threatened orchid species, was standardized using protocorm culture. FMS showed the best seed germination, and the same holds true for protocorm development. D. transparens protocorms were transplanted into medium that also contained plant growth regulators like BAP, KIN, NAA by itself or fortified with 10% C.W and the shooting and rooting of the plants were also evaluated (Table 1). The development of D. transparens protocorm and plantlets was successful under every test condition. Recent study on the asymbiotic germination of the immature embryos of D. transparens on full-strength MS medium, it was found that the maximum shoot multiplication of D. transparens occurred on this medium when it was fortified with 100 mL/L of coconut water along with 1 mg/L of BAP, and 2 mg/L of kinetin (Alam et al., 2002; Sunitibala et al., 2009). Study on D. transparens, which was similar, it was observed that BAP in conjunction with NAA induced best shoots. Contrary to our study, the use of NAA in the initial condition was not found to be active in the proliferation of the shoots, but the use of HMS along with NAA and CW was found to be significant to be helpful in elongation of the shoots. Similar findings were seen in D. densiflorum, where shoot development was improved by FMS fortified with coconut (Pant et al., 2022). According to other studies, a few Dendrobium orchids needed nutrient media with organic additives to induce multiple shoots. To encourage multiple shoots in D. antennathum, peptone was added to the medium (Kukulczanka and Wojciechowska, 1982), whereas yeast extract or urea was used for D. chrysanthum (Vij and Pathak, 1989). The most effective method for inducing multiple shoot and root micropropagation of D. transparens, according to the most recent results, was CW. The present study was supported by the observation that HMS and CW were used in the micropropagation of D. chryseum for multiple shoot regeneration. (Maharjan et al., 2020; Teixeira da Silva et al., 2015a). Similar findings showed that FMS supplemented with 1.5 mg/L NAA was best for D. transparens robust root and shoot elongation (Figure 2; Table 3). These findings were further proved by (Aktar et al., 2007), who discovered that Dendrobium sp. roots with the longest lengths were grown on MS medium supplemented with 1 mg/L IBA. The positive effect of auxins on root initials as seen by these responses was explained by (De Klerk et al., 1997). Shiau et al. (2005) reported that half-strength MS medium supplemented with 0.2 mg/L NAA was effective for the development of roots on D. candidum, supporting the current finding. The shoots of Dendrobium hybrids showed rooting on Went medium (VW) medium supplemented with 2 mg/L IBA and IAA in the earlier study (Dodds, 1991). Geodorum densiflorum shoots were established in-vitro on MS medium containing 1.0 M NAA (Sheelavantmath et al., 2000). According to a review of dendrobium micropropagation by (Teixeira da Silva et al., 2015b), BAP and NAA, either together or separately in the culture medium, are the most frequently used plant growth regulators (PGRs) in Dendrobium micropropagation. According to (Li et al., 2013), different species have different standardized media for inducing shoots and roots. For D. pendulum and D. primulinum, the appropriate media for shoot induction were HMS 0.5 mg/l BA + 0.1 mg/l NAA + 100 ml/l coconut water (CW), and for D. heterocarpum, HMS 0.25 mg/l BA + 0.1 mg/l NAA + 100 ml/l CW.
Compared to Ladder marker, there are 1 to 6 scorable bands that are roughly 1000 bp in size (100bp sized). More polymorphisms are a sign of more genetic diversity. However, the current study found that D. transperens had more genetic similarity than polymorphism (Figures 3, 4). The in-vitro cultured plants displayed 100% monomorphism between and within them, according to RAPD and ISSR markers. The current finding is corroborated by (Giri et al., 2012a), who discovered no differences in ISSR profiles between plantlets of Habenaria edgeworthii (Terrestrial orchids) that were in-vitro regenerated and derived from 6-benzyladenine (BAP) and a-naphthalene acetic acid (NAA) treatments. Inter-retrotransposon amplified polymorphism (IRAP) and start codon targeted (SCoT) profiles among the in-vitro regenerated plantlets of Ansellia africana (Leopard orchid) derived through TDZ and NAA treatments revealed a high level of genetic homogeneity (Bhattacharyya et al., 2018). Similar to this, (Roy et al., 2012) discovered a 5.81% variation in RAPD profiles among Cymbidium giganteum in-vitro regenerants produced as a result of thidiazuron (TDZ) treatment. Direct somatic embryo regenerated plants (94.22%) have higher genetic homogeneity than indirect organogenesis, according to (Sherif et al., 2018). In our study no genetic variation was observed between the in-vitro and wild D. transperens when analyzed using RAPD and ISSR markers.
Conclusion
The technique of in- vitro propagation can be effectively used to propagate medicinally important and commercially valuable plant species. In this study a highly reproducible, rapid and efficient protocol was established for high-frequency immature seed germination of D. transparens. The genetic fidelity analysis using two different markers to assess the similarity with the mother plant was carried out and no variations were detected in both tissue culture derived plantlets and the subsequently mother plant of D. transparens. When compared to their mother plant, the in-vitro regenerated plants displayed a high degree of genetic stability. The current technology aims to be a promising one for conservation and sustainable utilization of germplasm and producing D. transparens plantlets that are true to type in large scale. This technology can be economical and highly benefitted for long term in- vitro conservation of other orchids as orchid germination in nature is very difficult. To our knowledge, this is the first report on D. transparens in- vitro propagation and also assessment of genetic fidelity in the in-vitro generated plants of orchids.
Data availability statement
The original contributions presented in the study are included in the article/Supplementary material. Further inquiries can be directed to the corresponding author.
Author contributions
PJ designed, executed the experiments and prepared the manuscript. SP helped in the experimental analysis and assisted in manuscript preparation. LM helped in the experimental analysis. BP overall supervised the entire research work and critically evaluated the manuscript. All authors contributed to the article and approved the submitted version.
Funding
Author PJ acknowledges University Grant Commission, Nepal, for PhD Research Support Grants-2020 (Grant No. PhD-76-77-S&T-20) for fellowship support.
Conflict of interest
The authors declare that the research was conducted in the absence of any commercial or financial relationships that could be construed as a potential conflict of interest.
Publisher’s note
All claims expressed in this article are solely those of the authors and do not necessarily represent those of their affiliated organizations, or those of the publisher, the editors and the reviewers. Any product that may be evaluated in this article, or claim that may be made by its manufacturer, is not guaranteed or endorsed by the publisher.
Supplementary material
The Supplementary Material for this article can be found online at: https://www.frontiersin.org/articles/10.3389/fcosc.2022.1083933/full#supplementary-material
References
Aktar S., Nasiruddin K. M., Huq H. (2007). In-vitro root formation in Dendrobium orchid plantlets with IBA. J. Agric. Rural Dev., 5(1), 48.
Alam M. K., Rashid M. H., Hossain M. S., Salam M. A., Rouf M. A. (2002). In-vitro seed propagation of Dendrobium (Dendrobium transparens) orchid as influenced by different media. Int. J. Biotechnol. 1, 111–115. doi: 10.3923/biotech.2002.111.115
Bhattacharyya P., Kumaria S., Diengdoh R., Tandon P. (2014). Genetic stability and phytochemical analysis of the in-vitro regenerated plants of dendrobium nobile lindl., an endangered medicinal orchid. Meta. Gene 2, 489–504. doi: 10.1016/j.mgene.2014.06.003
Bhattacharyya P., Kumaria S., Job N., Tandon P. (2015). Phyto-molecular profiling and assessment of antioxidant activity within micropropagated plants of Dendrobium thyrsiflorum: a threatened, medicinal orchid. Plant Cell, Tissue and Organ Culture (PCTOC) 122(3), 535–550.
Bhattacharyya P., Kumar V., Van Staden J. (2018). In-vitro encapsulation based short term storage and assessment of genetic homogeneity in regenerated ansellia africana (Leopard orchid) using gene targeted molecular markers. Plant Cell Tissue Organ Cult. (PCTOC) 133 (2), 299–310. doi: 10.1007/s11240-018-1382-0
Bourgaud F., Gravot A., Milesi S., Gontier E. (2001). Production of plant secondary metabolites: a historical perspective. Plant Sci. 161 (5), 839–851. doi: 10.1016/S0168-9452(01)00490-3
De Klerk G. J., Ter Brugge J., Marinova S. (1997). Effectiveness of indoleacetic acid, indolebutyric acid and naphthaleneacetic acid during adventitious root formation in-vitro in malus ‘Jork 9’. Plant cell Tissue Organ cult. 49 (1), 39–44. doi: 10.1023/A:1005850222973
Devi S. P., Kumaria S., Rao S. R., Tandon P. (2014). Single primer amplification reaction (SPAR) methods reveal subsequent increase in genetic variations in micropropagated plants of Nepenthes khasiana Hook. f. maintained for three consecutive regenerations. Gene 538(1), 23–29.
Devi S. P., Kumaria S., Rao S. R., Tandon P. (2013). In-vitro propagation and assessment of clonal fidelity of nepenthes khasiana hook. f.: a medicinal insectivorous plant of India. Acta Physiol. Plant 35 (9), 2813–2820. doi: 10.1007/s11738-013-1314-x
Dohling S., Kumaria S., Tandon P. (2012). Multiple shoot induction from axillary bud cultures of the medicinal orchid, Dendrobium longicornu. AoB Plants 2012. pls032–pls032. doi: 10.1093/aobpla/pls032
Dodds J. H. (1991). In vitro Methods for Conservation of Plant Genetic Resources. Chapman and Hall, London. pp. 4–5.
Doyle J. (1991). “DNA Protocols for plants,” in Molecular techniques in taxonomy (Springer), 283–293. doi: 10.1007/978-3-642-83962-7_18
Espinosa-Leal C. A., Puente-Garza C. A., García-Lara S. (2018). In-vitro plant tissue culture: means for production of biological active compounds. Planta 248 (1), 1–18. doi: 10.1007/s00425-018-2910-1
Giri L., Jugran A., Rawat S., Dhyani P., Andola H., Bhatt I. D., et al. (2012a). In-vitro propagation, genetic and phytochemical assessment of habenaria edgeworthii: an important astavarga plant. Acta physiol. plant. 34, 869–875. doi: 10.1007/s11738-011-0884-8
Giri L., Dhyani P., Rawat S., Bhatt I. D., Nandi S. K., Rawal R. S., et al. (2012b). In-vitro production of phenolic compounds and antioxidant activity in callus suspension cultures of habenaria edgeworthii: a rare Himalayan medicinal orchid. Ind. Crops Prod. 39, 1–6. doi: 10.1016/j.indcrop.2012.01.024
Gutiérrez R. M. P. (2010). Orchids: A review of uses in traditional medicine, its phytochemistry and pharmacology. J. med. Plants Res. 4 (8), 592–638.
Haisel D., Hofman P., Vágner M., Lipavska H., Tichá I., Schäfer C. A., et al. (2001). Ex vitro phenotype stability is affected by in-vitro cultivation. Biol. plant. 44, 321–324. doi: 10.1023/A:1012415004676
Hussain M. S., Fareed S., Saba Ansari M., Rahman A., Ahmad I. Z., Saeed M. (2012). Current approaches toward production of secondary plant metabolites. J. Pharm. bioallied Sci. 4 (1), 10. doi: 10.4103/0975-7406.92725
Kumar A., Mishra P., Singh S. C., Sundaresan V. (2014). Efficiency of ISSR and RAPD markers in genetic divergence analysis and conservation management of Justicia adhatoda L., a medicinal plant. Plant Systematics and Evolution 300(6), 1409–1420.
Kukulczanka K., Wojciechowska U. (1982). Propagation of two dendrobium species by in-vitro culture. In-vitro Cult. XXI IHC 131, 105–110.
Li Y., Zhu D., Pan H., Zhang Q. (2013). In vitro propagation of three Dendrobium species from stems. Journal of Northeast Forestry University 41(8), 77–81.
Lo S. F., Nalawade S. M., Kuo C. L., Chen C. L., Tsay H. S. (2004). Asymbiotic germination of immature seeds, plantlet development and ex vitro establishment of plants of Dendrobium tosaense makino–a medicinally improrant orchid. In-vitro Cell. Dev. Biol.-Plant 40 (5), 528–535. doi: 10.1079/IVP2004571
Maharjan S., Thakuri L. S., Thapa B. B., Pradhan S., Pant K. K., Joshi G. P., et al. (2020). In-vitro propagation of the endangered orchid dendrobium chryseum rolfefrom protocorms culture. Nepal J. Sci. Technol. 19 (1), 39–47. doi: 10.3126/njst.v19i1.29737
Malabadi R. B., Mulgund G. S., Kallappa N. (2005). Micropropagation of Dendrobium nobile from shoot tip sections. J. Plant Physiol. 162 (4), 473–478. doi: 10.1016/j.jplph.2004.09.008
Murashige T., Skoog F. (1962). A revised medium for rapid growth and bio assays with tobacco tissue cultures. Physiol. plant. 15, 473–497. doi: 10.1111/j.1399-3054.1962.tb08052.x
Nayak N. R., Sahoo S., Patnaik S., Rath S. P. (2002). Establishment of thin cross section (TCS) culture method for rapid micropropagation of cymbidium aloifolium (L.) sw. and Dendrobium nobile lindl. (Orchidaceae). Sci. Hortic. 94 (1-2), 107–116.
Ochoa-Villarreal M., Howat S., Hong S., Jang M. O., Jin Y. W., Lee E. K., et al. (2016). Plant cell culture strategies for the production of natural products. BMB Rep. 49 (3), 149. doi: 10.5483/BMBRep.2016.49.3.264
Oliya B. K., Chand K., Thakuri L. S., Baniya M. K., Sah A. K., Pant B. (2021). Assessment of genetic stability of micropropagated plants of rhynchostylis retusa (L.) using RAPD markers. Sci. Hortic. 281, 110008.
Otero J. T., Ackerman J. D., Bayman P. (2002). Diversity and host specificity of endophytic rhizoctonia-like fungi from tropical orchids. Am. J. Bot. 89 (11), 1852–1858. doi: 10.3732/ajb.89.11.1852
Palombi M., Damiano C. (2002). Comparison between RAPD and SSR molecular markers in detecting genetic variation in kiwifruit (Actinidia deliciosa a. chev). Plant Cell Rep. 20 (11), 1061–1066. doi: 10.1007/s00299-001-0430-z
Pant B., Chand K., Paudel M. R., Joshi P. R., Thapa B. B., Park S. Y., et al. (2022). Micropropagation, antioxidant and anticancer activity of pineapple orchid: Dendrobium densiflorum Lindl. Journal of Plant Biochemistry and Biotechnology 31(2), 399–409.
Pandey S., Sundararajan S., Ramalingam S., Pant B. (2020). Effects of sodium nitroprusside and growth regulators on callus, multiple shoot induction and tissue browning in commercially important valeriana jatamansi Jones. Plant Cell Tissue Organ Cult. 142 (3), 653–660. doi: 10.1007/s11240-020-01890-7
Pant B. (2013). Medicinal orchids and their uses: tissue culture a potential alternative for conservation. Afr. J. Plant Sci. 7 (10), 448–467. doi: 10.5897/AJPS2013.1031
Pant B. (2014). “Application of plant cell and tissue culture for the production of phytochemicals in medicinal plants,” in Infectious diseases and nanomedicine II (Springer: New delhi), 25–39.
Pant B., Paudel M. R., Chand M. B., Pradhan S., Malla B. B., Raskoti B. B. (2018). Orchid diversity in two community forests of makawanpur district, central Nepal. J. Threat Taxa 10 (11), 12523–12530.
Pant B., Shah S., Shrestha R., Pandey S., Joshi P. R. (2017). “An overview on orchid endophytes,” in Mycorrhiza-nutrient uptake, biocontrol, ecorestoration (Cham: Springer), 503–524.
Parab G. V., Krishnan S. (2008). Assessment of genetic variation among populations of rhynchostylis retusa, an epiphytic orchid from goa, India using ISSR and RAPD markers.
Pfab M. F., Scholes M. A. (2004). Is the collection of aloe peglerae from the wild sustainable? an evaluation using stochastic population modelling. Biol. Conserv. 118 (5), 695–701. doi: 10.1016/j.biocon.2003.10.018
Pradhan S., Tiruwa B., Subedee B. R., Pant B. (2014). In-vitro germination and propagation of a threatened medicinal orchid, cymbidium aloifolium (L.) sw. through artificial seed. Asian Pac. J. Trop. Biomed. 4 (12), 971–976. doi: 10.12980/APJTB.4.2014APJTB-2014-0369
Rajbhandari K. R. (2015). A handbook of the orchids of Nepal (Government of Nepal, Ministry of Forests and Soil Conservation, Department of Plant Resources).
Rao S., Barman B. (2014). In-vitro micro propagation of Dendrobium chrysanthum wall. ex lindl. a threatened orchid. Acad. J. Biosci. 2, 39–42.
Regmi T., Pradhan S., Pant B. (2017). In-vitro mass propagation of an epiphytic orchid, cymbidium aloifolium (L.) sw., through protocorm culture. Biotechnol. J. Int. 19 (1), 1–6.
Roy J., Banerjee N. (2003). Induction of callus and plant regeneration from shoot-tip explants of Dendrobium fimbriatum var. oculatum lindl. Sci. Hortic. 97 (3-4), 333–340.
Roy A. R., Sajeev S., Pattanayak A., Deka B. C. (2012). TDZ induced micropropagation in cymbidium giganteum wall. ex lindl. and assessment of genetic variation in the regenerated plants. Plant Growth Regul. 68, 435–445. doi: 10.1007/s10725-012-9732-0
Salvi N. D., George L., Eapen S. (2001). Plant regeneration from leaf base callus of turmeric and random amplified polymorphic DNA analysis of regenerated plants. Plant cell Tissue Organ cult. 66 (2), 113–119. doi: 10.1023/A:1010638209377
Sharma R., De K. K., Sharma B., Majumdar S. (2005). Micropropagation ofDendrobium fimbriatum hook. by green pod culture. J. Plant Biol. 48 (2), 253–257.
Sheelavantmath S. S., Murthy H. N., Pyati A. N., Kumar H. A., Ravishankar B. V. (2000). In-vitro propagation of the endangered orchid, geodorum densiflorum (Lam.) schltr. through rhizome section culture. Plant Cell Tissue Organ Cult. 60 (2), 151–154. doi: 10.1023/A:1006426905052
Sherif N. A., Benjamin J. F., Kumar T. S., Rao M. V. (2018). Somatic embryogenesis, acclimatization and genetic homogeneity assessment of regenerated plantlets of anoectochilus elatus lindl, an endangered terrestrial jewel orchid. Plant Cell Tissue Organ Cult. 132, 303–316. doi: 10.1007/s11240-017-1330-4
Shiau Y. J., Nalawade S. M., Hsia C. N., Mulabagal V., Tsay H. S. (2005). In-vitro propagation of the Chinese medicinal plant, Dendrobium candidum wall. ex lindl., from axenic nodal segments. In-vitro Cell. Dev. Biol.-Plant 41 (5), 666–670.
Sunitibala H., Kishor R. (2009). Micropropagation of Dendrobium transparens L. from axenic pseudobulb segments. Indian J Biotechnol 8, 448–452
Teixeira da Silva J. A., Tsavkelova E. A., Zeng S., Ng T. B., Parthibhan S., Dobránszki J., et al. (2015a). Symbiotic in vitro seed propagation of dendrobium: fungal and bacterial partners and their influence on plant growth and development. Planta 242 (1), 1–22.
Teixeira da Silva J. A., Cardoso J. C., Dobránszki J., Zeng S. (2015b). Dendrobium micropropagation: a review. Plant Cell Rep. 34 (5), 671–704. doi: 10.1007/s00299-015-1754-4
Teixeira da Silva J. A., Zeng S., Galdiano R. F., Dobránszki J., Cardoso J. C., Vendrame W. A. (2014). In vitro conservation of dendrobium germplasm. Plant Cell Rep. 33 (9), 1413–1423. doi: 10.1007/s00299-014-1631-6
Vanisree M., Lee C.-Y., Lo S.-F., Nalawade S. M., Lin C. Y., Tsay H.-S. (2004). Studies on the production of some important secondary metabolites from medicinal plants by plant tissue cultures. Bot. Bull. Acad. Sin. 45 (1), 1–22.
Vij S. P., Pathak P. (1989). Micropropagation of dendrobium chrysanthum wall. through pseudobulb segments. J. Orchid Soc. India 3, 25–28.
Xu J., Han Q. B., Li S. L., Chen X. J., Wang X. N., Zhao Z. Z., et al. (2013). Chemistry, bioactivity and quality control of Dendrobium, a commonly used tonic herb in traditional Chinese medicine. Phytochem. Rev. 12 (2), 341–367. doi: 10.1007/s11101-013-9310-8
Keywords: Medicinal plants, Dendrobium, Protocorm, Primer, ISSR, RAPD, PCR
Citation: Joshi PR, Pandey S, Maharjan L and Pant B (2023) Micropropagation and assessment of genetic stability of Dendrobium transparens Wall. Ex Lindl. using RAPD and ISSR markers. Front. Conserv. Sci. 3:1083933. doi: 10.3389/fcosc.2022.1083933
Received: 29 October 2022; Accepted: 29 December 2022;
Published: 18 January 2023.
Edited by:
Anurag Dhyani, Jawaharlal Nehru Tropical Botanic Garden and Research Institute, IndiaReviewed by:
Mafatlal M. Kher, Ariel University, IsraelAabid Hussain Mir, University of Kashmir, India
Copyright © 2023 Joshi, Pandey, Maharjan and Pant. This is an open-access article distributed under the terms of the Creative Commons Attribution License (CC BY). The use, distribution or reproduction in other forums is permitted, provided the original author(s) and the copyright owner(s) are credited and that the original publication in this journal is cited, in accordance with accepted academic practice. No use, distribution or reproduction is permitted which does not comply with these terms.
*Correspondence: Bijaya Pant, Yi5wYW50QGNkYnR1LmVkdS5ucA==