- 1Urban Conservation Program, Global Conservation Programs, Wildlife Conservation Society, Bronx, NY, United States
- 2Tiger Program, Wildlife Conservation Society, Bronx, NY, United States
- 3Panthera, New York, NY, United States
- 4Nature Conservation Foundation, Mysore, India
- 5University of Maryland, College Park, College Park, MD, United States
- 6SparkGeo, Prince George, BC, Canada
- 7Rocky Mountain Research Station, USDA Forest Service, Flagstaff, AZ, United States
- 8US Geological Survey Southwest Biological Science Center, Flagstaff, AZ, United States
- 9WWF Tigers Alive, World Wide Fund for Nature, Montreal, QC, Canada
- 10IUCN Cat Specialist Group, c/o Foundation KORA, Ittigen, Switzerland
- 11WWF-India, World Wildlife Fund for Nature, New Delhi, India
- 12WWF Tigers Alive, World Wide Fund for Nature, Kathmandu, Nepal
- 13Department of National Parks, Wildlife and Plant Conservation, Government of Thailand, Bangkok, Thailand
- 14Species Conservation Action Team, IUCN Centre for Conservation Action, IUCN, Gland, Switzerland
- 15WWF Tigers Alive Initiative, c/o WWF Cambodia, Phnom Penh, Cambodia
- 16Indonesia Program, Wildlife Conservation Society, Bogor, Java, Indonesia
- 17Laos Program, Wildlife Conservation Society, Vientiane, Laos
- 18Thailand Program, Wildlife Conservation Society, Bangkok, Thailand
- 19Tiger Program, Government of Bhutan, Thimphu, Bhutan
Of all the ways human beings have modified the planet over the last 10,000 years, habitat loss is the most important for other species. To address this most critical threat to biodiversity, governments, non-governmental actors, and the public need to know, in near real-time, where and when habitat loss is occurring. Here we present an integrated habitat modelling system at the range-wide scale for the tiger (Panthera tigris) to measure and monitor changes in tiger habitat at range-wide, national, biome, and landscape scales, as often as the underlying inputs change. We find that after nearly 150 years of decline, effective potential habitat for the tiger seems to have stabilized at around 16% of its indigenous extent (1.817 million km2). As of the 1st of January 2020, there were 63 Tiger Conservation Landscapes in the world, covering 911,920 km2 shared across ten of the 30 modern countries which once harbored tiger populations. Over the last 20 years, the total area of Tiger Conservation Landscapes (TCLs) declined from 1.025 million km2 in 2001, a range-wide loss of 11%, with the greatest losses in Southeast Asia and southern China. Meanwhile, we documented expansions of modelled TCL area in India, Nepal, Bhutan, northern China, and southeastern Russia. We find significant potential for restoring tigers to existing habitats, identified here in 226 Restoration Landscapes. If these habitats had sufficient prey and were tigers able to find them, the occupied land base for tigers might increase by 50%. Our analytical system, incorporating Earth observations, in situ biological data, and a conservation-oriented modelling framework, provides the information the countries need to protect tigers and enhance habitat, including dynamic, spatially explicit maps and results, updated as often as the underlying data change. Our work builds on nearly 30 years of tiger conservation research and provides an accessible way for countries to measure progress and report outcomes. This work serves as a model for objective, range-wide, habitat monitoring as countries work to achieve the goals laid out in the Sustainable Development Goals, the 30×30 Agenda, and the Kunming-Montreal Global Biodiversity Framework.
1 Introduction
Of all the ways human beings have modified the planet over the last 10,000 years, habitat loss is the most important for other species (Tracewski et al., 2016; IPBES, 2019; Powers and Jetz, 2019; Leisher et al., 2022). Habitat describes where a species can find the resources to complete its life cycle (Hall et al., 1997). Loss of habitat diminishes the ability of populations to persist (Fahrig, 2019; Staude et al., 2020). There are several ways in which habitat loss occurs: whole-scale ecosystem conversion, degradation of existing habitat, fragmentation of habitat, and defaunation. These different forms of habitat loss can trigger cascading consequences for other species and ecosystem functions, including the ecosystem services on which human beings rely (Dirzo et al., 2014; Emer et al., 2020; Moreno-Mateos et al., 2020; Williams et al., 2020; Mahmood et al., 2021).
To address this critical threat to biodiversity, governments, non-governmental actors, and the public need to know where and when habitat loss is occurring. They need this information, not at the pace of scientific publications, which, for all the advances made in speeding peer review and digitizing the publication process in recent years, remains a ponderous, laborious, and, therefore, painfully slow procedure. Rather conservationists and civil society need information in as close to real-time as possible to measure losses, count gains, and react consequentially. The development of the global Internet provides a mechanism to share detailed data broadly and quickly at the requisite temporal and spatial scales.
In recent decades, government agencies such as the US National Aeronautics and Space Administration (NASA) and the European Space Agency (ESA) have made timely, high-resolution, landscape-level information from satellite sensors more accessible and affordable than ever before, fulfilling a desire for information about environmental issues and stimulating significant technological advancements in satellite engineering (Turner et al., 2015; Reddy et al., 2019; Vaz et al., 2020). Each year scientists propose new algorithms to combine and transform these data streams into validated, reliable data products that either measure directly, or provide proxies of, essential habitat elements (e.g. Pettorelli et al., 2014; Fernández et al., 2020). Increasingly those algorithms reside on distributed computer arrays such as Microsoft’s Planetary Computer (Microsoft, 2022) and Google Earth Engine (Google, 2022) that enable rapid computation and Internet sharing, which in turn enables fast iteration, learning, and improvement (e.g. Jones et al., 2022; Shirk et al., 2022).
The definition of habitat obviously depends on the life history of the organism in question (Hall et al., 1997); habitat is not the same for whales, wallabies, or tigers. Here we build a model appropriate for habitat of the tiger (Panthera tigris), which could be extended readily to other species with appropriate re-parameterization. Tigers are the world’s largest living cat species, a highly evolved, obligate carnivore whose ancestors inhabited Eurasia some 62 million years ago (Mazák, 1981; Mazák et al., 2011). Tiger habitat consists of areas with cover for hunting and raising cubs, sufficient availability of prey biomass, preferentially medium- and large-sized ungulates, and freedom from persecution by humans, the tiger’s main competitor (Gittleman and Harvey, 1982; Karanth and Sunquist, 1992; Smith, 1993; Miquelle et al., 1999a; Darimont et al., 2023). Before humans entered Asia, the tiger’s habitat was shaped mainly by the climate, as manifested through shifts in vegetation, prey base, and sea and ice levels (Cooper et al., 2016). The indigenous range of the tiger at the time of first impact by human society shows the species living from the Black Sea to the Pacific Ocean and from the southern margins of the boreal forest in Siberia to the tropical rainforests of Bali (Sanderson et al., in press). In political terms, thirty modern nation-states once had suitable habitat with resident tiger populations; as of this writing, only ten do.
Tigers are an endangered species in part because tigers have lost enormous areas of habitat (Goodrich et al., 2022). By one measure (Dinerstein et al., 2007), over 93% of tiger range is now empty of tigers; by another (Walston et al., 2010), nearly 99% of range had been lost, if one counts as viable tiger habitat only the places that are under conservation management and where tigers are known to raise cubs. The main mechanisms of range loss are direct habitat destruction, including physical conversion and fragmentation of suitable vegetation (Joshi et al., 2016; Poor et al., 2019) and the less visible, but no less deadly, depletion of prey populations (Karanth et al., 2004; Miquelle et al., 2015; Miquelle et al., 1999b; Jornburom et al., 2020.) Active persecution by humans has also depleted tiger numbers, including poaching for the illegal trade in tiger parts (Linkie et al., 2018; Villalva and Moracho, 2019; Skidmore, 2021); human-wildlife conflict (Lubis et al., 2020; Gulati et al., 2021); and the long-term consequences of once rampant sport hunting (Pikunov, 2014; Mandala, 2018). Unsustainable hunting of all wildlife, for example, with snares, can drive tigers extinct in otherwise high-quality habitat (O’Kelly et al., 2012; Gray et al., 2018; Figel et al., 2021).
The main mechanisms for conserving tigers, therefore, are halting habitat loss and degradation, preventing persecution, mitigating conflict, and restoring tigers and prey animal communities, all of which require a thorough understanding of habitat conditions and trends (Yang et al., 2019; Lubis et al., 2020; Ten et al., 2021; Adhiasto et al., 2023). Protected areas and Indigenous lands where natural ecosystems are actively managed on behalf of wild plants and animals are critical because they provide the legal and cultural enforcement mechanisms to limit damaging human activities (Soh et al., 2014; Sunarto et al., 2015; Karanth et al., 2020; Nijhawan and Mihu, 2020). Dynamic maps of habitat facilitate overlays with protected areas (UNEP-WCMC and IUCN, 2022), key biodiversity areas (KBA Partnership, 2023), and other spatial data reflecting safe-havens. Fortunately for tigers, their natural fecundity, ability to disperse long distances, and symbolic, spiritual, and ecological importance to human cultures across Asia contribute to the possibility of recovery (WCS Thailand, 2020; Jhala et al., 2021).
Concerns about habitat loss drove the first iteration of the Tiger Conservation Landscape analysis in the 1990s (Dinerstein et al., 1997). That analysis set the model of integrating satellite-derived observations with in situ knowledge of tiger populations to map large contiguous blocks of habitat (TCLs) and assess them with regard to ecological representation. In a second iteration, Sanderson et al. (2006) introduced the human footprint (Sanderson et al., 2002) into the analysis as a measure of human pressure, conducted extensive sensitivity testing, and reanalyzed TCLs using higher resolution satellite imagery and a renewed assessment of tiger distribution in the field and in situ knowledge of tiger populations (Sanderson et al., 2010). Subsequently, these maps have been cited in many analyses and planning efforts to save tiger habitat, plan surveys, and safeguard tigers (e.g. Dinerstein et al., 2007; Forrest et al., 2011; Global Tiger Initiative Secretariat, 2012; Joshi et al., 2016; Harihar et al., 2018; Sanderson et al., 2019; Sabu et al., 2022; Vasudeva et al., 2022).
This paper describes the third iteration of systematic improvements to the Tiger Conservation Landscape approach (abbreviated hereafter TCL 3.0; Figure 1; Table 1). As with previous iterations, TCL 3.0 integrates satellite-derived Earth Observation data with field-based observations of tigers in a well-defined and easy-to-understand spatial modelling framework to estimate how occupied and available habitat has changed annually between 2001 and 2020 using the Google Earth Engine (Gorelick et al., 2017). The primary analytical measure is “effective potential habitat”, meaning habitat with appropriate, tiger-specific, structural characteristics derived from remote sensing and sufficiently low levels of human influence based on a new analysis of the human footprint (Sanderson et al., 2022). We delineate landscapes as interconnected blocks of effective potential habitat, larger than a minimum patch size (which varies by ecoregion, Dinerstein et al., 2017) and minimal connectivity distances across non-habitat.
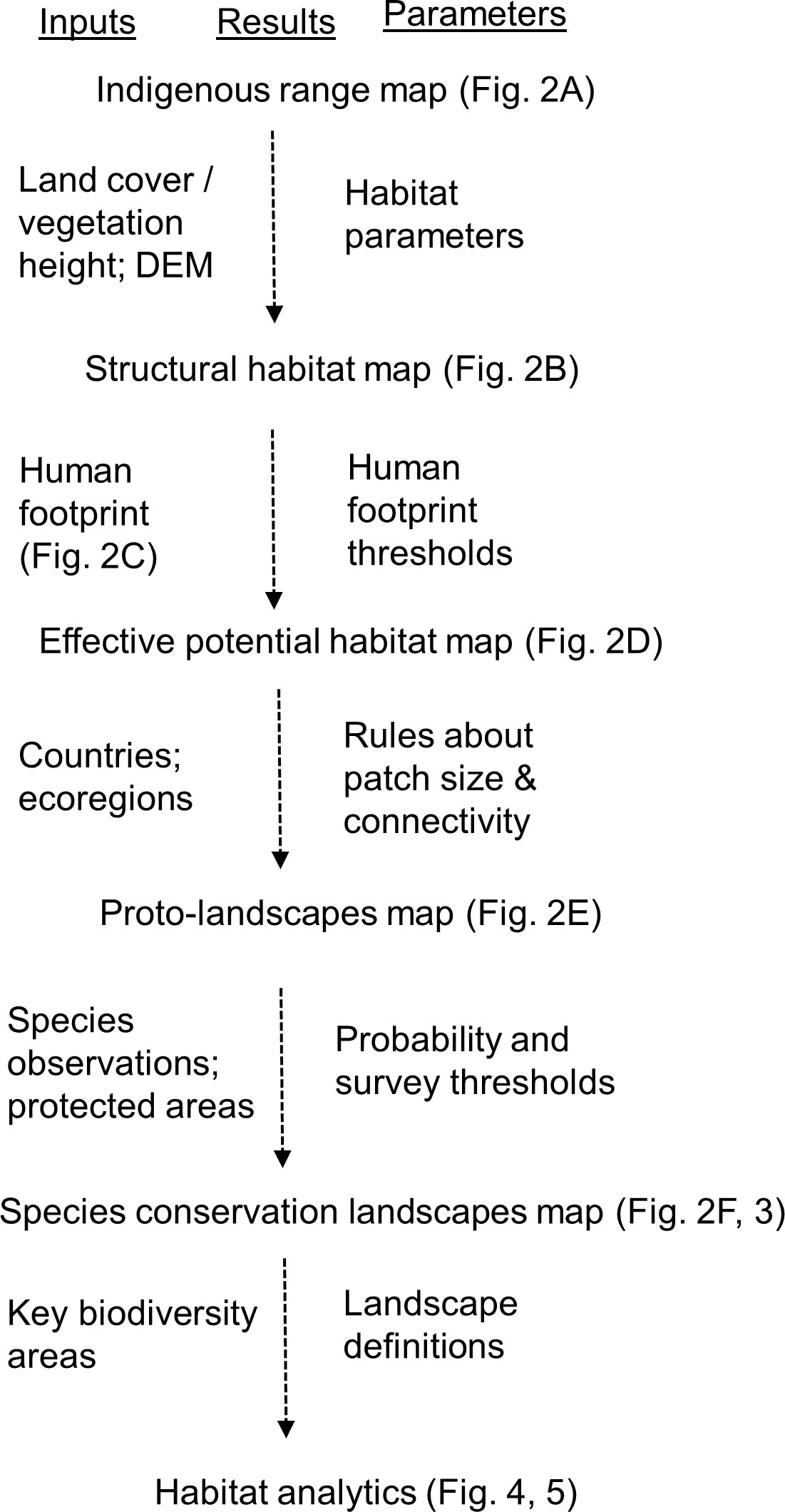
Figure 1 Schematic of analysis of the Species Conservation Landscapes including inputs and parameters.
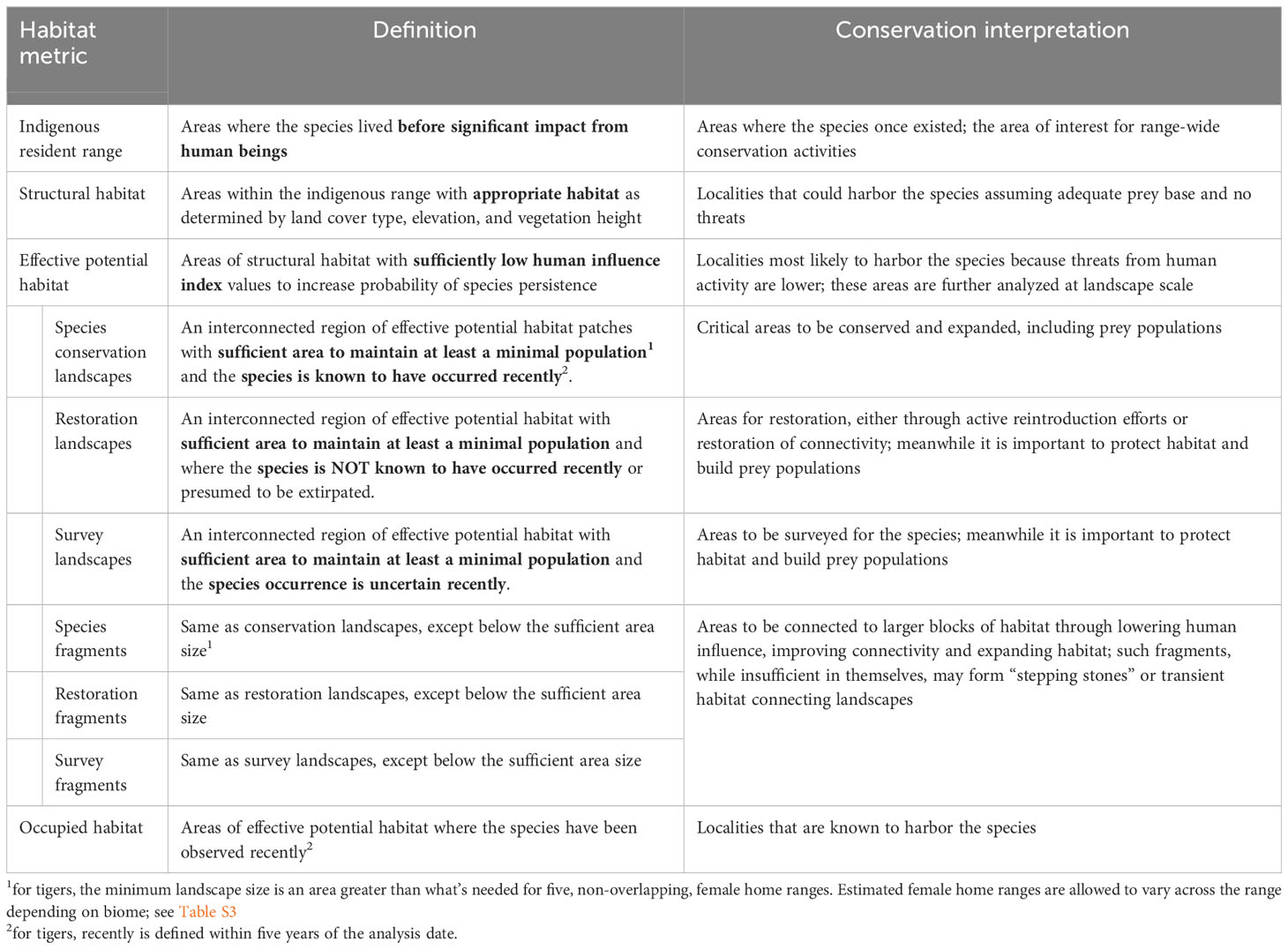
Table 1 Definition of species conservation habitat and landscape metrics with conservation interpretations for the tiger (Panthera tigris).
We now recognize six categories of habitat area (Table 1): Tiger Conservation Landscapes (TCLs), where tigers are found; Restoration Landscapes, where tigers are known or presumed to have been extirpated; and Survey Landscapes, where the status is unclear; and Tiger Fragments, Restoration Fragments, and Survey Fragments (respectively), where patches fall below the minimum patch size.
Our approach is related to, but different from, traditional habitat suitability index (HSI) approaches (e.g. Guisan et al., 2017). Habitat suitability models primarily aim to describe where a species might live, comparable to what we identify as “structural habitat” in our method (see below). While valuable, conservation action requires knowing much more than where a species might be; we also need to know what to do. Here we differentiate landscapes in specific terms that by definition suggest different sorts of conservation actions (Table 1). HSI models typically take observations as independent data to find dependencies among landscape factors (both biological and anthropogenic), usually through application of a statistical model. Here we model the habitat from first principles (e.g. land cover, then human footprint, then patch size and connectivity). Only later in the process do observations enter to classify the landscapes by type. The result is that for areas where tigers are no longer present, we can suggest why they have been extirpated, by measuring physical loss of structural habitat, trends in human influence (and implied conflict), and/or changes in patch size and fragmentation. Landscape classification suggests to conservation authorities where to address efforts and what kinds of efforts to ensure the long-term survival of tigers at range-wide, country-wide, and local scales.
Using our conservation-centered approach, here we summarize the current state of wild tiger habitat and discuss trends in habitat change over the last 20 years. We close with a few comments about how this approach to habitat mapping can be used for national-level reporting for international conventions and by countries to ensure the lasting existence of wild tigers on Earth.
2 Materials and methods
2.1 Study area
We analyzed tiger habitat annually, on January 1st of each year from 2001 – 2020, within the “likely resident indigenous range” of the tiger as defined in Sanderson et al. (in press) (Figure 2A). The indigenous range mapping indicates which ecoregions, or parts of ecoregions, were most likely to have resident tigers before human beings became a significant factor shaping the distribution of the species (Sanderson et al., 2019). This definition is consistent with how the indigenous range is defined by the IUCN Green Status Assessment process (Akçakaya et al., 2018; see also Stephenson et al., 2019). Within the study area, raster analyses were made over the 300 m grid cells coincident with the ESA CCI Landcover data (European Space Agency, 2017) used for the structural habitat mapping (see below). Previous TCL mappings used a base resolution of 1 km cells, so our landscapes are approximately 3x better resolved than earlier efforts.
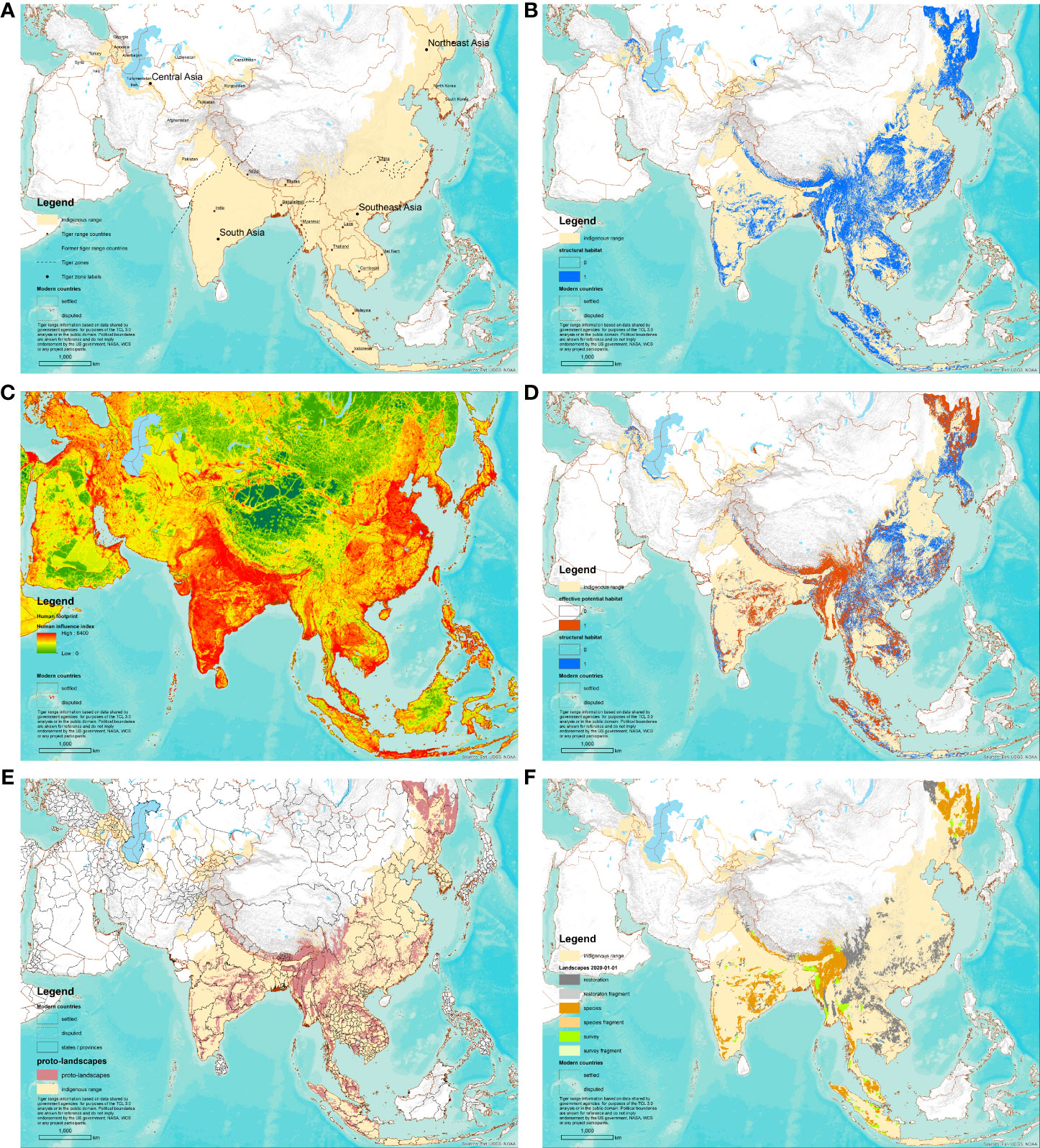
Figure 2 Species Conservation Landscape Mapping process, with application to tigers (Panthera tigris) for 1 January 2020. (A) Map of the likely resident indigenous range, with zone boundaries (dotted lines). (B) Map of structural habitat as revealed by land cover, elevation, and vegetation height. (C) Map of human footprint (Sanderson et al., in press). (D) Map of effective potential habitat (orange) overlaid structural habitat (blue). Areas of structural habitat above the human footprint threshold for each zone show as blue. (E) Map of proto-landscapes overlaid state or province boundaries for each current or former range state. (F) Map of six categories of Tiger Conservation Landscapes on 1 January 2020.
We divided the study area into four zones along ecoregional boundaries, representing major differences in tiger biology and status: South Asia, Southeast Asia, Northeast Asia, and Central Asia (Figure 2A). These zones were used to create a grid-based system for mapping species observations as areas of analysis for the human footprint (Sanderson et al., 2022) thresholds, and for assigning minimum patch sizes, as described below.
2.2 Extirpation masks
We also developed maps of ecoregions or parts of ecoregions where tigers had been declared extirpated, including broad swaths of the Black Sea region, Central Asia, Indochina, and central China, for each year from 2001 – 2020 (Sanderson et al., in press). For extirpated areas, we assigned the approximate date of extirpation and provided supporting references.
2.3 Species observations
We systematically reviewed the literature to identify peer-reviewed studies, government and non-governmental organization reports, and in some cases, newspaper accounts to develop a temporally and spatially explicit observational database from 1995 – 2021 for tigers. Observations include any information of direct observations of the species, including systematic surveys, that document whether or not the species was detected. We also included surveys for other mammal species (e.g. small cats, wild cattle) using techniques that would likely have detected and reported tigers, especially in parts of the range and/or time periods where or when no tiger-specific studies were available.
We began by searching Google Scholar and Web of Science using keywords “tiger(s)” OR “Panthera tigris” and “survey” OR “field” in journals focused on ecology, environmental science, and conservation. Next, we filtered the extensive literature by reviewing titles and abstracts and, where necessary, article contents, keeping only papers that provided first-hand observational data on tigers or where second-hand summaries provided access to otherwise unpublished information. We also contacted tiger biologists and conservation officers working in tiger range countries to share information and identify sources our review might have missed, including non-English language sources and experts (Table S1). Finally, for a few localities where scientific accounts were unavailable because of censorship or lack of data sharing, we supplemented scientific observations with accounts of human-wildlife conflict drawn from a search of newspaper and magazine accounts, confirming observations through independent sources and/or photographic evidence. We maintained all the bibliographic details in a read-only, public bibliography: https://www.zotero.org/groups/2516009/tiger_conservation/library. A full list of sources is provided in Table S2.
The four types of data were:
Camera trap studies (full details): Camera trap studies were represented with a deployment table, including information on location, deployment and pick-up dates, and a corresponding observation table, including dates of positive detections, sex and age class (adult or juvenile) of the tigers observed.
Camera trap studies (summary): Camera trap studies where the full details were unavailable, but the dates of study, study area, observed density, and measures of variation in that density (standard error or confidence intervals; see Moy, 2021) were available, we created observations in a modified version of the ad hoc template.
Sign surveys: Sign surveys were handled with a survey table describing the locations and replication of the surveys, and an observation table, describing which replicates had positive detections.
Ad hoc observations: All other observations were summarized in “ad hoc” observation tables, which gave the date and location of positive detections of tigers, with no measures of the search effort.
Observations were located by coordinates, if available, or by matching to a standardized grid reference, if not. We created an overlay of grid cells that varied in size in different zones of tiger range, depending on a summary of tiger home range information (Table S3). In South Asia, we used cells with a grid side of 4 km (area of 16 km2); in Southeast Asia, including Sumatra, we used 16 km cells (area of 256 km2); and in Northeast and Central Asia, we used 32 km cells (area of 1024 km2). If precise coordinate data were unavailable in the paper or from the study authors, we georeferenced map figures and extracted spatial locations according to the overlying grid cells.
Observations were dated to the day of the observation when possible, and if not to an observation period, with a start and end date. If survey dates were given in months (e.g. March 2004 – October 2004), we used the first and last days of the corresponding months. Where observations were in years, we used the first and last days of the corresponding years.
All observations were tagged with the observer’s name, institution, email address, and reference. For multi-authored studies, we identified the observer as the survey leader, where given, or the corresponding author’s name, where not. We agreed, as a matter of security for the safety of tigers and to respect the research interests of the investigators, not to reshare the point locality information used for the analysis. They do not appear on the website or in this paper.
2.4 Structural habitat
As ambush hunters, tigers need vegetative cover to stalk and kill prey (Sunarto et al., 2012). They also use thick vegetation to hide their cubs during weaning (Smith et al., 1998). We used land use/land cover data developed by the European Space Agency to identify land cover classes that are possibly tiger habitat and vegetation height product derived from NASA’s Landsat sensor (Potapov et al., 2019). Both datasets were rescaled to a common 300 m grid. For tigers, structural habitat was defined land use/land cover classes where tigers are typically found – essential habitat types with sufficient cover for tigers to hunt and raise cubs (Table S4). For mixed mosaic classes, we add another criterion, including areas with at least 5 meters of vegetation height (Figure 2B). For the Northeast Asia zone, we excluded areas 1000 meters above sea level; elsewhere we applied an elevation threshold of 3350 meters, though note that rarely tigers have been observed at higher elevations in the Himalayas (Adhikarimayum and Gopi, 2018; Shrestha et al., 2021).
2.5 Effective potential habitat
Factors invisible to satellite sensors impact where tigers can live (Redford, 1992; Harrison, 2011). Tigers are illegally hunted or killed in many parts of the range after human/wildlife conflict (Nyhus and Tilson, 2004; Karanth and Gopal, 2005; Musavi et al., 2006; Lubis et al., 2020). Tigers may also be depleted by the lack of prey caused by indirect competition with human hunters (Miquelle et al., 1999b; Karanth et al., 2004; Johnson et al., 2006). Because we lack range-wide, spatially and temporally explicit data on prey depletion and human disturbance, we use a proxy: the human influence index, or informally, the human footprint (Sanderson et al., 2002; Venter et al., 2016; Sanderson et al., 2022). The human influence index is a unitless, weighted sum based on human population density, infrastructure, accessibility, and power consumption on a 0 – 60 scale (Figure 2C). The result is a gradient map of human impacts that have been widely correlated to human disturbances and range collapse in large mammals (Yackulic et al., 2011; Di Marco et al., 2018; Tucker et al., 2018).
We recorded the human influence index values and zones where tigers have been observed between 2001 – 2020. Following methods developed by Sanderson et al. (2006), we randomly sampled the human influence index at the same number of locations within each zone. We repeated this analysis a thousand times for each year for each zone. Then, we calculated frequency histograms of human influence index values for the tiger detections and the random sample for each zone and subtracted them to show human influence values where tigers are more or less likely than random selections would suggest, equivalent to a chi-squared test (Nikulin, 1973). The lowest human influence value where the difference in frequency between the tiger and random distributions is zero, we call the “social tolerance threshold” (Figure S1).
To define effective potential habitat, we excluded areas of structural habitat where the human influence index value was greater than the social tolerance threshold for a given zone and year (Figure 2D). We applied the threshold from the Northeast Asia zone to the Central Asia zone.
2.6 Landscape delineation
We applied a two-step delineation process to group patches of effective potential habitat into landscapes. First, we found all patches of contiguous cells and tested them against core patch size specific to ecoregions (Table S3). A “core” patch size is defined as an area large enough to maintain at least five tigers, based on densities observed in the literature. “Stepping stone” patches were also recognized that were one-tenth the size of a core patch. These small patches are considered to provide temporary respites during movement, but the patches are not large enough to maintain a tiger for a breeding season. In a second step, we tested how patches were close enough to be considered potentially connected. If core patches lay within 4 km of each other, or were connected with “stepping stone” patches within 4 km, they were delineated as part of the same landscape (Figure 2E).
2.7 Landscape attribution
We attributed landscapes, or portions of landscapes, into one of six classes based on size, the presence of the species, and the survey effort applied to detect them, based on the observational data for the last five years. The classes are summarized in Table 1. We analyzed landscapes using underlying state/province boundaries of countries (administration level 1 as defined in the Global Administrative Database – see Anonymous, 2021). States/provinces represent potentially important political differences in management regime and cultural situations that might differentially impact portions of species conservation landscape (Figure 2E). Observations with coordinates were assigned with a point in polygon analysis. Observations located by grid cells were assigned based on the centroids of the grid cells to the overlapping landscape.
2.8 Probability of presence and level of survey effort
To integrate various survey data, estimate the effective amount of survey effort and account for variation in the size of landscape patches, we fit a multi-scale occupancy model (Nichols et al., 2008), in which the landscape patches were the coarser scale and grid cells were the finer scale (and whose dimensions were defined approximately based on average tiger home range size in that zone). In multi-scale occupancy models, occupancy at the finer scale is conditional on occupancy at the coarser scale – in other words, tigers can only occupy a grid cell within a landscape patch if the landscape patch is occupied; however, there can also be unoccupied grid cells within an occupied landscape patch. In this context, the finer scale occupancy would be equivalent to the probability of use. To fit the multiscale occupancy model, observations and effort associated with sign surveys and camera trap surveys with full details were attributed to grid cells nested within landscape patches. Occupancy at the coarser scale was a function of random effects for state/provinces, and fixed effects for area of state/province and proportion of the area protected. We standardized area of each subnational unit before fitting models. We used constant (e.g., no covariates) probability of detection for camera trap surveys and constant probability of detection for sign surveys.
Models were fit in JAGS (Plummer, 2003) run from Python using a Python interface to JAGS, PyJAGS (pyjags version 1.3.8) to analyze Bayesian hierarchical models. We chose a Bayesian framework because it allowed us incorporate the random effects detailed above more easily.
For each landscape patch, we estimated the unconditional probability that tigers were present in a landscape (given the landscape patch’s potential effective habitat area, protected area status, state/province, and ecoregion). We also estimated the conditional probably that tigers were present given the observational data from the last five years (e.g. for the 2020 analysis, observational data applicable, based on the start and end dates of the study, from 2016 – 2020). If ad hoc or camera trap summary data indicated that a landscape patch was occupied and its conditional probability was less than 1 (i.e., no tigers were seen in the detailed camera trap data or sign survey data), the conditional probability was changed after model fitting to 1. We measured survey effort of landscape patches by taking the ratio of the conditional to the unconditional probability subtracted from 1.
Tiger conservation landscapes were defined as areas with a conditional probability of the presence of tigers of >=99%. Survey landscapes have a lower conditional probability (<99%) and a survey effort below 0.6. Restoration landscapes have a lower conditional probability and a survey effort greater than 0.6.
After classifying the landscapes into the six classes, contiguous landscapes of the same class were recombined to create the final landscape delineation, as shown in Figures 2F, 3.
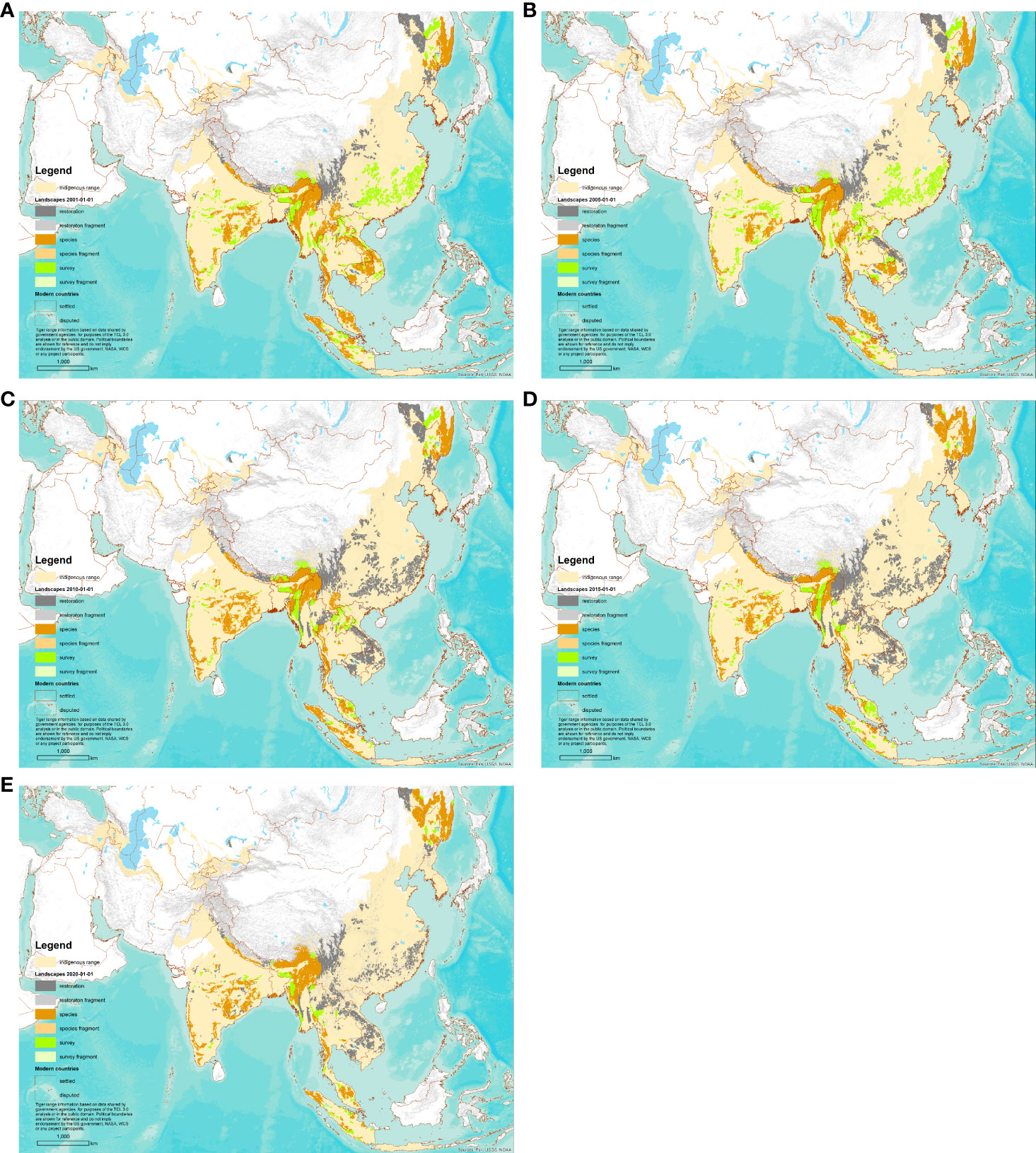
Figure 3 Map of Tiger Conservation Landscapes on five dates. Note: Figures 2F and 3E are the same. (A) 1 January 2001. (B) 1 January 2005. (C) 1 January 2010. (D) 1 January 2015. (E) 1 January 2020.
2.9 Landscape summary
We created several automated summaries to make the information contained in these data more tractable to policymakers and on-the-ground managers (Figures 4, 5; Tables 2–4). Each landscape is analyzed against the World Database of Protected Areas (UNEP-WCMC and IUCN, 2022) to measure the extent of protection and the Key Biodiversity Areas (IUCN, 2016) to measure its importance for meeting Sustainable Development Goals (United Nations, 2015) and other biodiversity-related targets. This information is available for each country via the project website at act-green.org
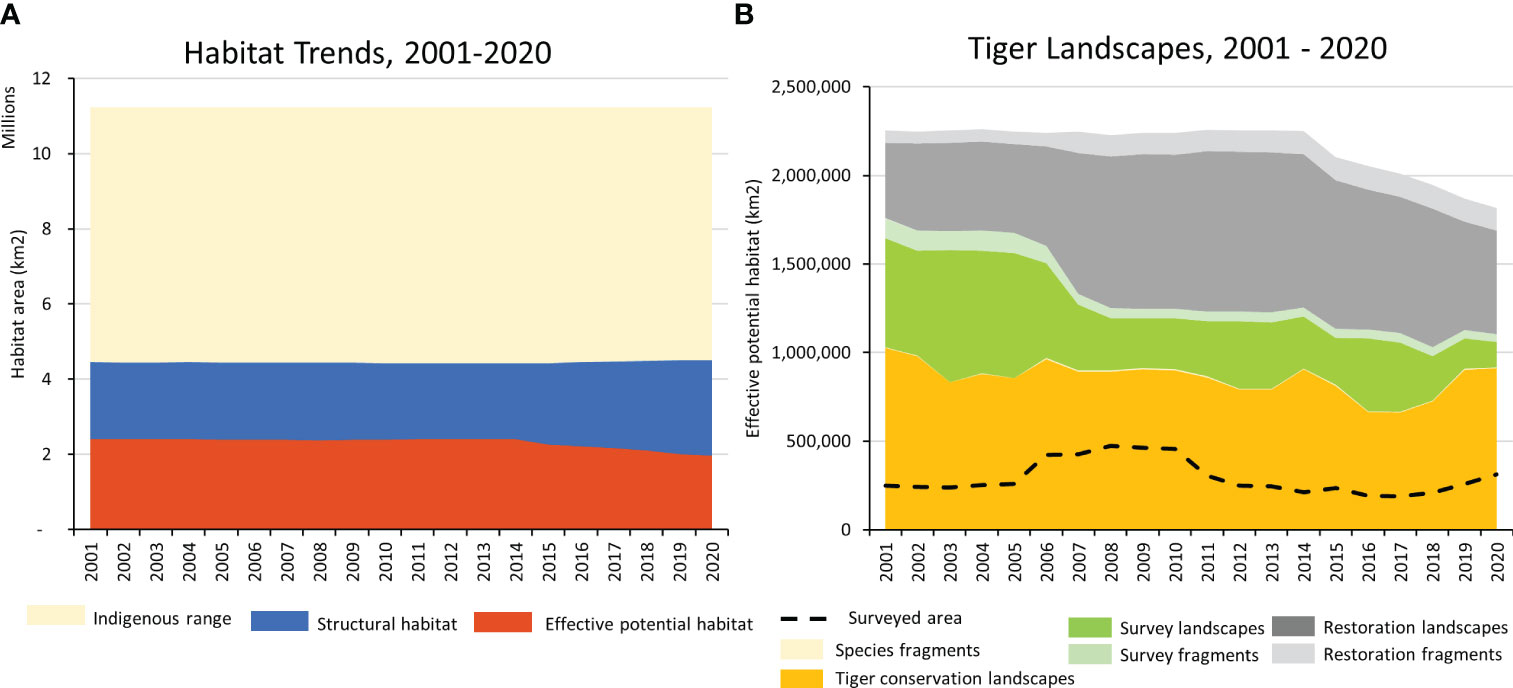
Figure 4 Range-wide trends. (A) Trends in structural habitat and effective potential habitat within the tiger’s likely resident indigenous range, 2001 - 2020. (B) Trends in Tiger Conservation Landscapes, Survey Landscapes, and Restoration Landscapes, fragmented landscapes, and estimated surveyed area from 2001 – 2020.
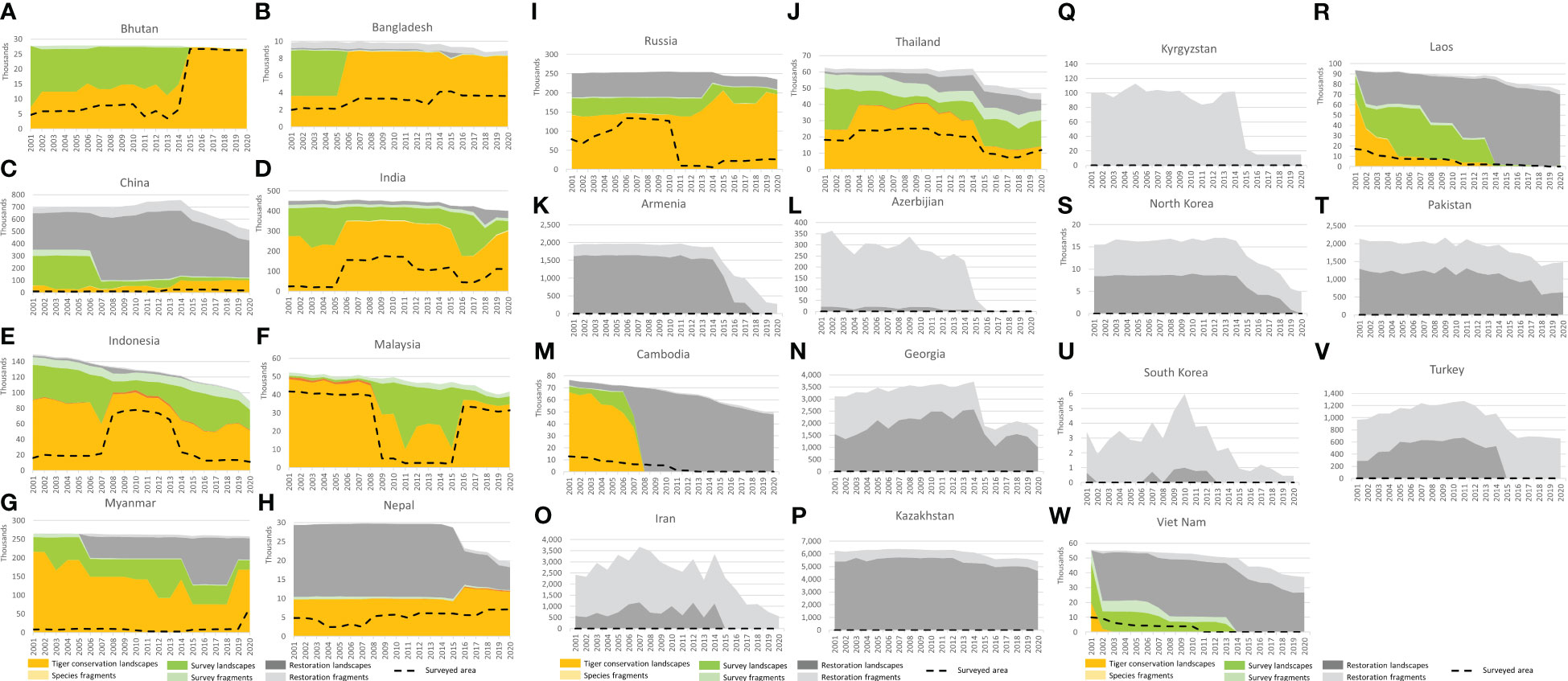
Figure 5 National level trends in Tiger Conservation Landscapes, Survey Landscapes, and Restoration Landscapes, fragmented landscapes, and estimated surveyed area from 2001 – 2020. The first 10 figures (A–J) show countries, where tigers lived in 2020. The remaining 13 figures (K–W) show former range states with some extant habitat, where tigers lived prior to 2020. The following six countries no longer have any effective potential habitat for the tiger: Afghanistan, Iraq, Syria, Tajikistan, Turkmenistan, and Uzbekistan, according to this analysis – see discussion. Note that y-axis values vary plot to plot. (A) Bhutan. (B) Bangladesh. (C) China. (D) India. (E) Indonesia. Fig. Malaysia. (G) Myanmar. (H) Nepal. (I) Russia. (J) Thailand. (K) Armenia. (L). Azerbaijan. (M). Cambodia. (N). Georgia. (O). Iran. (P). Kazakhstan. (Q). Kyrgyzstan. (R). Laos. (S). North Korea. (T). Pakistan. (U). South Korea. (V).Turkey. (W). Vietnam.
2.10 Data processing
The steps above were implemented on Google Earth Engine (Gorelick et al., 2017) in a task-based architecture, implemented in Python (Python Software Foundation, 2022) and designed to be recalculated as often as the underlying data change. All areas are reported based on Google Earth Engine’s area calculation procedure, which treats each pixel element as its true three-dimensional area, not dependent on any particular map projection. The open-source code is available at https://github.com/SpeciesConservationLandscapes.
2.11 Quality control
Each tiger observation study was reformatted into a data entry form compatible with the database schema. Another analyst (usually the senior author) separately reviewed each data entry form before inclusion in the database. Through extensive online consultations, we also reviewed the landscape outputs with tiger biologists and conservationists noted in Table S1. Our results are replicable by other scientists as our code is open-source, and we identify in detail the sources used (Table 2). Sensitivity testing of the model was conducted, as described in Sanderson et al. (2006).
3 Results
3.1 The state of tiger conservation landscapes on 1 January 2020
As of 1 January 2020, planet Earth possessed only 63 localities where wild tigers were known to occur. These 63 Tiger Conservation Landscapes covered 911,920 km2 across ten range states (Figures 1-3, 4A, Table 2). They represent only 8.2% of the tiger’s likely resident indigenous range, suggesting a loss of approximately 91.8% of the world’s tiger habitat.
These 63 areas are the key landscapes for tiger conservation. By definition, each TCL had a high probability of resident tiger populations during the previous five years (i.e., between 1 January 2015 and 31 December 2019) and enough habitat with sufficiently low levels of human influence to sustain a population of at least five adult females. Designating an area as a TCL does not mean tigers exist everywhere within the mapped habitat block(s), however. Within the TCLs, only approximately a third of the total TCL area (311,698 km2) has been surveyed in the previous five years (Figure 4B).
Eight of the 63 TCLs identified in 2020 were transboundary. The largest TCL in the world was the Northern Triangle of India, Myanmar, China, Bhutan, and Bangladesh, which covered 294,847 km2 in 2020, 32.3% of all TCL area worldwide. Unfortunately, tigers are unlikely to be continuously distributed throughout this vast area (Lwin et al., 2021; Sarkar et al., 2021; Sabu et al., 2022). Conflicts in Myanmar and India have constrained on-the-the ground surveys; the consequences of those conflicts for tigers and their prey remain poorly understood (Win, 2022).
All TCLs are important, but many were much smaller than the Northern Triangle. the Dawna Range, Ranthambore or Kaziranga TCLs, all cover less than 500 km2. India had the largest number of TCLs in a single country at 35 landscapes due to fragmentation of what was once a much more continuous habitat but also concerted conservation efforts (Aylward et al., 2022). Nepal had the fewest TCLs (1), but a critical one, the inter-connected and extensive Terai Arc TCL of flooded grasslands and riparian forests, shared with India (Biswas et al., 2020; Thapa et al., 2021; Yadav et al., 2022). Other TCLs representing a diversity of habitats are found in the Russian Far East, parts of Southeast Asia, and on the island of Sumatra, as described below.
TCLs comprised only 50% of Asia’s effective potential habitat extant on January 1, 2020 (Table 2). The remaining 50% was a combination of Restoration Landscapes, Survey Landscapes, or small fragments (tiger fragment, restoration fragment, or survey fragment; Table 1). Restoration Landscapes comprised 32% of the total effective potential habitat in 2020; Survey Landscapes, 8%, and all fragments, 9.3% (Table 2). Fragmentation has been increasing over time, generating shards of habitat that are of little use to tigers but may provide future building blocks for connectivity.
Our classification of landscapes is based on 102,418 observations collected from 362 unique sources over the last 20 years (Table S2). The majority of observations were of the “ad hoc” type, 55,684; sign survey observations, provided another 45,701 observations; and camera trap studies, 1,033 observations. By combining these observations with billions of satellite-based observations and human footprint calculations, we were able to follow range-wide trends in TCLs and related landscape types.
3.2 Trends in tiger conservation landscapes, 2001 - 2020
There are many ways to measure tiger habitat loss. The baseline deployed here is the “likely resident indigenous range”, an area of approximately 11.1 million km2 where tigers are thought to have once lived (Sanderson et al., in press). This enormous range in Asia encompasses areas included in 30 modern countries, from Turkey to Russia, and China to Indonesia (Table 2).
Since the time tigers were first significantly impacted by human beings, hundreds to thousands of years ago, up until 1 January 2020, tigers have lost an estimated 60% of the structural habitat, meaning that approximately 6.7 million km2 of vegetative cover suitable for tigers has been lost through conversion to other uses such as agriculture or urban development. High levels of human influence have reduced the utility of another 2.5 million km2 of structural habitat (23% of the indigenous range) through fragmentation, human-wildlife conflict, and likely concomitant reduction of the prey base. Direct persecution of tigers and/or tiger prey has further extirpated tigers from another 711,634 km2 of suitable habitat (6% of indigenous range) that otherwise appears to be structurally sound and with low levels of human influence, as measured by the mapping of Restoration Landscapes. Regarding tigers, these areas can be considered “empty forests” (sensu Redford, 1992). Finally, lack of knowledge constrains our ability to understand tiger habitat, as measured by the Survey Landscapes. The status of tigers remains uncertain in 188,364 km2 of habitat (2% of indigenous range), a decline of -75% since 2001. The decreasing amount of area needing survey reflects the increased effort to assess tiger status across Asia, but also underscores that there are fewer and fewer places to look.
Over the last 20 years, the total area of Tiger Conservation Landscapes (TCLs) declined from 1,025,488 km2 in 2001 to 911,901 km2 in 2020, a range-wide loss of 11% of TCL area (Figure 4B). TCLs also became more fragmented. The area of tiger fragments has increased 37%, from 3,477 km2 in 2001 to 4,787 km2 in 2020, though such fragments with tigers represent only a tiny percentage – less than 1% – of overall occupied habitat. Because of fragmentation, the number of TCLs has also increased, from 53 in 2001 to 63 in 2020.
These range-wide trends can be further decomposed at the national scale. In the following sections, we describe important differences in trends among tiger range countries over the first two decades of the 21st century.
3.2.1 South Asia (India, Nepal, Bhutan, Bangladesh)
In 2020, India had the greatest area of Tiger Conservation Landscapes (300,508 km2) of any range country, 33% of the global total (Table 2; Figure 5D). Our analysis suggests that India’s TCL has expanded by approximately 10% in area over the last 20 years, through a combination of conservation efforts and improved surveys, including several, massive, country-wide surveys (Jhala et al., 2008; Jhala et al., 2011; Jhala et al., 2015; Jhala et al., 2018). The quality and precision of these surveys have improved over time as new methods have been implemented (Jhala et al., 2021), making it possible for the Indian government and people to understand where its tigers are and how their long-term survival can be encouraged. These results are also critical for range-wide assessments such as TCL 3.0.
Nepal’s TCL area grew 21% from 2001 to 2020, especially after tigers were reported in the eastern part of the Terai Arc (Figure 5H; Lamichhane et al., 2018; Bista et al., 2021). Nepal’s TCL area lies in one, long, inter-connected landscape, the Terai Arc, shared with India. The connection to Bhutan and the North Triangle TCL through Sikkim has been lost, though a few recent sightings (Ganguli-Lachungpa, 1998; Umariya et al., 2022) suggest there may be hope for future reestablishment in that high-altitude area.
Bhutan’s tigers and tiger habitat are relatively well-protected compared to many parts of the range, with little overall change in landscape area over the last 20 years (Figure 5A). The apparent increase in TCL area from 2001 to 2020 is large at 274%, but that change reflects a relatively weak-observational base prior to the full country-wide survey in 2015 (Thinley and Curtis, 2015). Bhutan’s TCL area subsequently slightly declined (-1.8%) and, as of 2020 stands at 26,762 km2. Though not obvious because of the general connectivity of the habitat, the level of protection and the continuous cover has enabled tigers to reestablish in some previously vacated areas (Thinley et al., 2020).
Bangladesh’s tigers primarily live in the Sundarbans mangrove forest shared with India (Jhala et al., 2016; Hossain et al., 2018). Improved survey results since 2006 showed a TCL area that year of 8,783 km2 compared to 8308 km2 in 2020 (a marginal decline of 5% since 2006 but an apparent increase of 130% since 2001 because of additional survey efforts; Figure 5B). Sea level rise affecting the mangrove forest habitat is a major concern for the long-term future of Bangladesh’s tiger populations (Mukul et al., 2019), since they are extirpated from the northern part of the country. However, there may be some possibility of reestablishing tigers in the Chittagong Hills (Chakma, 2016; Creative Conservation Alliance, 2016), an area of habitat contiguous with the forests of western Myanmar.
3.2.2 Southeast Asia (Myanmar, Thailand, Malaysia, Indonesia, Vietnam, Cambodia, Laos)
The status of tigers in Southeast Asia has shifted from worrying to extremely concerning to dangerous. Three countries have lost tigers entirely over the last 20 years, and others have seen notable reductions in TCL area.
On the map, Myanmar is apparently in the best shape because large blocks of available habitat are revealed in structural habitat analysis, but the status of tigers in those landscapes is very poorly understood (Figure 5G; Rao et al., 2010; Ministry of Natural Resources and the Environment, 2020; Lwin et al., 2021). Civil disorder and strife have limited research access to many promising-looking areas over the last two decades. According to our analysis, Myanmar’s TCLs have lost a minimum of one-fifth (22%) of their area since 2001. Some areas mapped as TCL might be better classified as Survey or Restoration Landscapes, though they technically meet the minimum definition of a TCL given how interconnected forested areas appear in satellite-based analysis. The large “Northern Triangle” TCL in the northern part of the country remains connected with tiger habitat in northeast India, eastern Bangladesh, southern China, and Bhutan. Together these areas comprise the largest TCL in the world, however, the majority of this TCL may be missing its tigers. The total population of tigers within Myanmar was estimated to be less than 50 individuals (Ministry of Natural Resources and the Environment, 2020).
Thailand’s TCLs have lost 43% of their area since 2001 (Figure 5J). Relatively large blocks of habitat are present in the northern part of the country, where the status of tigers is unknown, but it seems unlikely that many, if any, tigers remain there. The status of such areas depends in part on what’s happening across the border in Myanmar, which is poorly understood. Total effective potential habitat has declined 25% over the last 20 years, and connectivity has dropped (Suttidate et al., 2021). On the positive side, the few landscapes where tigers are found today, in the eastern part of the country (Ash et al., 2020; Sukmasuang et al., 2020) and especially in the Western Forest complex, are frequently and thoroughly surveyed, well-protected, and maybe providing a source of dispersing tigers (WCS Thailand, 2020; Phumanee et al., 2021).
Malaysia’s TCLs have lost 29% of their area since 2001, and overall effective potential habitat is down 20% since 2001 (Figure 5F). Tiger numbers also appear to have dropped below 200 individuals (Ten et al., 2021). A gap in survey coverage between 2009 – 2015 caused an apparent precipitous 79% drop in TCL area between 2001 – 2011, but tigers probably persisted throughout this period, just in increasingly fragmented habitats. The largest TCL in 2020 is the Taman Negara – Hala Bala TCL, at 31,296 km2, which shares a small amount of habitat in extreme southern Thailand and is comprised of several large forest blocks at risk of further fragmentation by development in the north-central part of the mainland (Adyla et al., 2016; Rayan and Linkie, 2016; Ghazali et al., 2019; Kawanishi, 2020). The Endau Rompin TCL is an island of habitat in the south, divided by major highways from areas to the north (Gumal et al., 2014). Malaysia has no Restoration Landscapes.
Indonesia’s TCLs on Sumatra have declined -43% in area from 2001 – 2020, and overall effective potential habitat is down 40% over that same period (Figure 5E). Like Malaysia, there are no Restoration Landscapes as of 2020-01-01 in Indonesia. Tigers lived on Bali into the 1940s and Java into the 1980s (Xue et al., 2015), but no low human footprint forest blocks appear large enough to support even five tigers on those islands. On Sumatra, the Leuser Ecosystem in the north remains the largest remaining TCL, at 27,703 km2, though human-wildlife conflict remains a problem (Lubis et al., 2020). Several smaller TCLs persist in the western mountains, especially in and around Kerinci Seblat National Park, and a combination of TCLs and Survey Landscapes in the southwest around Bukit Barisan Selatan National Park (Pusparini et al., 2018). The relative number and status of TCL and Survey Landscapes across years vary because of gaps in the data, making it difficult to draw strong conclusions about trends from our analysis. Other work suggests that tiger population trends are mainly downward for tigers through a combination of poaching, human reprisals after conflict, loss of habitat connectivity, and deforestation (Sunarto et al., 2012; Risdianto et al., 2016; Luskin et al., 2017; Poor et al., 2019). Limitations on data availability meant we had to lean heavily into newspaper accounts of human-wildlife conflict (Marthy, 2021).
As noted above, Vietnam, Cambodia, and Laos all lost their tigers during the last 20 years (Gray et al., 2017; Rasphone et al., 2019; Vietnam News Agency, 2022). In these countries, total effective potential habitat has declined 33%, 35%, and 21%, respectively (see Figures 5M, R, W) Although the pattern may be correlative, not casual, loss of tigers seems to be accompanied by increased rates of loss of effective potential habitat. In Cambodia, tigers were extirpated around 2008. From 2002 – 2007, the annual rate of loss of effective potential habitat in Cambodia was, on average, 927 km2 per year. From 2008 – 2020, the rate of loss was 1,638 km2 per year, or an increase of 77% since extirpation. In Laos, tigers seem to have been extirpated around 2014. From 2002 – 2013, the annual rate of loss of effective potential habitat in Laos was on average 422 km2 per year. From 2014 – 2020 the average annual rate of loss was 2,114 km2, or a quadrupling of the rate of loss of tiger habitat (401% increase in the annual rate of loss post-extirpation). In Vietnam, tigers seem to have been extirpated around 2004. From 2002 – 2004, the annual rate of loss of effective potential habitat in Vietnam was, on average, 218 km2 per year. From 2005 – 2020, the annual rate of loss was 1,112 km2, or a quadrupling of the rate of loss of tiger habitat (410% increase in annual rate of loss post-extirpation). Although only a few data points, these results suggest that losing tigers is a leading indicator of deforestation.
3.3.3 Northeast Asia (China, Russia, North Korea, South Korea)
Eastern Russia remains a critical area for tiger conservation, both because of the size of the area and their ecological distinctiveness as a mixed temperate forest on the margins of the boreal (Seryodkin et al., 2017; Dou et al., 2019; Qi et al., 2021). Following India, Russia is the country with the most TCL area in the world (195,819 km2), 19% of the world’s total (Figure 5I). Russia’s TCL area has grown by 53,918 km2 (40%) since 2001, especially after the reintroduction of tigers in the Pri-Amur region in 2014 (Ning et al., 2019; Rozhnov et al., 2021). This area represents the only major expansion of tigers into a new TCL, as opposed to the fragmentation of existing TCLs, over the last 20 years.
China has more effective potential habitat than any country on Earth, but most of that habitat lacks tigers (76% of its effective potential habitat is in Restoration Landscapes or Restoration Fragments; see Figure 5C). Over the last twenty years, the so-called South China tiger has been extirpated (Zhang et al., 2019) and its former habitat severely fragmented (Qin et al., 2015), though cumulatively much habitat still exists. Over the last two decades, China has seen some expansion of TCL area in Northeast China, which is part of larger transboundary areas with Russia (McLaughlin, 2016; Wang et al., 2016; Qi et al., 2021). A smaller area of occupied tiger habitat also exists in southern China, part of the larger Northern Triangle TCL, in southern Tibet (Wang et al., 2019). Together Chinese TCLs in 2020 stood at 100,368 km2, a remarkable increase of +77% over 2001 levels, representing significant conservation investment (Zhou et al., 2022). In 2020, these areas represent 10% of the world’s total.
Tigers persisted on the Korean peninsula until the 1950s (Seeley and Skabelund, 2015). The best remaining prospects are fragmented habitats in North Korea on the border with China and Russia, part of a transboundary TCL (Figure 5S; Noone, 2018). Very little habitat with low human footprint persists in South Korea now (Figure 5U).
3.2.4 Central Asia
Central Asia has no tigers currently, but three range states still have effective potential tiger habitat of landscape-scale dimensions: Georgia (Figure 5N), Kazakhstan (Figure 5P), and Pakistan (Figure 5T). The best possibilities seem to exist in Kazakhstan in the river deltas adjacent to Lake Balkhash, an area currently being investigated for the possibility of reintroduction (Driscoll et al., 2012; WWF Russia, 2022). Restoration fragments remain in Iran (Figure 5O), Kyrgyzstan (Figure 5Q), and Turkey (Figure 5V), but are dwindling in number and area rapidly. Similar situations attain in Armenia (Figure 5K) and Azerbaijan (Figure 5L), though in the latter case, tigers were still extant into the 1970s (Faizolahi, 2016). All tiger habitat, as well as tigers themselves, have been lost in Afghanistan, Turkmenistan, Tajikistan, Uzbekistan, and arid portions of western China. Historically there were small amounts of habitat within the modern boundaries of Syria and Iraq, the only memory of which lurks in the species’ English name, associated with former habitat along the Tigris River (Liddell and Scott, 2007).
3.2.5 Tiger tolerance for human activity
Finally, we detected important variations in the tolerance tigers have to human activity in different parts of the range (Figure S1). In South Asia, the social tolerance threshold was 18 on the human footprint’s 0 – 64 scale; in Southeast Asia, 8; and in Northeast Asia, 5. Differences in conservation efforts, infrastructure development, human-wildlife conflict, and culture seem to underlie these results (Carter et al., 2020; Jhala et al., 2021; Macdonald et al., 2022; Mukhacheva et al., 2022), but these results point to issues where more trans-national research is warranted.
4 Discussion
4.1 The global state of wild tiger habitat
For tigers living in the wild, the news is not all bad. Encouragingly, this iconic species no longer teeters on the brink of extinction; an estimated 4,485 (3,726–5,578) tigers (Goodrich et al., 2022) live in the wild across ten countries in approximately a million square kilometers of habitat in the 63 Tiger Conservation Landscapes mapped here (Table 2). Globally the loss of structural habitat has been largely arrested, albeit at historically low overall amounts (~40% of the likely resident indigenous range) (Figure 4A). Similarly, effective potential habitat now stands at only 16% of the indigenous range. Most encouragingly, metrics of occupied tiger habitat (as measured by TCL area) have declined only 11% in a two-decade period when Asia’s population grew 14% to 2.36 billion people, and the East Asian & Pacific economy grew 248% to 27.13 trillion US dollars (World Bank, 2023). Observed TCL areas fluctuated year-by-year (Figure 4B), largely as a function of the available knowledge base. Improved information may show less loss in the future. Taken together, our results suggest that tigers may have reached the bottom of the species recovery curve (Moorcroft, 2017). Tiger habitat is less tiger-filled and more fragmented than at any time in the last 200 years, but perhaps now the world is poised for a recovery in tiger populations not seen in generations.
This relatively optimistic reading of the trends at the global scale (Figure 4) should not disguise the fact that tigers continue to lose habitat in many places, with different trajectories of loss in different nation-states (Figure 5). The mechanisms of habitat loss at the grossest level are loss of vegetation (i.e. structural habitat) and expansion of human encroachment (i.e. reducing effective potential habitat). The expansion of linear infrastructure remains a concern for tiger conservation (Carter et al., 2020; Nayak et al., 2020). Concomitant declines in the prey base are probably also important but were not estimated here. (Modelling prey remains an important research topic.) During the last 20 years, tigers have become extinct in three Southeast Asian countries (Cambodia, Laos, and Vietnam) and across a large part of southeastern China. Tigers remain under pressure everywhere, which is especially acute in Southeast Asia. The tiger’s situation in vast parts of Myanmar remains unclear and is probably poor; ongoing conflict limits our ability to understand what is happening below the canopy. Human-wildlife conflict is frequent in Sumatra, leading often to harsh reprisals (Figel et al., 2021; Widodo et al., 2022; Patana et al., 2023). Malaysia’s tiger populations may have stabilized but at low levels, but how low remains unclear until the results of the recently finished country-level survey are released (Ding, 2022). Human influence appears much more harmful for tigers in Southeast Asia than in South Asia (Figure S1). Though important questions about the situation remain, the evidence presented here suggests that without stepped-up conservation efforts, further losses are likely. Meanwhile, TCLs appear to be expanding in India, Nepal, Bhutan, northern China, and southeastern Russia.
Survey Landscapes have declined dramatically in the last 20 years (Figure 4B). Greatly expanded survey efforts, largely associated with increased use of remotely triggered camera traps (Karanth et al., 2004), have greatly increased scientific knowledge, allowing us to determine which areas with suitable habitat no longer have tigers (hence becoming Restoration Landscapes) or do still retain tigers, in which case they were incorporated as TCLs. The decline in area of Survey Landscapes represents our collectively improved knowledge of tiger distributions: we now know much more, even though there are fewer places where tigers persist. But because scientists often focus on repeated camera trap monitoring to detect trends in the best-known protected areas, large swaths of connected landscapes remain unsurveyed: typically, less than 50% of TCL area has confirmed sightings from the last five years. Therefore, even though we can confirm that a TCL retains tigers, in few cases, can we clearly understand what proportion of a TCL holds tigers and which areas are still in need of conservation efforts to allow populations to recover. These issues could use more attention in future iterations of the model.
TCL 3.0 demonstrates that there is still abundant effective potential habitat in Restoration Landscapes; these areas just lack tigers (Gray et al., 2023). If these extant habitats could be made suitable again in terms of prey base and were tigers to re-establish via natural dispersal or through active reintroduction efforts, we could increase the land base for tigers by 50%. Two conservation objectives – ensuring tigers are distributed at carrying capacity across the entirety of TCLs, and that they are recovered across Restoration Landscapes, could more than double, perhaps even triple, the number of free-living tigers on Earth (Lynam, 2010; Wikramanayake et al., 2011; Harihar et al., 2018). Given the tiger’s resilience and changes in Asia’s social and political landscape (Sanderson et al., 2019), this long-sought goal could be met within a human generation, if not sooner.
Models are only as useful as the data put into them. This model depends on in situ biological observations: surveys, with dates and locations, measures of effort, and verifiable evidence of tiger occurrence. The system doesn’t function without this information. Discovering a previously unknown or recently established tiger population is the easiest way to turn a landscape from green (Survey) to orange (TCL). On-going, extensive surveys in existing TCLs and Survey Landscapes are needed continuously to maintain the system and make its results useful.
Restoration Landscapes provide a remarkable, and until now, mostly unrealized opportunity to increase the area of occupied tiger range. Our analyses suggest that suitable habitat without tigers may be more susceptible to degradation and fragmentation than tiger-inhabited landscapes, as demonstrated by our analysis of what happened to former TCLs in Vietnam, Cambodia and Laos after extirpation. These findings highlight the urgency to halt deforestation, increase the prey base, and provide connection to TCLs to encourage dispersal. In some cases, where connectivity to an existent tiger population is not possible (e.g. the Balkash delta of Kazakhstan. some forest blocks in Thailand), active reintroduction efforts will be required. The Kazakhstan efforts seem particularly important because, were efforts to succeed, those tigers would be the first to live in Central Asia in more than a half-century.
Finally, there is – over some very long time scale – the issue of recovering some of the 60% of tiger indigenous range that has been lost, by redressing the conversion of former tiger structural habitat to other land use classes. Socioeconomic change in Asia, which has nothing per se to do with tigers, may provide enormous benefits to recovering tiger habitat (Sanderson et al., 2018; Sanderson et al., 2019). Rural-to-urban migration, urbanization, poverty reduction, and improved education may eventually make much more tiger habitat available, if (and only if) tigers remain in the wild and are capable of re-establishing in these areas. This is largely a civilizational project, to be enacted not only passively but proactively as national governments, civil society, and the global community pivot toward the realization that we are not separate from the Earth’s nature, but part of it, dependent as the tiger is, on the value of natural ecosystems for providing water, food and fiber, and a moderate climate. The United Nations Sustainable Development Goals and the recent “30 by 30” agreement adopted at the Convention on Biological Diversity CoP15 in Montreal (Einhorn, 2022), represent positive steps, which, if implemented well, can serve tigers, people, and the rest of nature.
4.2 The state of tiger habitat mapping
Habitat maps and metrics are essential documents to plan species conservation efforts, reverse defaunation, and expand environmental protection amid the paired biodiversity and climate crises. National leaders must understand trends to make informed policy decisions and fulfil their international obligations (Table 3). State and provincial officials need tabulations tailored to their local jurisdictions, with attention to protected areas, human-wildlife conflict, sustainable use, and connectivity to adjacent jurisdictions (see act-green.org). Non-governmental organizations and donors may be interested in trends of habitat availability range-wide (Table 2) and in different ecologically defined areas (e.g. ecoregions, biomes), to inform ecological representation (Table 4), or within collections of countries where they work (Table 3). “On-the-ground”, landscape managers are most interested in the fine but pertinent details: Where is my landscape changing? How much habitat is available right now? Does the model match expectations or not? How does it relate to comparable areas?
Remarkably, existing technology can go a long way to serving all of these needs simultaneously. As described here, we have constructed not a map of tiger-relevant landscapes, but a system for mapping them. That system depends on clearly-defined inputs (an indigenous range map, species observations, a notion of what land cover are suitable for the species, and the human footprint) and delivers clear-defined outputs suitable for planning tiger conservation efforts at landscape, national, and range-wide scales. Because it is a mapping of the system, updates are possible by “simply” changing the inputs and re-running the process. Because it is a system when new inputs become available, for example, for 2021 or 2036, we can produce new, comparable maps and statistical outputs to measure progress.
One implication of a “mapping system” versus “a map” is that there is no one final map. Each new version is an improvement on the previous attempt, dependent on improvements in the accuracy and timeliness of the inputs, where within a few hours, a new observation can literally change the map for tigers. Versioning of results and linking back clearly to the inputs become even more important, even as we try to draw declarative statements about the status of tigers and trends in their habitat. This dynamic assessment system lies at the core of any adaptive management strategy.
A real constraint on improving understanding rests on the sharing of species observations. Conservationists and biologists have made huge gains in survey methods, repetition of tiger surveys, and documenting distribution in scientific papers and reports. But will people share the raw data they so laboriously collect? In this instance, despite a promising start with some commitments, enormous efforts and huge amounts of time were required to obtain the in situ biological observations required. After relentless emailing and Zoom calls failed to produce enough information, we resorted to a systematic literature search to re-capture and re-digitize information that sits on laptops across Asia and elsewhere. To develop a twenty-year analytical synthesis, we had to read every paper and report we could find published over the last 25 years and schedule dozens of follow-up conversations with individual researchers and research teams to cajole them into what should be the regular scholarly practice of sharing data for conservation, for example, using public Internet fora such as the Global Biodiversity Information Facility (Edwards, 2004; Ivanova and Shashkov, 2021) or Wildlife Insights (Ahumada et al., 2020). Many eventually complied and shared, including most of the co-authors of this paper (See also Table S1). One would expect scientific publishing to be one mechanism for better data sharing (Christensen et al., 2019). However, we found that the wide variety of ways that data are published in scientific papers and uneven review standards across journals ensure plenty of scope for researchers to dull the precision of the original data, hide or not report important details, and obfuscate the observations, even as authors claim to illuminate conservation issues. Not only are such abuses of the scientific literature process annoying, they ultimately delay decision-making, which is the ostensible reason for most studies in the first place.
Were such difficulties to be overcome, whether through ongoing, brute force literature searches or by better data sharing, then systems such as TCL 3.0 could help countries report on their commitments to the Sustainable Development Goal (SDG) agenda (United Nations Department of Economic and Social Affairs, 2022) and the Global Biodiversity Framework under the Convention for Biological Diversity (CBD Secretariat, 2022). Among other goals, SDG signatory countries have committed to Goal 15, Life on Land, which enjoins nations to protect, restore, and promote the sustainable use of terrestrial ecosystems, sustainably manage forests, combat desertification, and halt and reverse land degradation and halt biodiversity loss. TCL 3.0 enables countries to integrate ecosystem and biodiversity values into national and local planning processes, to understand how tiger conservation landscapes are protected and overlap with key biodiversity areas, and to halt forests loss since tigers are a species of forests in most parts of the range. Similarly, TCL 3.0 enables countries to measure the ecological integrity implied by and reinforced through long-lasting tiger populations.
Finally, we note the cultural aspects of technological innovations such as TCL 3.0 among conservationists. This project has depended on collaboration from across the tiger range, between governments and non-governmental organizations, and among the NGO sectors. Although tiger habitat has been much reduced, tigers live in too many places for any one actor or organization to successfully conserve them on its own. Rather technological tools such as this one, which depend on collaboration, also provide a framework to make the necessary collaborations desirable and rewarding. To this end, we have worked hard to link these results to other assessments, including the recently completed IUCN Red List assessment (Goodrich et al., 2022) and the ongoing IUCN Green Status assessment (Hunter et al., in prep.) for tigers. One important collateral product of TCL 3.0, which was not achieved through either of the previous versions, was a new range-wide vision for tigers written by and jointly endorsed by a coalition of conservation organizations (Coalition for Securing a Viable Future for the Tiger, 2022). The success metrics specifically prescribed under this shared vision ensure the continued application of the TCL 3.0 system into the future, which will reinforce the kinds of collaborations and mutual calls to action that tigers, and other wildlife, so richly deserve.
5 Conclusions
What have we learned about tiger conservation over the last two decades? Conservation works when we choose to make it so. Species, even dangerous ones such as the tiger, can thrive on the 21st century Earth, if well-conserved. Conservation is straightforward. Don’t cut down their habitat. Don’t stalk them, harass them, or kill them or their prey. Control poaching and extinguish the illegal trade in tiger bones and parts. Prevent conflicts with people and livestock wherever possible, and where and when not, then mitigate losses to forestall retaliation. Tigers need and have a right to move, pass over or under highways, find prey and mates, and raise their families undisturbed; therefore, connections need to be established between landscapes where tigers can hunt in suitable vegetation and roam free from persecution. Where possible, restore native vegetation that supports native prey and, therefore, set the stage for the recovery of tigers. Deploying these strategies has enabled some countries to stabilize, even grow, tiger habitats, as we show here, notably in China, Russia, India, Bhutan, and Nepal. Monitoring progress at a pace relevant to decision-making is key. If these countries can do it, so can others, especially if the world community bands together to support tiger range states with funds, encouragement, science, and a bit of technological magic.
Data availability statement
The open-source code for this project is available at https://github.com/SpeciesConservationLandscapes. Summaries of the results, Earth Engine assets, and shapefiles of the landscape data for each year are available at YWN0LWdyZWVuLm9yZw==. All other data in the study are available on request from the corresponding author.
Author contributions
ES, KF, and DM conceived the project and this paper. PP analyzed the vegetation height data. ES, DM, KF, JS, and CY developed the methods. KF engineered the task architecture. KF, NR, JS, CY, and DuS wrote the computer code. SR, ES, DM, and SC reached out to governments. PC, AD, DM, AH, TG, JG, ML, WM, AP, AR, EF, UB, CB-W and HR contributed observations, among others (see Table S1). CC, LR, JM, and ES conducted the literature survey and formatted the data. ES, LR, and DeS analyzed the landscape results. ES, DM, AH, and LR drafted the manuscript. All authors contributed to the article and approved the submitted version.
Funding
The author(s) declare financial support was received for the research, authorship, and/or publication of this article.
Acknowledgments
The authors gratefully acknowledge the following grantors: NASA’s Sustaining Living Systems in a Time of Climate Variability and Change (NASA Grant Number 80NSSC19K0201); and institutional funds from the Wildlife Conservation Society and Panthera. This product article has been peer reviewed and approved for publication consistent with USGS Fundamental Science Practices (https://pubs.usgs.gov/circ/1367/). Any use of trade, product, or firm names is for descriptive purposes only and does not imply endorsement by the U.S. Government. The authors also wish to express their thanks to Steve Gallo, Timothy G. O’Brien, Mari Alexander, and to the dedicated thousands of tiger researchers and conservationists working in the field.
Conflict of interest
The author DuS is employed by company SparkGeo.
The remaining authors declare that the research was conducted in the absence of any commercial or financial relationships that could be construed as a potential conflict of interest.
Publisher’s note
All claims expressed in this article are solely those of the authors and do not necessarily represent those of their affiliated organizations, or those of the publisher, the editors and the reviewers. Any product that may be evaluated in this article, or claim that may be made by its manufacturer, is not guaranteed or endorsed by the publisher.
Supplementary material
The Supplementary Material for this article can be found online at: https://www.frontiersin.org/articles/10.3389/fcosc.2023.1191280/full#supplementary-material
References
Adhiasto D. N., Exploitasia I., Giyanto, Fahlapie P., Johnsen P., Andriansyah M. I., et al. (2023). A criminal justice response to address the illegal trade of wildlife in Indonesia. Conserv. Lett. 16, e12937. doi: 10.1111/conl.12937
Adhikarimayum A. S., Gopi G. V. (2018). First photographic record of tiger presence at higher elevations of the Mishmi Hills in the Eastern Himalayan Biodiversity Hotspot, Arunachal Pradesh, India. J. Threatened Taxa 10 (13), Article 13. doi: 10.11609/jott.4381.10.13.12833-12836
Adyla M. N. N., Ikhwan Z., Zuhairi M., Ngah, Shukor M. N. (2016). Diversity and activity pattern of wildlife inhabiting catchment of Hulu Terengganu Hydroelectric Dam, Terengganu, Peninsular Malaysia. AIP Conf. Proc. 1784 (1), 060038. doi: 10.1063/1.4966876
Ahumada J. A., Fegraus E., Birch T., Flores N., Kays R., O’Brien T. G., et al. (2020). Wildlife insights: A platform to maximize the potential of camera trap and other passive sensor wildlife data for the planet. Environ. Conserv. 47 (1), 1–6. doi: 10.1017/S0376892919000298
Akçakaya H. R., Bennett E. L., Brooks T. M., Grace M. K., Heath A., Hedges S., et al. (2018). Quantifying species recovery and conservation success to develop an IUCN Green List of Species. Conserv. Biol. 32, 1128–1138. doi: 10.1111/cobi.13112
Anonymous (2021) Global Administrative Areas (GADM) (2021). Available at: https://gadm.org/index.html (Accessed November 18, 2022).
Ash E., Hallam C., Chanteap P., Zaneta K., Macdonald D. W., Rojanachinda W., et al. (2020). Estimating the density of a globally important tiger (Panthera tigris) population: Using simulations to evaluate survey design in Eastern Thailand. Biol. Conserv. 241, 108349. doi: 10.1016/j.biocon.2019.108349
Aylward M., Sagar V., Natesh M., Ramakrishnan U. (2022). How methodological changes have influenced our understanding of population structure in threatened species: insights from tiger populations across India. Philos. Trans. R. Soc. B 377 (1852), 20200418. doi: 10.1098/rstb.2020.0418
Bista D., Lama S. T., Shrestha J., Rumba Y. B., Weerman J., Thapa M., et al. (2021). First record of Bengal Tiger, Panthera tigris tigris Linnaeus 1758 (Felidae), in eastern Nepal. Check List 17 (5), 1249–1253. doi: 10.15560/17.5.1249
Biswas S., Bhatt S., Sarkar D., Talukdar G., Pandav B., Mondol S. (2020). Assessing tiger corridor functionality with landscape genetics and modelling across Terai-Arc Landscape, India [Preprint]. Ecology 23, 949–966. doi: 10.1101/2020.10.24.353789
Carter N., Killion A., Easter T., Brandt J., Ford A. (2020). Road development in Asia: Assessing the range-wide risks to tigers. Sci. Adv. 6 (18), eaaz9619. doi: 10.1126/sciadv.aaz9619
CBD Secretariat (2022) Convention on Biological Diversity. Available at: https://www.cbd.int/convention/ (Accessed November 16, 2022).
Chakma S. (2016). Assessment of large mammals of the Chittagong Hill Tracts of Bangladesh with emphasis on tiger (Panthera tigris) (Dhaka, Bangladesh: University of Dhaka).
Christensen G., Dafoe A., Miguel E., Moore D. A., Rose A. K. (2019). A study of the impact of data sharing on article citations using journal policies as a natural experiment. PloS One 14 (12), e0225883. doi: 10.1371/journal.pone.0225883
Coalition for Securing a Viable Future for the Tiger (2022) For the Year of the Tiger, a shared vision for the future of the iconic cat (commentary). Mongabay Environmental News. Available at: https://news.mongabay.com/2022/01/for-the-year-of-the-tiger-a-shared-vision-for-the-future-of-the-iconic-cat-commentary/.
Cooper D. M., Dugmore A. J., Gittings B. M., Scharf A. K., Wilting A., Kitchener A. C. (2016). Predicted Pleistocene–Holocene range shifts of the tiger (Panthera tigris). Diversity Distributions 22, 1199–1211. doi: 10.1111/ddi.12484
Creative Conservation Alliance (2016). A Preliminary Wildlife Survey in Sangu-Matamuhuri Reserve Forest, Chittagong Hill Tracts, Bangladesh (Creative Conservation Alliance).
Darimont C. T., Cooke R., Bourbonnais M. L., Bryan H. M., Carlson S. M., Estes J. A., et al. (2023). Humanity’s diverse predatory niche and its ecological consequences. Commun. Biol. 6 (1), 609. doi: 10.1038/s42003-023-04940-w
Di Marco M., Venter O., Possingham H. P., Watson J. E. M. (2018). Changes in human footprint drive changes in species extinction risk. Nat. Commun. 9 (1), 4621. doi: 10.1038/s41467-018-07049-5
Dinerstein E., Loucks C., Wikramanayake E., Ginsberg J., Sanderson E., Seidensticker J., et al. (2007). The fate of wild tigers. BioScience 57, 508–514. doi: 10.1641/B570608
Dinerstein E., Olson D., Joshi A., Vynne C., Burgess N. D., Wikramanayake E., et al. (2017). An ecoregion-based approach to protecting half the terrestrial realm. BioScience 67, 534–545. doi: 10.1093/biosci/bix014
Dinerstein E., Wikramanayake E., Robinson J. G., Karanth K. U., Rabinowitz A. R., Olson D. M., et al. (1997) A Framework for Identifying High Priority Areas and Actions for the Conservation of Tigers in the Wild (Washington D.C: World Wildlife Fund-US, Wildlife Conservation Society, National Fish and Wildlife Foundation’s Save the Tiger Fund). Available at: https://www.panthera.org/sites/default/files/1995-0166-012_PART1.pdf (Accessed March 6, 2015).
Ding E. (2022). Is there still a chance to save the Malayan tiger? (Doha, Qatar: Al Jazerra). Available at: https://www.aljazeera.com/news/2022/3/7/Malaysia-last-chance-to-save-the-malayan-tiger.
Dirzo R., Young H. S., Galetti M., Ceballos G., Isaac N. J. B., Collen B. (2014). Defaunation in the anthropocene. Science 345, 401–406. doi: 10.1126/science.1251817
Dou H., Yang H., Smith J. L. D., Feng L., Wang T., Ge J. (2019). Prey selection of Amur tigers in relation to the spatiotemporal overlap with prey across the Sino–Russian border. Wildlife Biol. 2019 (1), Article 1. doi: 10.2981/wlb.00508
Driscoll C. A., Chestin I., Jungius H., Pereladova O., Darman Y., Dinerstein E., et al. (2012). A postulate for tiger recovery: the case of the Caspian Tiger. J. Threatened Taxa 4 (6), 2637–2643. doi: 10.11609/JoTT.o2993.2637-43
Edwards J. L. (2004). Research and societal benefits of the global biodiversity information facility. BioScience 54 (6), 485–486. doi: 10.1641/0006-3568(2004)054[0486:RASBOT]2.0.CO;2
Einhorn C. (2022). Nearly Every Country Signs On to a Sweeping Deal to Protect Nature (New York: The New York Times). Available at: https://www.nytimes.com/2022/12/19/climate/biodiversity-cop15-montreal-30x30.html.
Emer C., Jordano P., Pizo M. A., Ribeiro M. C., da Silva F. R., Galetti M. (2020). Seed dispersal networks in tropical forest fragments: Area effects, remnant species, and interaction diversity. Biotropica 52 (1), 81–89. doi: 10.1111/btp.12738
European Space Agency (2017) Land Cover CCI Product User Guide Version 2.0. Available at: https://maps.elie.ucl.ac.be/CCI/viewer/download/ESACCI-LC-Ph2-PUGv2_2.0.pdf.
Fahrig L. (2019). Habitat fragmentation: A long and tangled tale. Global Ecol. Biogeogr. 28, 33–41. doi: 10.1111/geb.12839
Faizolahi K. (2016). Tiger in Iran—Historical distribution, extinction causes and feasibility of reintroduction. Cat News 10, 5–12. URL: http://www.catsg.org/fileadmin/filesharing/5.Cat_News/5.3._Special_Issues/5.3.9._SI_10/Faizolahi_2016_Tiger_in_Iran.pdf.
Fernández N., Ferrier S., Navarro L. M., Pereira H. M. (2020). “Essential Biodiversity Variables: Integrating In-Situ Observations and Remote Sensing Through Modeling,” in Remote Sensing of Plant Biodiversity. Eds. Cavender-Bares J., Gamon J. A., Townsend & P.A., Springer, New York, 485–501. doi: 10.1007/978-3-030-33157-3_18
Figel J. J., Hambal M., Krisna I., Putra R., Yansyah D. (2021). Malignant snare traps threaten an irreplaceable megafauna community. Trop. Conserv. Sci. 14, 194008292198918. doi: 10.1177/1940082921989187
Forrest J. L., Bomhard B., Budiman A., Coad L., Cox N., Dinerstein E., et al. (2011). Single-species conservation in a multiple-use landscape: current protection of the tiger range. Anim. Conserv. 14, 283–294. doi: 10.1111/j.1469-1795.2010.00428.x
Ganguli-Lachungpa U. (1998). On the occurrence of the tiger Panthera tigris in Sikkim. J. Bombay Natural History Society 95 (1), 109–110. URL: https://archive.org/details/biostor-152548/page/n1/mode/2up
Ghazali A. N., Meisery A., Adam L., Hasnan M. H. S., Yazi M. F., Patah P. A., et al. (2019). Wildlife monitoring at Labis Timur Ecological Corridor (CFS2: PL1) in Johor, Malaysia. J. Wildlife Parks 34, 2. URL: https://jwp.wildlife.gov.my/index.php/jwp/article/view/4
Gittleman J. L., Harvey P. H. (1982). Carnivore home-range size, metabolic needs and ecology. Behav. Ecol. Sociobiol. 10, 57–63. doi: 10.1007/BF00296396
Global Tiger Initiative Secretariat (2012). Managing Tiger Conservation Landscapes and Habitat Connectivity: Threats and Possible Solutions (Washington, DC: The World Bank), 14–29.
Goodrich J., Wisibono H., Miquelle D., Lynam A., Sanderson E., Chapman S., et al. (2022). IUCN Red List Assessment of the Tiger (Panthera tigris) (International Union for the Conservation of Nature).
Google (2022) Google Earth Engine. Available at: https://earthengine.google.com (Accessed November 16, 2022).
Gorelick N., Hancher M., Dixon M., Ilyushchenko S., Thau D., Moore R. (2017). Google Earth Engine: Planetary-scale geospatial analysis for everyone. Remote Sens. Environ. 202, 18–27. doi: 10.1016/j.rse.2017.06.031
Gray T. N. E., Baltzer M. C., Gopal R., Seng T. (2017). Editorial—Not yet an obituary for Cambodia’s tigers. Cambodian J. Natural History 2, 137–139. URL: https://www.gardeningeye.com/download/201712_Cambodian-Journal-of-Natural-History.pdf#page=3
Gray T. N. E., Hughes A. C., Laurance W. F., Long B., Lynam A. J., O’Kelly H., et al. (2018). The wildlife snaring crisis: An insidious and pervasive threat to biodiversity in Southeast Asia. Biodiver. Conserv. 27 (4), 1031–1037. doi: 10.1007/s10531-017-1450-5
Gray T. N., Rosenbaum R., Jiang G., Izquierdo P., Yongchao J., Kesaro L., et al. (2023). Restoring Asia’s roar: Opportunities for tiger recovery across the historic range. Front. Conserv. Sci. 4. doi: 10.3389/fcosc.2023.1124340
Guisan A., Thuiller W., Zimmermann N. E. (2017). Habitat Suitability and Distribution Models: With Applications in R. 1st edition (Cambridge University Press).
Gulati S., Karanth K. K., Le N. A., Noack F. (2021). Human casualties are the dominant cost of human–wildlife conflict in India. Proc. Natl. Acad. Sci. 118, e1921338118. doi: 10.1073/pnas.1921338118
Gumal M., Abu Bakar M., Mohd Nawayai Y., Horng L. S., Lee B. P. Y., Chee Pheng L., et al. (2014). Small-medium wild cats of Endau Rompin landscape in Johor, Peninsular Malaysia. Cat News 8, 10–18. URL: https://www.researchgate.net/publication/264087117_Small-medium_wild_cats_of_Endau_Rompin_Landscape_in_Johor_Peninsular_Malaysia
Hall L. S., Krausman P. R., Morrison M. L. (1997). The Habitat concept and a plea for standard terminology. Wildlife Soc. Bull. 25, 173–182. URL: https://api.semanticscholar.org/CorpusID:19875449
Harihar A., Ghosh-Harihar M., MacMillan D. C. (2018). Losing time for the tiger Panthera tigris: Delayed action puts a globally threatened species at risk of local extinction. Oryx 52 (1), 78–88. doi: 10.1017/S0030605317001156
Harrison R. D. (2011). Emptying the forest: hunting and the extirpation of wildlife from tropical nature reserves. BioScience 61 (11), 919–924. doi: 10.1525/bio.2011.61.11.11
Hossain A. N. M., Lynam A. J., Ngoprasert D., Barlow A., Barlow C. G., Savini T. (2018). Identifying landscape factors affecting tiger decline in the Bangladesh Sundarbans. Global Ecol. Conserv. 13, e00382. doi: 10.1016/j.gecco.2018.e00382
IPBES (2019). Global assessment report on biodiversity and ecosystem services of the Intergovernmental Science-Policy Platform on Biodiversity and Ecosystem Services. Eds. Brondizio E. S., Settele J., Díaz S., Ngo H. T. (Bonn, Germany: IPBES secretariat), 1148. doi: 10.5281/zenodo.3831673.
IUCN (2016). A Global Standard for the identification of Key Biodiversity Areas, version 1.0 (International Union for the Conservation of Nature). Available at: https://portals.iucn.org/union/sites/union/files/doc/a_global_standard_for_the_identification_of_key_biodiversity_areas_final_web.pdf.
Ivanova N. V., Shashkov M. P. (2021). The possibilities of GBIF data use in ecological research. Russian J. Ecol. 52 (1), 1–8. doi: 10.1134/S1067413621010069
Jhala Y. V., Dey T. K., Qureshi Q., Kabir M. J., Borah J., Roy M. (2016). Status of tigers in the sundarban landscape Bangladesh and India (Bangladesh Forest Department; National Tiger Conservation Authority; Wildlife Institute of India), 66.
Jhala Y., Gopal R., Mathur V., Ghosh P., Negi H. S., Narain S., et al. (2021). Recovery of tigers in India: Critical introspection and potential lessons. People Nat. 3 (2), 281–293. doi: 10.1002/pan3.10177
Jhala Y. V., Gopal R., Qurushi R. (2008). Status of Tigers, Co-predators, and Prey in India (National Tiger Conservation Authority and Wildlife Institute of India).
Jhala Y. V., Qureshi Q., Gopal R. (2015). Status of tigers, copredators & prey in India 2014 (National Tiger Conservation Authority and Wildlife Institute of India).
Jhala Y. V., Qureshi Q., Gopal R., Sinha P. R. (2011). Status of tigers, co-predators and prey in India 2010 (National Tiger Conservation Authority, Govt. of India and Wildlife Institute of India).
Jhala Y. V., Qurushi R., Nayak A. K. (2018). Status of Tigers, Co-predators, and Prey 2018 (Summary Report. National Tiger Conservation Authority, Government of India, New Delhi & Wildlife Institute of India, Dehradun).
Johnson A., Vongkhamheng C., Hedemark M., Saithongdam T. (2006). Effects of human-carnivore conflict on tiger (Panthera tigris) and prey populations in Lao PDR. Anim. Conserv. 9 (4), 421. doi: 10.1111/j.1469-1795.2006.00049.x
Jones G. M., Shirk A. J., Yang Z., Davis R. J., Ganey J. L., Gutiérrez R. J., et al. (2022). Spatial and temporal dynamics of Mexican spotted owl habitat in the southwestern US. Landscape Ecol. 38, 23–37. doi: 10.1007/s10980-022-01418-8
Jornburom P., Duangchantrasiri S., Jinamoy S., Pattanavibool A., Hines J. E., Arnold T. W., et al. (2020). Habitat use by tiger prey in Thailand’s Western Forest Complex: What will it take to fill a half-full tiger landscape? J. Nat. Conserv. 58, 125896. doi: 10.1016/j.jnc.2020.125896
Joshi A. R., Dinerstein E., Wikramanayake E., Anderson M. L., Olson D., Jones B. S., et al. (2016). Tracking changes and preventing loss in critical tiger habitat. Sci. Adv. 2, e1501675. doi: 10.1126/sciadv.1501675
Karanth K. U., Gopal R. (2005). “An ecology-based policy framework for human–tiger coexistence in India,” in People and Wildlife, Conflict or Co-existence? Eds. Woodroffe R., Thirgood S., Rabinowitz &A. (New York: Cambridge University Press), 373–387.
Karanth K. U., Kumar N. S., Karanth K. K. (2020). Tigers against the odds: Applying macro-ecology to species recovery in India. Biol. Conserv. 252, 108846. doi: 10.1016/j.biocon.2020.108846
Karanth K. U., Nichols J. D., Kumar N. S., Link W. A., Hines J. E. (2004). Tigers and their prey: Predicting carnivore densities from prey abundance. Proc. Natl. Acad. Sci. 101, 4854–4858. doi: 10.1073/pnas.0306210101
Karanth K. U., Sunquist M. E. (1992). Population structure, density and biomass of large herbivores in the tropical forests of Nagarahole, India. J. Trop. Ecol. 8, 21–35. doi: 10.1017/S0266467400006040
Kawanishi K. (2020). Populaton Status of Tigers (Panthera tigris) in a primary rainforest of Peninsular Malaysia (Gainesville, FL, USA: University of Florida).
KBA Partnership (2023) Key Biodiversity Areas. Available at: https://www.keybiodiversityareas.org.
Lamichhane B. R., Pokheral C. P., Poudel S., Adhikari D., Giri S. R., Bhattarai S., et al. (2018). Rapid recovery of tigers Panthera tigris in Parsa Wildlife Reserve, Nepal. Oryx 52 (1), 16–24. doi: 10.1017/S0030605317000886
Leisher C., Robinson N., Brown M., Kujirakwinja D., Schmitz M. C., Wieland M., et al. (2022). Ranking the direct threats to biodiversity in sub-Saharan Africa. Biodiver. Conserv. 31, 1329–1343. doi: 10.1007/s10531-022-02394-w
Liddell H. G., Scott R. (2007). Liddell and Scott’s Greek-English Lexicon (Oxford: Simon Wallenburg Press).
Linkie M., Martyr D., Harihar A., Mardiah S., Hodgetts T., Risdianto D., et al. (2018). Asia’s economic growth and its impact on Indonesia’s tigers. Biol. Conserv. 219, 105–109. doi: 10.1016/j.biocon.2018.01.011
Lubis M. I., Pusparini W., Prabowo S. A., Marthy W., Tarmizi, Andayani N., et al. (2020). Unraveling the complexity of human–tiger conflicts in the Leuser Ecosystem, Sumatra. Anim. Conserv. 23, 741–749. doi: 10.1111/acv.12591
Luskin M. S., Albert W. R., Tobler M. W. (2017). Sumatran tiger survival threatened by deforestation despite increasing densities in parks. Nat. Commun. 8 (1), 1783. doi: 10.1038/s41467-017-01656-4
Lwin Y. H., Wang L., Li G., Maung K. W., Swa K., Quan R.-C. (2021). Diversity, distribution and conservation of large mammals in northern Myanmar. Global Ecol. Conserv. 29, e01736. doi: 10.1016/j.gecco.2021.e01736
Lynam A. J. (2010). Securing a future for wild Indochinese tigers: Transforming tiger vacuums into tiger source sites. Integr. Zool. 5 (4), 324–334. doi: 10.1111/j.1749-4877.2010.00220.x
Macdonald D. W., Johnson P. J., Burnham D., Dickman A., Hinks A., Sillero-Zubiri C., et al. (2022). Understanding nuanced preferences for carnivore conservation: To know them is not always to love them. Global Ecol. Conserv. 37, e02150. doi: 10.1016/j.gecco.2022.e02150
Mahmood T., Vu T. T., Campos-Arceiz A., Akrim F., Andleeb S., Farooq M., et al. (2021). Historical and current distribution ranges and loss of mega-herbivores and carnivores of Asia. PeerJ 9, e10738. doi: 10.7717/peerj.10738
Mandala V. R. (2018). Shooting a Tiger: Big-Game Hunting and Conservation in Colonial India (New York: Oxford University Press). doi: 10.1093/oso/9780199489381.001.0001
Marthy W. (2021). Unpublished summary of media accounts of human-wildlife conflict involving tigers on Sumatra 2017—2021 (Indonesia: Wildlife Conservation Society).
Mazák J. H., Christiansen P., Kitchener A. C. (2011). Oldest known pantherine skull and evolution of the tiger. PloS One 6, e25483. doi: 10.1371/journal.pone.0025483
Microsoft (2022) Microsoft planetary computer. Available at: https://planetarycomputer.microsoft.com/ (Accessed November 16, 2022).
Ministry of Natural Resources and the Environment (2020). Myanmar National Tiger Action Plan (Yangon, Myanmar: Government of Myanmar).
Miquelle D. G., Merrill T. W., Dunishenko Y. M., Smirnov E. N., Quigley H. B., Pikunov D. G., et al. (1999a). “A habitat protection plan for the Amur tiger: developing political and ecological criteria for a viable land-use plan.” In Riding the tiger; Meeting the needs of people and wildlife in Asia. Eds. Seidensticker J., Christie S., Jackson P. (Cambridge: Cambridge University Press), 273–295.
Miquelle D., Quigley H., Smirnov E. N., Merrill T., Myslenko A. E., Hornocker M. G. (1999b). “Hierarchical spatial analysis of Amur Tiger relationships to habitat and prey.” in Riding the tiger; Meeting the needs of people and wildlife in Asia. Eds. Seidensticker J., Christie S., Jackson P. (Cambridge: Cambridge University Press), 71–99.
Miquelle D. G., Smirnov E. N., Zaumyslova O. Y., Soutyrina S. V., Johnson D. H. (2015). Population dynamics of Amur tigers (Panthera tigris altaica) in Sikhote-Alin Biosphere Zapovednik: 1966–2012. Integr. Zool. 10, 315–328. doi: 10.1111/1749-4877.12141
Moorcroft D. (2017). Achieving sustainable species recovery: Lessons from the Stone-curlew LIFE project: RSPB EU LIFE+ end of project international conference (Cambridge, UK: Royal Society for the Protection of Birds). Available at: https://www.rspb.org.uk/globalassets/downloads/documents/conservation-projects/stone-curlew-project-conference-report.pdf.
Moreno-Mateos D., Alberdi A., Morriën E., van der Putten W. H., Rodríguez-Uña A., Montoya D. (2020). The long-term restoration of ecosystem complexity. Nat. Ecol. Evol. 4, 676–685. doi: 10.1038/s41559-020-1154-1
Moy J. (2021) Standard error or confidence intervals. Available at: https://gist.github.com/jessetmoy/a6c44721575161f39ecf18d92ba85e77.
Mukhacheva A. S., Bragina E. V., Miquelle D. G., Kretser H. E., Derugina V. V. (2022). Local Attitudes Toward Amur Tiger (Panthera tigris altaica) Conservation in the Russian Far East. Conserv. Soc. 20 (4), 304–312. doi: 10.4103/cs.cs_20_135
Mukul S. A., Alamgir M., Sohel Md. S.I., Pert P. L., Herbohn J., Turton S. M., et al. (2019). Combined effects of climate change and sea-level rise project dramatic habitat loss of the globally endangered Bengal tiger in the Bangladesh Sundarbans. Sci. Total Environ. 663, 830–840. doi: 10.1016/j.scitotenv.2019.01.383
Musavi A., Khan J. A., Kumar S., Khan A., Malik P. K., Kushwaha S., et al. (2006). A study of Tiger human conflict in buffer zone of the Corbett Tiger Reserve: Protected area-people relationship. Int. J. Ecol. Environ. Sci. 32, 241–257. URL: https://www.researchgate.net/publication/289025639_A_study_of_Tiger_human_conflict_in_buffer_zone_of_the_Corbett_Tiger_Reserve_Protected_area-people_relationship
Nayak R., Karanth K. K., Dutta T., Defries R., Karanth K. U., Vaidyanathan S. (2020). Bits and pieces: Forest fragmentation by linear intrusions in India. Land Use Policy 99, 104619. doi: 10.1016/j.landusepol.2020.104619
Nichols J. D., Bailey L. L., O’Connell A., Talancy N. W., Campbell Grant E. H., Gilbert A. T., et al. (2008). Multi-scale occupancy estimation and modelling using multiple detection methods. J. Appl. Ecol. 45, 1321–1329. doi: 10.1111/j.1365-2664.2008.01509.x
Nijhawan S., Mihu A. (2020). Relations of blood: hunting taboos and wildlife conservation in the idu mishmi of northeast India. J. Ethnobiol. 40 (2), 149–166. doi: 10.2993/0278-0771-40.2.149
Nikulin M. S. (1973). “Chi-squared test for norMality,” in Proceedings of the International Vilnius Conference on Probability Theory and Mathematics, International Vilnius Conference, Vilnius, Lithuania Vol. 2. 119–122.
Ning Y., Kostyria A. V., Ma J., Chayka M. I., Guskov V. Y., Qi J., et al. (2019). Dispersal of Amur tiger from spatial distribution and genetics within the eastern Changbai mountain of China. Ecol. Evol. 9 (5), 2415–2424. doi: 10.1002/ece3.4832
Noone G. (2018). On the hunt for North Korea’s last Siberian tigers (Wilmington, DE, USA: NK News - North Korea News). Available at: https://www.nknews.org/2018/01/on-the-hunt-for-north-koreas-last-siberian-tigers/.
Nyhus P. J., Tilson R. (2004). Characterizing human-tiger conflict in Sumatra, Indonesia: Implications for conservation. Oryx 38 (1), 68–74. doi: 10.1017/S0030605304000110
O’Kelly H. J., Evans T. D., Stokes E. J., Clements T. J., Dara A., Gately M., et al. (2012). Identifying conservation successes, failures and future opportunities; assessing recovery potential of wild ungulates and tigers in eastern Cambodia. PloS One 7 (10), e40482. doi: 10.1371/journal.pone.0040482
Patana P., Alikodra H. S., Mawengkang H., Harahap R. H. (2023). State of human tiger conflict around Gunung Leuser National Park in Langkat Landscape, North Sumatra, Indonesia. Biodiversitas J. Biol. Diversity 24 (2), Article 2. doi: 10.13057/biodiv/d240220
Pettorelli N., Laurance W. F., O’Brien T. G., Wegmann M., Nagendra H., Turner W. (2014). Satellite remote sensing for applied ecologists: Opportunities and challenges. J. Appl. Ecol. 51 (4), 839–848. doi: 10.1111/1365-2664.12261
Phumanee W., Steinmetz R., Phoonjampa R., Weingdow S., Phokamanee S., Bhumpakphan N., et al. (2021). Tiger density, movements, and immigration outside of a tiger source site in Thailand. Conserv. Sci. Pract. 3 (12), e560. doi: 10.1111/csp2.560
Pikunov D. G. (2014). Population and habitat of the amur tiger in the Russian far east. Achievements Life Sci. 8 (2), 145–149. doi: 10.1016/j.als.2015.04.004
Plummer M. (2003). “JAGS: A program for analysis of Bayesian graphical models using Gibbs sampling,” in Proceedings of the 3rd International Workshop on Distributed Statistical Computing (DSC 2003). 3rd International Workshop on Distributed Statistical Computing, Vienna, Austria. 20–22.
Poor E. E., Shao Y., Kelly M. J. (2019). Mapping and predicting forest loss in a Sumatran tiger landscape from 2002 to 2050. J. Environ. Manage. 231, 397–404. doi: 10.1016/j.jenvman.2018.10.065
Potapov P., Tyukavina A., Turubanova S., Talero Y., Hernandez-Serna A., Hansen M. C., et al. (2019). Annual continuous fields of woody vegetation structure in the Lower Mekong region from 2000-2017 Landsat time-series. Remote Sens. Environ. 232, 111278. doi: 10.1016/j.rse.2019.111278
Powers R. P., Jetz W. (2019). Global habitat loss and extinction risk of terrestrial vertebrates under future land-use-change scenarios. Nat. Climate Change 9, 323–329. doi: 10.1038/s41558-019-0406-z
Pusparini W., Batubara T., Surahmat F., Ardiantiono, Sugiharti T., Muslich M., et al. (2018). A pathway to recovery: The Critically Endangered Sumatran tiger Panthera tigris sumatrae in an ‘in danger’ UNESCO World Heritage Site. Oryx 52 (1), 25–34. doi: 10.1017/S0030605317001144
Python Software Foundation (2022) Python Language Reference, version 3. Available at: http://www.python.org.
Qi J., Gu J., Ning Y., Miquelle D. G., Holyoak M., Wen D., et al. (2021). Integrated assessments call for establishing a sustainable meta-population of Amur tigers in northeast Asia. Biol. Conserv. 261, 109250. doi: 10.1016/j.biocon.2021.109250
Qin Y., Nyhus P. J., Larson C. L., Carroll C. J. W., Muntifering J., Dahmer T. D., et al. (2015). An assessment of South China tiger reintroduction potential in Hupingshan and Houhe National Nature Reserves, China. Biol. Conserv. 182, 72–86. doi: 10.1016/j.biocon.2014.10.036
Rao M., Htun S., Zaw T., Myint T. (2010). Hunting, livelihoods and declining wildlife in the hponkanrazi wildlife sanctuary, North Myanmar. Environ. Manage. 46 (2), 143–153. doi: 10.1007/s00267-010-9519-x
Rasphone A., Kéry M., Kamler J. F., Macdonald D. W. (2019). Documenting the demise of tiger and leopard, and the status of other carnivores and prey, in Lao PDR’s most prized protected area: Nam Et - Phou Louey. Global Ecol. Conserv. 20, e00766. doi: 10.1016/j.gecco.2019.e00766
Rayan D. M., Linkie M. (2016). Managing conservation flagship species in competition: Tiger, leopard and dhole in Malaysia. Biol. Conserv. 204 (B), 360–366. doi: 10.1016/j.biocon.2016.11.009
Reddy C. S., Faseela V. S., Unnikrishnan A., Jha C. S. (2019). Earth observation data for assessing biodiversity conservation priorities in South Asia. Biodiver. Conserv. 28, 2197–2219. doi: 10.1007/s10531-018-1681-0
Risdianto D., Martyr D. J., Nugraha R. T., Harihar A., Wibisono H. T., Haidir I. A., et al. (2016). Examining the shifting patterns of poaching from a long-term law enforcement intervention in Sumatra. Biol. Conserv. 204, 306–312. doi: 10.1016/j.biocon.2016.10.029
Rozhnov V. V., Naidenko S. V., Hernandez-Blaco J. A., Chistopolova M. D., Sorokin P. A., Yachmennikova A. A., et al. (2021). Restoration of the Amur Tiger (Panthera tigris altaica) Population in the Northwest of Its Distribution Area. Biol. Bull. 48 (8), 1401–1423. doi: 10.1134/S1062359021080239
Sabu M. M., Pasha S. V., Reddy C. S., Singh R., Jaishanker R. (2022). The effectiveness of Tiger Conservation Landscapes in decreasing deforestation in South Asia: A remote sensing-based study. Spatial Inf. Res. 30 (1), 63–75. doi: 10.1007/s41324-021-00411-8
Sanderson E. W., Fisher K., Robinson N., Sampson D., Duncan A., Royte L. (2022) The March of the Human Footprint. Available at: https://ecoevorxiv.org/repository/view/3641/.
Sanderson E. W., Forrest J., Loucks C., Ginsberg J., Dinerstein E., Seidensticker J., et al. (2010). “Setting priorities for tiger conservation: 2005–2015,” in Tigers of the World: The Science, Politics, and Conservation of Panthera Tigris. Eds. Tilson R., Nyhus P. (Boston: William Andrew Publishing), 143–161.
Sanderson E. W., Forrest J., Loucks C., Ginsberg J., Dinerstein E., Seidensticker J., et al. (2006). Setting priorities for the conservation and recovery of wild tigers: 2005-2015. The Technical Assessment (US, Washington, D.C: National Fish and Wildlife Foundation - Save the Tiger Fund, Wildlife Conservation Society and World Wildlife Fund).
Sanderson E. W., Jaiteh M., Levy M. A., Redford K. H., Wannebo A. V., Woolmer G. (2002). The human footprint and the last of the wild. BioScience 52 (10), 891–904. doi: 10.1641/0006-3568(2002)052[0891:THFATL]2.0.CO;2
Sanderson, Miquelle, Harihar, Breitenmoser, Breitenmoser-Wursten, Cooper, et al. (in press). Mapping the indigenous range of the tiger (Panthera tigris). Diversity Distributions.
Sanderson E. W., Moy J., Rose C., Fisher K., Jones B., Balk D., et al. (2019). Implications of the shared socioeconomic pathways for tiger (Panthera tigris) conservation. Biol. Conserv. 231, 13–23. doi: 10.1016/j.biocon.2018.12.017
Sanderson E. W., Walston J., Robinson J. G. (2018). From bottleneck to breakthrough: urbanization and the future of biodiversity conservation. BioScience 68 (6), 412–426. doi: 10.1093/biosci/biy039
Sarkar M. S., Amonge D. E., Pradhan N., Naing H., Huang Z., Lodhi M. S. (2021). A review of two decades of conservation efforts on tigers, co-predators and prey at the junction of three global biodiversity hotspots in the transboundary far-eastern himalayan landscape. Animals 11 (8), Article 8. doi: 10.3390/ani11082365
Seeley J., Skabelund A. (2015). Tigers—Real and imagined—In korea’s physical and cultural landscape. Environ. History 20 (3), 475–503. doi: 10.1093/envhis/emv079
Seryodkin I. V., Miquelle D. G., Goodrich J. M., Kostryia A. V., Petrunenko Y. K. (2017). Interspecific Relationships between the Amur Tiger (Panthera tigris altaica) and the Brown (Ursus arctos) and Asiatic Black Bear (Ursus thibetanus). Zoologicheskii Zhurnal 96 (12), 1446–1458. doi: 10.7868/S0044513417120029
Shirk A. J., Jones G. M., Yang Z., Davis R. J., Ganey J. L., Gutiérrez R. J., et al. (2022). Automated habitat monitoring systems linked to adaptive management: a new paradigm for species conservation in an era of rapid environmental change. Landscape Ecol. 38, 7–22. doi: 10.1007/s10980-022-01457-1
Shrestha S., Sherpa A. P., Lama S., Tamang M., Paudel P. K. (2021). Tigers at higher elevations outside their range: What does it mean for conservation? Eco.Mont (Journal Protected Mountain Areas Research) 14 (1), 43–46. doi: 10.1553/eco.mont-14-1s43
Skidmore A. (2021). Using crime script analysis to elucidate the details of Amur tiger poaching in the Russian Far East. Crime Sci. 10, 16. doi: 10.1186/s40163-021-00150-z
Smith J. L. D. (1993). The role of dispersal in structuring the chitwan tiger population. Behaviour 124, 165–195. doi: 10.1163/156853993X00560
Smith J. L. D., Ahearn S. C., McDougal C. (1998). Landscape analysis of tiger distribution and habitat quality in Nepal. Conserv. Biol. 12, 1338–1346. doi: 10.1111/j.1523-1739.1998.97068.x
Soh Y. H., Carrasco L. R., Miquelle D. G., Jiang J., Yang J., Stokes E. J., et al. (2014). Spatial correlates of livestock depredation by Amur tigers in Hunchun, China: Relevance of prey density and implications for protected area management. Biol. Conserv. 169, 117–127. doi: 10.1016/j.biocon.2013.10.011
Staude I. R., Navarro L. M., Pereira H. M. (2020). Range size predicts the risk of local extinction from habitat loss. Global Ecol. Biogeogr. 29 (1), 16–25. doi: 10.1111/geb.13003
Stephenson P. J., Grace M. K., Akçakaya H. R., Rodrigues A. S. L., Long B., Mallon D. P., et al. (2019). Defining the indigenous ranges of species to account for geographic and taxonomic variation in the history of human impacts: reply to Sanderson 2019. Conserv. Biol. 33, 1211–1213. doi: 10.1111/cobi.13400
Sukmasuang R., Charaspet K., Panganta T., Pla-ard M., Khioesree N., Thongbanthum J. (2020). Diversity, abundance, activity period, and factors affecting the appearance of wildlife around the corridors between Khao Yai-Thap Lan National Parks, Thailand by camera trapping. Biodiversitas 21 (5), 1. doi: 10.13057/biodiv/d210563
Sunarto S., Kelly M. J., Parakkasi K., Hutajulu M. B. (2015). Cat coexistence in central Sumatra: Ecological characteristics, spatial and temporal overlap, and implications for management. J. Zool. 296 (2), 104–115. doi: 10.1111/jzo.12218
Sunarto S., Kelly M., Parakkasi K., Klenzendorf S., Septayuda E., Kurniawan H. (2012). Tigers need cover: multi-scale occupancy study of the big cat in sumatran forest and plantation landscapes. PloS One 7, e30859. doi: 10.1371/journal.pone.0030859
Suttidate N., Steinmetz R., Lynam A. J., Sukmasuang R., Ngoprasert D., Chutipong W., et al. (2021). Habitat connectivity for endangered Indochinese tigers in Thailand. Global Ecol. Conserv. 29, e01718. doi: 10.1016/j.gecco.2021.e01718
Ten D. C. Y., Jani R., Hashim N. H., Saaban S., Abu Hashim A. K., Abdullah M. T. (2021). Panthera tigris jacksoni Population Crash and Impending Extinction due to Environmental Perturbation and Human-Wildlife Conflict. Animals 11 (4), 1032. doi: 10.3390/ani11041032
Thapa K., Malla S., Subba S. A., Thapa G. J., Lamichhane B. R., Subedi N., et al. (2021). On the tiger trails: Leopard occupancy decline and leopard interaction with tigers in the forested habitat across the Terai Arc Landscape of Nepal. Global Ecol. Conserv. 25, e01412. doi: 10.1016/j.gecco.2020.e01412
Thinley P., Curtis P. D. (2015). Estimating wild tiger (Panthera tigris Linnaeus) abundance and density using a spatially-explicit capture-recapture model in Jigme Dorji National Park, Bhutan. Bhutan J. Natural Resour. Dev. 2 (1), 9. doi: 10.13140/RG.2.2.31715.78888
Thinley P., Dendup T., Rajaratnam R., Vernes K., Tempa K., Chophel T., et al. (2020). Tiger reappearance in Bhutan’s Bumdeling Wildlife Sanctuary: A case for maintaining effective corridors and metapopulations. Anim. Conserv. 23 (6), 629–631. doi: 10.1111/acv.12580
Tracewski Ł., Butchart S. H. M., Di Marco M., Ficetola G. F., Rondinini C., Symes A., et al. (2016). Toward quantification of the impact of 21st-century deforestation on the extinction risk of terrestrial vertebrates. Conserv. Biol. 30, 1070–1079. doi: 10.1111/cobi.12715
Tucker M. A., Böhning-Gaese K., Fagan W. F., Fryxell J. M., Van Moorter B., Alberts S. C., et al. (2018). Moving in the Anthropocene: Global reductions in terrestrial mamMalian movements. Science 359 (6374), 466–469. doi: 10.1126/science.aam9712
Turner W., Rondinini C., Pettorelli N., Mora B., Leidner A. K., Szantoi Z., et al. (2015). Free and open-access satellite data are key to biodiversity conservation. Biol. Conserv. 182, 173–176. doi: 10.1016/j.biocon.2014.11.048
Umariya S., Sylvia C., Chanchani P., Lachenpa P., Lachungpa D., Shrestha P., et al. (2022). Increasing evidence of tiger in North Sikkim, India. CATNews 74, 23–27. URL: https://www.researchgate.net/publication/357735655_Increasing_evidence_of_tiger_in_North_Sikkim_India
UNEP-WCMC and IUCN (2022) Protected Planet: The World Database on Protected Areas (WDPA), January 2022. Cambridge, UK: UNEP-WCMC and IUCN. Available at: www.protectedplanet.net.
United Nations (2015) Sustainable Development Goals. Available at: https://www.un.org/sustainabledevelopment/sustainable-development-goals/.
United Nations Department of Economic and Social Affairs (2022) Sustainable Development Goals. Available at: https://sdgs.un.org/goals (Accessed November 16, 2022).
Vasudeva V., Upgupta S., Singh A., Sherwani N., Dutta S., Rajaraman R., et al. (2022). Conservation prioritization in a tiger landscape: is umbrella species enough? Land 11 (3), 371. doi: 10.3390/land11030371
Vaz A. S., Moreno-Llorca R. A., Gonçalves J. F., Vicente J. R., Méndez P. F., Revilla E., et al. (2020). Digital conservation in biosphere reserves: Earth observations, social media, and nature’s cultural contributions to people. Conserv. Lett. 13, e12704. doi: 10.1111/conl.12704
Venter O., Sanderson E. W., Magrach A., Possingham H. P., Small C., Fekete B. M., et al. (2016). Sixteen years of change in the global terrestrial human footprint and implications for biodiversity conservation. Nat. Commun. 7, 12558. doi: 10.1038/ncomms12558
Vietnam News Agency (2022). 10 years of conservation: How many tigers left in the wild in Vietnam? (Hanoi, Vietnam: VietnamPlus). Available at: https://en.Vietnamplus.vn/10-years-of-conservation-how-many-tigers-left-in-the-wild-in-Vietnam/231530.vnp.
Villalva P., Moracho E. (2019). Tiger trade threatens big cats worldwide. Science 364, 743–743. doi: 10.1126/science.aax5200
Walston J., Karanth U., Stokes E. (2010). Avoiding the unthinkable: What will it cost to prevent tigers becoming extinct in the wild? (Bronx NY: Wildlife Conservation Society).
Wang T., Feng L., Mou P., Wu J., Smith J. L. D., Xiao W., et al. (2016). Amur tigers and leopards returning to China: Direct evidence and a landscape conservation plan. Landscape Ecol. 31 (3), 491–503. doi: 10.1007/s10980-015-0278-1
Wang Y., Liu W., Liu F., Li S., Zhu X., Jiang Z., et al. (2019). Investigation on the population of wild Bengal tiger (Panthera tigris tigris) in Medog, Tibet. Acta Theriologica Sin. 39 (5), 504. doi: 10.16829/j.slxb.150265
WCS Thailand (2020). Lessons Learned: The Recovery of Wild Tigers and other Threatened Wildlife in the Western Forest Complex 2005—2019 (Bronx NY: Wildlife Conservation Society).
Widodo F. A., Imron M. A., Sunarto S., Giordano A. J. (2022). Carnivores and their prey in Sumatra: Occupancy and activity in human-dominated forests. PloS One 17 (3), e0265440. doi: 10.1371/journal.pone.0265440
Wikramanayake E., Dinerstein E., Seidensticker J., Lumpkin S., Pandav B., Shrestha M., et al. (2011). A landscape-based conservation strategy to double the wild tiger population. Conserv. Lett. 4 (3), 219–227. doi: 10.1111/j.1755-263X.2010.00162.x
Williams B. A., Venter O., Allan J. R., Atkinson S. C., Rehbein J. A., Ward M., et al. (2020). Change in terrestrial human footprint drives continued loss of intact ecosystems. One Earth 3 (3), 371–382. doi: 10.1016/j.oneear.2020.08.009
Win T. L. (2022). Environment ignored as Myanmar struggles with coup (Berlin, Germany: Climate Diplomacy). Available at: https://climate-diplomacy.org/magazine/environment/environment-ignored-Myanmar-struggles-coup.
World Bank (2023) Population and GDP (current US$)—East Asia & Pacific. Available at: https://data.worldbank.org/indicator/.
WWF Russia (2022) The tiger reintroduction programme. Available at: https://wwf.ru/en/regions/central-asia/vosstanovlenie-turanskogo-tigra/ (Accessed November 28, 2022).
Xue H.-R., Yamaguchi N., Driscoll C. A., Han Y., Bar-Gal G. K., Zhuang Y., et al. (2015). Genetic Ancestry of the extinct Javan and Bali tigers. J. Heredity 106 (3), 247–257. doi: 10.1093/jhered/esv002
Yackulic C. B., Sanderson E. W., Uriarte M. (2011). Anthropogenic and environmental drivers of modern range loss in large mammals. Proc. Natl. Acad. Sci. 108 (10), 4024–4029. doi: 10.1073/pnas.1015097108
Yadav P. K., Brownlee M. T. J., Kapoor M. (2022). A systematic scoping review of tiger conservation in the Terai Arc Landscape and Himalayas. Oryx 56 (6), 888–896. doi: 10.1017/S0030605322001156
Yang H., Han S., Xie B., Mou P., Kou X., Wang T., et al. (2019). Do prey availability, human disturbance and habitat structure drive the daily activity patterns of Amur tigers (Panthera tigris altaica)? J. Zool. 307 (2), 131–140. doi: 10.1111/jzo.12622
Zhang W., Xu X., Yue B., Hou R., Xie J., Zou Z.-T., et al. (2019). Sorting out the genetic background of the last surviving south China tigers. J. Heredity 110 (6), 641–650. doi: 10.1093/jhered/esz034
Keywords: big cats, data sharing, habitat trend, species monitoring, google earth engine, species conservation landscapes (SCL), sustainable development goals (SDG), convention on biological diversity (CBD)
Citation: Sanderson EW, Miquelle DG, Fisher K, Harihar A, Clark C, Moy J, Potapov P, Robinson N, Royte L, Sampson D, Sanderlin J, Yackulic CB, Belecky M, Breitenmoser U, Breitenmoser-Würsten C, Chanchani P, Chapman S, Deomurari A, Duangchantrasiri S, Facchini E, Gray TNE, Goodrich J, Hunter L, Linkie M, Marthy W, Rasphone A, Roy S, Sittibal D, Tempa T, Umponjan M and Wood K (2023) Range-wide trends in tiger conservation landscapes, 2001 - 2020. Front. Conserv. Sci. 4:1191280. doi: 10.3389/fcosc.2023.1191280
Received: 21 March 2023; Accepted: 18 August 2023;
Published: 08 December 2023.
Edited by:
Vanessa Hull, University of Florida, United StatesReviewed by:
Sudeepto Bhattacharya, Shiv Nadar University, IndiaMinghai Zhang, Northeast Forestry University, China
Copyright © 2023 Sanderson, Miquelle, Fisher, Harihar, Clark, Moy, Potapov, Robinson, Royte, Sampson, Sanderlin, Yackulic, Belecky, Breitenmoser, Breitenmoser-Würsten, Chanchani, Chapman, Deomurari, Duangchantrasiri, Facchini, Gray, Goodrich, Hunter, Linkie, Marthy, Rasphone, Roy, Sittibal, Tempa, Umponjan and Wood. This is an open-access article distributed under the terms of the Creative Commons Attribution License (CC BY). The use, distribution or reproduction in other forums is permitted, provided the original author(s) and the copyright owner(s) are credited and that the original publication in this journal is cited, in accordance with accepted academic practice. No use, distribution or reproduction is permitted which does not comply with these terms.
*Correspondence: Eric W. Sanderson, ZXNhbmRlcnNvbkBueWJnLm9yZw==
†Present addresses: Chris Clark, National Resources Defense Council, San Francisco, CA, United States
Jesse Moy, State University of New York, Purchase, NY, United States
Nathaniel Robinson, The Nature Conservancy, Arlington, VA, United States
Eric W. Sanderson and Lucinda Royte, Urban Conservation Program, The New York Botanical Garden, Bronx, NY, United States