- Molecular Genetics, The Weizmann Institute of Science, Rehovot, Israel
In the era of bioinformatics and high-throughput techniques, it is tempting to forget the advantage of an old yet efficient and straightforward technique, ligand affinity chromatography, in the search for unknown proteins. This type of separation is based on an interaction between the target analyte potentially present in a crude mixture of proteins and a ligand coupled covalently to a resin. This process allows thousands-fold purification in a single step, which is crucial when using an extremely rich source of naturally occurring proteins such as human urine or plasma. Before the completion of The Genome Project, this method facilitated the rapid and reliable cloning of the corresponding gene based on the partial amino acid sequence of the isolated protein. Upon completion of this project, a partial protein sequence was enough to retrieve its complete mRNA and, hence, its complete protein sequence. Ligand affinity chromatography is indispensable for the isolation of both expected and unexpected binding proteins found by serendipity. My approach of combining a rich source of human proteins (1,000-fold concentrated human urine) together with this highly specific isolation method yielded proteins from both groups. The expected proteins included the two receptors for TNF (TBPI and TBPII), type I and type II interferon receptors (IFNα/βR, IFN-γR), and IL-6 and LDL receptors. The unexpected group of proteins included IL-18 binding protein (IL-18BP), IL-32 binding protein (Proteinase 3), and heparanase binding protein, the resistin. The discovery of the type I IFN receptor was a “eureka” moment in my life since it put an end to a 35-year worldwide search for this receptor. Using chemical purification methods, the TBPII might have never been discovered. Years later, TBPII was translated into the blockbuster drug Enbrel® to treat mainly rheumatoid arthritis. IFN-beta was translated into the blockbuster drug Rebif® to treat the autoimmune disease multiple sclerosis. IL-18BP translated into the drug Tadekinig alfa™ and is in a phase III clinical study for inflammatory and autoimmune pathologies. It has saved the lives of children born with mutations (NLRC4, XIAP) and is an example of personalized medicine. COVID-19 and CAR-T cytokine storms are the recent targets of IL-18BP.
Introduction
The ligand affinity chromatography approach aiming at the isolation of soluble receptors and binding proteins requires recombinant ligands in milligram amounts and monoclonal antibodies (mAb) as tools for purification, monitoring, and detection. The generation of monoclonal antibodies is relatively simple nowadays but some 45 years ago it was state-of-the-art science justifying publication in the most prominent scientific journals. I refer to the Kohler and Milstein hybridoma technique for the production of mAbs, for which they were awarded the Nobel prize in 1984. Kohler and Milstein immortalized antibody-producing cells by fusing them with tumor cells, a technique that allowed the unlimited production of mAbs with predetermined specificity (Kohler and Milstein, 1975; Leavy, 2016). Different from traditional hybridoma generation, very advanced molecular biology techniques have since been introduced to amplify the heavy and light chains of the antibody genes and produce them in either bacterial or mammalian systems using recombinant technology such as cloning, CRISPR/Cas9, or phage/yeast displays.
Obviously, in the 1980s and the 1990s, I used the old techniques. It was even more difficult when generating mAbs to biologically active compounds such as interferons and cytokines and their corresponding receptors since those are present as minor components in a complex mixture and also lose easily their biological activity. Thus, collecting enough protein that would suffice for immunization of mice and for screenings was a big issue. To guarantee success and ensure versatile mAbs suitable for binding, neutralization, affinity chromatography, ELISA, FACS, and Western blots, we developed a novel screening procedure. Together with other assays, this new screening assay was successfully employed for the identification of monoclonal antibodies to human IFN-a, IFN-b, IFN-g, IL-6, and TNF. Later, the corresponding mAbs were each coupled to a resin and recombinant forms of the various interferons and cytokines were purified in one step by affinity chromatography (Wilchek and Miron, 1974). A large amount of easily purified recombinant and biologically active interferons and cytokines enabled the discovery and isolation of the corresponding receptors and binding proteins via ligand affinity chromatography (Novick and Rubinstein, 2007).
Note that in those old days, our technology transfer section predicted no market would exist; therefore, none of our mAbs were commercialized.
The tools: Monoclonal antibodies
Monoclonal antibodies to IFN-a (1982)
In the early days of this field, all the steps leading to the successful production of the various mAbs were not less than a “Via Dolorosa”. It was laborious to generate enough antigen for immunizations and no less laborious to work out tailor-made screening procedures. First in a row were mAbs to IFN-a, the human leukocyte interferon (Novick et al., 1982a). To assure success in the generation of versatile mAbs, we developed a novel and unique screening procedure for the reliable identification of hybridomas secreting mAbs. The screening procedure was based on immunoprecipitation; namely, precipitation by a secondary antibody of a mixture of IFN-a incubated with the hybridoma supernatants. The precipitate was then dissociated with acid and the interferon present in the precipitate was tested by an anti-viral bioassay, all performed in 96-well microtiter plates. Each attempt screened 1,000 potential hybridomas. This novel technique guaranteed the selection of both neutralizing and binding mAbs, including those suitable for affinity chromatography of the antigen since this technique is based on a change in pH, either acid or basic, according to the stability of the tested cytokine. These conditions of rescuing the cytokine from the immunoprecipitate resemble the elution step in affinity chromatography. To assure success, two additional screening tests of the hybridomas were employed: 1. A solid-phase radioimmunoassay (sRIA) in which microtiter plates were coated with IFN-a, hybridoma supernatants were added, and positive hybridomas were detected by radioactively labeled secondary antibody. This assay screened for binding antibodies. 2. Neutralizing mAbs were screened using a bioassay based on cell cultures treated with IFN-a and challenged with the virus in the presence or absence of the tested hybridoma supernatants. The readout was a cytopathogenic effect exerted by the virus. Our vast and no-compromise screening approach yielded mAbs suitable for most purposes.
Anecdotally, in 1982, Charles Weissman (University of Zurich), a scientist known worldwide for his research on type I interferon, “ordered” from us a resin coupled to our anti IFN-a mAbs. I coupled 600 mg of anti IFN-α2 mAbs (#74.3) to 50 ml of agarose-polyacryl hydrazide beads (Wilchek and Miron, 1974). This amount is sufficient for the purification of roughly 25 mg of recombinant IFN-α2 in one step. Note that interferon is bioactive in a picogram range. Unfortunately, I never sent this column to Charlie Weismann since the institutions involved did not reach an agreement. Yet there is a silver lining to every cloud. Thus, thanks to my habit of storing everything, 10 years later, in 1992, these same stable beads played a key role in the characterization of the IFNα/β receptor. Yet another anecdote refers to the use of the affinity chromatography purified IFN-a that was further purified to a drug grade and used to treat patients with hairy cell leukemia in Israel.
Monoclonal antibodies to IFN-b (1982)
As described already, a key step in the preparation of specific monoclonal antibodies is the hybridoma screening assay, which must be successfully and satisfactorily practiced on the immunized mice before the generation of mAbs, and must also be adapted for the desired application of the antibodies. For example, high-affinity antibodies should be chosen for the development of sensitive immunoassays such as RIA or an ELISA, while the selection of antibodies that reversibly bind a given antigen is essential for efficient affinity chromatography. Thus, the newly developed assay for the screening of anti IFN-a mAbs that involved the combination of immunoprecipitation with a bioassay also served us well in the screening of hybridomas producing anti IFN-b mAbs (Novick et al., 1982b). First, mice were immunized with a natural IFN-b produced in poly(I):poly(C)-induced primary foreskin fibroblasts and partially purified on Blue Sepharose beads. Neutralizing antibodies were obtained only after seven injections. The spleen cells of the immunized mice were fused with myeloma tumor cells and the same assays as for the screening of the IFN-a specific hybridomas were used. A variety of mAbs was obtained. Aided by these mAbs, we were the first to describe the immunoaffinity chromatography of native and recombinant IFN-b. The pressure I experienced at that time was enormous since our laboratory, as well as a well-known pharma, was waiting for these antibodies, each for its own reasons. Overcoming a few “disasters” such as a sudden stop of mAbs production by several hybridomas, we finally produced a panel of well-performing mAbs. Milligram amounts of antibodies were coupled to a resin and used in the affinity chromatography purification of milligram amounts of a recombinant IFN-b secreted by Chinese hamster ovary (CHO) cell cultures (Chernajovsky, et al., 1984).
Recombinant IFN-b produced by CHO cells deserves a few words of clarification. Contrary to IFN-a, IFN-b is a glycosylated protein; therefore, when translating it into a drug, it was critical to produce a recombinant form of this IFN in mammalian cells to ensure its identity to human IFN-b. Yuti Chernajovsky had this vision and insisted on expressing it in the mammalian CHO cells rather than in E. coli that is imcapable of glycosylating the recombinant protein. Chernajovsky was at that time a PhD student of Prof. Michel Revel, a scientist known worldwide in the IFN field. The outstanding performance of our mAbs in affinity chromatography as well as an easy double mAb ELISA used for monitoring the purification steps and still used to date, were a turning point in the drug discovery process of the Serono (now Merck) and Interpharm companies. Batches of up to 15 mg of IFN-beta produced by the transformed CHO cells were purified repeatedly to homogeneity by affinity chromatography on ca.150 mg of one of our mAbs coupled to 24 ml of resin and reused many times. The assumption that multiple sclerosis (MS) has a viral etiology combined with known antiviral activity to IFN formed the rationale for translating the recombinant IFN-b into a blockbuster drug for the treatment of these patients. The viral etiology theory was abandoned and it was established that patients with MS benefit from the inhibitory effect of IFN-b on Th1 cytokines such as IFN-g and IL-12 (Cohan et al., 2021). Although the final drug, REBIF® (recombinant beta interferon) (Jaber et al., 2007) is chemically purified, our mAbs were indispensable in accelerating the protein characterization and the submission of its file to the FDA. These mAbs enabled high-scale and high yields of a biologically active IFN-b purification concomitant with easy, rapid, and reliable monitoring (sandwich ELISA). Indeed, the purified IFN is identical in size, activity, and immunospecificity to the natural human IFN-beta 1 glycoprotein. I am proud to have played a part in the development of the blockbuster drug REBIF®.
Monoclonal antibodies to IFN-g (1983)
To generate mAbs to type II IFN, mice were repeatedly immunized with a partially purified native IFN-g induced in mononuclear cells by phorbol-12-O-myristate13 acetate and phytohemagglutinin. Following the fusion of spleen cells to myeloma cells, the hybridoma supernatants were screened for the presence of anti IFN-g antibodies using the same three assays as used for the screening for type I IFNs mAbs but with one crucial modification: a basic solution rather than an acidic solution was used for the dissociation of the immunoprecipitate since type II IFN is acid-sensitive. Twenty-nine binding antibodies were obtained, among which many were highly neutralizing, and others were suitable for affinity chromatography, sandwich ELISA, and Western blotting (Novick et al., 1983) thus extending the repertoire of similar mAbs described by others. Milligram amounts of recombinant IFN-g expressed in CHO cells were purified (Mory et al., 1986) on these mAbs and served later in the ligand affinity purification of the cell surface IFN-g receptor (Novick et al., 1987) and its soluble form (Novick et al., 1989a). Notably, anti IFN-g mAbs developed by others translated to Emapalumab® (Novimmune SA), a drug approved in 2018 for primary hemophagocytic lymphohistiocytosis (HLH) (Cheloff and Al-Samkari, 2020). This drug is in clinical studies for COVID-19 to potentially reduce hyperinflammation (a cytokine storm) and respiratory distress in patients with COVID-19.
Monoclonal antibodies to TNF (1985)
Similarly to the interferons, the goal of the generation of mAbs to the natural TNF (named cytotoxin at that time) was mainly to provide a tool for its purification to homogeneity, a step that enabled the ultimate characterization of the cytokine (Hahn et al., 1985). Attempts to apply other isolation methods; e.g., those based on size and charge ended up with a very poor yield plus the loss of TNF biological activity. TNF was not my sandbox but, quoting others, I was instrumental in closely escorting the anti-TNF hybridoma screening procedure that was later defined as crucial. Since TNF binding rather than neutralization proved to be a more sensitive indicator of the presence of antibodies against this protein, the very unique hybridoma screening procedure developed for the screening of mAbs to IFN-g (Novick et al., 1983) was adopted. The glory of acknowledgment rather than authorship was bestowed upon me for this contribution (Hahn et al., 1985). Not many mAbs were obtained, yet one was suitable for immunoaffinity chromatography of TNF and, more importantly, one served as the basis of the various blockbuster drugs later named Remicade, Humira, and others (Monaco et al., 2014) developed for the treatment of Crohn’s disease, rheumatoid arthritis, and other inflammatory diseases.
Monoclonal antibodies to IL-6 (1989)
Having in-house recombinant IL-6 from two sources, E. coli and CHO cells, was an advantage since it increased the chances of the successful generation of mAbs to this cytokine. Thus, mice were immunized with IL-6 from one source (E. coli) while IL-6 from the second source, CHO cells, was used for the screening of hybridomas, an approach that reduced the detection of contaminants and increased the specificity. We finally produced novel, highly performing mAbs suitable for neutralization, affinity chromatography, ELISA, and Western blot (Novick et al., 1989b).
Affinity chromatography of recombinant IL-6 on these mAbs enabled later ligand affinity purification of the soluble IL-6 receptor (Novick et al., 1989a).
Monoclonal antibodies to glutamate oxaloacetate (GOT1) (2016)
Glutamic-oxaloacetic transaminase (also known as aspartate transaminase [AST]) is an enzyme that exists in cytoplasmic (GOT1) and mitochondrial (GOT2) forms and catalyzes the transfer of an α-amino group between aspartate and glutamate. Thus, it is involved in amino acid metabolism. In medicine, GOT is an important indicator of liver damage, plays a crucial role in the growth of malignant gliomas, and serves as a biomarker for acute myocardial infarction, muscle inflammation, and brain trauma. Thus, GOT1 was of potential therapeutic value and was proposed by Prof. Vivian Teichberg to serve as a scavenger of the circulating toxic glutamate (Ruban et al., 2012). Prof. David Mirelman from our institute took it upon himself to fulfill the will of the late Prof. Vivian Teichberg to translate GOT1 into a drug. I was asked to provide the tools and generate the then-non-existing mAbs against GOT1. Mice were immunized with a recombinant GOT1 expressed in E. coli. Spleen cells and the draining lymph nodes of cells these immunized mice were fused with myeloma cells, which guaranteed, as in previous fusions, the generation of many different mAbs. Binding antibodies were screened by a direct ELISA in which the microtiter plates were coated with the immunizing protein. A competitive ELISA was then used for the screening of neutralizing antibodies. mAbs capable of immunoaffinity, double sandwich ELISA, Western blotting, and blocking the enzymatic activity of natural GOT1 were obtained (unpublished data). These mAbs enabled of GOT1 in in vivo studies the monitoring. Preclinical studies with GOT1 including toxicology and pharmacokinetics were initiated in brain trauma and glioblastoma and although the results were promising, the project did not proceed to further clinical studies for commercial reasons.
The discovery of cytokine receptors and binding proteins
The year was 1989. The timing was perfect. The relevant receptors were unknown. We had the tools to uncover them. The tools were a variety of well-performing mAbs (Table 1) as well as the ligands, namely recombinant interferons and cytokines, in milligram amounts. We also had a very rich natural source of putative proteins, concentrated in normal human urine. I thought that the approach of combining this protein source with the very specific purification method, ligand affinity chromatography, would yield the desired soluble receptors. The grounds for such a move had been prepared: in 1987, I applied this approach and used ligand affinity chromatography for the purification of the novel cell-bound IFN-g receptor from yet another natural protein source; namely, huge batches of foreskin fibroblasts (1011 cells/batch) and human placentas (Novick et al., 1987). Urine has an advantage over other natural protein sources since it can be highly concentrated. Fractionation of the concentrated human urine enabled the discovery of expected soluble receptors as well as unexpected binding proteins.
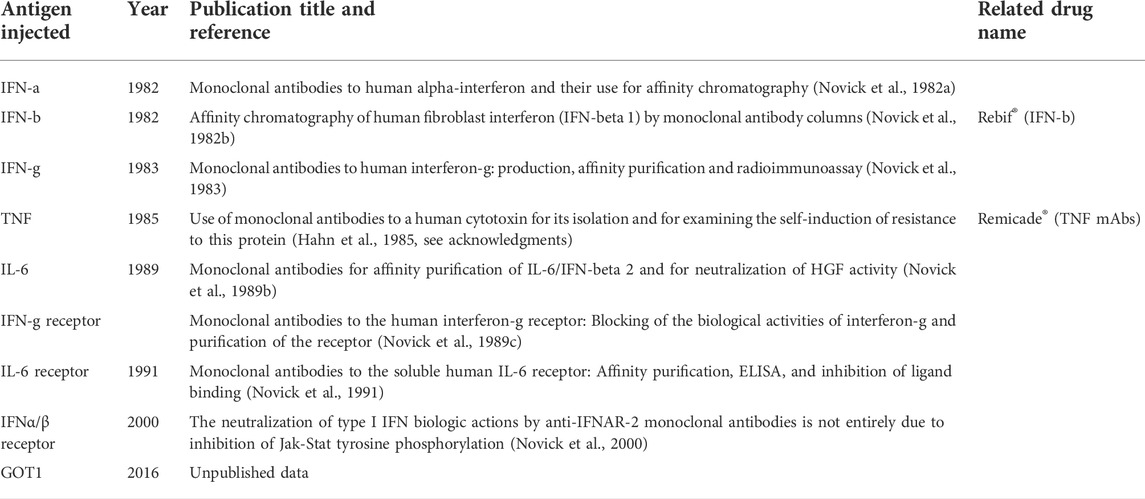
TABLE 1. Monoclonal antibodies generated by Daniela Novick et al. to various human interferons and cytokines and their receptors.
Soluble TNF receptors (1989)
While TNF was discovered in 1975 (Carswell et al., 1975), it took around 15 years to identify its receptors (Engelmann et al., 1990; Balkwill, 2009). Notably, and maybe even crucially, our discovery of TNF inhibitory binding proteins (TBPs) isolated from normal human urine enabled the identification of their cell surface counterparts, TNF receptor I and TNF receptor II (Engelmann et al., 1990), and revealed their roles in health and disease. These discoveries, ours and of others, opened a huge field of research and an avalanche of publications on the mechanism of action of this master cytokine, TNF, and established its involvement both in cell death, resistance to death, and the regulation of immune functions. A modified version of TBPII later launched drug discovery in the form of Etanercept/Enbrel®, a fusion protein with the Fc portion of immunoglobulin, a drug that is prescribed to millions of patients to treat rheumatoid arthritis, psoriasis, and other inflammatory diseases. I am proud to be its originator.
My approach of combining a rich and natural protein source with a very specific purification method, ligand affinity chromatography, was a real shortcut to success. TNF, named cytotoxin at that time, was the sandbox of an adjacent laboratory headed by Dr. Wallach and including two medical doctors (Dr. Med. Hartmut Engelmann and Dr. Dan Aderka). Their collective thought was that TNF is so toxic to the human body that the body most probably protects itself via a natural antidote. They aimed to isolate this antidote but needed help. Though my scientific grounds were prepared (Novick et al., 1987) it was quite brave at that time to secretly “confiscate” from the laboratory of Dr. David Wallach 2.5 mg of the ligand, the entire very precious TNF stock, in order to construct only one ligand affinity column. Wallach’s Ph.D. student, Dr. Med. Hartmut Engelmann, cooperated with me since he was frustrated with the tedious procedure used for the purification of TBPI, its very poor yield and hardly any bioactivity. Engelmann was about to abandon the project and go back to his country; however, I talked him into giving it yet another try, this time using my approach. Thus, my offer to apply the in-house preparation of crude urinary proteins on a TNF column, was perfect timing and made all the difference: a. It was a one-step purification procedure, compared to four inefficient purification steps used beforehand to purify TBPI. b. This column yielded not one TNF binding protein but two, the known TBPI and the unexpected TBPII. They both migrated as a single band with an apparent molecular size of ca. 30,000. Further fractionation by reversed-phase HPLC revealed two bioactive proteins with a protective effect against TNF. The specific activity of the eluted proteins from the ligand affinity column was ca. 20,000-fold higher than that of the loaded crude urinary preparation and with an 84% recovery of the bioactivity. The easy isolation method, the fantastic yield, and the N-terminal sequence of these two bioactive decoy soluble receptors enabled the characterization and cloning of their cell surface counterparts, thus unraveling the biology of TNF, the transcription factors involved in its mechanism of action, the route of receptor shedding, their levels in health and disease screened with the corresponding monoclonal antibodies, and more. TBPII was later translated into the blockbuster drug Enbrel™ for the treatment of rheumatoid arthritis and other pathologies. TBPII would have probably never been discovered by the multistep chromatographic procedure used until then to purify TBPI since the chemistry of TBPII is different from that of TBPI and it does not retain the first column used for the purification of TBPI (Engelmann et al., 1990). TBPII is my first example of serendipity.
Soluble receptors to IL-6 and IFN-g and monoclonal antibodies (1989)
The success of the purification of the TBPs called for “many happy returns”. It was clear to me that this indispensable protein source, human urine, contains additional binding proteins and that this indispensable method of purification, ligand affinity chromatography, could isolate them. Indeed, 1 week later, the flow-through of urinary proteins coming from the TNF column and depleted from the TNF binding proteins yielded two additional soluble receptors (Novick et al., 1989a). I isolated them on covalently coupled homemade recombinant IFN-g and IL-6 preparations. Our in-house panel of mAbs previously generated against the cell surface IFN-gR derived from human placentas (Novick et al., 1989c) revealed that our newly purified IFN-g receptor binding protein was, in fact, a soluble receptor. Similarly, the comparison of the N-terminal sequence (30 amino acids) of the IL-6 binding protein to the N-terminal sequence of a then-newly published IL-6 cell surface receptor (Yamasaki et al., 1988) established its identity as a soluble receptor corresponding to the extracellular portion of its membrane-bound counterpart. It was proposed then that this soluble receptor was most probably generated by alternative splicing rather than proteolytic cleavage (Horiuchi et al., 1994). To our surprise the IL-6 soluble receptor differed from other soluble receptors by being an agonist and not an antagonist (Novick et al., 1992b); however, we did not dare to publish this unexpected agonistic activity in real-time. Therefore, we were scooped. Our work and the work of others aided by mAbs to the soluble IL-6 receptor (Novick et al., 1991) and to the cytokine itself (Novick et al., 1989b) helped to establish IL-6 as a master cytokine in the regulation of hematopoietic and immune systems and demonstrated its involvement in many pro-inflammatory and autoimmune pathologies. Importantly, mAbs to the IL-6 receptor (not ours due to an intellectual property debate) were translated into the drug Tocilizumab, which was approved in 2010 for the treatment of rheumatoid arthritis and in 2021 for severe cases of COVID-19.
Soluble IFNα/βR (IFNAR2, 1992)
Remarkably, type I interferons were discovered in the late 1950s but the identification of their receptors, both soluble and membrane-bound, took some 35 years, until my approach, named by a colleague as “color writing”, came to the rescue. In 1992, it occurred to me that the 10-year-old preparation of Sepharose beads coupled covalently to anti IFN-α monoclonal antibodies, which was meant to be sent to Charles Weismann in Zurich (see under mAbs to IFN-a) was still with me and still active. It occured to me that I could take advantage of the law of mass action; namely, the very high concentration of the antibodies coupled to the beads would compensate for the very low putative binding protein concentration present in the urine sample. Since these antibodies were directed against the ligand, IFN-a, I understood that only cross-linking of radioactively labeled IFN with the putative binding protein present in the urine would enable the visualization of the complex. Therefore, I cross-linked radioactively labeled IFN-α2 to a 1-ml sample of 1,000-fold concentrated human urine (equivalent to 1 liter of urine) in the absence or presence of unlabeled IFN-α2, incubated it with those anti IFN-a monoclonal antibodies coupled to beads (50 μl), and loaded the eluate of these beads onto a gel. I will never forget the autoradiogram of this experiment showing a broad band of a ∼65 kDa complex that turned out to be the soluble type I IFN receptor coupled to its ligand, a band that was competed out with unlabeled IFN (Novick et al., 1992a). I knew at that moment that our protein was not the interferon receptor chain described by Uze in 1990 (Uzé et al., 1990) but rather a different chain, most likely a ligand-binding chain. Indeed, this binding protein proved to be a soluble receptor corresponding to the extracellular portion of its membrane-bound counterpart (later named IFNAR2). This discovery brought to an end a long-sought search for the ligand-binding chain of the cell-surface type I interferon receptor. The findings were published in Cell (“The human interferon α/β receptor: characterization and molecular cloning” (Novick et al., 1994). There are very few “eureka” moments in one’s career. This was one of mine. These findings opened a way to understanding this receptor’s mechanism of action and signaling properties. At the same time, the tyrosine kinase JAK1 was identified as one of the enzymes participating in type I IFN signal transduction; however, a physical link between an IFN receptor and this kinase had not been reported (Velazquez et al., 1992). I was the first to demonstrate this physical association (Novick et al., 1994). Obviously, we generated mAbs against our soluble IFNα/β receptor. Interestingly, the neutralization of type I IFN biological activities could not be entirely explained by the inhibition of JAK-STAT tyrosine phosphorylation, since we obtained antibodies that blocked various biological activities of type I IFN but did not inhibit IFNα/β-induced JAK-STAT tyrosine phosphorylation and ISGF3 complex formation (Novick et al., 2000). Notably, JAK1 inhibitors have been applied as therapeutics in recent years to immune and inflammatory diseases (Schwartz et al., 2017) and also tested in COVID-19, all pointing to the central role of interferon in various pathologies.
Very much intrigued by the type I IFN master cytokine, I was curious to decipher its mechanism of action. I proposed an experiment to uncover the chain of events in the association of the three players, interferon, the ligand binding receptor chain (IFNAR2), and the transducing receptor chain (IFNAR1). The key was utilizing a detergent at various steps of the experiment. Indeed, cross-linking of iodinated interferon to cells followed by immunoprecipitation with specific anti-receptor antibodies, either before the cells were solubilized with a detergent or after such solubilization, provided the answer. In the first scenario, the receptor chains stick together before cross-linking; thus, a large complex of all three components should be obtained following cross-linking. In the second scenario, the detergent treatment causes the receptor chains to fall apart; upon the binding of IFN to one of them, a smaller complex is formed, which can be identified by the corresponding antibodies to each receptor chain. The data indicated that type I IFN binds first to IFNAR2; thus, IFNAR2 is the ligand binding chain. Then IRNAR1, the transducing chain, joins to form a trimeric complex. The ultimate proof was obtained by repeating the experiment with recombinant mouse cells expressing each chain of the human receptor separately or together. Such cells were engineered by a very skilled molecular biologist in our laboratory, Dr. Batya Cohen. We could then state that the association of type I interferon receptor components is most probably ligand-induced (Cohen et al., 1995).
The type I IFN system is extensively studied during the present COVID-19 pandemic. An impaired type I IFN anti-viral response was observed in patients with COVID-19 patients. Big data analysis in a genome-wide association study showed that interferon deficiency is associated with disease severity and that reduced IFNAR2 expression is related to hospitalization (Pathak et al., 2021). Moreover, by sequencing patients’ whole genomes or exomes, inborn errors in type I IFN immunity were identified in adults and children with life-threatening COVID-19 (Zhang et al., 2020; Zhang et al., 2022). In other cohorts of patients severely ill with COVID-19, autoantibodies against type I IFNs were detected (Bastard et al., 2020; Solanich et al., 2021).
Soluble LDL receptor (1993)
In 1993, Dr. Dina Fischer in our laboratory identified an antiviral protein in culture supernatants of IFN-g-induced cells and showed that this protein specifically contributed to the inhibition of vesicular stomatitis virus (VSV) infectivity. Following its isolation and N-terminal sequencing, we were surprised to discover that this protein was a soluble LDL receptor. This unexpected result was worth publication in Science (Fischer et al., 1993). The isolation of this same protein from our normal human urine by immunoaffinity chromatography with anti-LDL receptor mAbs coupled to a resin proved that this in-vitro-identified soluble LDLR was also present in-vivo as yet another normal body constituent. Ten years later, in 2013 we showed that the cell surface LDLR serves as the major cellular entry port of VSV (Finkelshtein et al., 2013). VSV exhibits remarkably robust and pantropic infectivity, mediated by its coat protein, VSV-G. The identification of the LDL receptor as the entry receptor for this virus solved the mystery of its pantropism. Taking advantage of this property, recombinant forms of VSV and VSV-G-pseudotyped viral vectors are being developed for gene therapy, vaccination, and viral oncolysis, as well as in vivo and in vitro gene transduction.
IL-18 binding protein (1997)
Fascinated by our success in discovering soluble receptors, Prof. Charles Dinarello, a scientist known worldwide in the field of IL-1, proposed in 1997 to look for the receptor to yet another member of the IL-1 family, the human pro-inflammatory cytokine, IL-18. IL-18 was initially described in 1989 as IFN-γ inducing factor or IGIF (Nakamura et al., 1989). It is present constitutively in monocytes/macrophages, antigen-presenting cells, and epithelial cells of healthy humans and mice as an inactive precursor and is cleaved into its active form by caspase-1. IL-18 lacks a signal peptide and the involvement of gasdermin recently solved the enigma of its secretion mode (Xia et al., 2021).
The attempt to isolate the IL-18 receptor yielded a surprising result and is my second example of serendipity. I did not isolate the soluble IL-18 receptor but I did discover an IL-18 binding protein (IL-18BP). IL-18BP deviates from the “classical” definition of soluble receptors as it is encoded by a separate gene; thus, does not correspond to the extra-cellular, ligand-binding domain of the IL-18 receptor (Novick et al., 1999). The identification of IL-18BP was not straightforward. The first attempts failed, probably because the recombinant batches of IL-18 used for the ligand affinity chromatography were not fully active. Worldwide competition to identify the IL-18 receptor was tough but, finally, after 1 year of frustration, I isolated an IL-18 binding protein that then was mistakenly thought to be a soluble receptor. Initial N-terminal sequencing of the isolated protein yielded a mixture of two polypeptides, one of which was identified as the low molecular mass peptide defensin. Subtraction of the defensin sequence enabled us to identify five amino acid residues of a novel protein, IL-18BP. The head of our group, Prof. Menachem Rubinstein, a chemist by education, noticed that the second amino acid residue of IL-18BP was proline, the only amino acid that is not blocked by treatment with o-phthalaldehyde (OPA). He suggested treating the sample with OPA after the first sequencing cycle to block the defensin chain, a step that allowed us to read a clean sequence of 40 amino acid residues. A search of the EST database identified IL-18BP as a translation product of an uncharacterized human transcript, interestingly homologous to transcripts encoded by all Poxviruses.
Still believing that we had isolated the soluble IL-18 receptor, Soo-Hyun Kim, a PhD student in our laboratory tried to identify a cDNA encoding a transmembrane version of IL-18BP. Failing to find one, he then sequenced the entire IL-18BP gene and confirmed that it encoded only a secreted protein lacking homology to any trans-membranal protein. Therefore, we concluded that IL-18BP is distinct from the IL-18 receptor and could join osteoprotegerin as the second member of a unique family of binding proteins that are not soluble receptors.
The fact that all known Poxviruses express what we suggested to be viral IL-18BP indicated that these are potent immunosuppressors. Indeed, our further characterization of human IL-18BP established that it is a strong antagonist of IL-18, with a KD of 0.4 nM and an extremely low Koff (Kim et al., 2000). This very low Koff, namely a very slow rate of dissociation, was anticipated before the actual Koff measurements were performed. I noticed that IL-18BP release from the affinity column required at least eight column volumes of a low pH elution buffer (pH 2.2) (Novick et al., 1999), whereas other soluble receptors eluted as expected, as a sharp peak in two column volumes. A more recent publication by others reported even a 10-fold higher affinity of IL-18BP to its ligand (0.05 nM) (Girard et al., 2016). The reported affinity of IL-18 to its ligand-binding cell-surface-receptor chain is 18.5 nM (Wu et al., 2003), 45–370 lower than the affinity of IL-18BP to the same ligand.
IL-18 induces IFN-g. So far, IFN-g is the only known inducer of IL-18BP, as reported in 2002 by our PhD student Vladimir Hurgin (Hurgin et al., 2002). We proposed then that IL-18BP provides a negative feedback mechanism that reduces IL-18-elicited immune responses. Thus, the discovery of this naturally occurring antidote, IL-18BP, brought a new level of understanding of the tight control and regulation of IFN-γ activity in health and disease (Novick et al., 2013).
Some 20 years ago IL-18BP was translated by Ares Serono’s Inc. (now Merck) into the drug Tadekinig alfa™ and was found to be safe in phase I and phase Ib clinical studies (Tak et al., 2006). The indication chosen then, psoriatic arthritis, failed in the sense that the patients did not improve in the short time of this clinical study. However, the external administration of IL-18BP was effective in these patients as determined by the decrease in levels of the IFN-g biomarker, CD8+. Notably, there was no dropout from the clinical study, pointing to the lack of substantial adverse effects. Serono discontinued the project and in 2010 it was sublicensed to AB2 bio Ltd. (Lausanne, Switzerland). At this stage, the indications chosen for Tadekinig alfa™ were adult-onset Still’s disease (AOSD), refractory systemic juvenile idiopathic arthritis (sJIA), recurrent macrophage activation syndrome (MAS) (Gabay et al., 2018; Yasin et al., 2020a), and two devastating pathologies caused by genetic mutations. The latter deserves special attention. In 2014, Canna et al. (2016) described de novo missense gain-of-function mutations through constitutive caspase-1 activation, which affected the nucleotide-binding domain of the inflammasome component, NLRC4. This mutation results in IL-1 and IL-18 overproduction, recurrent fever flares, and MAS. IL-1 blocking did not prove effective enough; thus, the authors proposed IL-18 as a therapeutic target. In 2015, this suggestion proved dramatic when a critically ill baby with multi-organ failure was hospitalized at Philadelphia Children’s Hospital in the US. Whole-exome sequencing of the patient revealed that she carried the NLRC4 gain-of-function mutation. Luckily, Scott Canna, who characterized this mutation, was part of her medical care team. The FDA approved the IL-18BP antidote within hours and AB2 bio Ltd. supplied it instantly. The baby’s life was saved by this unique drug. Her colitis resolved in 3 days, the levels of inflammation markers decreased, and within 3 months she was discharged from the hospital. The girl had been treated with Tadekinig alfa for 7 years now on a compassionate basis, with no adverse effects.
In 2005, we reported severe IL-18 and IL-18BP imbalance in patients with secondary hemophagocytic lymphohistiocytosis (HLH) syndrome (Mazodier et al., 2005). One year later, an X-linked inhibitor of apoptosis (XIAP) deficiency mutation was described and characterized by hyperinflammation and immune dysregulation, which leads to multiple clinical manifestations, including HLH. This mutation results in the overproduction of the inflammasome-activated cytokines, IL-1 and IL-18, leading to life-threatening flares and frequent hospitalizations. Allogenic hematopoietic stem cell transplant is a curative option but is associated with a high risk of mortality. Therapy with IL-1, IL-6, TNF, and CD20 blockade failed, while experimental continuous monotherapy with Tadekinig alfa (rhIL-18BP), ongoing now for several years has proved successful, with no flares and no hospitalizations (Geerlinks et al., 2022; Higuchi et al., 2022). Yet another child was saved and, thanks to continuous compassionate treatment with Tadekinig alfa™, was weaned from all toxic non-specific medications and leads an almost normal life. Tadekinig alfa™ is currently in a phase III clinical trial in patients with pathogenic variants of NLRC4 and XIAP/BIRC4 (ClinicalTrials.gov Identifier: NCT03113760) and is considered for the treatment of patients with MAS for other reasons such as cancer and viral diseases including COVID-19. In addition, Tadekinig alfa™ has shown a favorable safety profile and efficacy in a phase II clinical study in adult-onset Still’s disease (Gabay et al., 2018). IL-18 seems to be a good biomarker in Still’s disease complicated with MAS (Yasin et al., 2020b) and in HLH (Krei et al., 2021); thus, IL-18BP is awaiting phase III clinical studies for these additional indications.
Another mutation concerns an IL-18BP loss-of-function mutation. Belkaya et al. reported a fatal inherited IL-18BP deficiency in human fulminant viral hepatitis A due to a deletion in IL18BP, which leads to dramatically elevated levels of IL-18, uncontrolled IFN-g production, enhanced NK cell activation, and, finally, fatal liver damage. The study concluded that human IL-18 is toxic to the liver and suggested IL-18BP as an antidote (Belkaya et al., 2019).
IL-18, a product of the NLRP3 inflammasome (Sharma and Kanneganti, 2021), can have negative effects e.g. in autoinflammatory diseases but can be a double-edged sword like in cancer (Fabbi et al., 2015). IL-18 in patients with multiple myeloma is deleterious since high IL-18 levels are associated with poor prognosis (Nakamura et al., 2018), pointing to the potential benefit of IL-18BP therapy. In a different microenvironment, IL-18 can have positive effects. As reported by Zhou et al. (2020), IL-18BP is a secreted immune checkpoint and barrier to IL-18 immunotherapy in NSCLC, colorectal cancer, and melanoma. Zhou et al. engineered a recombinant ‘decoy-resistant’ IL-18 (DR-18) that binds IL-18 and transduces the signal but does not bind IL-18BP. The combination of DR-18 and anti-PD-1 therapy produced a synergistic response that resulted in complete colorectal and melanoma tumor regression in mice.
Attempts have been made to improve chimeric antigen receptor T cells (CAR-T) therapy by applying genetic engineering strategies in which cytokines are generated to enhance the tumor-killing ability of CAR T cells. One such candidate is IL-18. Hu et al. (2017) showed that CD19-IL-18-producing CAR-T cells enhanced CAR-T cell proliferation and increased the anti-tumor effect in melanoma mice. Chmielewski and Abken (2017) engineered IL-18-secreting CAR T cells (IL-18 TRUCKs, namely, T cells redirected for universal cytokine-mediated killing) to create a cytotoxic and pro-inflammatory environment in advanced tumors. The IL-18 TRUCK cells improved the survival of mice with advanced pancreatic and lung tumors. Wolfgang Glienke (Glienke et al., 2022) generated IL-18-secreting TRUCKs specifically targeting the tumor antigen GD2, which led to the increased release of pro-inflammatory cytokines and cytolytic mediators and the increased recruitment of monocytes and NK cells. In addition, a team at the University of Pennsylvania is currently conducting a phase I clinical trial (NCT04684563) of co-expressing IL-18 CAR-T cells targeting CD-19 for the treatment of lymphoma.
The other edge of the IL-18 sword is CAR T-cell toxicities resembling secondary HLH/MAS, which occurs in a subset of patients succumbing to life-threatening cytokine release syndrome (CRS). A relatively high incidence of CAR T-cell-associated HLH presenting with highly elevated levels of IL-18 was observed in the CD22 CAR T treatment of relapsing patients or those nonresponsive to CD19 targeted therapies (Lichtenstein et al., 2021). This suggests a disruption in the endogenous feedback mechanism that regulates biologically active IL-18 levels and calls for preemptive strategies to prevent severe CRS. IL-18’s natural antidote, IL-18BP, is a major player in this fine-tuned regulation. Based on its efficacy in monogenic HLH and MAS, Tadekinig alfa™ is a candidate for the treatment of these patients with CRS.
Quite early in the present COVID-19 pandemic, IL-18 was identified as one of the biomarkers in the cytokine storm signature associated with disease severity, a condition described as a highway to hell (Canna and Cron, 2020; Fraser et al., 2020). In the course of the pandemic, the inflammasome was declared to be at the crux of severe disease via prolonged late-stage activation, including excessive cytokine release, damage to the pulmonary endothelium accompanied by infiltration of immune cells, and systemic hypercoagulability (Vora et al., 2021). A recent publication by Sefik et al. (2022) confirmed the role of the inflammasome in severe COVID-19 in general and IL-18 in particular. The authors suggest that the replicating SARS-CoV-2 virus in human macrophages activates the inflammasome and initiates an inflammatory cascade leading to pyroptosis. Therefore, blocking inflammasome activation during the chronic phase of the disease may attenuate the overactive immune-inflammatory response, allowing lung tissue recovery and preventing a transition to fibrosis. Once again, IL-18BP is a good candidate to treat these patients.
Overall, IL-18 is a pleiotropic cytokine involved both in innate and acquired immunity. Although it was originally discovered as a factor that induces IFN-g production by Th1 cells, IL-18 also acts on non-polarized T cells, NK cells, NKT cells, B cells, dendritic cells, and macrophages to produce IFN-g in the presence of IL-12. It induces chemokines and cell-adhesion molecules, stimulates inflammatory cytokine secretion such as IL-1 and TNF-a, and enhances NK-cell cytotoxicity. IL-18 without IL-12 but with IL-2 induces Th2 cytokine production. IL-18 with IL-3 induces mast cells and basophils to produce IL-4 and IL-13. IL-18 is involved in numerous pathologies, host defense, and cancer through the stimulation of NK and CD8 cytotoxicity, macrophage activation, and cytokine induction (Yasuda et al., 2019). No surprise that with such a vast scope of activities, it is tightly regulated.
Information on disease progression, severity, mortality, and survival, as well as the effects of therapy, can be gained by measuring the levels of relevant circulating cytokines. Indeed, we established collaborations with clinicians worldwide to measure the levels of various cytokines and their receptors in pathological conditions. It should be noted that what counts in pathology is the free cytokine. Thus, in any study on the role of a particular cytokine associated with a disease, concurrent measurement of naturally occurring, specific inhibitors of that cytokine is desirable to enable the calculation of the free cytokine. In 2001, aided by an ELISA for the measurement of IL-18BP, I introduced such a calculation of free IL-18 in healthy individuals and patients with sepsis as an example (Novick et al., 2001). The calculation is based on the law of mass action and involves four parameters: the concentrations of total IL-18 and total IL-18BP, 1:1 stoichiometry in the complex of IL-18 and IL-18BP, and a dissociation constant (Kd) of 0.4 nM. Subsequently, the calculation of free IL-18 levels became a part of many clinical studies (Novick et al., 2013; Harel et al., 2022). A specific assay that measures rather than calculates free IL-18 was developed by Girard et al. and AB2 bio (Lausanne, Switzerland) (Girard et al., 2016). The assay is based on the capture of free IL-18 in IL-18BP-coated microtiter plates and detection with non-neutralizing anti-IL-18 antibodies. Although IL-18 is not currently part of the diagnosis criteria, overabundant IL-18 production seems to be a hallmark in certain pathologies characterized by the dysregulation of the balance between IL-18 and IL-18BP (Mazodier et al., 2005; Girard et al., 2016; Weiss et al., 2018; Stone et al., 2022). Thus, elevated circulating free IL-18 levels may serve as a biomarker to identify patients who would most benefit from IL-18-blocking therapy.
What can we learn from IL-18BP knockout mice? In 2015, the groups of Flavell and Elinav showed that the deletion of Il18 or its receptor Il18r1 in mouse intestinal epithelial cells conferred protection from colitis and mucosal damage, while the deletion of Il18bp resulted in severe colitis associated with the loss of mature goblet cells and lethality. They proposed that a strict equilibrium of epithelial IL-18 signaling must be maintained and suggested that IL-18 targeting may prevent the pathologic breakdown of the mucosal barrier in human ulcerative colitis (Nowarski et al., 2015). Others have shown that IL-18BP is required for normal NK cell maturation, abundance, and function, and that its deficiency results in aberrant proportions of NK cell subsets and increased TNF-a production (Harms et al., 2017). In yet another mouse model, upon repeated toll-like receptor 9 stimulation, IL-18BP-knockout mice showed severe MAS manifestations, including increased weight loss, splenomegaly, anemia, thrombocytopenia, hyperferritinemia, and bone marrow hemophagocytosis, as well as elevated circulating free IL-18 levels, higher IFN-g production, and enhanced IFN-g molecular signature. Therefore, an imbalance between IL-18 and its natural inhibitor IL-18BP may lead to severe MAS, thus recapitulating some of the clinical findings of autoinflammatory syndromes such as AOSD and MAS in humans (Girard-Guyonvarc’h et al., 2018).
IL-32 binding protein (2005)
In 2005, using a microarray of the human lung carcinoma A549 cell line transduced with the IL-18 receptor beta chain and induced with IL-18, Kim et al. identified the function of the human natural killer cell transcript 4 (NK4) product and renamed it IL-32 (Kim et al., 2005). Kim showed that IL-32 is a proinflammatory cytokine capable of inducing TNF-a, IL-8, and MIP-2 and of activating nuclear factor-kappa B (NF- kB) and p38 mitogen-activated protein kinase. Seven isoforms of IL-32 were described, among which IL-32-theta is the most active (Shim et al., 2022a). Since its discovery, IL-32 has been shown to be involved in host defense, inflammatory disease, and cancer (Joosten et al., 2013; Shim et al., 2022b). Soo Hyun Kim, our former PhD student who cloned the IL-18BP (now an independent scientist), offered me a collaboration to isolate the putative IL-32 receptor. In an attempt to isolate this receptor, I employed once again affinity chromatography using IL-32a as the ligand and normal human urine as a source of protein. Human IL-32 in milligram amounts, an in-house product of Kim’s laboratory, was the ligand coupled to the resin. I did not identify the receptor; in fact, it remains unknown to date. Instead, I isolated an unexpected high-affinity (KD 1.2–2.65 nM) IL-32 binding protein, the enzyme proteinase 3 (PR3) (Novick et al., 2006). The binding of PR3 to IL-32 was recently confirmed (Liu et al., 2021). Moreover, the involvement of these players was demonstrated in myeloma, in which secreted IL-32 g was shown to act via PR3 to enhance PDL-1 expression (Liu et al., 2022). PR3 is also known as Wegener’s autoantigen and autoantibodies to PR3 are known as anti-neutrophil-cytoplasmic-autoantibodies (ANCA). These antibodies are not only the hallmark of Wegener’s granulomatosis but are also present in systemic and small vessel autoimmune vasculitic diseases. Elevated levels of IL-32 and PR3 have been reported in Wegener’s, also known as granulomatosis with polyangiitis (GPA) (Bae et al., 2012; Krajewska Wojciechowska et al., 2019). Overall, the discovery of IL-32 and its binding protein, PR3, has opened a vast field of research.
Heparanase-binding protein (2013)
I did not rest on my laurels and kept searching for receptors and binding proteins for orphan ligands. In a search for the receptor for the enzyme heparanase (Vlodavsky et al., 2020), I applied affinity chromatography using human heparanase as the ligand and normal human urine as the protein source. I found a very surprising binding protein, resistin. Heparanase binds resistin with high affinity and enhances its biological activity (Novick et al., 2014). Resistin was discovered in 2001 and was then thought to link obesity to diabetes (Steppan et al., 2001). Since then, it became clear that murine resistin differs from the human one. Murine resistin is an adipogen participating in metabolism, while human resistin is a pro-inflammatory cytokine linked to atherosclerosis, heart disease, and cancer (Tripathi et al., 2020). The receptors for heparanase and resistin are not known and the reasons why resistin is a heparanase binding protein remain an enigma, although both are linked to TLR-4, inflammation, metastasis, and vascularization.
Human urine, a natural source of soluble receptors and binding proteins
Before 1989, the only reported soluble cytokine receptor was that of IL-2 (Rubin et al., 1985). My addition of four soluble receptors to this one-item list was convincing proof that urine is an excellent source of naturally occurring proteins and that shedding of these protein from cells’ surface is a general phenomenon that occurs under normal physiological conditions. Luckily, at that time, urine was the source of the main product of Ares Serono, Inc.’s (now Merck) fertility hormone, Pergonal. Serono collected and concentrated urine from nuns in monasteries throughout Italy. Urine reflects the repertoire of proteins present also in the plasma yet has two advantages over plasma: a. It allows concentration by up to 1,000-fold. b. It hardly contains the very abundant proteins present in the plasma, such as immunoglobulins and albumin, since the kidney retains proteins with a molecular mass greater than that of albumin (≤67 kDa). Encouraged by the isolation of four binding proteins (TBPI, TBPII, and soluble receptors to IFN-g and IL-6), I offered Serono to isolate a binding protein to any given ligand. From that point on Serono, supplied us with 500–1,000-fold concentrated human urine batches. Each batch, equivalent to 500–1,000 liter of normal human urine, served for a single cycle of purification of ca. 1 mg of a binding protein to a ligand of choice. Each batch was loaded on the relevant column and, following extensive washes and elution by changing the pH of the buffer, the putative binding protein was obtained. Generally speaking, normal human urine contains soluble receptors and binding proteins in the nanogram range. Pathology might elevate these level up to thousands fold. Concentrating normal human urine 1,000-fold increases the binding protein levels to a microgram range. According to the law of mass action, the high concentration of the ligand coupled to the beads compensates for the low concentration of the binding protein in the urine and guarantees success and high yield in the purification process. Worth noting is that the binding proteins are quite stable; therefore, the same batches of concentrated urine were re-used for many years to isolate novel soluble receptors and binding proteins.
I end this historical review with the reminder that the term “affinity chromatography” was coined in 1968 by Cuatrecasas, Wilchek, and Anfinsen (Cuatrecasas et al., 1968) based on the notion that almost any given biomolecule has an inherent recognition site through which it recognizes a partner molecule. In my research, I have employed a specific case of affinity chromatography; namely, ligand affinity chromatography, which has yielded numerous unknown bioactive receptors and binding proteins, several of which have been translated into drugs.
Concluding remarks
My unique approach of combining a specific and efficient separation method with ligand affinity chromatography, together with a very rich protein source (concentrated human urine) has enabled repeated discoveries of soluble receptors and unexpected binding proteins (Table 2) (Novick and Rubinstein, 2012). The observed prevalence of these proteins in normal human urine suggests their immunoregulatory role, either in the elimination from the body fluids of their corresponding ligands via the kidney or when present in the plasma by modulation of the availability of these ligands in health and disease. The discovery of these cytokine receptors and binding proteins has opened unlimited avenues of basic research and enabled the study of their status in health and disease.
On a personal note, I would like to add that the repeated discoveries of these proteins would not be possible without passion, daring, ignoring criticism by peers, curiosity, ambition to succeed where others had failed, and most importantly hard work. I look back with satisfaction to learn that so many proteins I touched upon were pivotal in the present coronavirus disease 2019 (COVID-19) pandemic, while others have been translated into drugs for the treatment of inflammatory, autoimmune and viral diseases, and cancer. Lastly, I have been privileged to be a part of the translation from bench to bedside and the development of several blockbuster drugs used for the treatment of multiple sclerosis (Rebif®), Crohn’s disease (Remicade®), rheumatoid arthritis (Enbrel®), and the life-saving Tadekinig alfa™. I close by quoting Robert Frost: “Two roads diverged in the woods and I, I took the one less traveled by and that has made all the difference”.
Author contributions
DN is the sole author and conceived and wrote the manuscript.
Acknowledgments
The author is grateful to all members of the lab and collaborators whose names can be found in the text and references.
Conflict of interest
The author declares that the research was conducted in the absence of any commercial or financial relationships that could be construed as a potential conflict of interest.
Publisher’s note
All claims expressed in this article are solely those of the authors and do not necessarily represent those of their affiliated organizations, or those of the publisher, the editors, and the reviewers. Any product that may be evaluated in this article, or claim that may be made by its manufacturer, is not guaranteed or endorsed by the publisher.
Abbreviations
CAR T, chimeric antigen receptor T; IFN, interferon; JAK, just another kinase; GOT, glutamate oxaloacetate; HLH, hemophagocytic lymphohistiocytosis; IL, interleukin; LDL, low-density lipoprotein; mAb, monoclonal antibody; MAS, macrophage activation syndrome; NLRC4, nod-like receptor CARD domain 4; PR3, proteinase 3; TBP, TNF binding protein; XIAP, X-linked inhibitor of apoptosis.
References
Bae, S., Kim, Y. G., Choi, J., Hong, J., Lee, S., Kang, T., et al. (2012). Elevated interleukin-32 expression in granulomatosis with polyangiitis. Rheumatol. Oxf. 51 (11), 1979–1988. doi:10.1093/rheumatology/kes163
Balkwill, F. (2009). Tumour necrosis factor and cancer. Nat. Rev. Cancer 9 (5), 361–371. doi:10.1038/nrc2628
Bastard, P., Rosen, L. B., Zhang, Q., Michailidis, E., Hoffmann, H. H., Zhang, Y., et al. (2020). Autoantibodies against type I IFNs in patients with life-threatening COVID-19. Science 370 (6515), eabd4585. doi:10.1126/science.abd4585
Belkaya, S., Michailidis, E., Korol, C. B., Kabbani, M., Cobat, A., Bastard, P., et al. (2019). Inherited IL-18BP deficiency in human fulminant viral hepatitis. J. Exp. Med. 216 (8), 1777–1790. doi:10.1084/jem.20190669
Canna, S., Girard, C., Malle, L., de Jesus, A., Romberg, N., Kelsen, J., et al. (2016). Life-threatening NLRC4-associated hyperinflammation successfully treated with IL-18 inhibition. J. Allergy Clin. Immunol. 139 (5), 1698–1701. doi:10.1016/j.jaci.2016.10.022
Canna, S. W., and Cron, R. Q. (2020). Highways to hell: Mechanism based management of cytokine storm syndromes. J. Allergy Clin. Immunol. 146 (5), 949–959. doi:10.1016/j.jaci.2020.09.016
Carswell, E., Old, L. J., Kassel, R. L., Green, S., Fiore, N., and Williamson, B. (1975). An endotoxin-induced serum factor that causes necrosis of tumors. Proc. Natl. Acad. Sci. U. S. A. 72 (9), 3666–3670. doi:10.1073/pnas.72.9.3666
Cheloff, A. Z., and Al-Samkari, H. (2020). Emapalumab for the treatment of hemophagocytic lymphohistiocytosis. Drugs Today (Barc) 56 (7), 439–446. doi:10.1358/dot.2020.56.7.3145359
Chernajovsky, Y., Mory, Y., Chen, L., Marks, Z., Novick, D., Rubinstein, M., et al. (1984). Efficient constitutive production of human fibroblast interferon by hamster cells transformed with the IFN-beta 1 gene fused to an SV40 early promoter. DNA 3, 297–308. doi:10.1089/dna.1.1984.3.297
Chmielewski, M., and Abken, H. (2017). CAR T cells releasing IL-18 convert to T-bethigh FoxO1low effectors that exhibit augmented activity against advanced solid tumors. Cell Rep. 21 (11), 3205–3219. doi:10.1016/j.celrep.2017.11.063
Cohan, S., Hendin, B. A., Reder, A. T., Smoot, K., Avila, R., Mendoza, J. P., et al. (2021). Interferons and multiple sclerosis: Lessons from 25 Years of clinical and real-world experience with intramuscular interferon beta-1a (avonex). CNS Drugs 35, 743–767. doi:10.1007/s40263-021-00822-z
Cohen, B., Novick, D., Barak, S., and Rubinstein, M. (1995). Ligand-induced association of the type I interferon receptor components. Mol. Cell. Biol. 15 (8), 4208–4214. doi:10.1128/MCB.15.8.4208
Cuatrecasas, P., Wilchek, M., and Anfinsen, C. B. (1968). Selective enzyme purification by affinity chromatography. affinity chromatography. Proc. Natl. Acad. Sci. U. S. A. 61, 636–643. doi:10.1073/pnas.61.2.636
Engelmann, H., Novick, D., and Wallach, D. (1990). Two tumor necrosis factor-binding proteins purified from human urine. Evidence for immunological cross- reactivity with cell surface tumor necrosis factor receptors. J. Biol. Chem. 265 (3), 1531–1536. doi:10.1016/s0021-9258(19)40049-5
Fabbi, M., Carbotti, G., and Ferrini, S. (2015). Context-dependent role of IL-18 in cancer biology and counter-regulation by IL-18BP. J. Leukoc. Biol. 97 (4), 665–675. doi:10.1189/jlb.5RU0714-360RR
Finkelshtein, D., Werman, A., Novick, D., Barak, S., and Rubinstein, M. (2013). LDL receptor and its family members serve as the cellular receptors for vesicular stomatitis virus. Proc. Natl. Acad. Sci. U. S. A. 110 (18), 7306–7311. doi:10.1073/pnas.1214441110
Fischer, D., Tal, N., Novick, D., Barak, S., and Rubinstein, M. (1993). An antiviral soluble form of the LDL receptor induced by interferon. Science 262 (5131), 250–253. doi:10.1126/science.8211145
Fraser, D. D., Cepinskas, G., Slessarev, M., Martin, C., Daley, M., Miller, M. R., et al. (2020). Inflammation profiling of critically ill coronavirus disease 2019 patients. Crit. Care Explor. 2 (6), e0144. doi:10.1097/CCE.0000000000000144
Gabay, C., Fautrel, B., Rech, J., Spertini, F., Feist, E., Kötter, I., et al. (2018). Open-label, multicentre, dose-escalating phase II clinical trial on the safety and efficacy of tadekinig alfa (IL-18BP) in adult-onset Still's disease. Ann. Rheum. Dis. 77 (6), 840–847. doi:10.1136/annrheumdis-2017-212608
Geerlinks, A. V., and Dvorak, A. M., and XIAP Defciency Treatment Consortium (2022). A case of XIAP deficiency successfully managed with tadekinig alfa (rhIL-18BP). J. Clin. Immunol. 42 (4), 901–903. doi:10.1007/s10875-022-01236-2
Girard, C., Rech, J., Brown, M., Allali, A., Roux-Lombard, P., Spertini, F., et al. (2016). Elevated serum levels of free interleukin-18 in adult-onset Still's disease. Rheumatol. Oxf. 55 (12), 2237–2247. doi:10.1093/rheumatology/kew300
Girard-Guyonvarc’h, C., Palomo, J., Martin, P., Rodriguez, E., Troccaz, S., Palmer, G., et al. (2018). Unopposed IL-18 signaling leads to severe TLR9-induced macrophage activation syndrome in mice. Blood 131 (13), 1430–1441. doi:10.1182/blood-2017-06-789552
Glienke, W., Dragon, A. C., Zimmermann, K., Martyniszyn-Eiben, A., Mertens, M., Abken, H., et al. (2022). GMP-compliant manufacturing of TRUCKs: CAR T cells targeting GD2 and releasing inducible IL-18. Front. Immunol. 13, 839783. doi:10.3389/fimmu.2022.839783
Hahn, T., Toker, L., Budilovsky, S., Aderka, D., Eshhar, Z., and Wallach, D. (1985). Use of monoclonal antibodies to a human cytotoxin for its isolation and for examining the self-induction of resistance to this protein. Proc. Natl. Acad. Sci. U. S. A. 82 (11), 3814–3818. doi:10.1073/pnas.82.11.3814
Harel, M., Fauteux-Daniel, S., Girard-Guyonvarc’h, C., and Gabay, C. (2022). Balance between Interleukin-18 and Interleukin-18 binding protein in auto-inflammatory diseases. Cytokine 150, 155781. doi:10.1016/j.cyto.2021.155781
Harms, R., Creer, A. J., Lorenzo-Arteaga, K. M., Ostlund, K. R., and Sarvetnick, N. E. (2017). Interleukin (IL)-18 binding protein deficiency disrupts natural killer cell maturation and diminishes circulating IL-18. Front. Immunol. 8, 1020. doi:10.3389/fimmu.2017.01020
Higuchi, T., Izawa, K., Miyamoto, T., Honda, Y., Nishiyama, A., Shimizu, M., et al. (2022). An efficient diagnosis: A patient with X-linked inhibitor of apoptosis protein (XIAP) deficiency in the setting of infantile hemophagocytic lymphohistiocytosis was diagnosed using high serum interleukin-18 combined with common laboratory parameters. Pediatr. Blood Cancer 69 (8), e29606. doi:10.1002/pbc.29606
Horiuchi, S., Koyanagi, Y., Zhou, Y., Miyamoto, H., Tanaka, Y., Waki, M., et al. (1994). Soluble interleukin-6 receptors released from T cell or granulocyte/macrophage cell lines and human peripheral blood mononuclear cells are generated through an alternative splicing mechanism. Eur. J. Immunol. 24 (8), 1945–1948. doi:10.1002/eji.1830240837
Hu, B., Ren, J., Luo, Y., Keith, B., Young, R. M., Scholler, J., et al. (2017). Augmentation of antitumor immunity by human and mouse car T cells secreting IL-18. Cell Rep. 20 (13), 3025–3033. doi:10.1016/j.celrep.2017.09.002
Hurgin, V., Novick, D., and Rubinstein, M. (2002). The promoter of IL-18 binding protein: Activation by an IFN-gamma -induced complex of IFN regulatory factor 1 and CCAAT/enhancer binding protein beta. Proc. Natl. Acad. Sci. U. S. A. 99 (26), 16957–16962. doi:10.1073/pnas.262663399
Jaber, A. D., R., Giovannoni, G., Schellekens, H., Simsarian, J., and Antonelli, M. (2007). The Rebif® new formulation story. Drugs R D 8 (6), 335–348. doi:10.2165/00126839-200708060-00002
Joosten, L., Heinhuis, B., Netea, M. G., and Dinarello, C. A. (2013). Novel insights into the biology of interleukin-32. Cell. Mol. Life Sci. 70 (20), 3883–3892. doi:10.1007/s00018-013-1301-9
Kim, S., Eisenstein, M., Reznikov, L., Fantuzzi, G., Novick, D., Rubinstein, M., et al. (2000). Structural requirements of six naturally occurring isoforms of the IL-18 binding protein to inhibit IL-18. Proc. Natl. Acad. Sci. U. S. A. 97 (3), 1190–1195. doi:10.1073/pnas.97.3.1190
Kim, S., Han, S. Y., Azam, T., Yoon, D. Y., and Dinarello, C. A. (2005). Interleukin-32: A cytokine and inducer of TNFalpha. Immunity 22 (1), 131–142. doi:10.1016/j.immuni.2004.12.003
Kohler, G., and Milstein, C. (1975). Continuous cultures of fused cells secreting antibody of predefined specificity. Nature 256, 495–497. doi:10.1038/256495a0
Krajewska Wojciechowska, J., Kościelska-Kasprzak, K., Krajewski, W., and Morawski, K. (2019). Serum levels of interleukin-32 and interleukin-6 in granulomatosis with polyangiitis and microscopic polyangiitis: Association with clinical and biochemical findings. Eur. Cytokine Netw. 30 (4), 151–159. doi:10.1684/ecn.2019.0439
Krei, J., Møller, H. J., and Larsen, J. B. (2021). The role of interleukin-18 in the diagnosis and monitoring of hemophagocytic lymphohistiocytosis/macrophage activation syndrome - a systematic review. Clin. Exp. Immunol. 203(2), 174–182. doi:doi:10.1111/cei.13543
Lichtenstein, D., Schischlik, F., Shao, L., Steinberg, S. M., Yates, B., Wang, H. W., et al. (2021). Characterization of HLH-like manifestations as a CRS variant in patients receiving CD22 CAR T cells. Blood 138 (24), 2469–2484. doi:10.1182/blood.2021011898
Liu, D., Li, Q., Ding, H., Zhao, G., Wang, Z., Cao, C., et al. (2021). Placenta-derived IL-32β activates neutrophils to promote preeclampsia development. Cell. Mol. Immunol. 18 (4), 979–991. doi:10.1038/s41423-021-00636-5
Liu, Y., Yan, H., Gu, H., Zhang, E., He, J., Cao, W., et al. (2022). Myeloma-derived IL-32γ induced PD-L1 expression in macrophages facilitates immune escape via the PFKFB3-JAK1 axis. Oncoimmunology 11 (1), 2057837. doi:10.1080/2162402X.2022.2057837
Mazodier, K., Marin, V., Novick, D., Farnarier, C., Robitail, S., Schleinitz, N., et al. (2005). Severe imbalance of IL-18/IL-18BP in patients with secondary hemophagocytic syndrome. Blood 106 (10), 3483–3489. doi:10.1182/blood-2005-05-1980
Monaco, C., Nanchahal, J., Taylor, P., and Feldmann, M. (2014). Anti-TNF therapy: Past, present and future. Int. Immunol. 27 (1), 55–62. doi:10.1093/intimm/dxu102
Mory, Y., Ben-Barak, J., Segev, D., Cohen, B., Novick, D., Fischer, D. G., et al. (1986). Efficient constitutive production of human IFN-gamma in Chinese hamster ovary cells. DNA 5 (3), 181–193. doi:10.1089/dna.1986.5.181
Nakamura, K., Kassem, S., Cleynen, A., Chrétien, M. L., Guillerey, C., Putz, E. M., et al. (2018). Dysregulated IL-18 is a key driver of immunosuppression and a possible therapeutic target in the multiple myeloma microenvironment. Cancer Cell 33 (4), 634–648. doi:10.1016/j.ccell.2018.02.007
Nakamura, K., Okamura, H., Wada, M., Nagata, K., and Tamura, T. (1989). Endotoxin- induced serum factor that stimulates gamma interferon production. Infect. Immun. 57 (2), 590–595. doi:10.1128/iai.57.2.590-595.1989
Novick, D., Barak, S., Ilan, N., and Vlodavsky, I. (2014). Heparanase interacts with resistin and augments its activity. Plos One 9 (1), e85944. doi:10.1371/journal.pone.0085944
Novick, D., Cohen, B., and Rubinstein, M. (1992a). Soluble interferon-alpha receptor molecules are present in body fluids. FEBS Lett. 314 (3), 445–448. doi:10.1016/0014-5793(92)81523-o
Novick, D., Cohen, B., and Rubinstein, M. (1994). The human interferon alpha/beta receptor: Characterization and molecular cloning. Cell 77 (3), 391–400. doi:10.1016/0092-8674(94)90154-6
Novick, D., Engelmann, H., Revel, M., Leitner, O., and Rubinstein, M. (1991). Monoclonal antibodies to the soluble human IL-6 receptor: Affinity purification, ELISA, and inhibition of ligand binding. Hybridoma 10 (1), 137–146. doi:10.1089/hyb.1991.10.137
Novick, D., Engelmann, H., Wallach, D., and Rubinstein, M. (1989a). Soluble cytokine receptors are present in normal human urine. J. Exp. Med. 170 (4), 1409–1414. doi:10.1084/jem.170.4.1409
Novick, D., Eshhar, Z., Fischer, D. G., Friedlander, J., and Rubinstein, M. (1983). Monoclonal antibodies to human interferon-gamma: Production, affinity purification and radioimmunoassay. Embo J. 2 (9), 1527–1530. doi:10.1002/j.1460-2075.1983.tb01618.x
Novick, D., Eshhar, Z., Gigi, O., Marks, Z., Revel, M., and Rubinstein, M. (1982b). Affinity chromatography of human fibroblast interferon (IFN-beta 1) by monoclonal antibody columns. J. Gen. Virol. 64, 905–910. doi:10.1099/0022-1317-64-4-905
Novick, D., Eshhar, Z., Revel, M., and Mory, Y. (1989b). Monoclonal antibodies for affinity purification of IL-6/IFN- beta 2 and for neutralization of HGF activity. Hybridoma 8 (5), 561–567. doi:10.1089/hyb.1989.8.561
Novick, D., Eshhar, Z., and Rubinstein, M. (1982a). Monoclonal antibodies to human alpha-interferon and their use for affinity chromatography. J. Immunol. 129, 2244–2247.
Novick, D., Fischer, D., Reiter, Z., Eshhar, Z., and Rubinstein, M. (1989c). Monoclonal antibodies to the human interferon-gamma receptor: Blocking of the biological activities of interferon-gamma and purification of the receptor. J. Interferon Res. 9 (3), 315–328. doi:10.1089/jir.1989.9.315
Novick, D., Kim, S. H., Fantuzzi, G., Reznikov, L. L., Dinarello, C. A., and Rubinstein, M. (1999). Interleukin-18 binding protein: A novel modulator of the Th1 cytokine response. Immunity 10 (1), 127–136. doi:10.1016/s1074-7613(00)80013-8
Novick, D., Kim, S., Kaplanski, G., and Dinarello, C. A. (2013). Interleukin-18, more than a Th1 cytokine. Semin. Immunol. 25 (6), 439–448. doi:10.1016/j.smim.2013.10.014
Novick, D., Nabioullin, R. R., Ragsdale, W., McKenna, S., Weiser, W., Garone, L., et al. (2000). The neutralization of type I IFN biologic actions by anti-IFNAR-2 monoclonal antibodies is not entirely due to inhibition of Jak-Stat tyrosine phosphorylation. J. Interferon Cytokine Res. 20 (11), 971–982. doi:10.1089/10799900050198417
Novick, D., Orchansky, P., Revel, M., and Rubinstein, M. (1987). The human interferon-gamma receptor. Purification, characterization, and preparation of antibodies. J. Biol. Chem. 262, 8483–8487. doi:10.1016/s0021-9258(18)47439-x
Novick, D., Rubinstein, M., Azam, T., Rabinkov, A., Dinarello, C. A., and Kim, S. H. (2006). Proteinase 3 is an IL-32 binding protein. Proc. Natl. Acad. Sci. U. S. A. 103, 3316–3321. doi:10.1073/pnas.0511206103
Novick, D., and Rubinstein, M. (2012). Ligand affinity chromatography, an indispensable method for the purification of soluble cytokine receptors and binding proteins. Methods Mol. Biol. 820, 195–214. doi:10.1007/978-1-61779-439-1_12
Novick, D., and Rubinstein, M. (2007). The tale of soluble receptors and binding proteins: From bench to bedside. Cytokine Growth Factor Rev. 18, 525–533. doi:10.1016/j.cytogfr.2007.06.024
Novick, D., Schwartsburd, B., Pinkus, R., Suissa, D., Belzer, I., Sthoeger, Z., et al. (2001). A novel IL-18BP ELISA shows elevated serum IL-18BP in sepsis and extensive decrease of free IL-18. Cytokine 14 (6), 334–342. doi:10.1006/cyto.2001.0914
Novick, D., Shulman, L. M., Chen, L., and Revel, M. (1992b). Enhancement of interleukin 6 cytostatic effect on human breast carcinoma cells by soluble IL-6 receptor from urine and reversion by monoclonal antibody. Cytokine 4 (1), 6–11. doi:10.1016/1043-4666(92)90029-q
Nowarski, R., Jackson, R., Gagliani, N., de Zoete, M. R., Palm, N. W., Bailis, W., et al. (2015). Epithelial IL-18 equilibrium controls barrier function in colitis. Cell 163 (6), 1444–1456. doi:10.1016/j.cell.2015.10.072
Pathak, G., Singh, K., Miller-Fleming, T. W., Wendt, F. R., Ehsan, N., Hou, K., et al. (2021). Integrative genomic analyses identify susceptibility genes underlying COVID-19 hospitalization. Nat. Commun. 12 (1), 4569. doi:10.1038/s41467-021-24824-z
Ruban, A., Berkutzki, T., Cooper, I., Mohar, B., and Teichberg, V. I. (2012). Blood glutamate scavengers prolong the survival of rats and mice with brain-implanted gliomas. Invest. New Drugs 30 (6), 2226–2235. doi:10.1007/s10637-012-9799-5
Rubin, L., Kurman, C. C., Fritz, M. E., Biddison, W. E., Boutin, B., Yarchoan, R., et al. (1985). Soluble interleukin 2 receptors are released from activated human lymphoid cells in vitro. J. Immunol. 135 (5), 3172–3177.
Schwartz, D., Kanno, Y., Villarino, A., Ward, M., Gadina, M., and O'Shea, J. J. (2017). JAK inhibition as a therapeutic strategy for immune and inflammatory diseases. Nat. Rev. Drug Discov. 16 (12), 843–862. doi:10.1038/nrd.2017.201
Sefik, E., Qu, R., Junqueira, C., Kaffe, E., Mirza, H., Zhao, J., et al. (2022). Inflammasome activation in infected macrophages drives COVID-19 pathology. Nature 606 (7914), 585–593. doi:10.1038/s41586-022-04802-1
Sharma, B. R., and Kanneganti, T. D. (2021). NLRP3 inflammasome in cancer and metabolic diseases. Nat. Immunol. 22 (5), 550–559. doi:10.1038/s41590-021-00886-5
Shim, S., Lee, S., Hisham, Y., Kim, S., Nguyen, T. T., Taitt, A. S., et al. (2022b). A paradoxical effect of interleukin-32 isoforms on cancer. Front. Immunol. 13, 837590. doi:10.3389/fimmu.2022.837590
Shim, S., Lee, S., Hisham, Y., Kim, S., Nguyen, T. T., Taitt, A. S., et al. (2022a). Comparison of the seven interleukin-32 isoforms' biological activities: IL-32θ possesses the most dominant biological activity. Front. Immunol. 13, 837588. doi:10.3389/fimmu.2022.837588
Solanich, X., Rigo-Bonnin, R., Gumucio, V. D., Bastard, P., Rosain, J., Philippot, Q., et al. (2021). Pre-existing autoantibodies neutralizing high concentrations of type I interferons in almost 10% of COVID-19 patients admitted to intensive care in barcelona. J. Clin. Immunol. 41 (8), 1733–1744. doi:10.1007/s10875-021-01136-x
Steppan, C., Bailey, S. T., Bhat, S., Brown, E. J., Banerjee, R. R., Wright, C. M., et al. (2001). The hormone resistin links obesity to diabetes. Nature 409 (6818), 307–312. doi:10.1038/35053000
Stone, D., Ombrello, A., Arostegui, J. I., Schneider, C., Dang, V., de Jesus, A., et al. (2022). Excess serum interleukin-18 distinguishes patients with pathogenic mutations in PSTPIP1. Arthritis Rheumatol. 74 (2), 353–357. doi:10.1002/art.41976
Tak, P., Bacchi, M., and Bertolino, M. (2006). Pharmacokinetics of IL-18 binding protein in healthy volunteers and subjects with rheumatoid arthritis or plaque psoriasis. Eur. J. Drug Metab. Pharmacokinet. 31 (2), 109–116. doi:10.1007/BF03191127
Tripathi, D., Kant, S., Pandey, S., and Ehtesham, N. Z. (2020). Resistin in metabolism, inflammation, and disease. FEBS J. 287 (15), 3141–3149. doi:10.1111/febs.15322
Uzé, G., Lutfalla, G., and Gresser, I. (1990). Genetic transfer of a functional human interferon alpha receptor into mouse cells: Cloning and expression of its cDNA. Cell 60 (2), 225–234. doi:10.1016/0092-8674(90)90738-z
Velazquez, L., Fellous, M., Stark, G. R., and Pellegrini, S. (1992). A protein tyrosine kinase in the interferon alpha/beta signaling pathway. Cell 70 (2), 313–322. doi:10.1016/0092-8674(92)90105-l
Vlodavsky, I., Ilan, N., and Sanderson, R. D. (2020). Forty years of basic and translational heparanase research. Adv. Exp. Med. Biol. 1221, 3–59. doi:10.1007/978-3-030-34521-1_1
Vora, S., Lieberman, J., and Wu, H. (2021). Inflammasome activation at the crux of severe COVID-19. Nat. Rev. Immunol. 21 (11), 694–703. doi:10.1038/s41577-021-00588-x
Weiss, E. S., Girard-Guyonvarc’h, C., Holzinger, D., de Jesus, A. A., Tariq, Z., Picarsic, J., et al. (2018). Interleukin-18 diagnostically distinguishes and pathogenically promotes human and murine macrophage activation syndrome. Blood 131 (13), 1442–1455. doi:10.1182/blood-2017-12-820852
Wilchek, M., and Miron, T. (1974). Polymers coupled to agarose as stable and high capacity spacers. Methods Enzymol. 34, 72–76. doi:10.1016/s0076-6879(74)34008-6
Wu, C., Sakorafas, P., Miller, R., McCarthy, D., Scesney, S., Dixon, R., et al. (2003). IL-18 receptor β-induced changes in the presentation of IL-18 binding sites affect ligand binding and signal transduction. J. Immunol. 170 (11), 5571–5577. doi:10.4049/jimmunol.170.11.5571
Xia, S., Zhang, Z., Magupalli, V. G., Pablo, J. L., Dong, Y., Vora, S. M., et al. (2021). Gasdermin D pore structure reveals preferential release of mature interleukin-1. Nature 593 (7860), 607–611. doi:10.1038/s41586-021-03478-3
Yamasaki, K., Taga, T., Hirata, Y., Yawata, H., Kawanishi, Y., Seed, B., et al. (1988). Cloning and expression of the human interleukin-6 (BSF-2/IFN beta 2) receptor. Science 241 (4867), 825–828. doi:10.1126/science.3136546
Yasin, S., Fall, N., Brown, R. A., Henderlight, M., Canna, S. W., Girard-Guyonvarc’h, C., et al. (2020a). IL-18 as a biomarker linking systemic juvenile idiopathic arthritis and macrophage activation syndrome. Rheumatol. Oxf. 59 (2), 361–366. doi:10.1093/rheumatology/kez282
Yasin, S., Solomon, K., Canna, S. W., Girard-Guyonvarc'h, C., Gabay, C., Schiffrin, E., et al. (2020b). IL-18 as therapeutic target in a patient with resistant systemic juvenile idiopathic arthritis and recurrent macrophage activation syndrome. Rheumatol. Oxf. 59 (2), 442–445. doi:10.1093/rheumatology/kez284
Yasuda, K., Nakanishi, K., and Tsutsui, H. (2019). Interleukin-18 in health and disease. Int. J. Mol. Sci. 20 (3), 649. doi:10.3390/ijms20030649
Zhang, Q., Bastard, P., Liu, Z., Le Pen, J., Moncada-Velez, M., Chen, J., et al. (2020). Inborn errors of type I IFN immunity in patients with life-threatening COVID-19. Science 370 (6515), eabd4570. doi:10.1126/science.abd4570
Zhang, Q., Matuozzo, D., Le Pen, J., Lee, D., Moens, L., Asano, T., et al. (2022). Recessive inborn errors of type I IFN immunity in children with COVID-19 pneumonia. J. Exp. Med. 219 (8), e20220131. doi:10.1084/jem.20220131
Keywords: ligand affinity chromatography, IL-18BP, TBPII, IFNAR2, Rebif®, Enbrel®, Tadekinig alfa™, COVID 19
Citation: Novick D (2022) Nine receptors and binding proteins, four drugs, and one woman: Historical and personal perspectives. Front. Drug. Discov. 2:1001487. doi: 10.3389/fddsv.2022.1001487
Received: 23 July 2022; Accepted: 28 September 2022;
Published: 21 October 2022.
Edited by:
Jamie Berta Spangler, Johns Hopkins University, United StatesReviewed by:
Diana Boraschi, Shenzhen Institute of Advanced Technology (CAS), ChinaA. S. Kamalanathan, VIT University, India
Copyright © 2022 Novick. This is an open-access article distributed under the terms of the Creative Commons Attribution License (CC BY). The use, distribution or reproduction in other forums is permitted, provided the original author(s) and the copyright owner(s) are credited and that the original publication in this journal is cited, in accordance with accepted academic practice. No use, distribution or reproduction is permitted which does not comply with these terms.
*Correspondence: Daniela Novick, daniela.novick@weizmann.ac.il